Abstract
In vivo gene knockout studies in mice have revealed essential roles of the mitogen-activated protein kinases (MAPKs) in embryogenesis, but due to early lethality of the knockout embryos, the underlying mechanisms and specific developmental programs regulated by the MAPK pathways have remained largely unknown. In vitro differentiation of mouse embryonic stem cells (ESCs) have opened new possibilities for understanding lineage segregation and gene function in the developmental stages that are not normally accessible in vivo. Building on this technology, in combination with gene knockout cells, we investigated the roles of MKK4 and MKK7, two upstream kinases of the MAPKs, in early lineage specification. Our results show that MKK4 and MKK7 differentially regulate the JNK and p38 MAPKs and make distinct contributions to differentiation programs. In vitro ESC differentiation is a valuable system to investigate the molecular and signaling mechanisms of early embryogenesis.
The MKK4 and MKK7 are Upstream Kinases of the MAPKs
The MAPKs are intracellular enzymes activated by extracellular cues and in turn phosphorylate effector proteins to modulate cellular activity and function. In mammalian system, there are three major MAPK subgroups, including the Jun N-terminal kinase (JNK), the p38s and the extracellular signal regulated kinases (ERK).Citation1 The MAPKs are activated through phosphorylation on Thr and Tyr residues within the activation loop of the kinase domain. There are six major MAP2Ks responsible for MAPK phosphorylation, but each has selective substrate specificities.Citation2 In principle, the MEK1 and MEK2 are MAP2Ks upstream of the ERKs, the MKK3 and MKK6 are responsible for p38 activation, while the MKK4 and MKK7 preferentially activate the JNKs, and MKK4 has also been shown to activate the p38s ().Citation3,Citation4 Extracellular and intracellular stimuli, including peptide growth factors, cytokines, hormones, and various cellular stressors such as oxidative stress and endoplasmic reticulum stress, can activate the MAP2K-MAPK cascades, which in turn affect diverse cellular activities, such as proliferation, differentiation, survival and death.Citation5
The Roles of MKK4 and MKK7 in Mouse Embryonic Development
Signal transduction pathways are integral components of the developmental network that guides differentiation and cell fate determination. In vivo studies show that MKK4 and MKK7 have essential roles in embryonic development. Genetic ablation of Mkk4 in mice leads to lethality between embryonic day 10.5 (E10.5) and E12.5, and the knockout embryos display severe anemia, abnormal hepatogenesis and liver cell apoptosis.Citation6-Citation8 Genetic ablation of Mkk7 also leads to embryonic lethality, but in this case, the knockout embryos die between E11.5 and E13.5 due to disorganized liver and decreased hepatocyte proliferation.Citation9 Hence, MKK4 and MKK7 are both essential for embryonic survival, but they make different contributions to the developmental programs, so that MKK4 cannot compensate for loss of MKK7, and vice versa.
At an earlier developmental stage, however, MKK4 and MKK7 display some degree of redundancy for embryonic survival. While neither the Mkk4(-/-) nor the Mkk7(-/-) embryos die before E9.5, the Mkk4(-/-)Mkk7(-/-) double mutant mice do not survive beyond this point.Citation10 Based on the time of death, it is proposed that MKK4 and MKK7 are required for mammalian body plan organization. Toward this function, MKK4 makes a greater contribution than MKK7, because the Mkk7(+/+) and Mkk7(+/-) fetuses lacking MKK4 die earlier with more severe defects than the Mkk4(+/+) and Mkk4(+/-) fetuses lacking MKK7. The in vivo data together suggest that MKK4 and MKK7 have distinct and redundant roles in development, but the underlying mechanisms have remained largely unknown.
The Mechanisms of MKK4 and MKK7 in Embryogenesis
The distinct roles of MKK4 and MKK7 in embryogenesis may be attributed in part to their unique tissue distribution.Citation11 The MKK7 is ubiquitously expressed in the developing and adult tissues, with a gradual increased expression in selective sites, such as hair follicle, skin and brain at a later stage of embryogenesis.Citation12 The MKK4, on the other hand, displays a dynamic temporal spatial expression in embryogenesis. Specifically, strong MKK4 expression is detected in the central nervous system, liver and thymus at early stages of development. While expression in fetal liver and thymus gradually decreases as embryogenesis proceeds, expression in nervous system increases over time throughout postnatal development and remains at a stable level in adult brain.Citation13 The expression pattern provides anatomical basis for specific roles of MKK4 in hepatogenesis and neurogenesis during development and in adult brain. Specific Mkk4 gene ablation in the neuronal lineage, for example, causes severe neurological defects and premature death due to misalignment of the Purkinje cells in the cerebellum and radial migration in the cerebral cortex.Citation14
The biological activities of MKK4 and MKK7 are likely mediated by their downstream MAPKs, specifically, JNK and p38Citation2,Citation15-Citation20 MKK4 and MKK7 share 55% amino acid identity within the kinase domains, but they have quite different properties in JNK and p38 phosphorylation. First, MKK4 phosphorylates and activates the p38 MAPKs, but MKK7 does not. Second, while MKK4 and MKK7 both phosphorylate JNK, MKK4 preferentially phosphorylates the Tyr, whereas, MKK7 phosphorylates the Thr at the Thr-X-Tyr site. As the consequence, MKK4 and MKK7 each makes distinct contribution, while both are required for optimal JNK activity.Citation21 Hence, MKK4 and MKK7 may exert distinct effects on embryogenesis through differential activation of JNK and p38.
The mammalian systems have three Jnk genes, of which Jnk1 and Jnk2 are expressed ubiquitously, whereas, Jnk3 is expressed specifically in cells of the neuronal lineages.Citation5,Citation22-Citation24 Neither Jnk1 nor Jnk2 knockout perturbs fetal development; however, knocking out both results in death of the embryos at E11 due to defective neural tube formation and regional reduced apoptosis.Citation25,Citation26 The p38 family has four members, namely, p38α, p38β, p38γ and p38δ. Among these members, only p38α.has displayed an essential role in embryogenesis.Citation27-Citation29 Knockout p38α in mice causes embryonic lethality at E10.5 due to abnormal placenta development and erythropoiesis.Citation30-Citation33 Since none of the MAPK knockout phenotypes resembles that of Mkk4(-/-) or Mkk7(-/-), MKK4 and MKK7 must regulate embryogenesis through complex signaling mechanisms, not simply due to activation of JNK or p38.
In Vitro Embryonic Stem Cells (ESCs) as Surrogates to Understand the Mechanisms of Development
Embryonic development starts with the fertilization of the ovum, followed by rapid mitotic division and differentiation of embryonic stem cells (ESCs) in the inner cell mass. The ESCs first commit to ectoderm, mesoderm and endoderm lineages, followed by more restricted differentiation toward specific fate.Citation34 Ultimately, these processes lead to the generation of over 200 different mammalian cell types that are organized into tissues to provide all the functions required for viability and reproduction.
The ESCs captured from the inner cell mass of pre-implantation embryos can be expanded in vitro for extended periods of time.Citation35 These cells are able to self-renewal and maintain pluripotency in the presence of leukemia inhibitory factors (LIF), but will differentiate in the absence of LIF, giving rise to a broad spectrum of lineages.Citation36,Citation37 The most common in vitro differentiation protocol uses the “hanging drop,” in which the ESCs are forced to aggregate to form embryoid bodies (EBs), mimicking the inner cell mass of the developing embryos ().Citation38-Citation40 The EBs can continue to grow and differentiate spontaneously over time to generate a wide variety of cell types. By tracing lineage- and cell type-specific gene expression, we found that despite the potential of ESCs to produce all cell types, the EB culture produced most abundantly cells of mesodermal lineages, with limited commitment to endodermal and ectodermal lineages (). To extend the application of this system, a number of modified protocols have been developed to direct ESC differentiation along the endodermal and ectodermal lineages, so that the in vitro system can be used to generate a wide range of cell types, such as neuron, epithelium and endothelium.Citation41-Citation47
Figure 2. Schematic diagrams of embryonic stem cell differentiation. (A) Diagrammatic illustration of the early stage of fetal development and the in vivo origin of embryonic stem cells. (B) In vitro ESC differentiation using the “hanging drop” protocol. Differentiation is initiated when the cells are grown in the absence of anti-differentiation factors, such as LIF and fibroblast feeder cells. Approximately 500 cells/20 µl were applied to the lids of tissue culture plate, as hanging drops, to force the cells aggregate to form embryoid bodies (EBs). The EBs can further differentiate in vitro, leading to the generation of cell types of ectoderm, endoderm and mesoderm lineages.
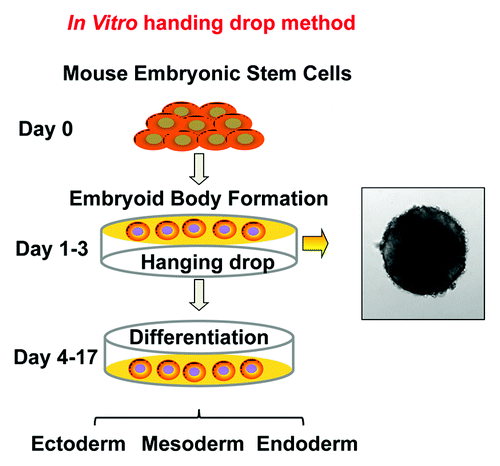
Figure 3. The differentiation profiles of EB. Wild type mouse ESCs were subjected to in vitro differentiation, following the “hanging drop” protocol and the EBs were transferred to a 10 cm2 bacterial plate at approximately 100 EBs per plate. Under this condition, the ESCs spontaneously differentiate into mesoderm, ectoderm and endoderm lineages. RNA was isolated at day 13 of differentiation and lineage specific markers were examined by real time RT-PCR. The most abundantly expressed genes in the EB culture were those for the mesodermal lineage, including genes specific for primitive mesoderm, Fibroblast growth factor 5 (Fgf5), Brachyury and Nodal, for chondrocytes, Collagen 2a2 (Col2a2) and Metalloproteinases 2 (Mmp2), and for myocytes, Myosin light chain (Mlc) and Myosin heavy chain a (Mhca) and Mhcb. Less abundantly expressed were genes for the endodermal lineage, including markers for definitive endoderm Cytokeratin 19 (Ck19) and liver endoderm, such as Forkhead box A2 (Foxa2) and GATA binding protein 4 (Gata4). The least expressed were genes for the ectodermal lineage, including markers for epidermis, such as Fillagrin, Loricrin and Involucrin, for neuronal lineages, such as Notch-1, Tubulin β 3 (Tubb3) and Vimentin. The sequences of forward primers used in PCR are: Gapdh, 5′-AACGACCCCTTC ATTGACC-3′, Ck19, 5′-TCAATGATCGTCTCGCCTCCTACT-3′, Foxa2, 5′-AAGTATGCTGG GAGCCGGAAGAT-3′, Gata4, 5′-CTG TCA TCT CAC TAT GGG CAC-3′, Filaggrin, 5′-GAAACGATATACCTG GAGATGC3′, Loricrin, 5′TGAGGAGACACTAGAATTGGG3′, Involucrin, 5′-GAGAAGCAGCATCAGAAGCC-3′, Notch-1, 5′-TCTGCTTATGCCTCAAGGGAACCA-3′, Tubb3, 5′-TTCTGGTGGACTTGGAACCTG GAA-3′, Vimentin, 5′-CTTTACTCAACTTTCCAGAGCC-3′, Fgf5, 5′-AGAGTGGGCATCGGTTTC-3′, Brachyury, 5′-TGCTGCAGTCCCATGATAACTGGT-3′, Nodal 5′-CCAACCATGCCTACATCCAG-3′, Col2a1, 5′-ATCTGCACTGAATGGCTGACCTGA-3′, Mmp2, 5′- AACCAGCCTTCTCCTTCAC-3′, Mlc, 5′- CTCCAAGAACAAGGACACTG-3′, Mhca, 5′-CTGCTGGAGAGGTTATTCCTC G-3′, Mhcb, 5′-TGCAAAGGCTCCAGGTCTGAGGGC-3′
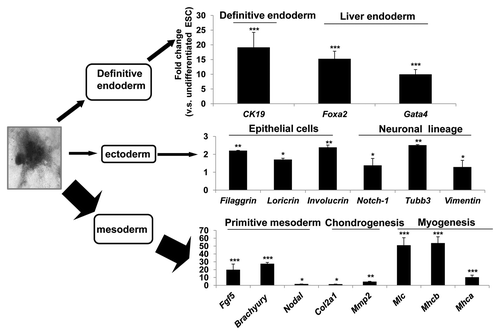
Although the spatial and temporal orchestration of early embryogenesis is missing in cell culture systems, many of the regulatory machineries affecting embryonic development also regulate ESC differentiation in vitro. In contrast to the in vivo studies limited in their ability to attain clear mechanistic insight into the effects of exogenous factors, the in vitro differentiation affords more controlled methods to present morphogens and environmental cues and directly assess differentiated cell phenotypes. An additional advantage is that the in vitro system can be coupled with genetic knockout to study gene functions in early developmental stages that are otherwise not accessible in vivo. For these reasons, the ESCs have emerged as a unique in vitro experimental system for the investigation of basic principles of mammalian cell differentiation and development.
Investigation of the Developmental Roles of MAPKs and MAP2Ks In Vitro
In vitro studies lead to the findings that the MAPKs are important for survival and apoptosis of ESCs in response to various extracellular signals, similar to their well-established functions in stress responses in other cells.Citation48-Citation54 It is also shown that each MAPK plays unique roles in differentiation and lineage specification. The JNKs, for example, are most important for mesodermal differentiation and less important for ectodermal and endodermal differentiation.Citation55 In addition, while JNK is required for induction of neuronal lineage, but inhibition of epithelial lineage by retinoic acid, a function likely mediated through suppression of the Wnt-4/Wnt-6 and BMP signaling pathways,Citation56-Citation58 it can also be activated by Wnt, leading to accelerated cardiac myocyte differentiation.Citation59 The p38, on the other hand, is essential for commitment of mouse ESCs into mesodermal lineages that lead to cardiac, endothelial, smooth muscle, and skeletal muscle differentiation, but inhibits early neural differentiation.Citation60-Citation65 In contrast to p38, which is dispensable for epithelial differentiation,Citation66 the ERKs are crucial for epithelial differentiation, in addition to neuronal differentiation.Citation67,Citation68
Relatively little is known about the roles MKK4 and MKK7 play in ESC differentiation. Available in vitro data suggest that neither MKK4 nor MKK7 is essential for differentiation of ESC to mast cellsCitation69 and that MKK4 is not required for hepatic maturation at the late stage of liver development.Citation70 We have recently investigated the signaling and functional properties of MKK4 and MKK7 in differentiation using single or combined gene knockout ESCs ().Citation71 Our results show that although MKK4 and MKK7 are dispensable for embryonic stem cell self-renewal and maintenance of pluripotency; they have complementary roles in supporting the survival of differentiated cells, likely through synergistic activation of the JNK-c-Jun cascades. We also show that MKK4, but not MKK7, is essential for activation of p38, leading to ATF2 activation and cardiomyocyte differentiation. This set of in vitro data has illustrated a MKK4-p38 cascade in cardiogenesis and a MKK4/MKK7-JNK cascade in cell survival during differentiation. Hence, MKK4 and MKK7 can differentially regulate the downstream MAPKs and make distinct contributions to the differentiation programs.
Figure 4. The roles of MKK4 and MKK7 in differentiation. A Schematic diagram summarizes the roles of MKK4 and MKK7 in vitro ESC differentiation. The MKK4 and MKK7 have complementary roles in activation of the JNK-c-Jun cascades that may be required for differentiated cell survival. Hence, loss of both MKK4 and MKK7 leads to senescence of the differentiated cells. On the other hand, MKK4 is essential for activation of the p38-ATF2/MEF2C cascades, while MKK7 may attenuate p38 activation. Consequently, the Mkk4(-/-) ESCs are defective in myosin heavy chain (MHC) induction and cardiomyocyte differentiation, but Mkk7(-/-) ESCs have enhanced MHC expression and cardiogenesis.
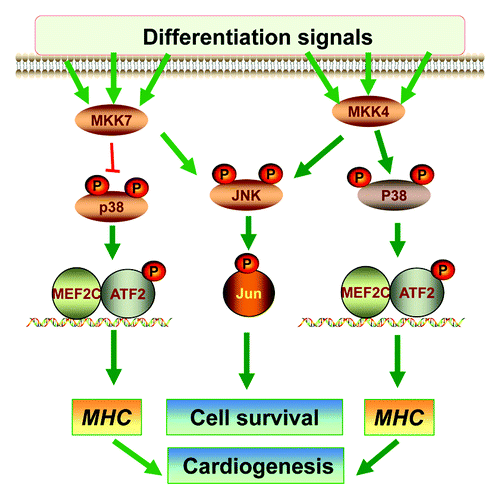
Conclusion and Perspectives
The in vitro ESC culture system, originally developed as a potential technique for cell replacement therapy, has become a powerful tool to understand the basic principles of development. This system offers a relatively inexpensive and easily manipulatable platform to identify extrinsic signals and intracellular factors that control the differentiation programs. Over the past few years, in vitro studies have considerably advanced our understanding of the MAPK signaling mechanisms in lineage specification; however, different experimental settings sometimes lead to opposite conclusions, partly due to variable effects of the MAPK inhibitors used in each given system.Citation72 One approach to overcome this problem is to take advantage of gene knockout mouse ESCs. Using this approach, we are able to obtain a clearer view of the signaling mechanisms and functions of MKK4 and MKK7 in lineage commitment. A major challenge of the in vitro system is the heterogeneity of the differentiated cell populations that not only complicate directed differentiation and propagation, but also make it difficult to assess the differentiation outcomes. Genetically manipulate ESCs have recently been developed to express green fluorescence protein (GFP) and/or selection markers under the control of a lineage-specific gene promoter. This will allow the specific cell types to be traced and purified during differentiation.Citation73 Incorporation of emerging new technologies holds great promises to extend the application of the in vitro system. For example, a recent study shows that chromatin immunoprecipitation and sequencing (ChiP-seq) technique has been applied to the in vitro ESC system, leading to discovery of a novel chromatin-modifying function of JNK in histone H3 Ser10 (H3S10) phosphorylation during neuronal differentiation.Citation74 While the in vitro findings still need to be validated in vivo, the rapid evolution of the in vitro system will undoubtedly make it extremely useful to illuminate the complex gene-gene and gene-environmental interaction mechanisms underlying development and diseases.
Disclosure of Potential Conflicts of Interest
No potential conflicts of interest were disclosed.
Acknowledgments
We thank Dr. Alvaro Puga for proof-reading the manuscript. Work described in the paper is supported in part by grants from National Institute of Health, NEI EY15227, and NIEHS P30 ES00609.
References
- Davis RJ. The mitogen-activated protein kinase signal transduction pathway. J Biol Chem 1993; 268:14553 - 6; PMID: 8325833
- Dérijard B, Raingeaud J, Barrett T, Wu IH, Han J, Ulevitch RJ, et al. Independent human MAP-kinase signal transduction pathways defined by MEK and MKK isoforms. Science 1995; 267:682 - 5; http://dx.doi.org/10.1126/science.7839144; PMID: 7839144
- Kyriakis JM, Avruch J. Mammalian mitogen-activated protein kinase signal transduction pathways activated by stress and inflammation. Physiol Rev 2001; 81:807 - 69; PMID: 11274345
- Schaeffer HJ, Weber MJ. Mitogen-activated protein kinases: specific messages from ubiquitous messengers. Mol Cell Biol 1999; 19:2435 - 44; PMID: 10082509
- Davis RJ. Signal transduction by the JNK group of MAP kinases. Cell 2000; 103:239 - 52; http://dx.doi.org/10.1016/S0092-8674(00)00116-1; PMID: 11057897
- Ganiatsas S, Kwee L, Fujiwara Y, Perkins A, Ikeda T, Labow MA, et al. SEK1 deficiency reveals mitogen-activated protein kinase cascade crossregulation and leads to abnormal hepatogenesis. Proc Natl Acad Sci U S A 1998; 95:6881 - 6; http://dx.doi.org/10.1073/pnas.95.12.6881; PMID: 9618507
- Nishina H, Vaz C, Billia P, Nghiem M, Sasaki T, De la Pompa JL, et al. Defective liver formation and liver cell apoptosis in mice lacking the stress signaling kinase SEK1/MKK4. Development 1999; 126:505 - 16; PMID: 9876179
- Yang D, Tournier C, Wysk M, Lu HT, Xu J, Davis RJ, et al. Targeted disruption of the MKK4 gene causes embryonic death, inhibition of c-Jun NH2-terminal kinase activation, and defects in AP-1 transcriptional activity. Proc Natl Acad Sci U S A 1997; 94:3004 - 9; http://dx.doi.org/10.1073/pnas.94.7.3004; PMID: 9096336
- Wada T, Joza N, Cheng HY, Sasaki T, Kozieradzki I, Bachmaier K, et al. MKK7 couples stress signalling to G2/M cell-cycle progression and cellular senescence. Nat Cell Biol 2004; 6:215 - 26; http://dx.doi.org/10.1038/ncb1098; PMID: 15039780
- Asaoka Y, Nishina H. Diverse physiological functions of MKK4 and MKK7 during early embryogenesis. J Biochem 2010; 148:393 - 401; PMID: 20801953
- Wang X, Destrument A, Tournier C. Physiological roles of MKK4 and MKK7: insights from animal models. Biochim Biophys Acta 2007; 1773:1349 - 57; http://dx.doi.org/10.1016/j.bbamcr.2006.10.016; PMID: 17157936
- Yao Z, Diener K, Wang XS, Zukowski M, Matsumoto G, Zhou G, et al. Activation of stress-activated protein kinases/c-Jun N-terminal protein kinases (SAPKs/JNKs) by a novel mitogen-activated protein kinase kinase. J Biol Chem 1997; 272:32378 - 83; http://dx.doi.org/10.1074/jbc.272.51.32378; PMID: 9405446
- Lee JK, Hwang WS, Lee YD, Han PL. Dynamic expression of SEK1 suggests multiple roles of the gene during embryogenesis and in adult brain of mice. Brain Res Mol Brain Res 1999; 66:133 - 40; http://dx.doi.org/10.1016/S0169-328X(99)00035-2; PMID: 10095085
- Wang X, Nadarajah B, Robinson AC, McColl BW, Jin JW, Dajas-Bailador F, et al. Targeted deletion of the mitogen-activated protein kinase kinase 4 gene in the nervous system causes severe brain developmental defects and premature death. Mol Cell Biol 2007; 27:7935 - 46; http://dx.doi.org/10.1128/MCB.00226-07; PMID: 17875933
- Brancho D, Tanaka N, Jaeschke A, Ventura JJ, Kelkar N, Tanaka Y, et al. Mechanism of p38 MAP kinase activation in vivo. Genes Dev 2003; 17:1969 - 78; http://dx.doi.org/10.1101/gad.1107303; PMID: 12893778
- Doza YN, Cuenda A, Thomas GM, Cohen P, Nebreda AR. Activation of the MAP kinase homologue RK requires the phosphorylation of Thr-180 and Tyr-182 and both residues are phosphorylated in chemically stressed KB cells. FEBS Lett 1995; 364:223 - 8; http://dx.doi.org/10.1016/0014-5793(95)00346-B; PMID: 7750576
- Lawler S, Fleming Y, Goedert M, Cohen P. Synergistic activation of SAPK1/JNK1 by two MAP kinase kinases in vitro. Curr Biol 1998; 8:1387 - 90; http://dx.doi.org/10.1016/S0960-9822(98)00019-0; PMID: 9889102
- Lin A, Minden A, Martinetto H, Claret FX, Lange-Carter C, Mercurio F, et al. Identification of a dual specificity kinase that activates the Jun kinases and p38-Mpk2. Science 1995; 268:286 - 90; http://dx.doi.org/10.1126/science.7716521; PMID: 7716521
- Tournier C, Dong C, Turner TK, Jones SN, Flavell RA, Davis RJ. MKK7 is an essential component of the JNK signal transduction pathway activated by proinflammatory cytokines. Genes Dev 2001; 15:1419 - 26; http://dx.doi.org/10.1101/gad.888501; PMID: 11390361
- Wada T, Nakagawa K, Watanabe T, Nishitai G, Seo J, Kishimoto H, et al. Impaired synergistic activation of stress-activated protein kinase SAPK/JNK in mouse embryonic stem cells lacking SEK1/MKK4: different contribution of SEK2/MKK7 isoforms to the synergistic activation. J Biol Chem 2001; 276:30892 - 7; http://dx.doi.org/10.1074/jbc.M011780200; PMID: 11418587
- Nishina H, Wada T, Katada T. Physiological roles of SAPK/JNK signaling pathway. J Biochem 2004; 136:123 - 6; http://dx.doi.org/10.1093/jb/mvh117; PMID: 15496581
- Gupta S, Barrett T, Whitmarsh AJ, Cavanagh J, Sluss HK, Dérijard B, et al. Selective interaction of JNK protein kinase isoforms with transcription factors. EMBO J 1996; 15:2760 - 70; PMID: 8654373
- Martin JH, Mohit AA, Miller CA. Developmental expression in the mouse nervous system of the p493F12 SAP kinase. Brain Res Mol Brain Res 1996; 35:47 - 57; http://dx.doi.org/10.1016/0169-328X(95)00181-Q; PMID: 8717339
- Yang D, Tournier C, Wysk M, Lu HT, Xu J, Davis RJ, et al. Targeted disruption of the MKK4 gene causes embryonic death, inhibition of c-Jun NH2-terminal kinase activation, and defects in AP-1 transcriptional activity. Proc Natl Acad Sci U S A 1997; 94:3004 - 9; http://dx.doi.org/10.1073/pnas.94.7.3004; PMID: 9096336
- Kuan CY, Yang DD, Samanta Roy DR, Davis RJ, Rakic P, Flavell RA. The Jnk1 and Jnk2 protein kinases are required for regional specific apoptosis during early brain development. Neuron 1999; 22:667 - 76; http://dx.doi.org/10.1016/S0896-6273(00)80727-8; PMID: 10230788
- Sabapathy K, Jochum W, Hochedlinger K, Chang L, Karin M, Wagner EF. Defective neural tube morphogenesis and altered apoptosis in the absence of both JNK1 and JNK2. Mech Dev 1999; 89:115 - 24; http://dx.doi.org/10.1016/S0925-4773(99)00213-0; PMID: 10559486
- Cuenda A, Rousseau S. p38 MAP-kinases pathway regulation, function and role in human diseases. Biochim Biophys Acta 2007; 1773:1358 - 75; http://dx.doi.org/10.1016/j.bbamcr.2007.03.010; PMID: 17481747
- Hu MC, Wang YP, Mikhail A, Qiu WR, Tan TH. Murine p38-delta mitogen-activated protein kinase, a developmentally regulated protein kinase that is activated by stress and proinflammatory cytokines. J Biol Chem 1999; 274:7095 - 102; http://dx.doi.org/10.1074/jbc.274.11.7095; PMID: 10066767
- Hui L, Bakiri L, Stepniak E, Wagner EF. p38alpha: a suppressor of cell proliferation and tumorigenesis. Cell Cycle 2007; 6:2429 - 33; http://dx.doi.org/10.4161/cc.6.20.4774; PMID: 17957136
- Adams RH, Porras A, Alonso G, Jones M, Vintersten K, Panelli S, et al. Essential role of p38alpha MAP kinase in placental but not embryonic cardiovascular development. Mol Cell 2000; 6:109 - 16; PMID: 10949032
- Allen M, Svensson L, Roach M, Hambor J, McNeish J, Gabel CA. Deficiency of the stress kinase p38alpha results in embryonic lethality: characterization of the kinase dependence of stress responses of enzyme-deficient embryonic stem cells. J Exp Med 2000; 191:859 - 70; http://dx.doi.org/10.1084/jem.191.5.859; PMID: 10704466
- Mudgett JS, Ding J, Guh-Siesel L, Chartrain NA, Yang L, Gopal S, et al. Essential role for p38alpha mitogen-activated protein kinase in placental angiogenesis. Proc Natl Acad Sci U S A 2000; 97:10454 - 9; http://dx.doi.org/10.1073/pnas.180316397; PMID: 10973481
- Tamura K, Sudo T, Senftleben U, Dadak AM, Johnson R, Karin M. Requirement for p38alpha in erythropoietin expression: a role for stress kinases in erythropoiesis. Cell 2000; 102:221 - 31; http://dx.doi.org/10.1016/S0092-8674(00)00027-1; PMID: 10943842
- Downs KM, Davies T. Staging of gastrulating mouse embryos by morphological landmarks in the dissecting microscope. Development 1993; 118:1255 - 66; PMID: 8269852
- Thomson JA, Itskovitz-Eldor J, Shapiro SS, Waknitz MA, Swiergiel JJ, Marshall VS, et al. Embryonic stem cell lines derived from human blastocysts. Science 1998; 282:1145 - 7; http://dx.doi.org/10.1126/science.282.5391.1145; PMID: 9804556
- Smith AG. Embryo-derived stem cells: of mice and men. Annu Rev Cell Dev Biol 2001; 17:435 - 62; http://dx.doi.org/10.1146/annurev.cellbio.17.1.435; PMID: 11687496
- Keller G. Embryonic stem cell differentiation: emergence of a new era in biology and medicine. Genes Dev 2005; 19:1129 - 55; http://dx.doi.org/10.1101/gad.1303605; PMID: 15905405
- Desbaillets I, Ziegler U, Groscurth P, Gassmann M. Embryoid bodies: an in vitro model of mouse embryogenesis. Exp Physiol 2000; 85:645 - 51; http://dx.doi.org/10.1017/S0958067000021047; PMID: 11187960
- Höpfl G, Gassmann M, Desbaillets I. Differentiating embryonic stem cells into embryoid bodies. Methods Mol Biol 2004; 254:79 - 98; PMID: 15041757
- Keller GM. In vitro differentiation of embryonic stem cells. Curr Opin Cell Biol 1995; 7:862 - 9; http://dx.doi.org/10.1016/0955-0674(95)80071-9; PMID: 8608017
- Aberdam E, Barak E, Rouleau M, de LaForest S, Berrih-Aknin S, Suter DM, et al. A pure population of ectodermal cells derived from human embryonic stem cells. Stem Cells 2008; 26:440 - 4; http://dx.doi.org/10.1634/stemcells.2007-0588; PMID: 18032703
- Bibel M, Richter J, Schrenk K, Tucker KL, Staiger V, Korte M, et al. Differentiation of mouse embryonic stem cells into a defined neuronal lineage. Nat Neurosci 2004; 7:1003 - 9; http://dx.doi.org/10.1038/nn1301; PMID: 15332090
- Strübing C, Ahnert-Hilger G, Shan J, Wiedenmann B, Hescheler J, Wobus AM. Differentiation of pluripotent embryonic stem cells into the neuronal lineage in vitro gives rise to mature inhibitory and excitatory neurons. Mech Dev 1995; 53:275 - 87; http://dx.doi.org/10.1016/0925-4773(95)00446-8; PMID: 8562428
- Rathjen J, Haines BP, Hudson KM, Nesci A, Dunn S, Rathjen PD. Directed differentiation of pluripotent cells to neural lineages: homogeneous formation and differentiation of a neurectoderm population. Development 2002; 129:2649 - 61; PMID: 12015293
- Lee SH, Lumelsky N, Studer L, Auerbach JM, McKay RD. Efficient generation of midbrain and hindbrain neurons from mouse embryonic stem cells. Nat Biotechnol 2000; 18:675 - 9; http://dx.doi.org/10.1038/76536; PMID: 10835609
- O’Shea KS. Neural differentiation of embryonic stem cells. Methods Mol Biol 2002; 198:3 - 14; PMID: 11951633
- Metallo CM, Ji L, de Pablo JJ, Palecek SP. Retinoic acid and bone morphogenetic protein signaling synergize to efficiently direct epithelial differentiation of human embryonic stem cells. Stem Cells 2008; 26:372 - 80; http://dx.doi.org/10.1634/stemcells.2007-0501; PMID: 17962700
- Nguyen Ngoc TD, Son YO, Lim SS, Shi X, Kim JG, Heo JS, et al. Sodium fluoride induces apoptosis in mouse embryonic stem cells through ROS-dependent and caspase- and JNK-mediated pathways. Toxicol Appl Pharmacol 2012; 259:329 - 37; http://dx.doi.org/10.1016/j.taap.2012.01.010; PMID: 22285274
- Chae HD, Broxmeyer HE. SIRT1 deficiency downregulates PTEN/JNK/FOXO1 pathway to block reactive oxygen species-induced apoptosis in mouse embryonic stem cells. Stem Cells Dev 2011; 20:1277 - 85; http://dx.doi.org/10.1089/scd.2010.0465; PMID: 21083429
- Kim MH, Kim MO, Heo JS, Kim JS, Han HJ. Acetylcholine inhibits long-term hypoxia-induced apoptosis by suppressing the oxidative stress-mediated MAPKs activation as well as regulation of Bcl-2, c-IAPs, and caspase-3 in mouse embryonic stem cells. Apoptosis 2008; 13:295 - 304; http://dx.doi.org/10.1007/s10495-007-0160-y; PMID: 18049903
- Huang FJ, Lan KC, Kang HY, Lin PY, Chan WH, Hsu YC, et al. Retinoic acid influences the embryoid body formation in mouse embryonic stem cells by induction of caspase and p38 MAPK/JNK-mediated apoptosis. Environ Toxicol 2011; Epub ahead of print http://dx.doi.org/10.1002/tox.20709; PMID: 21626648
- Lee SH, Heo JS, Lee MY, Han HJ. Effect of dihydrotestosterone on hydrogen peroxide-induced apoptosis of mouse embryonic stem cells. J Cell Physiol 2008; 216:269 - 75; http://dx.doi.org/10.1002/jcp.21402; PMID: 18330893
- Krishnamoorthy M, Heimburg-Molinaro J, Bargo AM, Nash RJ, Nash RJ. Heparin binding epidermal growth factor-like growth factor and PD169316 prevent apoptosis in mouse embryonic stem cells. J Biochem 2009; 145:177 - 84; http://dx.doi.org/10.1093/jb/mvn153; PMID: 19010935
- Hsuuw YD, Kuo TF, Lee KH, Liu YC, Huang YT, Lai CY, et al. Ginkgolide B induces apoptosis via activation of JNK and p21-activated protein kinase 2 in mouse embryonic stem cells. Ann N Y Acad Sci 2009; 1171:501 - 8; http://dx.doi.org/10.1111/j.1749-6632.2009.04691.x; PMID: 19723096
- Xu P, Davis RJ. c-Jun NH2-terminal kinase is required for lineage-specific differentiation but not stem cell self-renewal. Mol Cell Biol 2010; 30:1329 - 40; http://dx.doi.org/10.1128/MCB.00795-09; PMID: 20065035
- Aubert J, Dunstan H, Chambers I, Smith A. Functional gene screening in embryonic stem cells implicates Wnt antagonism in neural differentiation. Nat Biotechnol 2002; 20:1240 - 5; http://dx.doi.org/10.1038/nbt763; PMID: 12447396
- Haegele L, Ingold B, Naumann H, Tabatabai G, Ledermann B, Brandner S. Wnt signalling inhibits neural differentiation of embryonic stem cells by controlling bone morphogenetic protein expression. Mol Cell Neurosci 2003; 24:696 - 708; http://dx.doi.org/10.1016/S1044-7431(03)00232-X; PMID: 14664819
- Amura CR, Marek L, Winn RA, Heasley LE. Inhibited neurogenesis in JNK1-deficient embryonic stem cells. Mol Cell Biol 2005; 25:10791 - 802; http://dx.doi.org/10.1128/MCB.25.24.10791-10802.2005; PMID: 16314504
- Onizuka T, Yuasa S, Kusumoto D, Shimoji K, Egashira T, Ohno Y, et al. Wnt2 accelerates cardiac myocyte differentiation from ES-cell derived mesodermal cells via non-canonical pathway. J Mol Cell Cardiol 2012; 52:650 - 9; http://dx.doi.org/10.1016/j.yjmcc.2011.11.010; PMID: 22146296
- Aouadi M, Bost F, Caron L, Laurent K, Le Marchand Brustel Y, Binétruy B. p38 mitogen-activated protein kinase activity commits embryonic stem cells to either neurogenesis or cardiomyogenesis. Stem Cells 2006; 24:1399 - 406; http://dx.doi.org/10.1634/stemcells.2005-0398; PMID: 16424397
- Aouadi M, Laurent K, Prot M, Le Marchand-Brustel Y, Binétruy B, Bost F. Inhibition of p38MAPK increases adipogenesis from embryonic to adult stages. Diabetes 2006; 55:281 - 9; http://dx.doi.org/10.2337/diabetes.55.02.06.db05-0963; PMID: 16443758
- Graichen R, Xu X, Braam SR, Balakrishnan T, Norfiza S, Sieh S, et al. Enhanced cardiomyogenesis of human embryonic stem cells by a small molecular inhibitor of p38 MAPK. Differentiation 2008; 76:357 - 70; http://dx.doi.org/10.1111/j.1432-0436.2007.00236.x; PMID: 18021257
- Barruet E, Hadadeh O, Peiretti F, Renault VM, Hadjal Y, Bernot D, et al. p38 mitogen activated protein kinase controls two successive-steps during the early mesodermal commitment of embryonic stem cells. Stem Cells Dev 2011; 20:1233 - 46; http://dx.doi.org/10.1089/scd.2010.0213; PMID: 20954847
- Wu J, Kubota J, Hirayama J, Nagai Y, Nishina S, Yokoi T, et al. p38 Mitogen-activated protein kinase controls a switch between cardiomyocyte and neuronal commitment of murine embryonic stem cells by activating myocyte enhancer factor 2C-dependent bone morphogenetic protein 2 transcription. Stem Cells Dev 2010; 19:1723 - 34; http://dx.doi.org/10.1089/scd.2010.0066; PMID: 20412016
- Ding L, Liang XG, Hu Y, Zhu DY, Lou YJ. Involvement of p38MAPK and reactive oxygen species in icariin-induced cardiomyocyte differentiation of murine embryonic stem cells in vitro. Stem Cells Dev 2008; 17:751 - 60; http://dx.doi.org/10.1089/scd.2007.0206; PMID: 18484897
- Chakraborty S, Kang B, Huang F, Guo YL. Mouse embryonic stem cells lacking p38alpha and p38delta can differentiate to endothelial cells, smooth muscle cells, and epithelial cells. Differentiation 2009; 78:143 - 50; http://dx.doi.org/10.1016/j.diff.2009.05.006; PMID: 19539422
- Huang Z, Yu J, Toselli P, Bhawan J, Sudireddy V, Taylor L, et al. Angiotensin II type 1 and bradykinin B2 receptors expressed in early stage epithelial cells derived from human embryonic stem cells. J Cell Physiol 2007; 211:816 - 25; http://dx.doi.org/10.1002/jcp.20985; PMID: 17299793
- Li Z, Theus MH, Wei L. Role of ERK 1/2 signaling in neuronal differentiation of cultured embryonic stem cells. Dev Growth Differ 2006; 48:513 - 23; http://dx.doi.org/10.1111/j.1440-169X.2006.00889.x; PMID: 17026715
- Chayama K, Papst PJ, Garrington TP, Pratt JC, Ishizuka T, Webb S, et al. Role of MEKK2-MEK5 in the regulation of TNF-alpha gene expression and MEKK2-MKK7 in the activation of c-Jun N-terminal kinase in mast cells. Proc Natl Acad Sci U S A 2001; 98:4599 - 604; http://dx.doi.org/10.1073/pnas.081021898; PMID: 11274363
- Hamazaki T, Iiboshi Y, Oka M, Papst PJ, Meacham AM, Zon LI, et al. Hepatic maturation in differentiating embryonic stem cells in vitro. FEBS Lett 2001; 497:15 - 9; http://dx.doi.org/10.1016/S0014-5793(01)02423-1; PMID: 11376655
- Wang J, Chen L, Ko CI, Zhang L, Puga A, Xia Y. Distinct signaling properties of mitogen-activated protein kinase kinases 4 (MKK4) and 7 (MKK7) in embryonic stem cell (ESC) differentiation. J Biol Chem 2012; 287:2787 - 97; http://dx.doi.org/10.1074/jbc.M111.281915; PMID: 22130668
- Kempf H, Lecina M, Ting S, Zweigerdt R, Oh S. Distinct regulation of mitogen-activated protein kinase activities is coupled with enhanced cardiac differentiation of human embryonic stem cells. Stem Cell Res 2011; 7:198 - 209; http://dx.doi.org/10.1016/j.scr.2011.06.001; PMID: 21907163
- Wan CR, Chung S, Kamm RD. Differentiation of embryonic stem cells into cardiomyocytes in a compliant microfluidic system. Ann Biomed Eng 2011; 39:1840 - 7; http://dx.doi.org/10.1007/s10439-011-0275-8; PMID: 21336802
- Tiwari VK, Stadler MB, Wirbelauer C, Paro R, Schübeler D, Beisel C. A chromatin-modifying function of JNK during stem cell differentiation. Nat Genet 2012; 44:94 - 100; http://dx.doi.org/10.1038/ng.1036; PMID: 22179133