Abstract
As reading fiction can challenge us to better understand fact, using fake animals can sometimes serve as our best solution to understanding the behavior of real animals. The use of dummies, doppelgangers, fakes, and physical models have served to elicit behaviors in animal experiments since the early history of behavior studies, and, more recently, robotic animals have been employed by researchers to further coax behaviors from their study subjects. Here, we review the use of robots in the service of animal behavior, and describe in detail the production and use of one type of robot – “faux” frogs – to test female responses to multisensory courtship signals. The túngara frog (Physalaemus pustulosus) has been a study subject for investigating multimodal signaling, and we discuss the benefits and drawbacks of using the faux frogs we have designed, with the larger aim of inspiring other scientists to consider the appropriate application of physical models and robots in their research.
To better understand an animal’s behavior, biologists can experimentally manipulate the animal or its environment. One time-honored manipulation is to present live animals with artificial renderings (physical models) to examine behaviors in the real animals, either in the field or in the laboratory. The history of introducing physical models into animal behavior studies has progressed into a recent surge of animatronics, or the use of kinetic models. We produced robotic “faux” frogs to better understand the signaling and mate selection behavior of a tropical frog in a multimodal (acoustic + visual signals) context. Here, we present our experiences using faux frogs in the broader context of robots as experimental tools to tackle challenges such as those posed by studying multimodality. By sharing our approach’s effectiveness and limitations, we wish to trigger greater production of and discussion about the use of physical models in studies of animal behavior.
The Challenges of Multimodality
Considering how difficult it can be to experimentally test hypotheses that result in definitive answers about an animal’s behavior based on its vision, hearing, touch, taste, or smell, it becomes far more difficult to design experiments that give clear answers involving a combination of these perceptual modalities. In humans, for example, the acoustic perception of speech phenomes changes when the listener is also able to see the movement of the speaker’s lips – the well-known McGurk effect.Citation1 Receiver responses can be modulated by interactions among signal components in nonhuman animals as well, sometimes in unexpected ways.Citation2 Adding to the confusion is that traits conspicuous to humans are sometimes surprisingly unimportant as signal components in nonhuman animals.Citation3,Citation4 Controlling aspects of one perceptual modality and not another would be useful when investigating animal behavior, but is often impossible when working exclusively with living organisms. This challenge has led researchers to seek means other than relying exclusively on living animals to answer biological questions.
Use of Physical Models in the Study of Animal Behavior
Why would a biologist choose to conduct research with physical models rather than with living animals? Artifice, when introduced wisely, can broaden the scientist’s toolbox and hypothesis-testing potential. Physical models can be easier to handle than real animals, and can allow for a precise manipulation of traits. Physical models can imitate complicated phenomena,Citation5 or be simpleCitation6,Citation7 and inexpensive.Citation8 Researchers are not only able to control the way physical models look, but can control where they are placed in the environment, contributing to a more standardized, repeatable experimental design and for causal analysis of behavior. Physical models, especially robots, make it feasible to test the influence of individual signal components on receivers, as well as examine interactions among signal components. Robots can also be effective in determining the salience of putative signal components. A researcher using fake animals can fabricate and even exaggerate these components to render a super stimulus. One can also distort a signal in unnatural but useful ways, as with testing the consequences of asynchrony between acoustic and visual courtship components when a living animal is incapable of such behavior.Citation2
Use of physical models has successfully contributed to our understanding of a wide range of biological topics, with the study of animal behavior benefitting from physical models since at least the field’s earliest publications.Citation9 Bizarre, impossible taxidermy of predatory birds,Citation10 fake chameleon heads on sticks,Citation11 horse mannequins,Citation12 zebra costumes,Citation13 glass beads as termite eggs,Citation14 two-dimensional gull puppets,Citation6 a cotton-filled fabric Indian cobra,Citation15 and ewes of fake fur over wire and woodCitation16 are among the hordes of physical, non-robotic models that have been effectively placed among live animals in the service of animal behavior.
Rise of the Robots
Advances in technology, and accessibility and affordability of equipment have led to a new era of physical models used in biology, with the number of robots on the rise. Humans frequently exploit nature to design better robots,Citation17 and we occasionally design robots to increase our understanding of nature.Citation18 By simulating biological entities, robots can shed light on natural selection,Citation19,Citation20 animal physiology,Citation21,Citation22 biomechanics,Citation23-Citation26 and behaviorCitation18,Citation27-Citation30 without ever having to interact with living organisms.
Introducing robots to living organisms offers the biologist new opportunities to answer otherwise strategically problematic questions about natural social interactions. Robotic animals have been introduced to living mammals,Citation31,Citation32 birds,Citation33-Citation36 reptiles,Citation37,Citation38 fishCitation39-Citation41 and invertebrates.Citation42-Citation46 For a cursory review of robots used in animal behavior studies see refs. Citation47–Citation48.
Robotic amphibians have also graced the ethological stage, with the introduction of electromechanical dart-poison frog models by Narins et al. to study aggressionCitation49 and cross-modal integration.Citation50 Roland Rupp created these dart-poison frogs using silicone rubber with pneumatically-inflated vocal sacs, which when painted and presented on a turntable in a naturalistic setting, effectively elicited behaviors in naturally-occurring frogs. Likewise, Bryant Buchanan painted plaster models of squirrel treefrogs (Hyla squirella), which were employed by RCT. These simple treefrog robots had manually inflatable vocal sacs made of condoms, and were used to test the role of visual vs. acoustic signals in mate choice.Citation51 Later, Taylor et al. introduced automated robotic túngara frogs (P. pustulosus) to continue testing aspects of multimodal signaling,Citation52 the subject of this article. A comparative study of signaling by squirrel treefrogs and túngara frogs again relied on responses of real frogs to robots.Citation4 Finally, Caldwell et al.Citation53 staged contests between real red-eyed treefrogs (Agalychnis callidryas), then tested whether a male’s tremulations triggered contests by using models for visual and vibrational playback. Loosely connected limbs allowed the frog model (fabricated by David McCornack) to tremulate when coupled with an electronic shaker. In each of these studies: dart-poison frogs, squirrel treefrogs, túngara frogs, and red-eyed treefrogs, behaviors of real frogs were elicited in a repeatable, standardized fashion by robots. Another shared aspect of these faux frog studies was the aim to understand the relative importance of signals catering to different perceptual modalities.
Robots and Realism
How realistic does a robot need to be? When producing models, erring on the side of realism has its advantages as well as its disadvantages. The time, energy, and expense invested producing realistic models may be wasted if the information it provides is beyond the perceptual capacity of the animal recipient. A realistic model can add unwanted or excessive information, obfuscating the actual components responsible for eliciting responses in real animals. For instance, a realistic model made of materials that produce odors undetected by the human researcher could conceivably confound results when exposed to a test subject. Parsimony is often the goal for a researcher because with a simple design one can strive to reach a simple answer. What, then, can be gained by having a realistic model over a simplified model? Realistic models offer the opportunity to match natural inputs as completely as possible so that something meaningful can be said of naturally occurring phenomena. An overly simple model, while it could help us to focus on the salient feature or features of potential interest, can also miss out on revealing behaviors that are more biologically relevant. If the sight of a moving sphere is attractive to a female, it says little about how alluring that moving sphere is in the context of acoustic, tactile, and additional visual elements accompanying the moving sphere in a natural setting. An important first step is to observe how animals behave under natural conditions in order to recognize natural reactions by animals when introduced to robots. One could experimentally test for the minimum threshold of desired realism that is practical, but such testing can be time-consuming, and may never satisfy our desire to know what is actually happening in nature.
Faux Túngara Frogs
Male túngara frogs (P. pustulosus) produce courtship vocalizations, and these vocalizations are accompanied by a conspicuous inflation of a large vocal sac in the male’s throat. We created replicas of male túngara frogs to decipher which signaling components, or combination of signaling components (acoustic + visual), are important for a female when selecting a mate. BAK fabricated mixed-media models with expandable latex vocal sacs (), and JS designed and built a pneumatic system to remotely control the timing of the faux frog’s vocal sac inflations and the timing of digitized calls (). These calls were broadcast by speakers in an arena constructed by RCT in which real female frogs were presented with faux male frogs or only a speaker playing synthesized male courtship calls (). In this system, a servo motor drives a pump into a standard 20 mL syringe with Luer-Lok air fitting (Becton, Dickinson and Co.) which pushes air to expand the vocal sac. This system controls volume of air and speed of inflation/deflation. The audio input circuit board allows the researcher to manually adjust the synchrony of the vocal sac inflation as it relates to the audio output of the experiment. In this paper, we present a more visually explicit, step-wise description of the production of our models than in previous publications, but for further details about methods and materials, see ref. Citation52 and www.pupating.org.
Figure 1. Production of faux frog models. To create a proportionally and structurally accurate male túngara frog, molds were made of a preserved frog specimen. (A) The specimen was partially embedded in a non-sulfur based clay (so as not to react with silicone) and surrounded by a cardboard dam. Depressions were made in the clay to serve as “keys” to lock the resulting two-part mold. Feet were severed and adhered to the bottom of a cardboard box using cyanoacrylate glue, and a one-part mold was made. Once the silicone molds cured and the frog specimens were removed, (B) urethane castes were made of the emaciated, preserved frog body, and a body was sculpted to appear inflated using sculpting epoxy over the urethane cast and over wire armature legs. Details, including body texture and eyes, were added using Elmer’s glue. The completed prototype was molded by (C) embedding it in clay, (D) pouring the first part with silicone, and, once cured, (E) peeling the clay off, spraying the silicone with a mold release (or brushing with Vaseline), then pouring the second part. The keys to lock this two-part mold took the form of a square ridge (E) and canal. Urethane casts were poured, and touched up by removing flashing and sanding seams. The feet were produced by (F) injecting hotmelt glue into the silicone mold, (G) cutting out the feet with surgical or sewing scissors, then (H) attaching to the body by heating each foot base with a soldering gun and pressing to the distal end of each urethane leg. Drilling holes in leg and foot, and inserting wire in both ends prior to heating the foot base strengthened the connection. A drill (Dremel Inc., New York) was used to hollow out the body from mouth to anal region, and tiny screws were glued into drilled holes in venter using two-ton epoxy. (I) The frogs were painted by mixing acrylics to match colors found on live frogs, (J) spraying with an airbrush as basecoats, then (K) applying acrylic paints with a fine brush. To match the glistening surface of a live male, the models were sprayed and sealed, then left to dry. Several inexpensive materials can function as vocal sac surrogates, including latex balloons or condoms (L), but urinary catheters appeared to last longest. (M) The catheter balloons were partially inflated, then painted by spraying with flexible automotive paint and brushing white stripes with a fabric paint. (N) Finally, the catheter was threaded through the model body and connected to the controller unit. Image N is a composite of two photographs. Scale bars (B, H, N) = 1 cm. Product details can be found in ref. Citation52.
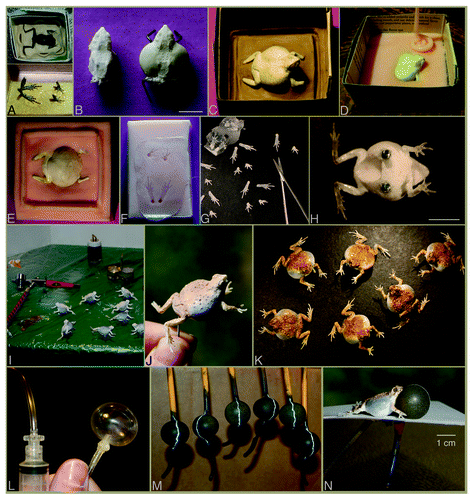
Figure 2. Robotic frog controller, shown as (A) an exploded view of digitally-rendered components and (B) in box with opened lid. Components include (1) Servo Motor with built-in programmable controller, (2) 20 ml syringe embedded in a block of milled Delrin, and (3) audio input and controller circuit. (C) Arena (1.8 x 1.8 min) within which female was released from under a centrally-placed funnel to select one of two potential call sources (black speakers) with or without a robotic male. Product details, including additional controller components, can be found in ref. Citation52.
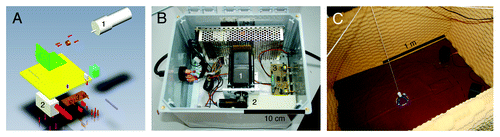
How realistic does a male túngara frog robot need to be? Females apparently respond to the inflating artificial vocal sac as the call is being played no differently than they do when the model of the frog’s body accompanies these stimuli.Citation52 Is the inflating artificial vocal sac sufficiently alluring and did we needlessly surpass this threshold of visual stimulation when creating realistic models to accompany the vocal sacs ()? When túngara male frogs court, the vocalization is the dominant signal component, but females respond significantly more when the call is accompanied by an inflating artificial vocal sac,Citation52,Citation54 and when both the call and the vocal sac inflation are in synchrony.Citation2 Frogs are very responsive to motion and nocturnally active frogs may process relatively low-resolution images,Citation55,Citation56 so the moving latex vocal sac may be sufficient for the female under certain testing conditions. If greater realism stimulates more natural reactions in the female, other testing conditions may benefit from having the model of the male frog’s body along with the inflating artificial vocal sac and call. The value of such realism when light levels vary, or additional perceptual modalities (touch, smell, and taste) are investigated in terms of how they affect a female’s decision-making is a subject for future studies.
Challenges and Areas For Improvement
Using robotic models comes with assumptions. Although our frog models appear realistic, they are imperfect, and we assume the deviations from nature are unimportant (the behavior suggests this is true). Replicating nature has its challenges, and the most influential challenge we encountered when creating faux túngara frogs relates to their small size (ca. 1.5 g). As a result, our controller and mechanical parts were externalized. The pneumatic system allowed us to deliver air to a tiny location, controlling speed and volume of vocal sac inflations. Settling on standard pneumatic components allowed us to focus on vocal sac (balloon) strength, durability, and realism. After testing an array of balloons and condoms, we chose medical urinary catheters for their strength and regularity in terms of their duration of operation. While the system has survived years of successful operation, several aspects of our system could be improved.
1. The pneumatic system with servo creates considerable mechanical noise when operating. In the field, this has resulted in the unit being placed in a fabricated sound-isolation box to minimize noise.
2. The artificial vocal sac itself produces mechanical noise when it is inflated and deflated. Depending on the perceptual capacities of living subjects exposed to the calling robot and the questions posed by researchers, the experimental design may require controlling for this noise.
3. The pump mechanism uses a standard 20 mL syringe, which has a limited duration of only 1–2 mo, after which this part malfunctions or fails entirely. While this is not an expensive component, it requires time to replace because much of the controller must be taken apart to access it.
4. Each catheter inflates approximately 250 times (30/min for 8–9 min) before failing. The catheters are both expensive as disposable units and labor-intensive in terms of their preparation.
5. The combination of pneumatic parts and catheters was originally intended to be a flexible system that could be adapted to other frog experiments with vocal sacs of different sizes. In fact, the system has run into physical limits due to the limited expansion capacity of the catheters in combination with the loss of transmitted volume due to air compression. The longer the tube leading to the catheter balloon means less air reaching the balloon itself, and the slower the inflation and deflation rate of the balloon. These physical limitations demand careful calibration that does not presently allow for great flexibility in terms of replacing with different catheter models.
6. The frog body was sculpted to appear inflated so that a female would perceive either an inflated vocal sac or an inflated body when approaching a model. A more accurate model would alternate inflations of these two body regions.
Alternative Robots and Alternatives To Robots
Although expense of technical components is generally decreasing, designing and fabricating systems from scratch can be prohibitively expensive for many researchers. Alternatives to our design are many, and less expensive approaches include creating frogs with vocal sacs made of different materials (), or vocal sacs that inflate by hand.Citation51 The controller unit can also change significantly, and we are presently in the process of testing a controller unit that is powered by compressed air rather than by a motor. A mechanical alternative to our robotic frog’s pneumatics would be to use gel-filled balloons which, when squeezed, produce the desired vocal sac volume and shape, while withstanding repeated use and rubbing against the vocal sac opening in the model ().
Figure 3. Premise for possible alternative designs of model frog with extendable vocal sac made of gel-filled balloon.
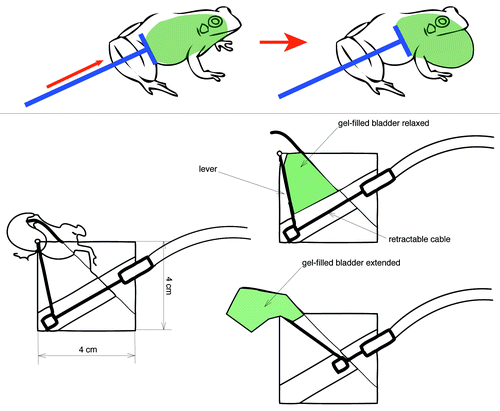
Research questions should be the motivating force behind designing alternative methods. A túngara frog researcher, for example, may wish to investigate the importance of a frog’s body cavity inflations during courting, or of a male frog’s response to external inputs, such as the physical collision by a female frog, which elicits a change in the male’s call composition.Citation57 For questions relating to body cavity inflations, a robot with alternating vocal sac and body inflations would be desirable. For questions relating to a male frog’s response to collisions with females, a “smart” frog model that changes its call composition in response to female proximity or female contact would be the design objective. An alternative to the smart, automatically responsive robot could be a remotely-controlled robot, as used by Patricelli et al.Citation35
Alternatives to using robots exist, of course, and the choice of using still images, mirrors, static models, video or audio playbacks, or manipulated playbacks should be made with careful consideration of questions posed, with availability of technical expertise and financial resources also taken into consideration. Modifying living animals can be effective, as with painting wings of moths in studies of mimicry,Citation58 or implanting interfaces to remotely control flight in cyborg beetles.Citation59,Citation60 Cybernetics has the potential to revolutionize animal behavior research by offering a novel means of controlling behavior in a standardized, repeatable way, although one drawback is that not all of the cyborg’s actions may be predictably standardized or controlled for. Controlling a cyborg’s flight direction, for example, could hypothetically result in an undesirable emission of chemicals by the cyborg, with untold behavioral consequences on sender or receiver. A well-tested, powerful, and flexible alternative to physical model production is the use of computer-animated stimuliCitation61 to stage animal interactions. Like robots, computer animations can provide dynamic visual stimuli, but unlike robots, computer animations offer the benefit of total control over movement properties and capture complex behavioral nuances that may not be feasible or possible with robots. Limitless programming aside, however, computer animations presently suffer from human sensory-biased limitationsCitation61 in the same ways as do videos.Citation52,Citation62 Whether or not technological innovations overcome limitations such as matching visual sensitivity of nonhuman animal subjects, or include 3D virtual realities that respond to an untethered subject’s motions, they will help to define the future of artifice in the study of animal behavior.
Conclusion
The relatively recent panoply of robotic animals in animal behavior studies is an effect of technological progress and is driven by questions that would otherwise demand unreasonable control over or manipulation of a living animal’s behavior. Biologists can address complicated questions related to multimodal signaling by testing real animals’ responses to robots. Robots, when appropriately constructed and used, can open avenues of experimental study, and this paper is, in part, a methodological behind-the-scenes exposé of one type of robot, with a discussion of its assets and drawbacks. More elaborate robots have been used in the service of animal behavior and far more intricate, versatile robots are doubtlessly on the way. Future discussions of the value and applicability of robotics in animal behavior should and will become more frequent as technological progress continues, and hypotheses probe what have been historically untenable realms.
Disclosure of Potential Conflicts of Interest
No potential conflicts of interest were disclosed.
Acknowledgments
We thank Frantisek Baluska for inviting us to submit an addendum article to our previous faux frog work, and one anonymous reviewer for thorough and thoughtful review. Bryant Buchanan provided early insights and constructive comments on multimodal signaling in anurans. This work was supported by the National Science Foundation (IBN 0517328 to MJ Ryan and RC Taylor).
References
- McGurk H, MacDonald J. Hearing lips and seeing voices. Nature 1976; 264:746 - 8; http://dx.doi.org/10.1038/264746a0; PMID: 1012311
- Taylor RC, Klein BA, Stein J, Ryan MJ. Multimodal signal variation in space and time: how important is matching a signal with its signaler?. J Exp Biol 2011; 214:815 - 20; http://dx.doi.org/10.1242/jeb.043638; PMID: 21307068
- de Luna AG, Hödl W, Amézquita A. Colour, size and movement as visual subcomponents in multimodal communication by the frog Allobates femoralis.. Anim Behav 2010; 79:739 - 45; http://dx.doi.org/10.1016/j.anbehav.2009.12.031
- Taylor RC, Klein BA, Stein J, Ryan MJ. Inter-signal interaction and uncertain information in anuran multimodal signals. Curr Zool 2011; 57:153 - 61
- Hassenstein B. Considerations on the use of models in biology. Universitas (Stuttg) 1983; 25:275 - 80
- Tinbergen N, Perdeck AC. On the stimulus situation releasing the begging response in the newly hatched herring gull chick (Larus Argentatus Argentatus Pont.). Behavior 1950; 3:1 - 39; http://dx.doi.org/10.1163/156853951X00197
- Eisner T, Kriston I, Aneshansley DJ. Defensive behavior of a termite (Nasutitermes exitiosus). Behav Ecol Sociobiol 1976; 1:83 - 125; http://dx.doi.org/10.1007/BF00299954
- Yeager J, Wooten C, Summers K. A new technique for the production of large numbers of clay models for field studies of predation. Herpetol Rev 2011; 42:357 - 9
- Peters H. Experimentelle Untersuchungen über die Brutpflege von Haplochromis multicolor, einem maulbrütenden Knochenfisch (Insbesondere zur Analyse eines “angeborenen Schemas”). Z Tierpsychol 1937; 1:201 - 18; http://dx.doi.org/10.1111/j.1439-0310.1937.tb01423.x
- Curio VE. Funktionsweise und Stammesgeschichte des Flugfeinderkennens einiger Darwinfinken (Geospizinae). Z Tierpsychol 1969; 26:394 - 487; http://dx.doi.org/10.1111/j.1439-0310.1969.tb01958.x
- Kästle W. Soziale Verhaltensweisen von Chamäleone aus der pumilus- und bitaeniatus-Gruppe. Z Tierpsychol 1967; 24:313 - 41; http://dx.doi.org/10.1111/j.1439-0310.1967.tb00582.x; PMID: 5627117
- Grzimek B. Begrüßung zweier Pferde. Das Erkennen von Phantomen und Bildern. Z Tierpsychol 1943; 5:465 - 80; http://dx.doi.org/10.1111/j.1439-0310.1943.tb00661.x
- Grzimek B. Attrappenversuche mit Zebras und Löwen in der Serengeti. Z Tierpsychol 1960; 17:351 - 7; http://dx.doi.org/10.1111/j.1439-0310.1960.tb00211.x
- Matsuura K. Termite-egg mimicry by a sclerotium-forming fungus. Proc Biol Sci 2006; 273:1203 - 9; http://dx.doi.org/10.1098/rspb.2005.3434; PMID: 16720392
- Ramakrishnan U, Coss RG, Schank J, Dharawat A, Kim S. Snake species discrimination by wild bonnet macaques (Macaca radiata). Ethology 2005; 111:337 - 56; http://dx.doi.org/10.1111/j.1439-0310.2004.01063.x
- Walser ES, Walters E, Hague P, Williams T. Responses of lambs to model ewes. Behav 1985; 95:110 - 20; http://dx.doi.org/10.1163/156853985X00073
- Pfeifer R, Lungarella M, Iida F. Self-organization, embodiment, and biologically inspired robotics. Science 2007; 318:1088 - 93; http://dx.doi.org/10.1126/science.1145803; PMID: 18006736
- Webb B. Robots in invertebrate neuroscience. Nature 2002; 417:359 - 63; http://dx.doi.org/10.1038/417359a; PMID: 12015617
- Floreano D, Keller L. Evolution of adaptive behaviour in robots by means of Darwinian selection. PLoS Biol 2010; 8:e1000292; http://dx.doi.org/10.1371/journal.pbio.1000292; PMID: 20126252
- Long JH Jr., Koob TJ, Irving K, Combie K, Engel V, Livingston N, et al. Biomimetic evolutionary analysis: testing the adaptive value of vertebrate tail stiffness in autonomous swimming robots. J Exp Biol 2006; 209:4732 - 46; http://dx.doi.org/10.1242/jeb.02559; PMID: 17114406
- Schank JC, May CJ, Tran JT, Joshi SS. A biorobotic investigation of Norway rat pups (Rattus norvegicus) in an arena. Adapt Behav 2004; 12:161 - 73; http://dx.doi.org/10.1177/105971230401200303
- Lepora NF, Fox CW, Evans MH, Diamond ME, Gurney K, Prescott TJ. Optimal decision-making in mammals: insights from a robot study of rodent texture discrimination. J R Soc Interface 2012; 9:1517 - 28; http://dx.doi.org/10.1098/rsif.2011.0750; PMID: 22279155
- Dickinson MH, Götz KG. Unsteady aerodynamic performance of model wings at low Reynolds numbers. J Exp Biol 1993; 174:45 - 64
- Hu DL, Chan B, Bush JWM. The hydrodynamics of water strider locomotion. Nature 2003; 424:663 - 6; http://dx.doi.org/10.1038/nature01793; PMID: 12904790
- Esposito CJ, Tangorra JL, Flammang BE, Lauder GV. A robotic fish caudal fin: effects of stiffness and motor program on locomotor performance. J Exp Biol 2012; 215:56 - 67; http://dx.doi.org/10.1242/jeb.062711; PMID: 22162853
- Leftwich MC, Tytell ED, Cohen AH, Smits AJ. Wake structures behind a swimming robotic lamprey with a passively flexible tail. J Exp Biol 2012; 215:416 - 25; http://dx.doi.org/10.1242/jeb.061440; PMID: 22246250
- Kuwana Y, Nagasawa S, Shimoyama I, Kanzaki R. Synthesis of the pheromone-oriented behaviour of silkworm moths by a mobile robot with moth antennae as pheromone sensors. Biosens Bioelectron 1999; 14:195 - 202; http://dx.doi.org/10.1016/S0956-5663(98)00106-7
- Grasso F, Consi T, Mountain D, Atema J. Biomimetic robot lobster performs chemo-orientation in turbulence using a pair of spatially separated sensors: progress and challenges. Robot Auton Syst 2000; 30:115 - 31; http://dx.doi.org/10.1016/S0921-8890(99)00068-8
- Krieger MJB, Billeter J-B, Keller L. Ant-like task allocation and recruitment in cooperative robots. Nature 2000; 406:992 - 5; http://dx.doi.org/10.1038/35023164; PMID: 10984052
- Floreano D, Mitri S, Magnenat S, Keller L. Evolutionary conditions for the emergence of communication in robots. Curr Biol 2007; 17:514 - 9; http://dx.doi.org/10.1016/j.cub.2007.01.058; PMID: 17320390
- Rundus AS, Owings DH, Joshi SS, Chinn E, Giannini N. Ground squirrels use an infrared signal to deter rattlesnake predation. P Natl Acad Sci USA 2007; 104:14372-14376; DOI: 10.1073_pnas.0702599104.
- Partan SR, Larco CP, Owens MJ. Wild tree squirrels respond with multisensory enhancement to conspecific robot alarm behaviour. Anim Behav 2009; 77:1127 - 35; http://dx.doi.org/10.1016/j.anbehav.2008.12.029
- Patricelli GL, Uy JAC, Walsh G, Borgia G. Male displays adjusted to female’s response. Nature 2002; 415:279 - 80; http://dx.doi.org/10.1038/415279a; PMID: 11796996
- Göth A, Evans CS. Social responses without early experience: Australian brush-turkey chicks use specific visual cues to aggregate with conspecifics. J Exp Biol 2004; 207:2199 - 208; http://dx.doi.org/10.1242/jeb.01008; PMID: 15159424
- Patricelli G, Coleman SW, Borgia G. Male satin bowerbirds (Ptilonorhynchus violaceus) adjust their display intensity in response to female startling: an experiment with robotic females. Anim Behav 2006; 71:49 - 59; http://dx.doi.org/10.1016/j.anbehav.2005.03.029
- Patricelli GL, Krakauer AH. Tactical allocation of effort among multiple signals in sage grouse: an experiment with a robotic female. Behav Ecol 2010; 21:97 - 106; http://dx.doi.org/10.1093/beheco/arp155
- Martins EP, Ord TJ, Davenport SW. Combining motions into complex displays: playbacks with a robotic lizard. Behav Ecol Sociobiol 2005; 58:351 - 60; http://dx.doi.org/10.1007/s00265-005-0954-2
- Ord TJ, Stamps JA. Species identity cues in animal communication. Am Nat 2009; 174:585 - 93; http://dx.doi.org/10.1086/605372; PMID: 19691435
- MacLaren RD, Rowland WJ, Morgan N. Female preferences for sailfin and body size in the sailfin molly, Poecilia latipinna.. Ethology 2004; 110:363 - 79; http://dx.doi.org/10.1111/j.1439-0310.2004.00974.x
- Gumm JM, Gonzalez R, Aspbury AS, Gabor CR. Do I know you? Species recognition operates within and between the sexes in a unisexual-bisexual species complex of mollies. Ethology 2006; 112:448 - 57; http://dx.doi.org/10.1111/j.1439-0310.2005.01175.x
- Faria JJ, Dyer JRG, Clément RO, Couzin ID, Holt N, Ward AJW, et al. A novel method for investigating the collective behaviour of fish: introducing ‘Robofish’. Behav Ecol Sociobiol 2010; 64:1211 - 8; http://dx.doi.org/10.1007/s00265-010-0988-y
- Halloy J, Sempo G, Caprari G, Rivault C, Asadpour M, Tâche F, et al. Social integration of robots into groups of cockroaches to control self-organized choices. Science 2007; 318:1155 - 8; http://dx.doi.org/10.1126/science.1144259; PMID: 18006751
- Ings TC, Chittka L. Speed-accuracy tradeoffs and false alarms in bee responses to cryptic predators. Curr Biol 2008; 18:1520 - 4; http://dx.doi.org/10.1016/j.cub.2008.07.074; PMID: 18771920
- Reaney LT, Sims RA, Sims SWM, Jennions MD, Backwell PRY. Experiments with robots explain synchronized courtship in fiddler crabs. Curr Biol 2008; 18:R62 - 3; http://dx.doi.org/10.1016/j.cub.2007.11.047; PMID: 18211839
- Callander S, Jennions MD, Backwell PRY. Female choice over short and long distances: neighbor effects. Behav Ecol Sociobiol 2011; 65:2071 - 8; http://dx.doi.org/10.1007/s00265-011-1216-0
- Ings TC, Wang M-Y, Chittka L. Colour-independent shape recognition of cryptic predators by bumblebees. Behav Ecol Sociobiol 2012; 66:487 - 96; http://dx.doi.org/10.1007/s00265-011-1295-y
- Knight J. Animal behaviour: when robots go wild. Nature 2005; 434:954 - 5; http://dx.doi.org/10.1038/434954a; PMID: 15846317
- Krause J, Winfield AFT, Deneubourg J-L. Interactive robots in experimental biology. Trends Ecol Evol 2011; 26:369 - 75; http://dx.doi.org/10.1016/j.tree.2011.03.015; PMID: 21496942
- Narins PM, Hödl W, Grabul DS. Bimodal signal requisite for agonistic behavior in a dart-poison frog, Epipedobates femoralis.. Proc Natl Acad Sci U S A 2003; 100:577 - 80; http://dx.doi.org/10.1073/pnas.0237165100; PMID: 12515862
- Narins PM, Grabul DS, Soma KK, Gaucher P, Hödl W. Cross-modal integration in a dart-poison frog. Proc Nat Acad Sci USA 2005; 102:2425-2429; DOI: 10.1073_pnas.0406407102.
- Taylor RC, Buchanan BW, Doherty JL. Sexual selection in the squirrel treefrog Hyla squirella: the role of multimodal cue assessment in female choice. Anim Behav 2007; 74:1753 - 63; http://dx.doi.org/10.1016/j.anbehav.2007.03.010
- Taylor RC, Klein BA, Stein J, Ryan MJ. Faux frogs: multimodal signalling and the value of robotics in animal behaviour. Anim Behav 2008; 76:1089 - 97; http://dx.doi.org/10.1016/j.anbehav.2008.01.031
- Caldwell MS, Johnston GR, McDaniel JG, Warkentin KM. Vibrational signaling in the agonistic interactions of red-eyed treefrogs. Curr Biol 2010; 20:1012 - 7; http://dx.doi.org/10.1016/j.cub.2010.03.069; PMID: 20493702
- Rosenthal GG, Rand AS, Ryan MJ. The vocal sac as a visual cue in anuran communication: an experimental analysis using video playback. Anim Behav 2004; 68:55 - 8; http://dx.doi.org/10.1016/j.anbehav.2003.07.013
- Lettvin JY, Maturana HR, McCulloch WS, Pitts WH. What the frog’s eye tells the frog’s brain. In: Corning, WC Balaban, M, eds. The Mind: Biological Approaches to its Functions. New York, NY: J Wiley, 1968:233-258.
- Land MF, Nilsson DE. Animal Eyes. New York, NY: Oxford University Press, 2002.
- Akre KL, Ryan MJ. Female túngara frogs elicit more complex mating signals from males. Behav Ecol 2011; 22:846 - 53; http://dx.doi.org/10.1093/beheco/arr065
- Jeffords MR, Sternburg JG, Waldbauer GP. Batesian mimicry: field demonstration of the survival value of pipevine swallowtail and monarch color patterns. Evolution 1979; 33:275 - 86; http://dx.doi.org/10.2307/2407618
- Sato H, Maharbiz MM. Recent developments in the remote radio control of insect flight. Front Neurosci 2010; 4(Article 199):1-11; 10.3389/fnins.2010.00199.
- Maharbiz MM, Sato H. Cyborg beetles. Sci Am 2010; 303:94 - 9; http://dx.doi.org/10.1038/scientificamerican1210-94; PMID: 21141365
- Woo KL, Rieucau G. From dummies to animations: a review of computer-animated stimuli used in animal behavior studies. Behav Ecol Sociobiol 2011; 65:1671 - 85; http://dx.doi.org/10.1007/s00265-011-1226-y
- D’Eath RB. Can video images imitate real stimuli in animal behaviour experiments?. Biol Rev Camb Philos Soc 1998; 73:267 - 92; http://dx.doi.org/10.1017/S0006323198005179