Abstract
Non-symbiotic hemoglobins (nsHbs) are O2-binding proteins widely distributed in land plants, including primitive bryophytes. Little is known about the properties of bryophyte nsHbs. Here, we report the spectroscopic characterization of two moss recombinant nsHbs, CerpurnsHb of Ceratodon purpureus and PhypatnsHb of Physcomitrella patens. Spectra showed that the absorption maxima of the ferrous and ferric forms of recombinant CerpurnsHb are located at 418, 531 and 557 nm and 407, 537, 569 (shoulder) and 632 (shoulder) nm, respectively, and of PhypatnsHb are located at 422, 529 and 557 nm and 407, 531, 571 (shoulder) and 647 (shoulder) nm, respectively. These absorption maxima are similar to those of rice Hb1. Also, the absorption maxima of the oxygenated ferrous form of recombinant CerpurnsHb and PhypatnsHb are located at 412, 541 and 575 nm and 414, 541 and 574 nm, respectively, similar to those of oxygenated rice Hb1 and cowpea leghemoglobin II. This evidence indicates that CerpurnsHb and PhypatnsHb are mostly hexacoordinate and that they bind O2.
Non-symbiotic hemoglobins (nsHbs) are O2-binding proteins widely distributed in land plants, from primitive bryophytes to evolved monocots and dicots.Citation1 Based on O2-affinity and sequence similarity nsHbs are classified into type 1 and type 2 (nsHbs-1 and nsHbs-2, respectively).Citation2,Citation3 The O2-affinity of nsHbs-1 is very high because of an extremely low O2-dissociation rate constant.Citation3-Citation5 Analyses by X-ray crystallography, site-directed mutagenesis and visible spectroscopy revealed that the extremely low O2-dissociation rate constant of nsHbs-1 primarily results from Fe-heme hexacoordination by distal His.Citation4,Citation6 Absorption spectra of nsHbs-1 are similar to those of other Hbs, however nsHbs-1 exhibit distinctive absorption maxima in the deoxyferrous (Hb2+) form. Specifically, nsHb-12+ exhibits peaks at ~526 and ~556 nm, which is characteristic of hexacoordinate Hbs.Citation4,Citation7 In contrast, pentacoordinate Hbs exhibit a broad peak centered at ~556 nm in their Hb2+ form.Citation7,Citation8
Little is known about the biophysical properties of primeval land plant nsHbs (e.g., the postulated ancestors of nsHbs-1 and nsHbs-2 1), such as bryophyte nsHbs. To better understand these nsHbs, the structure of a moss (Ceratodon purpureus) nsHb (CerpurnsHb) was modeled.Citation9 The predicted structure suggested that Fe-heme in this protein is hexacoordinate, however this observation has not been verified experimentally. Here, we report the generation and spectroscopic characterizations of recombinant CerpurnsHb and Physcomitrella patens nsHb (PhypatnsHb).
We generated recombinant CerpurnsHb and PhypatnsHb from plasmids pCR2.1::CerHbCitation9 and pCRII::B46,Citation10 essentially as described by Arredondo-Peter et al.Citation4,Citation8 Inserts were subcloned into the plasmid pET28b (Novagen), generating constructs pET28b::CerpurnsHb and pET28b::PhypatnsHb, then transformed into E. coli Tuner(DE3)pLacI. The inserts within plasmids pET28b::CerpurnsHb and pET28b::PhypatnsHb were fully sequenced. Soluble extracts were obtained from recombinant E. coli pET28b::CerpurnsHb and pET28b::PhypatnsHb, and recombinant CerpurnsHb and PhypatnsHb were detected by SDS-PAGE. Soluble extracts were subjected to spectroscopic analysis using soluble extracts from untransformed E. coli Tuner(DE3)pLacI as blanks. Ferrous Hb was oxidized to ferric (Hb3+) Hb by adding potassium ferricyanide; Hb2+ was formed by adding sodium dithionite; and air was bubbled through the Hb2+ solution to generate the O2-ligated (Hb2+O2) form of Hb.
DNA sequencing detected no mutations within the inserts of plasmids pET28b::CerpurnsHb and pET28b::PhypatnsHb. Thus, the sequences of recombinant CerpurnsHb and PhypatnsHb were identical to that predicted by the pCR2.1::CerHb and pCRII::B46 plasmids. SDS-PAGE analysis showed that recombinant CerpurnsHb and PhypatnsHb of the expected molecular masses (19.6 and 19.9 KD, respectively) were synthesized by E. coli Tuner(DE3)pLacI (). Spectra of recombinant CerpurnsHb and PhypatnsHb were highly similar to those of other Hbs (). The absorption maxima of Hb2+ and Hb3+ forms of recombinant CerpurnsHb are located at 418, 531 and 557 nm and 407, 537, 569 (shoulder) and 632 (shoulder) nm, respectively, and in PhypatnsHb at 422, 529 and 557 nm and 407, 531, 571 (shoulder) and 647 (shoulder) nm, respectively, similar to those of rice Hb1. Also, the absorption maxima of the Hb2+O2 form of recombinant CerpurnsHb and PhypatnsHb were located at 412, 541 and 575 nm and 414, 541 and 574 nm, respectively, similar to those of oxygenated rice Hb1 and cowpea LbII (). This evidence indicates that CerpurnsHb and PhypatnsHb are hexacoordinate and that they bind O2. However, the 531 nm maximum of CerpurnsHb2+ and 529 nm maximum of PhypatnsHb2+ are weak compared with the maximum of rice Hb1.Citation4 This observation suggests that hexacoordination is partial in both CerpuprnsHb2+ and PyspatnsHb2+ and that these proteins may exist in a mixture of hexa- and pentacoordinate forms. Thus, it is likely that the O2-affinities of CerpurnsHb and PhypatnsHb are higher than those reported for other hexacoordinate land plant nsHbs. An unusual characteristic of the oxygenated CerpurnsHb2+ and PhypatnsHb2+ spectra was the existence of absorption peaks at 645 nm ( and ). These spectra are similar to that from recombinant human histoglobin obtained from E. coli grown in a fermentation apparatus aerated using pure oxygen.Citation11 This observation suggests that Fe-heme is in the high-spin form in oxygenated CerpurnsHb2+ and PhypatnsHb2+, however the origin of peaks at 645 nm is not known. The results reported here provide knowledge of the spectroscopic properties of bryophyte nsHbs and corroborate the Fe-heme hexacoordination predicted for modeled CerpurnsHb.Citation9
Figure 1. Detection of recombinant CerpurnsHb and PhypatnsHb synthesized by E. coli Tuner(DE3)pLacI. Aliquots (~30 μg of total protein) were separated by SDS-PAGE in a 15% gel. Line 1, molecular mass markers; lines 2 and 3, soluble extract of the untransformed E. coli Tuner(DE3)pLacI; line 4, soluble extract of the E. coli Tuner(DE3)pLacI transformed with the pET28b::CerpurnsHb construct; line 5, soluble extract of the E. coli Tuner(DE3)pLacI transformed with the pET28b::PhypatnsHb construct. Arrows indicate the recombinant CerpurnsHb and PhypatnsHb. Markers are shown in kD.
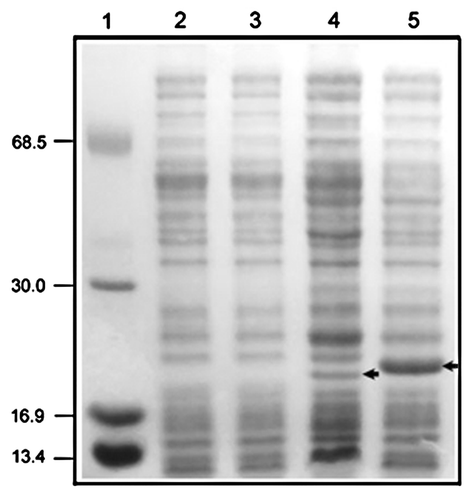
Figure 2. Absorption spectra of E. coli Tuner(DE3)pLacI soluble extracts containing the recombinant CerpurnsHb (A) and PhypatnsHb (B). Blue lines, Hb3+ form; red lines, Hb2+O2 form; and black lines, Hb2+ form.
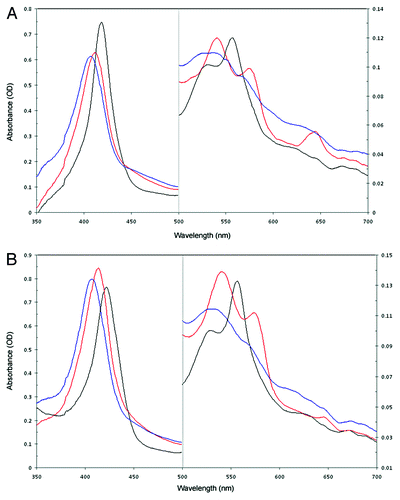
Table 1. Spectral characteristics of C. purpureus and P. patens recombinant nsHbs and hexacoordinate rice Hb1Citation4 and human neuroglobin,Citation12 and pentacoordinate cowpea LbIICitation8
Abbreviations: | ||
CerpurnsHb | = | Ceratodon purpureus non-symbiotic hemoglobin |
Hb | = | hemoglobin |
Lb | = | leghemoglobin |
nsHb | = | non-symbiotic hemoglobin |
PhypatnsHb | = | Physcomitrella patens non-symbiotic hemoglobin |
Acknowledgments
Authors wish to express their gratitude to Miss Gillian Klucas for English corrections. Work in the authors´ laboratory has been funded by SEP-PROMEP (grant no. UAEMor-PTC-01–01/PTC23) and Consejo Nacional de Ciencia y Tecnología (CoNaCyT grant nos. 25229N and 42873Q), México. C. V.-L. is a postdoctoral fellow supported by CoNaCyT.
Disclosure of Potential Conflicts of Interest
No potential conflicts of interest were disclosed.
References
- Vázquez-Limón C, Hoogewijs D, Vinogradov SN, Arredondo-Peter R. The evolution of land plant hemoglobins. Plant Sci 2012; 191-192:71 - 81; http://dx.doi.org/10.1016/j.plantsci.2012.04.013; PMID: 22682566
- Smagghe BJ, Hoy JA, Percifield R, Kundu S, Hargrove MS, Sarath G, et al. Review: correlations between oxygen affinity and sequence classifications of plant hemoglobins. Biopolymers 2009; 91:1083 - 96; http://dx.doi.org/10.1002/bip.21256; PMID: 19441024
- Trevaskis B, Watts RA, Andersson CR, Llewellyn DJ, Hargrove MS, Olson JS, et al. Two hemoglobin genes in Arabidopsis thaliana: the evolutionary origins of leghemoglobins. Proc Natl Acad Sci U S A 1997; 94:12230 - 4; http://dx.doi.org/10.1073/pnas.94.22.12230; PMID: 9342391
- Arredondo-Peter R, Hargrove MS, Sarath G, Moran JF, Lohrman J, Olson JS, et al. Rice hemoglobins. Gene cloning, analysis, and O2-binding kinetics of a recombinant protein synthesized in Escherichia coli. Plant Physiol 1997; 115:1259 - 66; http://dx.doi.org/10.1104/pp.115.3.1259; PMID: 9390447
- Duff SMG, Wittenberg JB, Hill RD. Expression, purification, and properties of recombinant barley (Hordeum sp.) hemoglobin. Optical spectra and reactions with gaseous ligands. J Biol Chem 1997; 272:16746 - 52; http://dx.doi.org/10.1074/jbc.272.27.16746; PMID: 9201978
- Hargrove M, Brucker EA, Stec B, Sarath G, Arredondo-Peter R, Klucas RV, et al. Crystal structure of a non-symbiotic hemoglobin. Structure 2000; 8:1005 - 14; http://dx.doi.org/10.1016/S0969-2126(00)00194-5; PMID: 10986467
- Kakar S, Hoffman FG, Storz JF, Fabian M, Hargrove MS. Structure and reactivity of hexacoordinate hemoglobins. Biophys Chem 2010; 152:1 - 14; http://dx.doi.org/10.1016/j.bpc.2010.08.008; PMID: 20933319
- Arredondo-Peter R, Moran JF, Sarath G, Luan P, Klucas RV. Molecular cloning of the cowpea (Vigna unguiculata) leghemoglobin II gene and expression of its cDNA in Escherichia coli; purification and characterization of the recombinant protein. Plant Physiol 1997; 114:493 - 500; http://dx.doi.org/10.1104/pp.114.2.493; PMID: 9193085
- Garrocho-Villegas V, Arredondo-Peter R. Molecular cloning and characterization of a moss (Ceratodon purpureus) nonsymbiotic hemoglobin provides insight into the early evolution of plant nonsymbiotic hemoglobins. Mol Biol Evol 2008; 25:1482 - 7; http://dx.doi.org/10.1093/molbev/msn096; PMID: 18420592
- Arredondo-Peter R, Ramírez M, Sarath G, Klucas RV. Sequence analysis of an ancient hemoglobin cDNA isolated from the moss Physcomitrella patens (Accession No. AF218049). Plant Physiol 2000; 122:1457; PMID: 10759541
- Trent JT 3rd, Hargrove MS. A ubiquitously expressed human hexacoordinate hemoglobin. J Biol Chem 2002; 277:19538 - 45; http://dx.doi.org/10.1074/jbc.M201934200; PMID: 11893755
- Dewilde S, Kiger L, Burmester T, Hankeln T, Baudin-Creuza V, Aerts T, et al. Biochemical characterization and ligand binding properties of neuroglobin, a novel member of the globin family. J Biol Chem 2001; 276:38949 - 55; http://dx.doi.org/10.1074/jbc.M106438200; PMID: 11473128