Abstract
It is becoming evident that physical forces in the microenvironment play a key role in regulating many important aspects of cell biology. However, although mechanical cues are known to have clear effects over the long-term (days), the short-term (seconds to minutes) cellular responses to mechanical stimuli are less well characterized. In our recent study, we exposed committed fibroblast cells to well controlled nanoscale forces while simultaneously imaging force transduction through the actin cytoskeleton. One of the earliest responses of a cell to physical force is rapid deformation of the cytoskeleton, taking place over the course of seconds. We were able to directly visualize deformation, force-propagation and strain dynamics in actin stress fibers in response to a relatively simple mechanical stimulus. Moreover, these dynamics were also dependent on myosin-driven contractility and the presence of an intact microtubule cytoskeleton. Interestingly, although stem cells are sensitive to mechanical cues, they do not display the same degree of stress fiber organization as observed in committed cells indicating the possibility of alternative sensing and mechanotransduction mechanisms.
The cellular cytoskeleton is a complex interconnected network, composed of a dynamic and complex array of structural and regulatory proteins that permit the cell to adapt the many cues in its microenvironment.Citation1 Specifically, living cells actively respond to mechanical forces and changes in the material properties of the microenvironment.Citation2,Citation3 These mechanical cues have a significant role to play in regulating and controlling cell biology and behavior.Citation2,Citation3 The process of mechanotransduction (the conversion of mechanical cues into biological outcomes) is under intense investigation as these pathways play key roles in a diverse number of processes including, determining stem cell fate, malignancy, migration, proliferation and myogenesis.Citation4-Citation8 Importantly, these processes take place over days to weeks and indicate the importance of mechanical cues acting over long timescales.
Of particular importance in the ability of a cell to respond to its mechanical microenvironment are actin stress fibers. These structures are constantly undergoing a state of reorganization, transmitting and relaying nanomechanical forces in the process. The cytoskeleton acts as an interface, intricately connecting the cell to the external microenvironment through physical connections at focal adhesion and integrin sites.Citation1,Citation3 Indeed, acto-myosin contractility plays a large role in the ability of cells to sense the mechanical properties of their microenvironment through the generation of traction forces.Citation9 Importantly, the physical properties of the microenvironment are also well known to alter cytoskeletal organization and cell behavior demonstrating the intimate link between the cytoarchitecture and the microenvironment.Citation1,Citation3 In addition to this, local forces applied to the cell membrane can result in force transduction through the cell, activation of mechanosensitive ion channels, secondary messenger release and gene regulation.Citation10-Citation17 As opposed to the processes discussed above, these events are activated rapidly, taking place on the second to minute timescale. However, the short-term response of a cell to mechanical force remains poorly understood even though it has a major role to play in the long-term outcome.Citation11
We recently generated NIH3T3 fibroblast cells transiently expressing actin-EGFP and mechanically stimulated them with well-controlled nanonewton forces with an atomic force microscope (AFM) mounted on a laser scanning confocal microscope (LSCM) ().Citation11 This approach allowed us to simultaneously expose cells to local nanonewton forces while quantifying force transduction through the actin cytoskeleton. With the AFM tip directly over the nucleus, forces from 0nN to 20nN were applied and actin deformation and force transduction was quantified. These studies revealed that following a locally applied force, the F-actin cytoskeleton does not undergo a global isotropic deformation, but rather, highly localized and heterogeneous deformation occurring throughout the cell (). Two distinct actin displacements were observed: an initial localized deformation occurring within ~20μm from the point of contact (but not at the point of contact), followed by a localized deformation far from the point of contact, including the retraction of distant stress fibers at the cell edges due to focal adhesion remodeling. To assess the involvement of the microtubule network, cells were pre-treated with 10μM of nocodazole, a microtubule polymerizing inhibitor.Citation11 Results indicate that the actin cytoskeleton underwent a much smaller deformation, comparable to that of control cells not subjected to any force. This suggests that the microtubule network is highly involved in anisotropic force transduction pathways observed following a nanomechanical force. In addition, we utilized an approach in which actin stress fibers were patterned into segments by photobleaching the EGFP every 5μm along their length ().Citation11,Citation18,Citation19 This allowed us to quantify any local contraction or stretching (strain) of stress fibers by simply quantifying changes in length of each individual segment. We observed that stress fibers underwent heterogeneous strain fluctuations all along their length, exhibiting a complex array of regions undergoing stretch and contraction (). In response to mechanical stimulation we observed a ~50% increase in the magnitude of the strain fluctuations along stress fibers and no clear signs of concerted contraction or stretch along the entire length of the fiber. These results imply that the elasticity (or Young’s modulus) of actin stress-fibers varies along their length. This is likely due to the cross-linked nature of the actin cytoskeleton. Regions more highly cross-linked will have a high effective elasticity than regions with fewer crosslinks. Therefore, acto-myosin driven contractility would cause strain dynamics that would appear heterogeneous.
Figure 1. Applying local mechanical forces to living NIH3T3 cells with simultaneous AFM and LSCM. (A) An NIH3T3 cells transiently expressing actin-EGFP. The AFM cantilever is positioned above the cell nucleus and used to apply local nanonewton forces. (B) Heat map lateral actin deformation in a cell exposed to a 20nN stimulus at the position marked with an ‘x’. Highly localized deformations take place far form the point of contact and evolve in time. Experimental details in reference Citation11. Figure adapted from reference Citation11. Scale bars in both (A) and (B) are 15 μm.
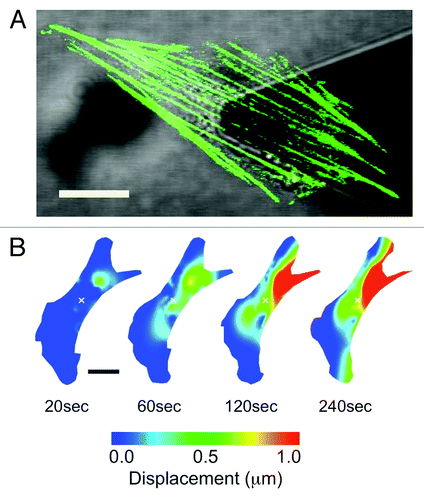
Figure 2. Quantifying strain dynamics in actin stress fibers. (A) Actin-EGFP stress fibers photobleached every 5 μm to create a segmented appearance (stress fibers do remain intact). As the stress fibers undergo stretch and contraction the segments will change length allowing the quantification of internal strain. (B) Strain dynamics taking place along stress fibers are heterogeneous and evolve in time. Negative strain values correspond to regions undergoing contraction and positive strain values correspond to regions undergoing stretch. Experimental details in reference Citation11. Figure adapted from reference Citation11. Scale bars in both (A) and (B) are 15 μm.
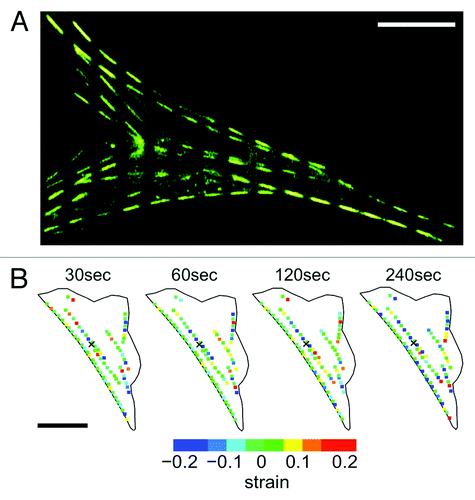
Our study has demonstrated that force-induced cytoskeletal remodeling occurs through a complex and localized response that is highly dependent on the presence of myosin-driven contractility and an intact microtubule network. A possible explanationCitation11 for the observed strain dynamics involves increased Ca2+ concentration following an applied force, which would induce a calmodulin-dependent activation of myosin light chain kinase (MLCK). Myosin II is phosphorylated by this kinase in addition to ROCK (rho kinase), causing an increase in stress fiber formation and contraction.
Interestingly, uncommitted stem cells are well known to exhibit extreme sensitivity to mechanical cues occurring in the microenvironment. Stem cells will commit to a particular lineage based on the elasticity of the matrix on which they are propagating. As well, local and global mechanical stresses occurring in the microenvironment can also stimulate cells to commit to a particular fate.Citation20,Citation21 However, as demonstrated in another previous study from our lab,Citation22 mouse embryonic stem cells (E14 and ES-D3 cell lines) do not display well-defined actin stress-fibers comparable to those observed in committed cells (). Conversely, we found that these cells display an intact microtubule cytoskeleton. Moreover, it has been well documented that stem cells generally display a poorly organized and diffuse actin cytoskeleton in soft microenvironments.Citation7,Citation23,Citation24 Therefore, we speculate that uncommitted stem cells may possess alternative, yet complementary, pathways to sense and respond to mechanical cues occurring in their microenvironment. Importantly, it is becoming increasingly recognized that microtubules also play an important role in governing the mechanical properties of the cell as they play a role in generating traction forces and mediating focal adhesion dynamics.Citation25-Citation27 Our recent studyCitation11 has revealed that local nanoscale forces are rapidly and anisotropically transduced and focused throughout the cell body. However, these pathways are unlikely to be universal, rather they are likely dependent on cell type, the mechanical properties of the microenvironment and possibly even the development stage of the cell. This rich biological complexity provides many opportunities for the study of the fundamental mechanical pathways that have such clear importance in diverse aspects of cell biology.
Figure 3. Actin stress fibers in (A) NIH3T3 and (B) pluripotent ES-D3 mouse embryonic stem cells (mESCs). Cells were fixed and stained for actin (phalloidin conjugated to Alexa Fluor 546, white) and the nucleus (DAPI, blue). Stress fibers are numerous and highly organized in committed NIH3T3 cells. Pluripotent mESCs (confirmed by staining for the presence of the transcription factor OCT4, not shown) are generally significantly smaller than NIH3T3 cells and display fewer stress fibers. The actin is generally diffuse and poorly organized. However, small punctate actin protrusions are observed. Experimental details in reference Citation11. Scale bars in both (A) and (B) are 20 μm.
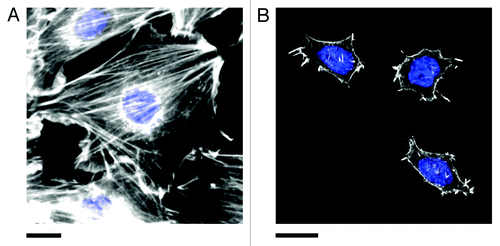
Disclosure of Potential Conflict of Interest
No potential conflicts of interest were disclosed.
Acknowledgments
L.G. was supported by graduate fellowships from the Natural Sciences and Engineering Research Council (NSERC) and the Ontario Graduate Scholarships program. A.E.P. gratefully acknowledges generous support from the Canada Research Chairs (CRC) program and a Province of Ontario Early Researcher Award. This work was supported by an NSERC Discovery Grant, an NSERC Discovery Accelerator Supplement and a CRC Operating Grant.
References
- Fletcher DA, Mullins RD. Cell mechanics and the cytoskeleton. Nature 2010; 463:485 - 92; http://dx.doi.org/10.1038/nature08908; PMID: 20110992
- Janmey PA, Miller RT. Mechanisms of mechanical signaling in development and disease. J Cell Sci 2011; 124:9 - 18; http://dx.doi.org/10.1242/jcs.071001; PMID: 21172819
- Buxboim A, Ivanovska IL, Discher DE. Matrix elasticity, cytoskeletal forces and physics of the nucleus: how deeply do cells ‘feel’ outside and in?. J Cell Sci 2010; 123:297 - 308; http://dx.doi.org/10.1242/jcs.041186; PMID: 20130138
- Butcher DT, Alliston T, Weaver VM. A tense situation: forcing tumour progression. Nat Rev Cancer 2009; 9:108 - 22; http://dx.doi.org/10.1038/nrc2544; PMID: 19165226
- DuFort CC, Paszek MJ, Weaver VM. Balancing forces: architectural control of mechanotransduction. Nat Rev Mol Cell Biol 2011; 12:308 - 19; http://dx.doi.org/10.1038/nrm3112; PMID: 21508987
- Engler AJ, Griffin MA, Sen S, Bönnemann CG, Sweeney HL, Discher DE. Myotubes differentiate optimally on substrates with tissue-like stiffness: pathological implications for soft or stiff microenvironments. J Cell Biol 2004; 166:877 - 87; http://dx.doi.org/10.1083/jcb.200405004; PMID: 15364962
- Engler AJ, Sen S, Sweeney HL, Discher DE. Matrix elasticity directs stem cell lineage specification. Cell 2006; 126:677 - 89; http://dx.doi.org/10.1016/j.cell.2006.06.044; PMID: 16923388
- Kumar S, Weaver VM. Mechanics, malignancy, and metastasis: the force journey of a tumor cell. Cancer Metastasis Rev 2009; 28:113 - 27; http://dx.doi.org/10.1007/s10555-008-9173-4; PMID: 19153673
- Chan CE, Odde DJ. Traction dynamics of filopodia on compliant substrates. Science 2008; 322:1687 - 91; http://dx.doi.org/10.1126/science.1163595; PMID: 19074349
- Hayakawa K, Tatsumi H, Sokabe M. Actin stress fibers transmit and focus force to activate mechanosensitive channels. J Cell Sci 2008; 121:496 - 503; http://dx.doi.org/10.1242/jcs.022053; PMID: 18230647
- Guolla L, Bertrand M, Haase K, Pelling AE. Force transduction and strain dynamics in actin stress fibres in response to nanonewton forces. J Cell Sci 2012; 125:603 - 13; http://dx.doi.org/10.1242/jcs.088302; PMID: 22389400
- Pelling AE, Veraitch FS, Chu CP, Mason C, Horton MA. Mechanical dynamics of single cells during early apoptosis. Cell Motil Cytoskeleton 2009; 66:409 - 22; http://dx.doi.org/10.1002/cm.20391; PMID: 19492400
- Na S, Collin O, Chowdhury F, Tay B, Ouyang M, Wang Y, et al. Rapid signal transduction in living cells is a unique feature of mechanotransduction. Proc Natl Acad Sci U S A 2008; 105:6626 - 31; http://dx.doi.org/10.1073/pnas.0711704105; PMID: 18456839
- Poh YC, Na S, Chowdhury F, Ouyang M, Wang Y, Wang N. Rapid activation of Rac GTPase in living cells by force is independent of Src. PLoS One 2009; 4:e7886; http://dx.doi.org/10.1371/journal.pone.0007886; PMID: 19924282
- Wang J, Chen H, Seth A, McCulloch CA. Mechanical force regulation of myofibroblast differentiation in cardiac fibroblasts. Am J Physiol Heart Circ Physiol 2003; 285:H1871 - 81; PMID: 12842814
- Sbrana F, Sassoli C, Meacci E, Nosi D, Squecco R, Paternostro F, et al. Role for stress fiber contraction in surface tension development and stretch-activated channel regulation in C2C12 myoblasts. Am J Physiol Cell Physiol 2008; 295:C160 - 72; http://dx.doi.org/10.1152/ajpcell.00014.2008; PMID: 18480300
- Formigli L, Meacci E, Sassoli C, Chellini F, Giannini R, Quercioli F, et al. Sphingosine 1-phosphate induces cytoskeletal reorganization in C2C12 myoblasts: physiological relevance for stress fibres in the modulation of ion current through stretch-activated channels. J Cell Sci 2005; 118:1161 - 71; http://dx.doi.org/10.1242/jcs.01695; PMID: 15728255
- Kölsch A, Windoffer R, Würflinger T, Aach T, Leube RE. The keratin-filament cycle of assembly and disassembly. J Cell Sci 2010; 123:2266 - 72; http://dx.doi.org/10.1242/jcs.068080; PMID: 20554896
- Colombelli J, Besser A, Kress H, Reynaud EG, Girard P, Caussinus E, et al. Mechanosensing in actin stress fibers revealed by a close correlation between force and protein localization. J Cell Sci 2009; 122:1665 - 79; http://dx.doi.org/10.1242/jcs.042986; PMID: 19401336
- Guilak F, Cohen DM, Estes BT, Gimble JM, Liedtke W, Chen CS. Control of stem cell fate by physical interactions with the extracellular matrix. Cell Stem Cell 2009; 5:17 - 26; http://dx.doi.org/10.1016/j.stem.2009.06.016; PMID: 19570510
- Chowdhury F, Na S, Li D, Poh YC, Tanaka TS, Wang F, et al. Material properties of the cell dictate stress-induced spreading and differentiation in embryonic stem cells. Nat Mater 2010; 9:82 - 8; http://dx.doi.org/10.1038/nmat2563; PMID: 19838182
- Hemsley AL, Hernandez D, Mason C, Pelling AE, Veraitch FS. Precisely delivered nanomechanical forces induce blebbing in undifferentiated mouse embryonic stem cells. Cell Health Cytoskeleton 2011; 3:23 - 34
- Guvendiren M, Burdick JA. Stiffening hydrogels to probe short- and long-term cellular responses to dynamic mechanics. Nat Commun 2012; 3:792; http://dx.doi.org/10.1038/ncomms1792; PMID: 22531177
- Discher DE, Janmey P, Wang YL. Tissue cells feel and respond to the stiffness of their substrate. Science 2005; 310:1139 - 43; http://dx.doi.org/10.1126/science.1116995; PMID: 16293750
- Ezratty EJ, Partridge MA, Gundersen GG. Microtubule-induced focal adhesion disassembly is mediated by dynamin and focal adhesion kinase. Nat Cell Biol 2005; 7:581 - 90; http://dx.doi.org/10.1038/ncb1262; PMID: 15895076
- Palazzo AF, Gundersen GG. Microtubule-actin cross-talk at focal adhesions. Sci STKE 2002; 2002:pe31.
- Rape A, Guo WH, Wang YL. Microtubule depolymerization induces traction force increase through two distinct pathways. J Cell Sci 2011; 124:4233 - 40; http://dx.doi.org/10.1242/jcs.090563; PMID: 22193960