Abstract
CFTR is a PKA activated Cl- channel expressed in the apical membrane of fluid transporting epithelia. We previously demonstrated that c-Cbl decreases CFTR stability in the plasma membrane by facilitating its endocytosis and lysosomal degradation in human airway epithelium. The most common mutation associated with cystic fibrosis, deletion of Phe508 (∆F508), leads to a temperature sensitive biosynthetic processing defect in the CFTR protein. Mature ∆F508-CFTR that has been rescued by low temperature or chemical chaperones is partially functional as a Cl- channel but has decreased plasma membrane stability due to altered post-maturational trafficking. Our present data demonstrate that c-Cbl controls the post-maturational trafficking of rescued ∆F508-CFTR. Partial depletion of c-Cbl increased stability of the plasma membrane associated mature ∆F508-CFTR and the ∆F508-CFTR mediated Cl- secretion. These data indicate that correcting the post-maturational trafficking of ∆F508-CFTR may represent a therapeutic approach complementary to the biosynthetic rescue. Because c-Cbl functions as an adaptor and scaffolding protein during CFTR endocytosis, we propose that interfering with the c-Cbl mediated endocytic recruitment of ∆F508-CFTR may increase stability of ∆508-CFTR in the plasma membrane after its biosynthetic rescue.
Introduction
The cystic fibrosis transmembrane conductance regulator (CFTR) is a cAMP-activated Cl- channel that mediates transepithelial Cl- secretion in various fluid-transporting epithelia.Citation1-Citation3 Dysfunctions of the CFTR protein caused by CFTR gene mutations result in reduction or absence of Cl- transport and lead to a common fatal genetic disease, cystic fibrosis (CF).Citation4 In the airway, CFTR plays a critical role in regulating mucociliary clearance by maintaining the airway surface liquid.Citation5,Citation6 Control of CFTR mediated Cl- secretion across polarized epithelial cells is achieved at the level of both CFTR Cl- channel activity and protein stability in the plasma membrane.Citation7,Citation8 The plasma membrane stability of CFTR depends on its biosynthetic processing and post-maturational trafficking, which involves endocytic uptake followed either by recycling to the plasma membrane or degradation in the lysosome.Citation4,Citation8 The long plasma membrane stability of CFTR stands in contrast to its inefficient biosynthetic processing and depends primarily on efficient recycling to compensate for rapid endocytosis.Citation9,Citation10
Both the biosynthetic processing and post-maturational trafficking of CFTR are critically affected by the most common disease-associated mutation in the CFTR gene caused by loss of Phe508 (∆F508).Citation4 The ∆F508 mutation leads to a temperature sensitive processing defect that results in biosynthetic arrest of the ∆F508-CFTR protein in the immature, partially glycosylated form (band B) retained in the endoplasmic reticulum (ER). Low temperature and chemical chaperones rescue the biosynthetic processing defect of ∆F508-CFTR. The biosynthetic rescue allows the immature form of ∆F508-CFTR to exit the ER, complete glycosylation while passing through the Golgi system, and traffic to the cell membrane as a mature band C. Because rescued ∆F508-CFTR (r∆F508-CFTR) is partially functional as a Cl- channel, therapies based on correction of the biosynthetic processing defect have been highly anticipated.Citation11-Citation13 Despite showing promising results in cultured cells, a small molecule corrector VX-809 had disappointing results in patients homozygous for the ∆F508 mutation.Citation14 r∆F508-CFTR has decreased plasma membrane stability due to altered post-maturational trafficking.Citation15,Citation16 Correcting the post-maturational trafficking defect of r∆F508-CFTR may represent a therapeutic approach complementary to the biosynthetic rescue. Thus, CFTR interactions with protein adaptors involved in either endocytic uptake or lysosomal degradation or both are the logical targets for stabilizing CFTR at the plasma membrane. Indeed, targeting Dab2 or the CFTR associated ligand (CAL) increases plasma membrane stability and Cl- channel function of ∆F508-CFTR.Citation17-Citation22 Similarly, silencing c-Cbl increases the plasma membrane stability and Cl- channel function of the wild type (WT)-CFTR.Citation23 The effects of c-Cbl on the r∆F508-CFTR stability and Cl- channel function have not been determined.
c-Cbl is a multifunctional protein with ubiquitin ligase activity and protein adaptor function.Citation24 Our recently published data demonstrate that c-Cbl facilitates CFTR endocytosis functioning as a protein scaffold rather than the ubiquitin ligase.Citation23 The ubiquitin ligase activity of c-Cbl is required during the post-endocytic trafficking of CFTR to the lysosome.Citation23 Data presented in this manuscript extend our previous work and demonstrate that c-Cbl controls the post-maturational trafficking and function of r∆F508-CFTR in polarized human airway epithelial cells. Our data indicate that correcting the post-maturational trafficking defect of r∆508-CFTR could increase the efficacy of the biosynthetic rescue of ∆508-CFTR. We propose that targeting the c-Cbl mediated endocytic recruitment of r∆F508-CFTR may serve as a useful biochemical tool to uncovering future disease modifying approaches for CF complementary to biosynthetic rescue.
Results
Silencing c-Cbl increases abundance of the the plasma membrane associated r∆F508-CFTR in polarized human airway epithelial cells
Studies were conducted to determine whether c-Cbl regulates the post-maturational trafficking of r∆F508-CFTR. If c-Cbl facilitates r∆F508-CFTR endocytosis and lysosomal degradation, we predicted that c-Cbl depletion would attenuate the post-maturational degradation and thus, would increase the plasma membrane abundance of r∆F508-CFTR. To test this prediction, c-Cbl abundance was reduced in CFBE41o- cells stably expressing ∆F508-CFTR by RNA mediated interference (siRNA). CFBE41o- cells were transfected with siRNA specific for a non-conserved region of the human c-Cbl sequence (si-c-Cbl) or with a non-silencing, negative siRNA control (si-CTRL) as previously described.Citation23 Low temperature (27°C) was used to facilitate the biosynthetic processing and rescue expression of the mature, fully glycosylated band C of ∆F508-CFTR.Citation16 In addition, the effect of silencing c-Cbl on the biosynthetic processing of ∆F508-CFTR was examined in cells cultured exclusively at 37°C. Partial silencing of c-Cbl increased the steady-state abundance of r∆F508-CFTR band C (). By contrast, partial silencing of c-Cbl did not affect the abundance of the partially glycosylated or band B of ∆F508-CFTR after temperature rescue, indicating that c-Cbl does not affect ∆F508-CFTR protein translation (). Moreover, in the absence of temperature rescue, partial depletion of c-Cbl did not increase the abundance of ∆F508-CFTR band C, indicating that c-Cbl does not facilitate the biosynthetic processing of ∆F508-CFTR ().
Figure 1. western blots demonstrating the effects of c-Cbl depletion on ∆F508-CFTR abundance in whole cell lysates (WCL) at steady-state in polarized CFBE41o- cells. Cells were transfected with 50 nM siRNA against the human c-Cbl gene (s-ic-Cbl) or the non-silencing negative siRNA control (si-CTRL). Low temperature (27°C) for 48h was used to rescue the biosynthetic processing defect of ∆F508-CFTR. Representative western blots (A, the bottom panel) and summary of experiments (B) demonstrating that si-c-Cbl decreased the c-Cbl protein abundance in WCL. Low temperature (27°C) did not affect the c-Cbl silencing efficiency. Low temperature rescued the abundance of the mature, plasma membrane associated, fully glycosylated ∆F508-CFTR band C. Partial silencing of c-Cbl increased the steady-state abundance of ∆F508-CFTR band C in polarized CFBE41o- cells (A; the top panel and C). By contrast, partial silencing of c-Cbl did not increase the steady-state abundance of the partially glycosylated ∆F508-CFTR band B (A and C). Silencing c-Cbl in cells not subjected to low temperature (37°C) did not increase the abundance of the ∆F508-CFTR band C, indicating that c-Cbl does not facilitate the biosynthetic processing of ∆F508-CFTR (A). The ∆F508-CFTR abundance was normalized for actin. Actin expression was used as a loading control. *, p < 0.05 vs. siCTRL. Five experiments/group. Error bars, SE.
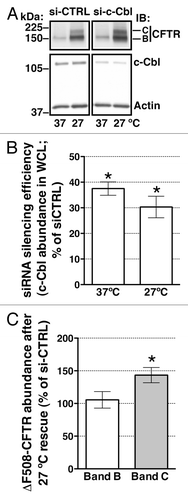
To gain additional evidence that the increased abundance of r∆F508-CFTR band C after c-Cbl depletion resulted from changes in the post-maturational trafficking rather than increased protein translation, we examined the disappearance of r∆F508-CFTR band C in polarized CFBE41o- cells over time at 37°C in the presence of 20 µg/ml cycloheximide to inhibit protein translation. As illustrated in and C, si-c-Cbl increased stability of r∆F508-CFTR band C without affecting the stability of other membrane associated proteins, namely the Epidermal Growth Factor Receptor (EGFR) and Transferrin Receptor (TfR). In additional experiments the stability of r∆F508-CFTR was monitored over time at 27°C ( and C). Low temperature alone decreased the disappearance of r∆F508-CFTR band C because protein trafficking and degradation slow down at 27°C (si-CTRL at 27°C vs. 37°C). Silencing c-Cbl had a synergistic effect and prevented the disappearance of r∆F508-CFTR band C compared with control. The complementary effect of si-c-Cbl and low temperature indicates that silencing c-Cbl attenuates r∆F508-CFTR endocytosis and degradation. By contrast, si-c-Cbl did not slow the disappearance of ∆F508-CFTR band B ( and D). Taken together, the above data indicate that in human airway epithelial cells, endogenous c-Cbl decreases stability of the plasma membrane associated r∆F508-CFTR by controlling its post-maturational trafficking.
Figure 2. western blots demonstrating the effects of c-Cbl depletion on ∆F508-CFTR abundance in whole cell lysates (WCL) in polarized CFBE41o- cells as a function of time. Cells were transfected with 50 nM siRNA against the human c-Cbl gene (si-c-Cbl) or the non-silencing negative siRNA control (si-CTRL). Low temperature (27°C) for 48h was used to facilitate the biosynthetic processing of ∆F508-CFTR band C. Disappearance of ∆F508-CFTR band C (A and C) or band B (A and D) from WCL was monitored over time in the presence of 20 µg/ml cycloheximide (CHX) at 37°C (A, C and D) or 27°C (B–D). In si-CTRL-transfected cells, at least 50% of ∆F508-CFTR band C disappeared in 4h. Thus, data are reported at the 4h time point (C and D). Representative western blots (A) and summary of experiments (C) demonstrating that partial silencing of c-Cbl attenuated the disappearance of the temperature rescued ∆F508-CFTR band C at 37°C without affecting the stability of the Epidermal Growth Factor Receptor (EGFR) or Transferrin Receptor (TfR). Low temperature (27°C) alone decreased the disappearance of the rescued ∆F508-CFTR band C and partial silencing of c-Cbl had a synergistic effect and completely prevented the disappearance of the temperature rescued ∆F508-CFTR band C (B and C). Partial silencing of c-Cbl did not attenuate the disappearance of the ∆F508-CFTR band B at 37°C or 27°C (A, B and D). The ∆F508-CFTR abundance was normalized for ezrin. Ezrin expression was used as a loading control. *, p < 0.05 vs. time zero in siCTRL. Five experiments/group. Error bars, SE.
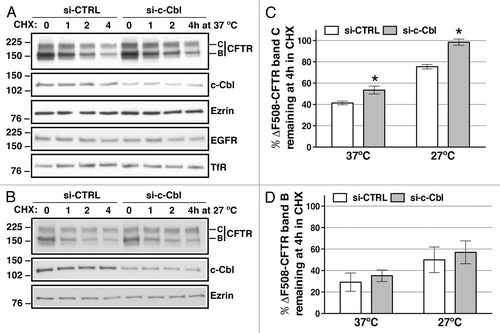
Silencing c-Cbl increases r∆F508-CFTR-mediated Cl- secretion across polarized human airway epithelial cells
Control of CFTR mediated Cl- secretion across polarized epithelial cells is achieved at the level of both CFTR Cl- channel activity and protein stability in the plasma membrane. Because silencing c-Cbl increased the abundance and stability of r∆F508-CFTR band C we assayed the functionality of the rescued fraction, predicting that it would also increase the r∆F508-CFTR mediated Cl- secretion. To facilitate the biosynthetic processing and rescue the expression of mature ∆F508-CFTR we used low temperature (27°C). Partial silencing of c-Cbl increased the forskolin/IBMX-stimulated Cl- currents (Isc) across CFBE41o- monolayers ( and B).
Figure 3. Ussing chamber experiments performed to determine the effects of c-Cbl depletion on the ∆F508-CFTR mediated Cl- secretion across CFBE41o- monolayers. Cells were transfected with 50 nM siRNA against the human c-Cbl gene (si-c-Cbl) or the non-silencing negative siRNA control (si-CTRL). Low temperature (27°C; A and B) or a corrector CF-106951 (C and D) for 48h was used to facilitate the biosynthetic processing and rescue abundance of ∆F508-CFTR band C. CFBE41o- cells were bathed in solutions with apical-to-basolateral Cl- gradient in the presence of amiloride (50 μM) in the apical bath solution to inhibit Na+ absorption through ENaC. Isc was stimulated with forskolin (20 μM) and IBMX (50 μM) added to the apical and basolateral bath solution. Thiazolidonone CFTRinh-172 (5 μM) was added to the apical bath solution to inhibit CFTR-mediated Isc. Data are expressed as net stimulated Isc, calculated by subtracting the baseline Isc from the peak stimulated Isc. Si-CTRL did not affect the forskolin/IBMX-stimulated Isc across CFBE41o- cells compared with the non-transfected cells (data not shown). Si-c-Cbl did not change the transepithelial resistance across cell monolayers (398.8 ± 72 Ωcm2 vs. 404.7 ± 140.8 Ωcm2 for the si-CTRL and si-c-Cbl transfected cells, respectively). Representative experiment (A) and summary of data (B) demonstrating that partial silencing of c-Cbl increased the forskolin/IBMX-stimulated Isc across CFBE41o-cells after the low temperature rescue. Moreover partial silencing of c-Cbl increased the forskolin/IBMX-stimulated Isc across CFBE41o-cells after the CF-106951 rescue (C and D). *, p < 0.05 vs. siCTRL. Six monolayers/group. Error bars, SE.
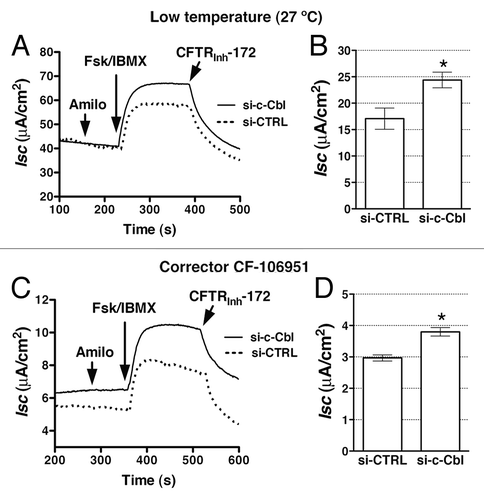
Low temperature is non-specific for correcting the biosynthetic defect of ∆F508-CFTR and is not suitable for use in patients. Several small molecules called CFTR correctors have been shown to rescue the functional defect of ∆F508-CFTR by correcting its biosynthetic processing defect in cultured cells.Citation11-Citation13,Citation25 The present generation of CFTR correctors does not improve the decreased plasma membrane stability of r∆F508-CFTR and their clinical efficacy has been very limited in patients homozygous for the ∆F508 mutation.Citation14,Citation25 Thus, we examined whether increasing stability of r∆F508-CFTR by silencing c-Cbl would complement the corrector effect on the functional defect of ∆F508-CFTR. Despite an overall lower Isc at 37°C compared with 27°C, partial silencing of c-Cbl increased the forskolin/IBMX-stimulated Isc across CFBE41o- monolayers after rescue with the CFTR corrector CF-106951 ( and D). These results are consistent with the above data that si-c-Cbl increased r∆F508-CFTR abundance and stability and demonstrate that endogenous c-Cbl inhibits the r∆F508-CFTR-mediated Cl- secretion by limiting the r∆F508-CFTR Cl- channel abundance in the plasma membrane in human airway epithelial cells. These data indicate that targeting the interaction between r∆F508-CFTR and c-Cbl could serve as a complementary approach to the biosynthetic rescue in order to corret the functional defect of ∆F508-CFTR.
Discussion
Data presented in this manuscript demonstrate that c-Cbl regulates the r∆F508-CFTR mediated Cl- secretion by controling the post-maturational trafficking of r∆F508-CFTR in polarized human airway epithelial cells. Several lines of evidence in the present study support our conclusion. Compared with controls, partial silencing of c-Cbl increased the steady-state abundance and stability of the mature, plasma membrane associated ∆F508-CFTR after its biosynthetic rescue (Figs. One and 2). Moreover, partial silencing of c-Cbl increased the r∆F508-CFTR mediated Cl- secretion (). We previously reported a similar effect of c-Cbl on the WT-CFTR and demonstrated that c-Cbl controls the post-maturational trafficking of WT-CFTR by facilitating WT-CFTR endocytosis and lysosomal degradation in human airway epithelial cells.Citation23 Data in this manuscript indicate that c-Cbl does not decrease the ∆F508-CFTR protein translation because c-Cbl depletion did not increase the abundance of the partially glycosylated ∆F508-CFTR (). Moreover, c-Cbl has no effect on the biosynthetic processing of the partially glycosylated immature ∆F508-CFTR because c-Cbl depletion did not increase the abundance of fully glycosylated mature ∆F508-CFTR in the absence of biosynthetic rescue (). Similar abundance of c-Cbl at 27°C and 37°C confirm that differences observed in experiments performed at these temperatures did not result from differences in siRNA silencing efficiency.
Correcting ∆F508-CFTR to resemble WT-CFTR functionally will require rescue of the biosynthetic processing defect, increasing the Cl- channel activity, and stabilizing r∆F508-CFTR in the plasma membrane. Recent data demonstrate promising effects of combining a corrector with a potentiator — an agent that increases the r∆F508-CFTR Cl- channel function — although the level of functional correction by such complementary therapy in CF patients is still far from that of WT-CFTR.Citation26 Partial depletion of c-Cbl increased stability of r∆F508-CFTR and increased the r∆F508-CFTR mediated Cl- secretion (Figs. Two and 3). Our data demonstrate that controlling the post-maturational trafficking of r∆F508-CFTR should be considered as a complementary approach to improve the efficacy of correction of the functional defect of ∆F508-CFTR in CF patients.
c-Cbl is a multifunctional protein critical for many cellular functions.Citation24 In our study depletion of c-Cbl by 60–70% did not change the stability of membrane receptors such as EGFR and TfR or the cytoskeletal proteins actin and ezrin and did not change the transepithelial resistance while increasing the stability and function of r∆F508-CFTR (–). We previously demonstrated that off-target effects can be prevented by partial depletion of target proteins at levels similar to those used in our study.Citation17,Citation23 Depletion of endogenous c-Cbl is a useful approach to elucidate its function in the post-maturational trafficking of r∆F508-CFTR in cultured cells. Such approach would be impractical in vivo where control of the post-maturational trafficking of r∆F508-CFTR requires high specificity. Targeting directly the interaction between c-Cbl and r∆F508-CFTR will allow c-Cbl to control other cellular functions and thus, will be highly specific. We propose that targeting the c-Cbl–r∆F508-CFTR interaction may serve as a useful biochemical tool to uncovering disease modifying, complementary approaches for CF. Future studies will focus on elucidating the complex formation between c-Cbl and r∆F508-CFTR in human airway epithelial cells.
Materials and Methods
Cell lines and cell culture
CFBE41o- cells stably expressing ∆F508-CFTR were a generous gift from Dr. J.P. Clancy, Cincinnati Children’s Hospital Medical Center Cincinnati, OH.Citation27 CFBE41o- cells were seeded on Transwell (4.67 cm2 or 44 cm2) (Corning Corporation) permeable supports coated with plating medium containing Dulbecco’s modified Eagle’s medium (DMEM; Invitrogen, Carlsbad, CA) and 10% purified collagen (PureColTM; Advanced Biomatrix, San Diego, CA) and maintained as polarized monolayers in air-liquid interface culture as described previously.Citation16,Citation17,Citation23 To rescue the biosynthetic processing of ∆F508-CFTR cells were cultured in fresh medium at 27°C for 48 h before experiments.Citation16,Citation28 Alternatively, to rescue the biosynthetic processing of ∆F508-CFTR the corrector CF-106951 [1-(benzo[d][1,3]dioxol-5-yl)-N-(5-((S)-(2-chlorophenyl)((R)-3-hydroxypyrrolidin-1-yl)methyl)thiazol-2-yl]cyclopropanecarboxamide; 6 µM) was added daily in fresh medium and cells were cultured at 37°C for 48 h before experiments (Cystic Fibrosis Foundation Therapeutic Inc.; http://www.cftrfolding.org)
Antibodies and reagents
The following antibodies were used: mouse anti-human CFTR (clone 596; Cystic Fibrosis Foundation Therapeutics, Inc.), anti-ezrin (BD Biosciences, San Jose, CA), anti-actin (mouse monoclonal or rabbit polyclonal; Sigma-Aldrich, St.Louis, MO), rabbit anti-c-Cbl (C-15; Santa Cruz Biotechnology, Santa Cruz, CA), rabbit anti-Epidermal Growth Factor Receptor (EGFR) D38B1 (Cell Signaling Technology, Davners, MA) and mouse anti-human Transferrin Receptor (TfR; CD71; eBioscience, Inc., SanDiego, CA). Horseradish peroxidase-conjugated goat anti-mouse and goat anti-rabbit (BioRad Laboratories; Hercules, CA) secondary antibodies were used. All antibodies were used at the concentrations recommended by the manufacturer or as indicated in the figure legends.
RNA mediated interference
Transfection of CFBE41o- cells with siRNA targeting human c-Cbl gene (si-c-Cbl; Hs_Cbl_7 siRNA) or the non-silencing siRNA control (AllStars, si-CTRL; Qiagen, Valencia, CA) was conducted using HiPerFect Transfection Reagent (Qiagen) according to the manufacturer’s instructions as described in our recent publication.Citation23 Briefly, CFBE41o- cells were plated on tissue culture plates and incubated with the optimized transfection mixture containing 50 nM of siRNA at 37°C. After 24 h, cells were trypsinized and plated on collagen-coated Transwell permeable supports and cultured for an additional 4 d to establish polarized monolayers (total 5 d in culture). Silencing the target genes under these conditions resulted in the corresponding protein depletion by at least 60% ().
Biochemical determination of the complex (band C) and partially glycosyleted (band B) ∆F508-CFTR
The biosynthetic defect caused by the ∆F508 mutation results in processing arrest of the partially glycosylated, immature ∆F508-CFTR band B.Citation4 Temperature rescue (27°C for 48 h) was used to correct the biosynthetic defect and facilitate processing to the mature, fully glycosylated form of ∆F508-CFTR band C. Because CFTR band C is expressed at the cell surface, unlike band B, the abundance of band C in whole cell lysates (WCL) was used as a measure of the cell surface associated r∆F508-CFTR.Citation16 To inhibit protein translation cells were incubated with cycloheximide (Sigma-Aldrich; 20 µg/ml), at 37°C or 27°C and the disappearance of the fully and partially glycosylated form of ∆F508-CFTR was monitored over time.Citation23 CFBE41o- cells expressing ∆F508-CFTR were lysed in buffer containing 25 mM HEPES, pH 8.0, 1% Triton, 10% glycerol, and Complete Protease Inhibitor Cocktail (Roche Applied Science, Indianapolis, IN), as described previously.Citation17,Citation23 Proteins were visualized by western blotting with specific primary antibodies and horseradish peroxidase secondary antibodies using the Western Lightning™ Plus-ECL detection system (Perkin Elmer Inc.; Waltham, MA) followed by chemiluminesence. Quantification of the immunoreactive bands was performed by densitometry using exposures within the linear dynamic range of the film.
Ussing chamber measurements
Ussing chamber measurements were performed as previously described.Citation23 Monolayers of airway cells grown on Snapwell permeable supports were mounted in an Ussing-type chamber (Physiologic Instruments; San Diego, CA) and bathed in solutions (see below) maintained at 37°C and stirred by bubbling with 5% CO2 / 95% air. Short circuit current (Isc) was measured by voltage-clamping the transepithelial voltage across the monolayers to 0 mV with a voltage/current clamp (model VCC MC8, Physiologic Instruments) as previously described.Citation23 Data collection and analysis were done with Acquire and Analyze Data Acquisition System (Physiologic Instruments). CFBE41o- cells were bathed in solutions with an apical-to-basolateral Cl- gradient in the presence of amiloride (50 µM) in the apical bath solution to inhibit Na+ absorption. The apical bath solution contained (in mM): 115 Na-gluconate, 5 NaCl, 25 NaHCO3, 3.3 KH2PO4, 0.8 K2HPO4, 1.2 MgCl2, 1.2 CaCl2, 10 mannitol (pH 7.4). The basolateral bath solution contained (in mM): 120 NaCl, 25 NaHCO3, 3.3 KH2PO4, 0.8 K2HPO4, 1.2 MgCl2, 1.2 CaCl2, 10.0 glucose (pH 7.4). A low Cl-, high-Na+, high-gluconate, apical bath solution was used to prevent cell swelling due to the increased apical Cl- permeability under these conditions, as previously described.Citation23 Isc was stimulated with 20 µM forskolin and 50 µM IBMX added to the apical and basolateral bath solution. Thiazolidinone CFTRinh-172 (5 µM; Tocris Bioscience; Ellisville, MO) was added to the apical bath solution to inhibit CFTR-mediated Isc. Data are expressed as Forskolin/IBMX stimulated Isc, calculated by subtracting the baseline Isc from the peak stimulated Isc.
Data analysis and statistics
Statistical analysis of the data was performed using GraphPad Prism version 4.0 for Macintosh (GraphPad Software Inc., San Diego, CA). The means were compared by a two-tailed t-test. A P value < 0.05 was considered significant. Data are expressed as mean ± SE.
Acknowledgments
We would like to thank Dr. John Wakefield from Tranzyme, Inc. (Birmingham, AL) who generated the stable CFBE41o- cell lines, and Dr. J.P. Clancy from Cincinnati Children’s Hospital Medical Center Cincinnati, OH for providing the cells. This study was supported by the National Institutes of Health Grants R01HL090767, R01HL090767–02S1 (to A.S.U.). This work was also supported by the Pennsylvania Department of Health, Health Research Formula Fund to the Children's Hospital of Pittsburgh of the UPMC Health System (to A.S.U.).
Disclosure of Potential Conflicts of Interest
No potential conflicts of interest were disclosed.
References
- Riordan JR, Rommens JM, Kerem B, Alon N, Rozmahel R, Grzelczak Z, et al. Identification of the cystic fibrosis gene: cloning and characterization of complementary DNA. Science 1989; 245:1066 - 73; http://dx.doi.org/10.1126/science.2475911; PMID: 2475911
- Rommens JM, Iannuzzi MC, Kerem B, Drumm ML, Melmer G, Dean M, et al. Identification of the cystic fibrosis gene: chromosome walking and jumping. Science 1989; 245:1059 - 65; http://dx.doi.org/10.1126/science.2772657; PMID: 2772657
- Howard M, Jiang X, Stolz DB, Hill WG, Johnson JA, Watkins SC, et al. Forskolin-induced apical membrane insertion of virally expressed, epitope-tagged CFTR in polarized MDCK cells. Am J Physiol Cell Physiol 2000; 279:C375 - 82; PMID: 10913004
- Riordan JR. CFTR function and prospects for therapy. Annu Rev Biochem 2008; 77:701 - 26; http://dx.doi.org/10.1146/annurev.biochem.75.103004.142532; PMID: 18304008
- Boucher RC. New concepts of the pathogenesis of cystic fibrosis lung disease. Eur Respir J 2004; 23:146 - 58; http://dx.doi.org/10.1183/09031936.03.00057003; PMID: 14738247
- Tarran R, Button B, Boucher RC. Regulation of normal and cystic fibrosis airway surface liquid volume by phasic shear stress. Annu Rev Physiol 2006; 68:543 - 61; http://dx.doi.org/10.1146/annurev.physiol.68.072304.112754; PMID: 16460283
- Bertrand CA, Frizzell RA. The role of regulated CFTR trafficking in epithelial secretion. Am J Physiol Cell Physiol 2003; 285:C1 - 18; PMID: 12777252
- Guggino WB, Stanton BA. New insights into cystic fibrosis: molecular switches that regulate CFTR. Nat Rev Mol Cell Biol 2006; 7:426 - 36; http://dx.doi.org/10.1038/nrm1949; PMID: 16723978
- Lukacs GL, Segal G, Kartner N, Grinstein S, Zhang F. Constitutive internalization of cystic fibrosis transmembrane conductance regulator occurs via clathrin-dependent endocytosis and is regulated by protein phosphorylation. Biochem J 1997; 328:353 - 61; PMID: 9371688
- Prince LS, Peter K, Hatton SR, Zaliauskiene L, Cotlin LF, Clancy JP, et al. Efficient endocytosis of the cystic fibrosis transmembrane conductance regulator requires a tyrosine-based signal. J Biol Chem 1999; 274:3602 - 9; http://dx.doi.org/10.1074/jbc.274.6.3602; PMID: 9920908
- Van Goor F, Hadida S, Grootenhuis PD, Burton B, Stack JH, Straley KS, et al. Correction of the F508del-CFTR protein processing defect in vitro by the investigational drug VX-809. Proc Natl Acad Sci U S A 2011; 108:18843 - 8; http://dx.doi.org/10.1073/pnas.1105787108; PMID: 21976485
- Rogan MP, Stoltz DA, Hornick DB. Cystic fibrosis transmembrane conductance regulator intracellular processing, trafficking, and opportunities for mutation-specific treatment. Chest 2011; 139:1480 - 90; http://dx.doi.org/10.1378/chest.10-2077; PMID: 21652558
- Lubamba B, Dhooghe B, Noel S, Leal T. Cystic fibrosis: insight into CFTR pathophysiology and pharmacotherapy. Clin Biochem 2012; 45:1132 - 44; http://dx.doi.org/10.1016/j.clinbiochem.2012.05.034; PMID: 22698459
- Clancy JP, Rowe SM, Accurso FJ, Aitken ML, Amin RS, Ashlock MA, et al. Results of a phase IIa study of VX-809, an investigational CFTR corrector compound, in subjects with cystic fibrosis homozygous for the F508del-CFTR mutation. Thorax 2012; 67:12 - 8; http://dx.doi.org/10.1136/thoraxjnl-2011-200393; PMID: 21825083
- Sharma M, Pampinella F, Nemes C, Benharouga M, So J, Du K, et al. Misfolding diverts CFTR from recycling to degradation: quality control at early endosomes. J Cell Biol 2004; 164:923 - 33; http://dx.doi.org/10.1083/jcb.200312018; PMID: 15007060
- Swiatecka-Urban A, Brown A, Moreau-Marquis S, Renuka J, Coutermarsh B, Barnaby R, et al. The short apical membrane half-life of rescued {Delta}F508-cystic fibrosis transmembrane conductance regulator (CFTR) results from accelerated endocytosis of {Delta}F508-CFTR in polarized human airway epithelial cells. J Biol Chem 2005; 280:36762 - 72; http://dx.doi.org/10.1074/jbc.M508944200; PMID: 16131493
- Cihil KM, Ellinger P, Fellows A, Stolz DB, Madden DR, Swiatecka-Urban A. Disabled-2 protein facilitates assembly polypeptide-2-independent recruitment of cystic fibrosis transmembrane conductance regulator to endocytic vesicles in polarized human airway epithelial cells. J Biol Chem 2012; 287:15087 - 99; http://dx.doi.org/10.1074/jbc.M112.341875; PMID: 22399289
- Madden DR, Swiatecka-Urban A. Tissue-specific control of CFTR endocytosis by Dab2: Cargo recruitment as a therapeutic target. Commun Integr Biol 2012; 5:473 - 6; Error! Hyperlink reference not valid http://dx.doi.org/10.4161/cib.21375; PMID: 23181163
- Cheng J, Moyer BD, Milewski M, Loffing J, Ikeda M, Mickle JE, et al. A Golgi-associated PDZ domain protein modulates cystic fibrosis transmembrane regulator plasma membrane expression. J Biol Chem 2002; 277:3520 - 9; http://dx.doi.org/10.1074/jbc.M110177200; PMID: 11707463
- Wolde M, Fellows A, Cheng J, Kivenson A, Coutermarsh B, Talebian L, et al. Targeting CAL as a negative regulator of DeltaF508-CFTR cell-surface expression: an RNA interference and structure-based mutagenetic approach. J Biol Chem 2007; 282:8099 - 109; http://dx.doi.org/10.1074/jbc.M611049200; PMID: 17158866
- Cushing PR, Vouilleme L, Pellegrini M, Boisguerin P, Madden DR. A stabilizing influence: CAL PDZ inhibition extends the half-life of ΔF508-CFTR. Angew Chem Int Ed Engl 2010; 49:9907 - 11; http://dx.doi.org/10.1002/anie.201005585; PMID: 21105033
- Roberts KE, Cushing PR, Boisguerin P, Madden DR, Donald BR. Computational design of a PDZ domain peptide inhibitor that rescues CFTR activity. PLoS Comput Biol 2012; 8:e1002477; http://dx.doi.org/10.1371/journal.pcbi.1002477; PMID: 22532795
- Ye S, Cihil K, Stolz DB, Pilewski JM, Stanton BA, Swiatecka-Urban A. c-Cbl facilitates endocytosis and lysosomal degradation of cystic fibrosis transmembrane conductance regulator in human airway epithelial cells. J Biol Chem 2010; 285:27008 - 18; http://dx.doi.org/10.1074/jbc.M110.139881; PMID: 20525683
- Schmidt MH, Dikic I. The Cbl interactome and its functions. Nat Rev Mol Cell Biol 2005; 6:907 - 18; http://dx.doi.org/10.1038/nrm1762; PMID: 16227975
- Molinski S, Eckford PD, Pasyk S, Ahmadi S, Chin S, Bear CE. Functional rescue of F508del-CFTR using small molecule correctors. Front Pharmacol 2012; 3:160; http://dx.doi.org/10.3389/fphar.2012.00160; PMID: 23055971
- Vertex Pharmaceuticals. Final data from phase 2 combination study of VX-809 and KALYDECO™ (ivacaftor) showed statistically significant improvements in lung function in people with cystic fibrosis who have two copies of the F508del mutation. 2012; http://investors.vrtx.com/releasedetail.cfm?releaseid=687394.
- Hentchel-Franks K, Lozano D, Eubanks-Tarn V, Cobb B, Fan L, Oster R, et al. Activation of airway cl- secretion in human subjects by adenosine. Am J Respir Cell Mol Biol 2004; 31:140 - 6; http://dx.doi.org/10.1165/rcmb.2004-0012OC; PMID: 15039139
- Denning GM, Anderson MP, Amara JF, Marshall J, Smith AE, Welsh MJ. Processing of mutant cystic fibrosis transmembrane conductance regulator is temperature-sensitive. Nature 1992; 358:761 - 4; http://dx.doi.org/10.1038/358761a0; PMID: 1380673