Abstract
For centuries, scientists and physicians have been captivated by the consistent left-right (LR) asymmetry of the heart, viscera, and brain. A recent study implicated tubulin proteins in establishing laterality in several experimental models, including asymmetric chemosensory receptor expression in C. elegans neurons, polarization of HL-60 human neutrophil-like cells in culture, and asymmetric organ placement in Xenopus. The same mutations that randomized asymmetry in these diverse systems also affect chirality in Arabidopsis, revealing a remarkable conservation of symmetry-breaking mechanisms among kingdoms. In Xenopus, tubulin mutants only affected LR patterning very early, suggesting that this axis is established shortly after fertilization. This addendum summarizes and extends the knowledge of the cytoskeleton’s role in the patterning of the LR axis. Results from many species suggest a conserved role for the cytoskeleton as the initiator of asymmetry, and indicate that symmetry is first broken during early embryogenesis by an intracellular process.
Introduction
With a few notable exceptions, vertebrates (and many invertebrates) have bilaterally symmetric external bodyplans. Yet these same animals exhibit consistent asymmetries in the position or anatomy of internal organs such as the heart, viscera, and brain. Asymmetry of the left-right (LR) axis is a fascinating area of research, and the existence of this asymmetry raises many fundamental questions for biologists: What are the implications of asymmetry for the function and physiology of the internal organs? Why are all normal individuals not only asymmetric, but all asymmetric in the same direction (rather than a 50:50 mix)? At what developmental stage is asymmetry initiated? How conserved are the mechanisms that produce asymmetry across species? And how is consistent asymmetry generated in a world where no macroscopic feature of chemistry or physics distinguishes left from right?
Asymmetry extends beyond the internal bodyplan and is therefore relevant to other fields of science.Citation1 A number of syndromes and drug effects exhibit sidedness of presentation in human patients.Citation2-Citation6 In the field of oncology, LR biases have recently been uncovered for health endpoints including the siteCitation7 and the prognosis and likelihood of metastasisCitation8-Citation10 for several types of cancer. In the fields of behavioral science and neurology, strong LR biases have been noted in behavioral features such as hand dominance,Citation11 and altered neurological asymmetries have been linked to diseases and disabilities such as dyslexia and schizophrenia.Citation12-Citation14 There also appear to be biological associations between handedness and other disease outcomes including susceptibility to intestinal parasites,Citation15 asthma, and autoimmune diseases,Citation16,Citation17 suggesting brain-body links that should continue to be explored in the context of laterality. Finally, LR asymmetry is an important topic for embryologists, neonatologists, and pediatricians. Although defects in LR asymmetry are not terribly common compared with other birth defects (affecting 1:6000 live births), there are severe medical consequences for individuals with some of these conditions including heterotaxia (the lack of concordance between internal organs, allowing each organ to individually “decide” on its placement on the left or right side of the body), single organ inversions such as dextrocardia (the reversal in position and morphology of the heart), and isomerisms (symmetry of the LR axis, leading to either duplication or complete loss of single organs such as the spleen). Patients with complete reversal of asymmetry (situs inversus) have fewer health consequences than these other conditions because conditions such as heterotaxia and isomerisms often involve inappropriate connections between the heart, lungs, and other visceral organs.Citation18-Citation21
It is widely acknowledged that the generation of asymmetry in embryos requires 3 separate stepsCitation22: 1) the breaking of symmetry, allowing the LR axis to be consistently oriented relative to the dorsal-ventral and anterior-posterior axes, 2) the translation of these initial asymmetries to produce asymmetric gene expression on the left and right sides of the body, and 3) the translation of asymmetric gene expression into asymmetric position and morphology of the internal organs (). In contrast to these last 2 steps, which have been well characterized in a number of model species,Citation23-Citation27 there remains significant debate over the mechanisms that operate in the first step, i.e., the origin of symmetry breaking.Citation28-Citation31
Figure 1. Establishing the LR axis is a 3-step process. In the first step, symmetry is broken; after the anterior-posterior and dorsal-ventral axes are established in the radially symmetrical egg, the LR axis can be defined. In the second step, the early asymmetries are translated to asymmetric expression of genes such as the Nodal-Lefty-Pitx2 cassette. Finally, this asymmetric gene expression is translated into the asymmetric positioning and shape of the internal organs.
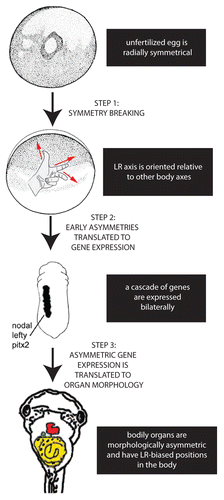
Models for LR Asymmetry
One model for symmetry breaking proposes that ciliary motion within a small pocket of tissue in the neurulating embryo produces LR asymmetries. In this ciliary model of LR asymmetry, the movement of cilia produces a directional fluid flow within this fluid-filled space, termed the node (mouse), the gastrocoel roof plate (GRP, Xenopus), or Kupffer’s Vesicle (KV, zebrafish). Because of their consistent structural chirality and tilt, cilia can produce a right-to-left current that asymmetrically distributes particles and can trigger mechanosensory cilia on one side of the embryo.Citation29,Citation32-Citation37 The ciliary model is appealing because it derives laterality from a biochemical structure, and there is a strong correlation between ciliopathies and LR patterning defects in human patients.Citation29,Citation37,Citation38 However, a number of inconsistencies have arisen between this model and experimental data, including the identification of numerous species (such as pigCitation39 and chickCitation40,Citation41) that orient their LR axis without cilia or prior to the appearance of motile cilia,Citation28,Citation39,Citation42 the existence of genetic mutants with defects in ciliary function but normal LR asymmetry,Citation41,Citation43-Citation46 and the appearance of consistent asymmetries long before ciliary flow,Citation47-Citation60 among others.Citation23,Citation61-Citation64
The existence of consistently asymmetric animals without cilia at the node commits the cilia model to requiring a highly divergent origin of asymmetry among speciesCitation23,Citation61 – an evolutionarily unlikely scenario. For all of the above reasons, alternative models have been proposed that focus on highly conserved intracellular/cytoplasmic pathways. The general class of intracellular models postulates that asymmetry originates during very early developmental stages due to the chiral nature of the cytoskeleton.Citation63,Citation65,Citation66 In contrast to the ciliary model’s focus on relatively late extracellular fluid flows, the intracellular model postulates that asymmetry is generated from within early embryonic cells where an oriented chiral cytoskeleton is amplified to tissue-level asymmetries. One hypothesis is that a chiral cytoskeleton directs the distribution of chromatids during the first cleavage stage,Citation67,Citation68 and that these chromatids are differentially imprinted, allowing for the distinction of the left and right sides.Citation66,Citation68,Citation69 Another hypothesis postulates that the chiral cytoskeleton actively directs the asymmetric distribution of proteins including K+ channels and H+ pumps by intracellular motors,Citation54,Citation70 which produce consistent biases in the pH and transmembrane voltage on the left and right sides of the embryo.Citation50,Citation71 These biases establish bioelectrical gradients that drive the asymmetric distribution of small charged molecules such as serotonin through gap junctions from left to right in the early embryoCitation49,Citation72,Citation73 (). Serotonin, which accumulates on the right side of the embryo, can epigenetically repress the expression of asymmetric genes such as Xnr-1, the Xenopus homolog of the highly conserved left-side marker Nodal.Citation74
Figure 2. The role of ion flux in the intracellular model of LR asymmetry. (A) A chiral cytoskeleton asymmetrically distributes mRNAs that encode a number of ion transporters including H+ pumps and K+ channels. (B) The biased expression of these ion transporters in the ventral right blastomere establishes a LR difference in resting potential between the L and R sides (due to the flow of positive ions from the ventral right side of the embryo, the blastomeres in this part of the embryo become relatively negative). (C) With the exception of the ventral-most blastomeres, all of the blastomeres of the embryo are connected via a series of open gap junctions. The relatively negative nature of the ventral right blastomere establishes a net flow of positively charged signaling molecules such as serotonin to this portion of the embryo.Citation192-Citation195 While this scheme illustrating the amplification of cell chirality into multi-cellular gradients has best been worked out in Xenopus, it remains to be tested in other model species to determine how similar physiological pathways could map onto phyla with different bodyplans.
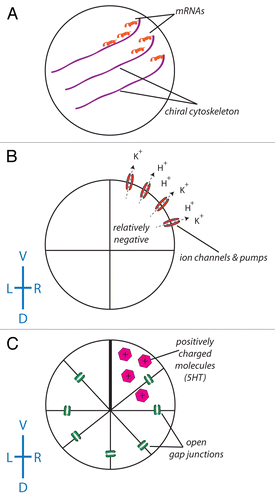
Experimental evidence supports both of these intracellular models, and they are mutually compatible. Furthermore, we have recently proposed 2 novel possibilities.Citation62 One is a unified model of asymmetry, whereby LR asymmetry is initiated during the early cleavage stages of development via inherent chirality of the cytoskeleton, and asymmetric information is amplified during subsequent developmental stages via the asymmetric intracellular distribution of serotonin and the asymmetric motion of cilia to distribute extracellular morphogens and ions. According to this unified model, LR asymmetry is best established when all mechanisms are working properly, but the LR axis can still be oriented in species lacking one or more parts of these pathways (for example, in species that lack cilia). The other recently proposed model is the stochastic model of asymmetry, which proposes that embryos of one species can stochastically choose among one of several pathways to pattern their LR axis: within any clutch of embryos, some use one pathway to initiate asymmetry, and others “choose” another pathway. This maximizes the chance that at least some individuals in a population will be unaffected by environmental factors that affect one of the LR pathways, and explains why most perturbations of a LR pathway component affect some but not all embryos in any experimental population.
Importantly, all of these models, including the ciliary model, point to aspects of the cytoskeleton as a central feature of asymmetry. This addendum will focus on recent studies examining the role of the cytoskeleton in the establishment of LR asymmetry, emphasizing conservation of mechanisms across species and even kingdoms. There is now strong evidence that asymmetry is an ancient, highly conserved feature of single cells and higher organisms.
The cytoskeleton: A central actor in models of LR asymmetry
The centrosome is comprised of 2 centrioles and a matrix of proteins including γ-tubulin that forms the microtubule organizing center (MTOC).Citation75 The centrosome has functional and structural asymmetries that are considered essential to maintain cell polarity and asymmetry,Citation76-Citation78 and indeed for linking the microtubule cytoskeleton to large-scale axes of the organism.Citation76,Citation79 For example, the pair of centrioles that comprise the centrosome are asymmetric in size and only the maternal centriole can become a basal body and nucleate the formation of cilia.Citation80 Centriole asymmetry is also important for the biased segregation of mRNAs and transcription factors.Citation81,Citation82 The mother centriole is responsible for cell geometry and relative positioning of other organelles within the cell.Citation83-Citation85 The basal body itself has inherent asymmetries: when some protists undergo the G1 to S transition and extend a new cilium, the newly formed basal body completes a highly conserved leftward rotation around the old cilium.Citation86
All of the models of LR asymmetry described above are intimately linked by their reliance on the cytoskeleton to initiate LR asymmetry (). In the 2 variants of the intracellular model of LR asymmetry, the implicated cytoskeleton is solely localized within the cell, without any external features (i.e., projections such as cilia). In the model implicating ion flux, the chirality of the microtubule and actin filaments controls the directionality of cytoplasmic tracks for motor proteins such as kinesin and dynein, thus regulating asymmetric movement of intracellular cargo. Specifically, it postulates that the MTOC sets up a cytoskeleton that is oriented with respect to the other 2 axes of the prospective embryo, and is subtly biased to the right, to allow some maternal proteins to be preferentially enriched on the right side during early cleavage stages.Citation87 For the mechanism involving asymmetric chromatid segregation, the cytoskeletal component is also intracellular, involving the machinery that controls chromatid movements during cell divisions.Citation67,Citation68 In the ciliary model of LR asymmetry, the cytoskeleton is implicated both within the cilia itself and in the basal body, the anchor for the cilia in the cell.Citation88
Figure 3. Centrosomes are implicated in 3 major models of LR asymmetry. (A) The centrosome itself is asymmetric. The centrosome has 2 centrioles (shown as red barrels) that are asymmetric in size; these are always found at a right angle to each other. The two centrioles and a matrix of proteins including γ-tubulin (orange lines) together form the MTOC. (B) In the asymmetric chromatid segregation model, the MTOC controls chromatid movements during cell divisions. (C) In the ion flux model, the MTOC serves to establish the chiral cytoskeleton, which eventually leads to the asymmetric distribution of ion transporters. (D) In the cilia model, the basal body sits at the base of the cilia and, through the movements of the cilia, generates asymmetric fluid flow.
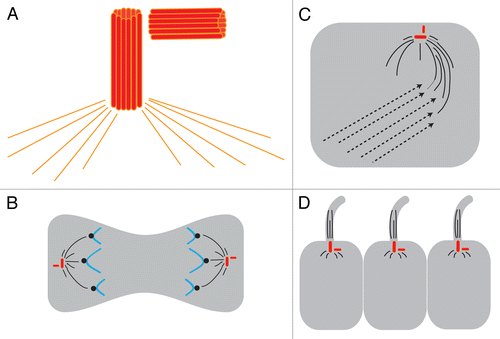
Other aspects of the cytoskeleton generate various kinds of polarity. For example, in cultured monolayers undergoing a scratch-wound assay, individual cells at the “wound” edge repolarize so that the centrosome, golgi apparatus, and lamellipodia are on the side of the cell facing the wound, whereas the nucleus, stress fibers, and mature focal adhesions form on the side furthest from the wound edge.Citation89 This re-organization is dependent on CDC42,Citation90,Citation91 a protein involved in apical-basal polarity that has been implicated in LR patterning in XenopusCitation92 and the asymmetric cell protrusions needed to establish the LR axis in C. elegans.Citation93 Together, these data suggest that there are highly conserved aspects of asymmetry that operate in single cells, invertebrates, and vertebrates, and the cytoskeleton has a central role in the major models of LR asymmetry. As will be discussed below, it appears that a number of the pathways involved in cell polarity decisions are intimately linked with LR asymmetry.Citation93,Citation94
From plants to frogs: A clear role for the cytoskeleton
The majority of plants display radial or bilateral symmetry in their flowers and leaves, yet some species demonstrate asymmetries that manifest in specific organs.Citation95 For example, asymmetry is often observed in the handedness of coiling as stems grow around other objects and can be seen in the twisting of axial organs.Citation96 The leaves, flowers, roots, and stems of Arabidopsis are normally organized in radially symmetrical patterns. However, fascinating molecular genetic studies have revealed a role for tubulins in maintaining this apparent symmetry (similar to the way in which retinoic acid signaling symmetrizes inherently-asymmetric somite patterningCitation97). α-tubulin is a protein that dimerizes with β-tubulin to make microtubules. Mutations in α-tubulin at the intradimer interface produce Arabidopsis plants with left-handed chiral roots and stems and clockwise-bending leaves and flowers.Citation98 Similarly, mutations in a core subunit of γ-tubulin-containing complexes also produce plants with these morphological asymmetries.Citation99 Collectively, these studies indicate that alterations in the architecture of microtubules regulate large-scale symmetry properties of this plant.
In contrast to the cilia model, which must postulate divergent origins of asymmetry in animals such as mouse, pig, chick, frog, and invertebrates, the intracellular model is compatible with an ancient, highly conserved role for the symmetry-breaking mechanism. To directly test this key distinction between the 2 paradigms, a recent studyCitation100 assessed the widest-possible conservation of asymmetry mechanisms. First, mRNA encoding tubulins bearing mutations homologous to those that induce asymmetry in Arabidopsis were introduced into Xenopus embryos. Expression of mRNA encoding mutant α-tubulin or a mutated form of the γ-tubulin associated protein tubgcp2 induced heterotaxia, even when targeted to cells that do not contribute to the ciliated organ (GRP), but only when these mRNAs were injected at the 1-cell stage. When injections occurred at the 2-cell stage or later, normal LR patterning occurred, even when mRNAs were targeted to the ciliated cells of the GRP. Injections with mutant tubgcp2 mRNA also disrupted asymmetry and directionality of microtubule-dependent motor protein transport—important aspects of the intracellular model of LR asymmetry. Finally, expression of either mutant tubgcp2 or mutant α-tubulin altered LR biases in the expression of cofilin-1a, a polarity protein that mediates actin-tubulin interactionsCitation101,Citation102 that was found to be enriched on the right half of the wild-type 4-cell Xenopus embryo. Indeed, an unbiased proteomic analysis of the 4-cell frog embryo revealed a number of maternal products that were differentially localized between the left and right sides; expression of mutant cytoskeletal components abolished or reversed LR biases in a number of such proteins, including ion transporters, other cytoskeletal components, and proteins involved in other cellular processes. Taken together, the data clearly indicate that in the frog embryo, consistent LR asymmetry already exists by the second cell division, and involves some aspect of tubulin-directed intracellular activity (such as transport of cargo).
Continuing the strategy of testing the same mechanism in highly diverse phyla with very different bodyplans, the same tubulin mutations were tested in the nematode model. In C. elegans, there are 2 AWC olfactory neurons that are morphologically symmetrical but display LR biases in expression of chemosensory receptorsCitation103; the mechanisms responsible for producing these asymmetries involve gap junctions and ion flows—2 components of LR patterning in several vertebrate species.Citation104,Citation105 Previous studies have demonstrated that these neural asymmetries can be disrupted by treating C. elegans embryos with pharmaceuticals that disrupt microtubule polymerization.Citation104 Expression of mutant α-tubulin prevented chemosensory receptor asymmetry (based on the expression of a reporter gene str-2p) in 58% of the embryos, a very significant change from wild type C. elegans where asymmetric expression of str-2p was observed in all worms.Citation106
Importantly, consistent asymmetry does not require a multicellular structure (such as a node or embryonic blastoderm) at all: a number of single cells exhibit consistent chirality in their movements and behaviors.Citation94,Citation107-Citation113 To test the same cytoskeletal component in human cells and probe the relevance of this component to cellular asymmetry in the absence of any coordinated fluid flows, the effects of mutant α-tubulin were investigated in the human neutrophil-like cell line HL-60.Citation106 In wild-type cultured HL-60 cells, one “dorso-ventral” axis is defined by the interaction of the cells with the culture plate (vs. interaction of the cell’s top surface with the culture media); a second axis (“anterior-posterior,” perpendicular to the first) can be identified by drawing a line from the nucleus to the centrosome, thus a pseudo-LR axis can be defined based on these other 2 axes. Wild-type HL-60 cells typically extend a pseudopod to the left of the nucleus-centrosome axis following exposure to fMLP, a chemoattractant.Citation94 This leftward bias can be abolished by pharmaceuticals that disrupt microtubule polymerization. Transfected HL-60 cells expressing mutant α-tubulin no longer displayed leftward biases in response to fMLP—the mutant proteins abolished this form of LR asymmetry in cultured human cellsCitation106 as they did in vivo.
The role for tubulins in LR patterning in Arabidopsis, Xenopus, C. elegans, and cultured human neutrophils reveals a remarkable degree of conservation of this molecular mechanism. Collectively, these studies implicate the microtubule cytoskeleton in a number of types of asymmetries operating across multiple levels of biological organization, and suggest different amplification mechanisms within the very different bodyplans.
How early are the earliest steps of asymmetry?
Mutant tubulin mRNAs disrupted LR patterning in Xenopus tadpoles, but only if present right after fertilizationCitation106 – injection at the 2-cell stage was already too late to affect asymmetry. Because mRNAs begin to be translated within a short time of their introduction to the Xenopus embryo (i.e., Lobikin et al. demonstrate strong expression at the 2-cell stage when injected prior to the first cleavageCitation106), the results constrain the role of tubulin to events that occur within the first hour or 2 of fertilization, at the 1- or 2-cell stage. This timing is consistent with both the model of asymmetrically segregating maternal ion translocator proteinsCitation23,Citation114 and the chromatid segregation model.Citation66,Citation67
Similar results have been obtained for a limited number of other reagents, mostly limited to manipulations that target the cytoskeleton (although ion flows exhibit similar timing requirementsCitation115). In one example, treatment of embryos with 2,3-butanedione monoxime (BDM), a myosin inhibitor, randomizes the LR axis, but only when administered prior to the first cell cleavage.Citation47 In fact, studies focused on identifying the critical period for this compound reveal a very short period during the 1-cell stage where embryos are most sensitive, whereas BDM treatments even during the late 1-cell stage have no effect on LR patterning. In another example, low frequency vibrations were shown to disrupt LR patterning in Xenopus and zebrafish embryosCitation116,Citation117; mechanistic studies in Xenopus revealed that these vibrations affect the early cytoskeleton and the integrity of tight junctions in the early cleavage stage embryo.Citation116
Interestingly, the molecular motors that control cytoskeletal movement have been modeled as ratchets.Citation118 The stepwise motion of the motor along the polymer is powered by the thermal energy (Brownian motion) of the fibril. The motor uses ATP hydrolysis to resist the movement in a particular direction; myosin V is a left-handed spiral motor moving toward the plus end of actin,Citation119 while myosin II is right-handed spiral motor.Citation120 It may thus be speculated that molecular vibrations affect the structure of the cytoskeleton as a component of symmetry breaking,Citation121 and could impact the delivery of molecular cargo by increasing the thermal energy of the cytoskeletal components. Consistent with an early role for endogenous molecular motion in the earliest steps of laterality establishment, sinusoidal vibrations applied to frog embryos that started at the 1-cell stage were able to disrupt LR patterning; they were significantly more effective than vibrations that commenced at the 2-cell stage (31% vs. 19%) and more than twice as effective as vibrations that began at after 6 rounds of cell division (12%).Citation116
A final example used classical developmental biology techniques to probe the timing of initiation of LR asymmetry.Citation122 Xenopus embryos were UV-irradiated at the 1-cell stage to prevent cortical rotation,Citation123 thus effectively removing all axial patterning information. When patterning information was restored at the 1-cell stage via mechanical tipping, a method that uses gravity to physically induce cortical rotation,Citation124 normal LR patterning resulted.Citation122 However, if axial patterning was induced slightly later (either via injection of lithium chloride at the 32-cell stage, which begins inducing dorsal determinants immediately, or via injection of XSiamois, a molecular component of Spemann’s organizer, which induces zygotic transcription at midblastula transition),Citation125,Citation126 LR patterning defects were observed.Citation122 This experiment confirmed that initiation of LR asymmetry occurs prior to the 32-cell stage.
These timing experiments need to be considered in the context of the numerous additional experiments that have revealed consistent biases in mRNA localization, protein expression, and physiological endpoints during early cleavage stages.Citation50,Citation55,Citation70,Citation71 Furthermore, many of these early cleavage stage asymmetries have been shown to be disrupted by treatment with reagents that target microtubules and/or actin filaments.Citation54 Collectively, these results suggest that the earliest steps in the LR asymmetry pathway are occurring very early—within the first cleavage stages, and likely prior to the first cell cleavage. It should be noted that while none of these have been tested in mammalian embryos yet, even mouse embryo cells are known to not be LR-equivalent by the 8-cell stage,Citation127 consistent with early models.
Starting with the cytoskeleton: a model for how the LR axis stays on track
In many species, the sperm contributes a centrosome to the resulting embryo at the time of fertilization as well as other essential components of the MTOC that attract proteins to this complex from the egg’s stores.Citation128,Citation129 Experiments involving the fertilization of an egg with multiple sperm (i.e., polyspermy) have revealed the consequences of having multiple centrosomes in an early embryo; these embryos typically develop multiple cleavage furrows, distribute their chromosomes unevenly between blastomeres, and fail to develop beyond the early cleavage stages.Citation76,Citation130 Additional studies have shown that the centrosome itself is sufficient to induce cleavage of an unfertilized egg, indicating that this structure plays a central role in the presence and localization of the first cleavage plane.Citation131 Centrosome location has been linked to symmetry breaking in a number of organisms, perhaps most clearly understood in C. elegansCitation132 and neuronal polarity.Citation133 Moreover, it is now known that asymmetry of the centriole plays a critical determining role in shaping the internal organization of cells through the attachment of microtubule rootles to specific sets of microtubule triplets on the centriole.Citation134,Citation135 Finally, data on the role of MTOC in guiding cell migration at wound edges reveal the central role of the cytoskeletal organizing center in orienting cellular axes with respect to large-scale external signals.Citation89-Citation91,Citation136
We propose that asymmetry is an ancient aspect of life and that the mechanism for its orientation is very deeply conserved. Our model postulates that the cytoskeleton is the origin of asymmetry in all of these species, and that the orientation of the MTOC with the 2 major embryonic axes at fertilization is sufficient to generate asymmetry in individual cells as well as the early embryo. The MTOC thus plays the role of the F-molecule that was theorized by Brown and WolpertCitation137: a structure that allows the LR axis to be oriented consistently with respect to the dorsal-ventral and anterior-posterior axes. Because the centrosome is asymmetric (), the MTOC itself has inherent asymmetries that can be amplified to cell-level asymmetries.Citation79,Citation138 It also is known to serve as an intracellular anchor, allowing for the assembly of chiral cytoskeletal tracks that can be used for directed and biased intracellular transport,Citation61,Citation65,Citation139,Citation140 and has been linked to laterality disturbances in nematodes,Citation141 zebrafish,Citation142 and humans.Citation143 We postulate that the orientation of this chiral cytoskeleton relative to the other axes can be established based on the sperm entry point.Citation139,Citation144 The unfertilized egg has radial symmetry, but like other single cells,Citation94,Citation109,Citation145 it has a chiral actin cytoskeletonCitation47; only after fertilization is the radial symmetry broken, because the point of sperm entry offers a distinct point on the circumference at which the counterclockwise direction of the actin uniquely orients a linear LR axis (). Such an intracellular LR axis is then used to redistribute maternal components to the right side, as has been shown to occur by the 4-cell stage.Citation70,Citation100 The earliest events establishing concordant linkage between the 3 major axes thus involve not only tubulin, as shown by Lobikin et al. and previous work implicating microtubules in asymmetry of a range of model species, but also actin; indeed, a number of actin-regulating proteins such as inversin and myosin are functionally implicated in laterality.Citation113,Citation146-Citation149 Consistent with this, the Lobikin 2012 study showed asymmetric localization of cofilin, a protein that mediates interaction between actin and tubulin and regulates cell polarization.Citation101,Citation102
Figure 4. The centrosome could be the F-molecule orienting the 3 axes at fertilization. (A) The unfertilized egg, seen from the animal pole, is radially symmetric about the animal-vegetal axis but contains a chiral actin cytoskeleton.Citation47 (B) At fertilization, the sperm entry point produces the first “point” around the circumference that is different from any other. The direction of the actin cytoskeleton at this point establishes a linear LR axis—a convergence of the 3 axes that could enable the MTOC to be uniquely oriented and thus establish directional asymmetries in the microtubule cytoskeleton that would underlie asymmetric transport of intracellular components.Citation70
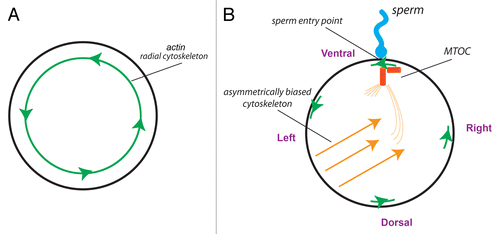
With the MTOC and the cytoskeleton establishing LR asymmetry shortly after fertilization, a number of additional steps are required to amplify and enhance these very early asymmetries. These include the asymmetric localization of ion channels and transporters, and the subsequent asymmetric distribution of intracellular signaling molecules including serotonin.Citation48-Citation50,Citation70-Citation74,Citation150-Citation154 Later-functioning mechanisms such as asymmetric cell migration,Citation39 asymmetric deposition of extracellular matrix components,Citation51,Citation52,Citation57,Citation155 and asymmetric fluid flowCitation37 may also serve as mechanisms to amplify the earliest asymmetries. Finally, a large body of knowledge about planar cell polarity indicates that small asymmetries can be amplified over whole tissues via a conserved set of intracellular proteins,Citation87 and global PCP orientation is now known to be set by microtubulesCitation156; recent evidence suggests that planar cell polarity proteins can alter the biased position of cilia at the node,Citation157 but can also affect LR patterning via cilia-independent mechanisms.Citation92,Citation158
Indeed, the picture painted of such early LR asymmetry mechanisms looks strikingly isomorphic to the current understanding of other examples of axial patterning throughout the animal kingdom. For example: “Single-cell patterning begins with an asymmetric cue that orients the axis of polarity. Despite great diversity in the types of cues, common mechanisms appear to mediate the polarizing response. Rho-family GTPases initially process and reinforce polarity cues by remodeling cortical actin, and these local asymmetries are subsequently propagated to the microtubules, membrane and secretory pathway to generate the final pattern”Citation159. Consistent with this, recent data implicated Rab GTPases in early asymmetry in Xenopus.Citation160 Interestingly, elegant molecular biomechanics work now shows that single cell chirality can drive the asymmetry of individual organs, such as the fruit fly hindgut,Citation161 as well as that of the whole bodyplan.
The study discussed in detail above implicates α-tubulin in a number of highly diverse organisms, and previous studies have implicated aspects of the intracellular cytoskeleton in LR patterning of ciliates,Citation162 Arabidopsis,Citation96 C. elegans,Citation163 Drosophila,Citation149 snails,Citation164 and Xenopus.Citation47,Citation54 Experimental evidence indicates that a number of downstream mechanisms may also be conserved between some plants, invertebrates, and vertebrates (), but additional experiments using a variety of model species are needed to dissect these factors in a greater number of species. Below, we outline some strategies for the study of LR patterning in a uniquely tractable model system – the Xenopus laevis embryo, which facilitates experiments both on the earliest steps of asymmetry and on later components such as planar polarity and ciliary flows.
Table 1. Cytoskeletal proteins have a conserved role in LR patterning
Recommendations for design of studies of LR asymmetry
As discussed elsewhere,Citation62 a few key strategies must be kept in mind when probing functions of new pathways in asymmetry, as many studies do not assess whether LR-relevant targets may have early roles (i.e., prior to stages where cilia are present). Moreover, most experimental designs used to provide data on putative LR functions of proteins at the nodal cilia in fact cannot rule out other intracellular roles.
Strategy 1: Comparison of mRNA injections during the early 1-cell stage with injections at the 2-cell, 4-cell, or later stages. A number of studies have shown that molecular constructs are effective at disrupting the LR axis only when they are introduced during the earliest stages of development, but are less effective – or ineffective – at later stages, even when targeted to cells that contribute to flow at the node.Citation55,Citation106,Citation140,Citation150 Similarly, experiments can compare the effects of injections of mRNA (translated within a short period of injection) with the effects of injections of DNA (which will not be translated until after midblastula transition - several hours after fertilization, when thousands of cells are present).Citation71 In addition to these early vs. late injections, additional tools are available for use in other developmental models including the use of inducible promoters in mice (which could be used to drive expression of key proteins at cleavage stages vs at primitive streak stages), and drug exposures beginning at various time-points in Xenopus and zebrafish. Collectively, these tools can distinguish between proteins with early and late roles.
Strategy 2: Targeting mRNAs to specific regions of the embryo. A good fate map has been produced for Xenopus,Citation165,Citation166 and subsequent experiments have revealed that only dorsal blastomeres, and more specifically the regions corresponding to the dorsal marginal zone, contribute to the GRP.Citation167,Citation168 Further analyses indicate that only the cilia on the left side of the midline are required for flow at the GRPCitation169; thus, only injections targeted to the left dorsal blastomere should influence LR patterning via alterations in ciliary flow. Conversely, injections in the right ventral blastomere should not influence ciliary flow, and thus any effects observed from injections at this site support cilia-independent effects. In zebrafish, injections can be targeted to the KV or to the entire embryoCitation170; while no available technique targets expression to all regions of the embryo with the exception of the KV, a comparison of the effects of ubiquitous expression to the effects of KV-restricted expression can be made. In mice, recent studiesCitation171 showed that node-only rescues of deleted genes do not fully restore normal asymmetry, but no studies yet examine what happens when some of the gene products are present only at early stages, or everywhere but the node.
Strategy 3: Early injections that localize mRNAs to small regions of the embryo. For mRNA constructs that are effective only when introduced at the 1-cell stage, or in species that do not have an obvious fate-map, and thus cannot be directly targeted in the left-dorsal or right-ventral blastomeres, it is helpful to inject these constructs in biased locations of the 1-cell embryo (i.e., some injections at the animal-most point, others at equatorial points) and include tagged lineage labels. This allows for a posteriori sorting, such that the localization of the construct to specific structures in the embryo can be related to the incidence of LR patterning defects.
For all of these experiments, we recommend using mRNAs that encode wild-type, mutant, constitutively active, and/or dominant negative forms of the protein of interest. Morpholinos should be avoided because they cannot affect maternal proteins—studies using morpholinos (and RNAi) are completely blind to any LR mechanisms depending on maternal proteins working prior to zygotic transcription, which are plentiful in species such as Xenopus.Citation48,Citation70,Citation160,Citation172,Citation173 Comparing injections of mRNA vs. linearized DNA constructs allows for a comparison of early vs. late mechanisms for the same molecular target. An important toolkit is also provided by pharmacological blockers and activators; it is easy to compare the effects of exposure beginning early in development with those of exposure that only starts after particular time-pointsCitation174,Citation175 (this strategy does not rely on wash-out of drug).
A number of previous publications have used these experimental approaches to identify proteins involved in early, cilia-independent mechanisms of establishing LR asymmetry (reviewed in 61). As a proof-of-principle, we have examined additional constructs using these experimental guidelines and have identified specific early roles for cytoskeletal proteins as well as roles for other proteins that are distinct from ciliary flow at the GRP (see Supplemental Materials for detailed information about materials and methods). The first construct we examined was a mutant form of tubulin binding cofactor B (TBCB), which regulates cell polarity and microtubule dynamics in DrosophilaCitation176; using the protocol outlined in Strategy 1 above, we found that expression of TBCB mutant mRNA at the 1-cell stage induced heterotaxia in 15% of frog embryos (). In contrast, injections at 2-cell or 4-cell had no effect on LR patterning, even when TBCB mutant mRNA was targeted to the GRP (via injections in the dorsal left blastomere).
Figure 5. Using experimental strategies to probe the early roles of cytoskeletal proteins on LR patterning. (A) Using strategy 1 (early vs. late injections), we found that expression of mutant TBCB mRNA altered LR patterning only when injected at 1-cell, indicative of a very early role for this tubulin binding protein. (B) Using strategy 2 (targeting to the GRP via the dorsal left [DL] blastomere vs. targeting to a region that does not contribute to flow at the GRP via the ventral right [VR] blastomere), we determined that expression of dominant negative CDC42 was effective at disrupting LR patterning, regardless of where it was targeted. These results indicate a GRP-independent role of CDC42 on orientation of the LR axis. (C) In contrast, expression of 5HT-R3A mRNA only disrupted LR patterning when targeted to the ventral right (VR) blastomere, signaling a GRP-independent role for 5HT. These results were previously reported in reference 154. (D) Using strategy 1, we found that early injections of mutant Kif3B mRNA effectively disrupted LR patterning. Using strategy 2, we observed that injections in the dorsal left (DL) blastomere were significantly more effective than injections in the ventral right (VR) blastomere, indicative of a role of Kif3B at the GRP. (E) To further probe the early role of Kif3B, we used strategy 3, injecting 1-cell embryos in a biased manner with the mutant Kif3B construct and a lineage label. When we post-sorted the tadpoles and scored them for heterotaxia, we observed a significant number with LR defects, regardless of whether the injections included the GRP (DL) or not (VR). These results suggest that Kif3B has an early role in LR patterning that is distinct from ciliary flow at the GRP, and likely has a second role in LR patterning at the GRP. For all panels, * indicates significant differences from controls (P < 0.01) and # identifies significant differences between the indicated groups.
![Figure 5. Using experimental strategies to probe the early roles of cytoskeletal proteins on LR patterning. (A) Using strategy 1 (early vs. late injections), we found that expression of mutant TBCB mRNA altered LR patterning only when injected at 1-cell, indicative of a very early role for this tubulin binding protein. (B) Using strategy 2 (targeting to the GRP via the dorsal left [DL] blastomere vs. targeting to a region that does not contribute to flow at the GRP via the ventral right [VR] blastomere), we determined that expression of dominant negative CDC42 was effective at disrupting LR patterning, regardless of where it was targeted. These results indicate a GRP-independent role of CDC42 on orientation of the LR axis. (C) In contrast, expression of 5HT-R3A mRNA only disrupted LR patterning when targeted to the ventral right (VR) blastomere, signaling a GRP-independent role for 5HT. These results were previously reported in reference 154. (D) Using strategy 1, we found that early injections of mutant Kif3B mRNA effectively disrupted LR patterning. Using strategy 2, we observed that injections in the dorsal left (DL) blastomere were significantly more effective than injections in the ventral right (VR) blastomere, indicative of a role of Kif3B at the GRP. (E) To further probe the early role of Kif3B, we used strategy 3, injecting 1-cell embryos in a biased manner with the mutant Kif3B construct and a lineage label. When we post-sorted the tadpoles and scored them for heterotaxia, we observed a significant number with LR defects, regardless of whether the injections included the GRP (DL) or not (VR). These results suggest that Kif3B has an early role in LR patterning that is distinct from ciliary flow at the GRP, and likely has a second role in LR patterning at the GRP. For all panels, * indicates significant differences from controls (P < 0.01) and # identifies significant differences between the indicated groups.](/cms/asset/e783c160-9043-4722-a179-74d8b282ceb2/kcib_a_10927155_f0005.gif)
We also examined a construct encoding a dominant negative of the Rho GTPase and cell polarity protein CDC42,Citation177 which we had previously observed induced LR patterning defects when injected at the 1-cell stage.Citation92 Using the protocol outlined in Strategy 2 above, we determined that expression of dominant negative CDC42 induced heterotaxia whether it was injected in blastomeres that contribute to ciliary flow at the GRP (dorsal left), or to blastomeres that have no such contribution (ventral right) (). These results suggest that CDC42 has a role in LR patterning, but that altered CDC42 expression anywhere in the embryo can induce heterotaxia and does not rely on interaction with cilia. These results are similar to the third example, expression of a mutant serotonin receptor (5HT-R3A). As we reported previously,Citation154 expression of this mutant receptor in either the ventral right or the dorsal left blastomere randomized LR patterning, but the effect was significantly greater when expressed on the ventral right ()—in cells that do not contribute to nodal flow.
In a final example, we examined the effects of a mutant kinesin, Kif3B, on LR patterning. Kinesins have previously been implicated in mouse LR asymmetry (interpreted as ciliary defectsCitation178) but also have intracellular transport roles.Citation54,Citation70 Injections at 1-cell were the most effective at randomizing the LR axis, with 24% of injected embryos developing heterotaxia (). At 4-cell, injections in the dorsal left blastomere also induced significant levels of heterotaxia, but injections in the ventral right blastomere were not effective. To determine whether Kif3B affects LR patterning solely because of its role in cilia, we utilized the protocol described in Strategy 3 above, injecting the mutant Kif3B mRNA construct during the early 1-cell stage in biased locations of the embryo. When we post-sorted the embryos based on the localization of a co-injected lineage label, we found that injections corresponding to the GRP and the dorsal right of the embryo were highly effective (47% heterotaxia; ). Surprisingly, we also observed significant heterotaxia in embryos where the construct was targeted far from the GRP (i.e., corresponding to the ventral right blastomere, 20% heterotaxia). These results suggest that there is a likely role for Kif3B in the GRP, but that there are other early roles for Kif3B that are distinct from ciliary flow.
Collectively, these examples illustrate experimental design that facilitates an unbiased study of determinants of the LR pathway, and provide tools to dissect out those that act independent of ciliary flow. Similar to what was shown previously for both α-tubulin and γ-tubulin-containing complexes,Citation106 alterations in TBCB at the 1-cell stage significantly affect LR patterning whereas expression of mutant TBCB in later stages has no effect on the LR axis. It is crucial that these strategies also be extended to reagents that regulate chromatid segregation, to begin to truly test that model in the context of known downstream components of LR patterning.
Future Prospects
A number of key open questions remain in the study of LR asymmetry. One major issue surrounds the identification of the best animal model to understand how the LR axis is established in humans, in order to study human laterality diseases in the optimal biological context. As we have noted previously, the mouse may not be a good model for understanding human LR pathologies.Citation61,Citation62 Compared with other amniotes like chick and pig, the mouse has a fundamentally different embryonic bodyplan and major differences in LR patterning stepsCitation39; diseases that are known to respect the midline in humans (i.e., CHILD syndrome, which produces LR-bilateral separation of pigmentation in human patients) have mosaic patterns in the mouse.Citation179-Citation181 Thus, while the mouse may not be the best animal model to understand the mechanisms responsible for laterality in humans, it remains to be shown which alternative model may be better. A promising possibility is afforded by the pig and rabbit, if techniques for manipulating much earlier stages than gastrulation can be developed.Citation39,Citation182,Citation183
The data from frog embryos suggest a scheme in which physiological signaling amplifies single-cell chirality into gene expression on a large scale. This is remarkably similar to what has been seen in C. elegansCitation93,Citation132,Citation184 and snails.Citation185 Thus, it is plausible that not only proteins such as α-tubulin are highly conserved, but also general schemes for leveraging body asymmetry from molecular chirality may be likewise conserved between vertebrates and invertebrates.
The cilia model of LR asymmetry is bolstered by the fact that human patients and animal mutants with ciliopathies often have LR patterning defects.Citation37,Citation186-Citation188 Yet, the interpretation of these studies’ data almost universally omits the fact that “ciliary” proteins also have intracellular functions. Indeed it is a prediction of the intracellular model that defects in MTOC or related cytoskeletal proteins will produce laterality defects and, as a side effect, a ciliary phenotype. Although these functions are difficult to dissociate in organisms in which non-node cells cannot easily be targeted with reagents, a few mutations have now been characterized that show that LR phenotypes and ciliary properties do not track together as well as is commonly thought.Citation41,Citation43-Citation46,Citation154
Moving forward, it is clear that these mutant proteins should be examined in a number of animal models including mouse, frog, fish, and chick, with specific attention to possible early roles as distinct from ciliary functions. Disruptions to these proteins that are limited to the cells that contribute to the ciliated node, as well as disruptions that are limited to all of the cells except those contributing to the node, are expected to bring to light the role of cytoskeletal proteins in LR patterning. This type of study design will help to determine whether these “ciliary” proteins are acting in the LR pathway solely due to their roles in the cilia, or whether they play a wider part in the intracellular cytoskeleton. The very recent finding of actin filament-containing filopodia that cross the blastocoel and deliver vesicles across relatively large distances in the developing embryoCitation189 will also have to be considered with respect to their possible contribution to the redistribution of molecular cargo.
It is also clear that more quantitative data are needed to better study the effects of molecular genetic reagents and manipulations on LR patterning. We have conducted a number of meta-analyses of the literature quantifying statistical relationships between penetrance of reagents on asymmetric gene expression and on asymmetric organ situs.Citation62,Citation154,Citation190 These analyses suggest gaps in the 3-step pathway from symmetry breaking to asymmetric organ placement (); there may be unidentified mechanisms acting as check-points throughout the LR pathway, allowing for some laterality errors to be corrected during embryogenesis.Citation191 Quantitative data have been extremely helpful in comparing ciliary parameters with laterality outcomes in zebrafish,Citation154 yet similar robust measures of cilia number, length, and flow rates are not available for the mouse model because few studies have assessed these endpoints, and those that did typically provide large ranges for the measured values and often rely on data from only 2 or 3 embryos in a treatment class,Citation62 which makes statistical evaluation impossible.
Finally, discussions within the LR community have identified some frustrations with the laterality models and the idea that one is correct and the others must be wrong. In a first step to a potential conciliation, we proposed the unified model of LR asymmetry, which postulates that cilia may have a role in amplifying LR information in some species, downstream of highly conserved cytoskeletal events that initiate asymmetry. Experiments could test whether cilia have an amplifying role in the LR pathway: reagents that target early mechanisms (i.e., BDM or mutant α-tubulin mRNAs, which affect the cytoskeleton but are only effective very early) could be combined with reagents that directly disrupt ciliary flow (i.e., methylcellulose injected into the GRP, which changes the viscosity of the fluid in this space) to determine whether additive effects can be obtained. This would also address the speculative proposalCitation62 that in any population of embryos, some are utilizing different pathways than others to reach correct asymmetry (thus explaining why the penetrance of any one type of inhibition is often incomplete at the level of the population, but very effective for some number of specific individuals).
In conclusion, evidence from a number of species points to the intracellular cytoskeleton as the initiator of LR asymmetry. New studies have identified how specific components of the cytoskeleton including α-tubulin and cytoskeletal-associated molecular motors can establish asymmetry during the earliest stages of embryogenesis. Recent results reveal considerable conservation of mechanisms, as well as divergent pathways that may have arisen in only a small number of species to accommodate distinct bodyplan architectures. Addressing which mechanisms operate in humans, and thus which animal model is most appropriate to study human laterality disorders, remains a critically important issue in the field of evolutionary developmental biology.
Additional material
Download Zip (180.1 KB)Disclosure of Potential Conflicts of Interest
No potential conflicts of interest were disclosed.
Acknowledgments
The authors are grateful to members of the Levin Laboratory for helpful discussions and support, and to an anonymous reviewer for suggesting the idea of symmetry breaking by microtubules and actin working as thermal ratchets. We also thank Joseph Frankel, Wallace Marshall, and Janine Beisson for their contributions to this field and fruitful conversations. This work was supported by an American Heart Association Established Investigator Grant [grant number 0740088N to Levin M]; and the National Institutes of Health [grant R01-GM077425 to Levin M, fellowship F32GM087107 to Vandenberg LN].
Supplemental Materials
Supplemental materials may be found here: www.landesbioscience.com/journals/cib/article/27155
References
- Aw S, Levin M. What’s left in asymmetry?. Dev Dyn 2008; 237:3453 - 63; http://dx.doi.org/10.1002/dvdy.21560; PMID: 18488999
- Li CC, Chodirker BN, Dawson AJ, Chudley AE. Severe hemihypotrophy in a female infant with mosaic Turner syndrome: a variant of Russell-Silver syndrome?. Clin Dysmorphol 2004; 13:95 - 8; http://dx.doi.org/10.1097/00019605-200404000-00008; PMID: 15057125
- Rajakulendran S, Kuntzer T, Dunand M, Yau SC, Ashton EJ, Storey H, McCauley J, Abbs S, Thonney F, Leturcq F, et al. Marked hemiatrophy in carriers of Duchenne muscular dystrophy. Arch Neurol 2010; 67:497 - 500; http://dx.doi.org/10.1001/archneurol.2010.58; PMID: 20385919
- Bod M, Czeizel A, Lenz W. Incidence at birth of different types of limb reduction abnormalities in Hungary 1975-1977. Hum Genet 1983; 65:27 - 33; http://dx.doi.org/10.1007/BF00285024; PMID: 6642505
- Paulozzi LJ, Lary JM. Laterality patterns in infants with external birth defects. Teratology 1999; 60:265 - 71; http://dx.doi.org/10.1002/(SICI)1096-9926(199911)60:5<265::AID-TERA7>3.0.CO;2-H; PMID: 10525204
- Gurnett CA, Alaee F, Kruse LM, Desruisseau DM, Hecht JT, Wise CA, Bowcock AM, Dobbs MB. Asymmetric lower-limb malformations in individuals with homeobox PITX1 gene mutation. Am J Hum Genet 2008; 83:616 - 22; http://dx.doi.org/10.1016/j.ajhg.2008.10.004; PMID: 18950742
- Wilting J, Hagedorn M. Left-right asymmetry in embryonic development and breast cancer: common molecular determinants?. Curr Med Chem 2011; 18:5519 - 27; http://dx.doi.org/10.2174/092986711798347252; PMID: 22172062
- Meguid RA, Slidell MB, Wolfgang CL, Chang DC, Ahuja N. Is there a difference in survival between right- versus left-sided colon cancers?. Ann Surg Oncol 2008; 15:2388 - 94; http://dx.doi.org/10.1245/s10434-008-0015-y; PMID: 18622647
- Benedix F, Kube R, Meyer F, Schmidt U, Gastinger I, Lippert H, Colon/Rectum Carcinomas (Primary Tumor) Study Group. Comparison of 17,641 patients with right- and left-sided colon cancer: differences in epidemiology, perioperative course, histology, and survival. Dis Colon Rectum 2010; 53:57 - 64; http://dx.doi.org/10.1007/DCR.0b013e3181c703a4; PMID: 20010352
- Singh H, Nugent Z, Demers AA, Kliewer EV, Mahmud SM, Bernstein CN. The reduction in colorectal cancer mortality after colonoscopy varies by site of the cancer. Gastroenterology 2010; 139:1128 - 37; http://dx.doi.org/10.1053/j.gastro.2010.06.052; PMID: 20600026
- Corballis MC. The evolution and genetics of cerebral asymmetry. Philos Trans R Soc Lond B Biol Sci 2009; 364:867 - 79; http://dx.doi.org/10.1098/rstb.2008.0232; PMID: 19064358
- Corballis MC. Lateralization of the human brain. Prog Brain Res 2012; 195:103 - 21; http://dx.doi.org/10.1016/B978-0-444-53860-4.00006-4; PMID: 22230625
- Crow TJ. The ‘big bang’ theory of the origin of psychosis and the faculty of language. Schizophr Res 2008; 102:31 - 52; http://dx.doi.org/10.1016/j.schres.2008.03.010; PMID: 18502103
- Leonard CM, Eckert MA. Asymmetry and dyslexia. Dev Neuropsychol 2008; 33:663 - 81; http://dx.doi.org/10.1080/87565640802418597; PMID: 19005910
- Uslu H, Dane S, Uyanik MH, Ayyildiz A. Relationships between intestinal parasitosis and handedness. Laterality 2010; 15:465 - 74; http://dx.doi.org/10.1080/13576500903049316; PMID: 19603343
- Krommydas GC, Gourgoulianis KI, Raftopoulos V, Kotrotsiou E, Paralikas T, Agorogiannis G, Molyvdas PA. Non-right-handedness and asthma. Allergy 2004; 59:892 - 3; http://dx.doi.org/10.1111/j.1398-9995.2004.00524.x; PMID: 15230828
- McManus IC, Bryden MP, Bulman-Fleming MB. Handedness and autoimmune disease. Lancet 1993; 341:891 - 2; http://dx.doi.org/10.1016/0140-6736(93)93098-L; PMID: 8096587
- Cohen MS, Anderson RH, Cohen MI, Atz AM, Fogel M, Gruber PJ, Lopez L, Rome JJ, Weinberg PM. Controversies, genetics, diagnostic assessment, and outcomes relating to the heterotaxy syndrome. Cardiol Young 2007; 17:Suppl 2 29 - 43; http://dx.doi.org/10.1017/S104795110700114X; PMID: 18039397
- Casey B, Hackett BP. Left-right axis malformations in man and mouse. Curr Opin Genet Dev 2000; 10:257 - 61; http://dx.doi.org/10.1016/S0959-437X(00)00085-X; PMID: 10826993
- Hackett BP. Formation and malformation of the vertebrate left-right axis. Curr Mol Med 2002; 2:39 - 66; http://dx.doi.org/10.2174/1566524023363031; PMID: 11898848
- Peeters H, Devriendt K. Human laterality disorders. Eur J Med Genet 2006; 49:349 - 62; http://dx.doi.org/10.1016/j.ejmg.2005.12.003; PMID: 16461029
- Vandenberg LN, Levin M. Perspectives and open problems in the early phases of left-right patterning. Semin Cell Dev Biol 2009; 20:456 - 63; http://dx.doi.org/10.1016/j.semcdb.2008.11.010; PMID: 19084609
- Levin M, Palmer AR. Left-right patterning from the inside out: widespread evidence for intracellular control. Bioessays 2007; 29:271 - 87; http://dx.doi.org/10.1002/bies.20545; PMID: 17295291
- Tabin CJ. Establishing robust left-right asymmetry in the vertebrate embryo. Dev Cell 2011; 20:e2; http://dx.doi.org/10.1016/j.devcel.2011.05.019
- Levin M. Left-right asymmetry and the chick embryo. Semin Cell Dev Biol 1998; 9:67 - 76; http://dx.doi.org/10.1006/scdb.1997.0192; PMID: 9572116
- Nakamura T, Mine N, Nakaguchi E, Mochizuki A, Yamamoto M, Yashiro K, Meno C, Hamada H. Generation of robust left-right asymmetry in the mouse embryo requires a self-enhancement and lateral-inhibition system. Dev Cell 2006; 11:495 - 504; http://dx.doi.org/10.1016/j.devcel.2006.08.002; PMID: 17011489
- Nakamura T, Hamada H. Left-right patterning: conserved and divergent mechanisms. Development 2012; 139:3257 - 62; http://dx.doi.org/10.1242/dev.061606; PMID: 22912409
- Spéder P, Petzoldt A, Suzanne M, Noselli S. Strategies to establish left/right asymmetry in vertebrates and invertebrates. Curr Opin Genet Dev 2007; 17:351 - 8; http://dx.doi.org/10.1016/j.gde.2007.05.008; PMID: 17643981
- Norris DP. Cilia, calcium and the basis of left-right asymmetry. BMC Biol 2012; 10:102; http://dx.doi.org/10.1186/1741-7007-10-102; PMID: 23256866
- Vandenberg LN, Levin M. Far from solved: a perspective on what we know about early mechanisms of left-right asymmetry. Dev Dyn 2010; 239:3131 - 46; http://dx.doi.org/10.1002/dvdy.22450; PMID: 21031419
- Vandenberg LN, Levin M. A unified model for left-right asymmetry? Comparison and synthesis of molecular models of embryonic laterality. Dev Biol 2013; 379:1 - 15; http://dx.doi.org/10.1016/j.ydbio.2013.03.021; PMID: 23583583
- Tabin CJ, Vogan KJ. A two-cilia model for vertebrate left-right axis specification. Genes Dev 2003; 17:1 - 6; http://dx.doi.org/10.1101/gad.1053803; PMID: 12514094
- McGrath J, Brueckner M. Cilia are at the heart of vertebrate left-right asymmetry. Curr Opin Genet Dev 2003; 13:385 - 92; http://dx.doi.org/10.1016/S0959-437X(03)00091-1; PMID: 12888012
- McGrath J, Somlo S, Makova S, Tian X, Brueckner M. Two populations of node monocilia initiate left-right asymmetry in the mouse. Cell 2003; 114:61 - 73; http://dx.doi.org/10.1016/S0092-8674(03)00511-7; PMID: 12859898
- Yost HJ. Left-right asymmetry: nodal cilia make and catch a wave. Curr Biol 2003; 13:R808 - 9; http://dx.doi.org/10.1016/j.cub.2003.09.051; PMID: 14561422
- Tanaka Y, Okada Y, Hirokawa N. FGF-induced vesicular release of Sonic hedgehog and retinoic acid in leftward nodal flow is critical for left-right determination. Nature 2005; 435:172 - 7; http://dx.doi.org/10.1038/nature03494; PMID: 15889083
- Basu B, Brueckner M. Cilia multifunctional organelles at the center of vertebrate left-right asymmetry. Curr Top Dev Biol 2008; 85:151 - 74; http://dx.doi.org/10.1016/S0070-2153(08)00806-5; PMID: 19147005
- Shiratori H, Hamada H. The left-right axis in the mouse: from origin to morphology. Development 2006; 133:2095 - 104; http://dx.doi.org/10.1242/dev.02384; PMID: 16672339
- Gros J, Feistel K, Viebahn C, Blum M, Tabin CJ. Cell movements at Hensen’s node establish left/right asymmetric gene expression in the chick. Science 2009; 324:941 - 4; http://dx.doi.org/10.1126/science.1172478; PMID: 19359542
- Männer J. Does an equivalent of the “ventral node” exist in chick embryos? A scanning electron microscopic study. Anat Embryol (Berl) 2001; 203:481 - 90; http://dx.doi.org/10.1007/s004290100183; PMID: 11453165
- Bangs F, Antonio N, Thongnuek P, Welten M, Davey MG, Briscoe J, Tickle C. Generation of mice with functional inactivation of talpid3, a gene first identified in chicken. Development 2011; 138:3261 - 72; http://dx.doi.org/10.1242/dev.063602; PMID: 21750036
- Okumura T, Utsuno H, Kuroda J, Gittenberger E, Asami T, Matsuno K. The development and evolution of left-right asymmetry in invertebrates: lessons from Drosophila and snails. Dev Dyn 2008; 237:3497 - 515; http://dx.doi.org/10.1002/dvdy.21788; PMID: 19035360
- Serluca FC, Xu B, Okabe N, Baker K, Lin SY, Sullivan-Brown J, Konieczkowski DJ, Jaffe KM, Bradner JM, Fishman MC, et al. Mutations in zebrafish leucine-rich repeat-containing six-like affect cilia motility and result in pronephric cysts, but have variable effects on left-right patterning. Development 2009; 136:1621 - 31; http://dx.doi.org/10.1242/dev.020735; PMID: 19395640
- Zhao C, Malicki J. Genetic defects of pronephric cilia in zebrafish. Mech Dev 2007; 124:605 - 16; http://dx.doi.org/10.1016/j.mod.2007.04.004; PMID: 17576052
- Valente EM, Logan CV, Mougou-Zerelli S, Lee JH, Silhavy JL, Brancati F, Iannicelli M, Travaglini L, Romani S, Illi B, et al. Mutations in TMEM216 perturb ciliogenesis and cause Joubert, Meckel and related syndromes. Nat Genet 2010; 42:619 - 25; http://dx.doi.org/10.1038/ng.594; PMID: 20512146
- Zeng H, Hoover AN, Liu A. PCP effector gene Inturned is an important regulator of cilia formation and embryonic development in mammals. Dev Biol 2010; 339:418 - 28; http://dx.doi.org/10.1016/j.ydbio.2010.01.003; PMID: 20067783
- Danilchik MV, Brown EE, Riegert K. Intrinsic chiral properties of the Xenopus egg cortex: an early indicator of left-right asymmetry?. Development 2006; 133:4517 - 26; http://dx.doi.org/10.1242/dev.02642; PMID: 17050623
- Levin M, Thorlin T, Robinson KR, Nogi T, Mercola M. Asymmetries in H+/K+-ATPase and cell membrane potentials comprise a very early step in left-right patterning. Cell 2002; 111:77 - 89; http://dx.doi.org/10.1016/S0092-8674(02)00939-X; PMID: 12372302
- Fukumoto T, Kema IP, Levin M. Serotonin signaling is a very early step in patterning of the left-right axis in chick and frog embryos. Curr Biol 2005; 15:794 - 803; http://dx.doi.org/10.1016/j.cub.2005.03.044; PMID: 15886096
- Adams DS, Robinson KR, Fukumoto T, Yuan S, Albertson RC, Yelick P, Kuo L, McSweeney M, Levin M. Early, H+-V-ATPase-dependent proton flux is necessary for consistent left-right patterning of non-mammalian vertebrates. Development 2006; 133:1657 - 71; http://dx.doi.org/10.1242/dev.02341; PMID: 16554361
- Kramer KL, Barnette JE, Yost HJ. PKCgamma regulates syndecan-2 inside-out signaling during xenopus left-right development. Cell 2002; 111:981 - 90; http://dx.doi.org/10.1016/S0092-8674(02)01200-X; PMID: 12507425
- Fukumoto T, Levin M. Asymmetric expression of Syndecan-2 in early chick embryogenesis. Gene Expr Patterns 2005; 5:525 - 8; http://dx.doi.org/10.1016/j.modgep.2004.12.001; PMID: 15749081
- Stern CD, Yu RT, Kakizuka A, Kintner CR, Mathews LS, Vale WW, Evans RM, Umesono K. Activin and its receptors during gastrulation and the later phases of mesoderm development in the chick embryo. Dev Biol 1995; 172:192 - 205; http://dx.doi.org/10.1006/dbio.1995.0015; PMID: 7589799
- Qiu D, Cheng SM, Wozniak L, McSweeney M, Perrone E, Levin M. Localization and loss-of-function implicates ciliary proteins in early, cytoplasmic roles in left-right asymmetry. Dev Dyn 2005; 234:176 - 89; http://dx.doi.org/10.1002/dvdy.20509; PMID: 16059906
- Bunney TD, De Boer AH, Levin M. Fusicoccin signaling reveals 14-3-3 protein function as a novel step in left-right patterning during amphibian embryogenesis. Development 2003; 130:4847 - 58; http://dx.doi.org/10.1242/dev.00698; PMID: 12930777
- Albrieux M, Villaz M. Bilateral asymmetry of the inositol trisphosphate-mediated calcium signaling in two-cell ascidian embryos. Biol Cell 2000; 92:277 - 84; http://dx.doi.org/10.1016/S0248-4900(00)01066-2; PMID: 11043415
- Kramer KL, Yost HJ. Ectodermal syndecan-2 mediates left-right axis formation in migrating mesoderm as a cell-nonautonomous Vg1 cofactor. Dev Cell 2002; 2:115 - 24; http://dx.doi.org/10.1016/S1534-5807(01)00107-1; PMID: 11782319
- Ohkawara B, Niehrs C. An ATF2-based luciferase reporter to monitor non-canonical Wnt signaling in Xenopus embryos. Dev Dyn 2011; 240:188 - 94; http://dx.doi.org/10.1002/dvdy.22500; PMID: 21128306
- Roberts RM, Katayama M, Magnuson SR, Falduto MT, Torres KE. Transcript profiling of individual twin blastomeres derived by splitting two-cell stage murine embryos. Biol Reprod 2011; 84:487 - 94; http://dx.doi.org/10.1095/biolreprod.110.086884; PMID: 21076082
- Sun JH, Zhang Y, Yin BY, Li JX, Liu GS, Xu W, et al. Differential expression of Axin1, Cdc25c and Cdkn2d mRNA in 2-cell stage mouse blastomeres. Zygote 2011; •••:1 - 6; PMID: 22071115
- Vandenberg LN, Levin M. Far from solved: a perspective on what we know about early mechanisms of left-right asymmetry. Dev Dyn 2010; 239:3131 - 46; http://dx.doi.org/10.1002/dvdy.22450; PMID: 21031419
- Vandenberg LN, Levin M. A unified model for left-right asymmetry? Comparison and synthesis of molecular models of embryonic laterality. Dev Biol 2013; 379:1 - 15; http://dx.doi.org/10.1016/j.ydbio.2013.03.021; PMID: 23583583
- Levin M. Hypothesis: motor proteins and ion pumps, not ciliary motion, initiate LR asymmetry. Bioessays 2003; 25:1002 - 10; http://dx.doi.org/10.1002/bies.10339; PMID: 14505367
- Levin M. Left-right asymmetry in embryonic development: a comprehensive review. Mech Dev 2005; 122:3 - 25; http://dx.doi.org/10.1016/j.mod.2004.08.006; PMID: 15582774
- Levin M, Nascone N. Two molecular models of initial left-right asymmetry generation. Med Hypotheses 1997; 49:429 - 35; http://dx.doi.org/10.1016/S0306-9877(97)90092-X; PMID: 9421811
- Klar AJ. A model for specification of the left-right axis in vertebrates. Trends Genet 1994; 10:392 - 6; http://dx.doi.org/10.1016/0168-9525(94)90055-8; PMID: 7809944
- Armakolas A, Klar AJ. Left-right dynein motor implicated in selective chromatid segregation in mouse cells. Science 2007; 315:100 - 1; http://dx.doi.org/10.1126/science.1129429; PMID: 17204651
- Sauer S, Klar AJ. Left-right symmetry breaking in mice by left-right dynein may occur via a biased chromatid segregation mechanism, without directly involving the Nodal gene. Front Oncol 2012; 2:166; http://dx.doi.org/10.3389/fonc.2012.00166; PMID: 23316472
- Klar AJ. Support for the selective chromatid segregation hypothesis advanced for the mechanism of left-right body axis development in mice. Breast Dis 2008; 29:47 - 56; PMID: 19029624
- Aw S, Adams DS, Qiu D, Levin M. H,K-ATPase protein localization and Kir4.1 function reveal concordance of three axes during early determination of left-right asymmetry. Mech Dev 2008; 125:353 - 72; http://dx.doi.org/10.1016/j.mod.2007.10.011; PMID: 18160269
- Morokuma J, Blackiston D, Levin M. KCNQ1 and KCNE1 K+ channel components are involved in early left-right patterning in Xenopus laevis embryos. Cell Physiol Biochem 2008; 21:357 - 72; http://dx.doi.org/10.1159/000129628; PMID: 18453744
- Fukumoto T, Kema I, Nazarenko D, Levin M. Serotonin is a novel very early signaling mechanism in left-right asymmetry. Dev Biol 2003; 259:490
- Levin M, Mercola M. Gap junctions are involved in the early generation of left-right asymmetry. Dev Biol 1998; 203:90 - 105; http://dx.doi.org/10.1006/dbio.1998.9024; PMID: 9806775
- Carneiro K, Donnet C, Rejtar T, Karger BL, Barisone GA, Díaz E, Kortagere S, Lemire JM, Levin M. Histone deacetylase activity is necessary for left-right patterning during vertebrate development. BMC Dev Biol 2011; 11:29; http://dx.doi.org/10.1186/1471-213X-11-29; PMID: 21599922
- Kellogg DR, Moritz M, Alberts BM. The centrosome and cellular organization. Annu Rev Biochem 1994; 63:639 - 74; http://dx.doi.org/10.1146/annurev.bi.63.070194.003231; PMID: 7979251
- Bornens M. The centrosome in cells and organisms. Science 2012; 335:422 - 6; http://dx.doi.org/10.1126/science.1209037; PMID: 22282802
- Frankel J. Positional information in unicellular organisms. J Theor Biol 1974; 47:439 - 81; http://dx.doi.org/10.1016/0022-5193(74)90209-4; PMID: 4215927
- Frankel J. Positional information in cells and organisms. Trends Cell Biol 1992; 2:256 - 60; http://dx.doi.org/10.1016/0962-8924(92)90191-O; PMID: 14731517
- Beisson J, Jerka-Dziadosz M. Polarities of the centriolar structure: morphogenetic consequences. Biol Cell 1999; 91:367 - 78; PMID: 11419478
- Carvalho-Santos Z, Azimzadeh J, Pereira-Leal JB, Bettencourt-Dias M. Evolution: Tracing the origins of centrioles, cilia, and flagella. J Cell Biol 2011; 194:165 - 75; http://dx.doi.org/10.1083/jcb.201011152; PMID: 21788366
- Lambert JD, Nagy LM. Asymmetric inheritance of centrosomally localized mRNAs during embryonic cleavages. Nature 2002; 420:682 - 6; http://dx.doi.org/10.1038/nature01241; PMID: 12478296
- Fuentealba LC, Eivers E, Geissert D, Taelman V, De Robertis EM. Asymmetric mitosis: Unequal segregation of proteins destined for degradation. Proc Natl Acad Sci U S A 2008; 105:7732 - 7; http://dx.doi.org/10.1073/pnas.0803027105; PMID: 18511557
- Feldman JL, Geimer S, Marshall WF. The mother centriole plays an instructive role in defining cell geometry. PLoS Biol 2007; 5:e149; http://dx.doi.org/10.1371/journal.pbio.0050149; PMID: 17518519
- Cowan CR, Hyman AA. Centrosomes direct cell polarity independently of microtubule assembly in C. elegans embryos. Nature 2004; 431:92 - 6; http://dx.doi.org/10.1038/nature02825; PMID: 15343338
- Uzbekov RE, Alieva IB. The centrosome--a riddle of the “cell processor.”. Tsitologiia 2008; 50:91 - 112; PMID: 18540189
- Lacomble S, Vaughan S, Gadelha C, Morphew MK, Shaw MK, McIntosh JR, Gull K. Basal body movements orchestrate membrane organelle division and cell morphogenesis in Trypanosoma brucei. J Cell Sci 2010; 123:2884 - 91; http://dx.doi.org/10.1242/jcs.074161; PMID: 20682637
- Aw S, Levin M. Is left-right asymmetry a form of planar cell polarity?. Development 2009; 136:355 - 66; http://dx.doi.org/10.1242/dev.015974; PMID: 19141667
- Mizuno N, Taschner M, Engel BD, Lorentzen E. Structural studies of ciliary components. J Mol Biol 2012; 422:163 - 80; http://dx.doi.org/10.1016/j.jmb.2012.05.040; PMID: 22683354
- Desai RA, Gao L, Raghavan S, Liu WF, Chen CS. Cell polarity triggered by cell-cell adhesion via E-cadherin. J Cell Sci 2009; 122:905 - 11; http://dx.doi.org/10.1242/jcs.028183; PMID: 19258396
- Palazzo AF, Joseph HL, Chen YJ, Dujardin DL, Alberts AS, Pfister KK, Vallee RB, Gundersen GG. Cdc42, dynein, and dynactin regulate MTOC reorientation independent of Rho-regulated microtubule stabilization. Curr Biol 2001; 11:1536 - 41; http://dx.doi.org/10.1016/S0960-9822(01)00475-4; PMID: 11591323
- Luxton GW, Gundersen GG. Orientation and function of the nuclear-centrosomal axis during cell migration. Curr Opin Cell Biol 2011; 23:579 - 88; http://dx.doi.org/10.1016/j.ceb.2011.08.001; PMID: 21885270
- Vandenberg LN, Levin M. Polarity proteins are required for left-right axis orientation and twin-twin instruction. Genesis 2012; 50:219 - 34; http://dx.doi.org/10.1002/dvg.20825; PMID: 22086838
- Pohl C, Bao Z. Chiral forces organize left-right patterning in C. elegans by uncoupling midline and anteroposterior axis. Dev Cell 2010; 19:402 - 12; http://dx.doi.org/10.1016/j.devcel.2010.08.014; PMID: 20833362
- Xu J, Van Keymeulen A, Wakida NM, Carlton P, Berns MW, Bourne HR. Polarity reveals intrinsic cell chirality. Proc Natl Acad Sci U S A 2007; 104:9296 - 300; http://dx.doi.org/10.1073/pnas.0703153104; PMID: 17517645
- Endress PK. Evolution of floral symmetry. Curr Opin Plant Biol 2001; 4:86 - 91; http://dx.doi.org/10.1016/S1369-5266(00)00140-0; PMID: 11163173
- Hashimoto T. Molecular genetic analysis of left-right handedness in plants. Philos Trans R Soc Lond B Biol Sci 2002; 357:799 - 808; http://dx.doi.org/10.1098/rstb.2002.1088; PMID: 12079675
- Vermot J, Pourquié O. Retinoic acid coordinates somitogenesis and left-right patterning in vertebrate embryos. Nature 2005; 435:215 - 20; http://dx.doi.org/10.1038/nature03488; PMID: 15889094
- Thitamadee S, Tuchihara K, Hashimoto T. Microtubule basis for left-handed helical growth in Arabidopsis.. Nature 2002; 417:193 - 6; http://dx.doi.org/10.1038/417193a; PMID: 12000963
- Nakamura M, Hashimoto T. A mutation in the Arabidopsis gamma-tubulin-containing complex causes helical growth and abnormal microtubule branching. J Cell Sci 2009; 122:2208 - 17; http://dx.doi.org/10.1242/jcs.044131; PMID: 19509058
- Lobikin M, Wang G, Xu J, Hsieh YW, Chuang CF, Lemire JM, Levin M. Early, nonciliary role for microtubule proteins in left-right patterning is conserved across kingdoms. Proc Natl Acad Sci U S A 2012; 109:12586 - 91; http://dx.doi.org/10.1073/pnas.1202659109; PMID: 22802643
- Dawe HR, Minamide LS, Bamburg JR, Cramer LP. ADF/cofilin controls cell polarity during fibroblast migration. Curr Biol 2003; 13:252 - 7; http://dx.doi.org/10.1016/S0960-9822(03)00040-X; PMID: 12573223
- Mseka T, Bamburg JR, Cramer LP. ADF/cofilin family proteins control formation of oriented actin-filament bundles in the cell body to trigger fibroblast polarization. J Cell Sci 2007; 120:4332 - 44; http://dx.doi.org/10.1242/jcs.017640; PMID: 18042624
- Chuang CF, Vanhoven MK, Fetter RD, Verselis VK, Bargmann CI. An innexin-dependent cell network establishes left-right neuronal asymmetry in C. elegans.. Cell 2007; 129:787 - 99; http://dx.doi.org/10.1016/j.cell.2007.02.052; PMID: 17512411
- Chang C, Hsieh YW, Lesch BJ, Bargmann CI, Chuang CF. Microtubule-based localization of a synaptic calcium-signaling complex is required for left-right neuronal asymmetry in C. elegans. Development 2011; 138:3509 - 18; http://dx.doi.org/10.1242/dev.069740; PMID: 21771813
- Oviedo NJ, Levin M. Gap junctions provide new links in left-right patterning. Cell 2007; 129:645 - 7; http://dx.doi.org/10.1016/j.cell.2007.05.005; PMID: 17512395
- Lobikin M, Wang G, Xu J, Hsieh YW, Chuang CF, Lemire JM, Levin M. Early, nonciliary role for microtubule proteins in left-right patterning is conserved across kingdoms. Proc Natl Acad Sci U S A 2012; 109:12586 - 91; http://dx.doi.org/10.1073/pnas.1202659109; PMID: 22802643
- Heacock AM, Agranoff BW. Clockwise growth of neurites from retinal explants. Science 1977; 198:64 - 6; http://dx.doi.org/10.1126/science.897684; PMID: 897684
- Wan LQ, Vunjak-Novakovic G. Micropatterning chiral morphogenesis. Commun Integr Biol 2011; 4:745 - 8; PMID: 22446544
- Wan LQ, Ronaldson K, Park M, Taylor G, Zhang Y, Gimble JM, Vunjak-Novakovic G. Micropatterned mammalian cells exhibit phenotype-specific left-right asymmetry. Proc Natl Acad Sci U S A 2011; 108:12295 - 300; http://dx.doi.org/10.1073/pnas.1103834108; PMID: 21709270
- Frankel J. Intracellular handedness in ciliates. CIBA Found Symposium: Biological Asymmetry and Handedness, 1991:73-88.
- Nelsen EM, Frankel J, Jenkins LM. Non-genic inheritance of cellular handedness. Development 1989; 105:447 - 56; PMID: 2612360
- Alpatov VV. Specific action of optical isomers of mepacrine upon dextral and sinistral strains of Bacillus mycoides Flügge. Nature 1946; 158:838; http://dx.doi.org/10.1038/158838a0; PMID: 20276889
- Chen TH, Hsu JJ, Zhao X, Guo C, Wong MN, Huang Y, Li Z, Garfinkel A, Ho CM, Tintut Y, et al. Left-right symmetry breaking in tissue morphogenesis via cytoskeletal mechanics. Circ Res 2012; 110:551 - 9; http://dx.doi.org/10.1161/CIRCRESAHA.111.255927; PMID: 22223355
- Levin M. Is the early left-right axis like a plant, a kidney, or a neuron? The integration of physiological signals in embryonic asymmetry. Birth Defects Res C Embryo Today 2006; 78:191 - 223; http://dx.doi.org/10.1002/bdrc.20078; PMID: 17061264
- Kawakami Y, Raya A, Raya RM, Rodríguez-Esteban C, Izpisúa Belmonte JC. Retinoic acid signalling links left-right asymmetric patterning and bilaterally symmetric somitogenesis in the zebrafish embryo. Nature 2005; 435:165 - 71; http://dx.doi.org/10.1038/nature03512; PMID: 15889082
- Vandenberg LN, Pennarola BW, Levin M. Low frequency vibrations disrupt left-right patterning in the Xenopus embryo. PLoS One 2011; 6:e23306; http://dx.doi.org/10.1371/journal.pone.0023306; PMID: 21826245
- Vandenberg LN, Stevenson C, Levin M. Low frequency vibrations induce malformations in two aquatic species in a frequency-, waveform-, and direction-specific manner. PLoS One 2012; 7:e51473; http://dx.doi.org/10.1371/journal.pone.0051473; PMID: 23251546
- Gebhardt JC, Clemen AE, Jaud J, Rief M. Myosin-V is a mechanical ratchet. Proc Natl Acad Sci U S A 2006; 103:8680 - 5; http://dx.doi.org/10.1073/pnas.0510191103; PMID: 16731631
- Ali MY, Uemura S, Adachi K, Itoh H, Kinosita K Jr., Ishiwata S. Myosin V is a left-handed spiral motor on the right-handed actin helix. Nat Struct Biol 2002; 9:464 - 7; http://dx.doi.org/10.1038/nsb803; PMID: 12006986
- Nishizaka T, Yagi T, Tanaka Y, Ishiwata S. Right-handed rotation of an actin filament in an in vitro motile system. Nature 1993; 361:269 - 71; http://dx.doi.org/10.1038/361269a0; PMID: 8423853
- Gardiner J, Marc J, Overall R. Cytoskeletal thermal ratchets and cytoskeletal tensegrity: determinants of brain asymmetry and symmetry?. Front Biosci 2008; 13:4649 - 56; http://dx.doi.org/10.2741/3030; PMID: 18508536
- Vandenberg LN, Levin M. Consistent left-right asymmetry cannot be established by late organizers in Xenopus unless the late organizer is a conjoined twin. Development 2010; 137:1095 - 105; http://dx.doi.org/10.1242/dev.041798; PMID: 20215347
- Scharf SR, Gerhart JC. Axis determination in eggs of Xenopus laevis: a critical period before first cleavage, identified by the common effects of cold, pressure and ultraviolet irradiation. Dev Biol 1983; 99:75 - 87; http://dx.doi.org/10.1016/0012-1606(83)90255-5; PMID: 6684607
- Scharf SR, Gerhart JC. Determination of the dorsal-ventral axis in eggs of Xenopus laevis: complete rescue of uv-impaired eggs by oblique orientation before first cleavage. Dev Biol 1980; 79:181 - 98; http://dx.doi.org/10.1016/0012-1606(80)90082-2; PMID: 7409319
- Kao KR, Elinson RP. Dorsalization of mesoderm induction by lithium. Dev Biol 1989; 132:81 - 90; http://dx.doi.org/10.1016/0012-1606(89)90207-8; PMID: 2917698
- Nascone N, Mercola M. Organizer induction determines left-right asymmetry in Xenopus.. Dev Biol 1997; 189:68 - 78; http://dx.doi.org/10.1006/dbio.1997.8635; PMID: 9281338
- Gardner RL. Normal bias in the direction of fetal rotation depends on blastomere composition during early cleavage in the mouse. PLoS One 2010; 5:e9610; http://dx.doi.org/10.1371/journal.pone.0009610; PMID: 20224769
- Schatten H, Sun QY. New insights into the role of centrosomes in mammalian fertilization and implications for ART. Reproduction 2011; 142:793 - 801; http://dx.doi.org/10.1530/REP-11-0261; PMID: 21911443
- Snook RR, Hosken DJ, Karr TL. The biology and evolution of polyspermy: insights from cellular and functional studies of sperm and centrosomal behavior in the fertilized egg. Reproduction 2011; 142:779 - 92; http://dx.doi.org/10.1530/REP-11-0255; PMID: 21964827
- Mitchison T, Wühr M, Nguyen P, Ishihara K, Groen A, Field CM. Growth, interaction, and positioning of microtubule asters in extremely large vertebrate embryo cells. Cytoskeleton (Hoboken) 2012; 69:738 - 50; http://dx.doi.org/10.1002/cm.21050; PMID: 22786885
- Tournier F, Karsenti E, Bornens M. Parthenogenesis in Xenopus eggs injected with centrosomes from synchronized human lymphoid cells. Dev Biol 1989; 136:321 - 9; http://dx.doi.org/10.1016/0012-1606(89)90259-5; PMID: 2583369
- Bienkowska D, Cowan CR. Centrosomes can initiate a polarity axis from any position within one-cell C. elegans embryos. Curr Biol 2012; 22:583 - 9; http://dx.doi.org/10.1016/j.cub.2012.01.064; PMID: 22425158
- Lalli G. Crucial polarity regulators in axon specification. Essays Biochem 2012; 53:55 - 68; http://dx.doi.org/10.1042/bse0530055; PMID: 22928508
- Marshall WF. Centriole asymmetry determines algal cell geometry. Curr Opin Plant Biol 2012; 15:632 - 7; http://dx.doi.org/10.1016/j.pbi.2012.09.011; PMID: 23026116
- Marshall WF. Origins of cellular geometry. BMC Biol 2011; 9:57; http://dx.doi.org/10.1186/1741-7007-9-57; PMID: 21880160
- Rizzolo LJ, Joshi HC. Apical orientation of the microtubule organizing center and associated gamma-tubulin during the polarization of the retinal pigment epithelium in vivo. Dev Biol 1993; 157:147 - 56; http://dx.doi.org/10.1006/dbio.1993.1119; PMID: 8482407
- Brown NA, Wolpert L. The development of handedness in left/right asymmetry. Development 1990; 109:1 - 9; PMID: 2209459
- Beisson J, Wright M. Basal body/centriole assembly and continuity. Curr Opin Cell Biol 2003; 15:96 - 104; http://dx.doi.org/10.1016/S0955-0674(02)00017-0; PMID: 12517710
- Vandenberg LN, Levin M. Perspectives and open problems in the early phases of left-right patterning. Semin Cell Dev Biol 2009; 20:456 - 63; http://dx.doi.org/10.1016/j.semcdb.2008.11.010; PMID: 19084609
- Vandenberg LN, Morrie RD, Seebohm G, Lemire JM, Levin M. Rab GTPases are required for early orientation of the left-right axis in Xenopus.. Mech Dev 2013; 130:254 - 71; http://dx.doi.org/10.1016/j.mod.2012.11.007; PMID: 23354119
- Bergmann DC, Lee M, Robertson B, Tsou MF, Rose LS, Wood WB. Embryonic handedness choice in C. elegans involves the Galpha protein GPA-16. Development 2003; 130:5731 - 40; http://dx.doi.org/10.1242/dev.00839; PMID: 14534142
- May-Simera HL, Kai M, Hernandez V, Osborn DP, Tada M, Beales PL. Bbs8, together with the planar cell polarity protein Vangl2, is required to establish left-right asymmetry in zebrafish. Dev Biol 2010; 345:215 - 25; http://dx.doi.org/10.1016/j.ydbio.2010.07.013; PMID: 20643117
- Ansley SJ, Badano JL, Blacque OE, Hill J, Hoskins BE, Leitch CC, Kim JC, Ross AJ, Eichers ER, Teslovich TM, et al. Basal body dysfunction is a likely cause of pleiotropic Bardet-Biedl syndrome. Nature 2003; 425:628 - 33; http://dx.doi.org/10.1038/nature02030; PMID: 14520415
- Aw S, Levin M. What’s left in asymmetry?. Dev Dyn 2008; 237:3453 - 63; http://dx.doi.org/10.1002/dvdy.21560; PMID: 18488999
- Chen TH, Hsu JJ, Zhao X, Guo C, Wong MN, Huang Y, Li Z, Garfinkel A, Ho CM, Tintut Y, et al. Left-right symmetry breaking in tissue morphogenesis via cytoskeletal mechanics. Circ Res 2012; 110:551 - 9; http://dx.doi.org/10.1161/CIRCRESAHA.111.255927; PMID: 22223355
- Werner ME, Ward HH, Phillips CL, Miller C, Gattone VH 2nd, Bacallao RL. Inversin modulates the cortical actin network during mitosis. Am J Physiol Cell Physiol 2013; 305:C36 - 47; http://dx.doi.org/10.1152/ajpcell.00279.2012; PMID: 23515530
- Morgan D, Turnpenny L, Goodship J, Dai W, Majumder K, Matthews L, Gardner A, Schuster G, Vien L, Harrison W, et al. Inversin, a novel gene in the vertebrate left-right axis pathway, is partially deleted in the inv mouse. Nat Genet 1998; 20:149 - 56; http://dx.doi.org/10.1038/2450; PMID: 9771707
- Nürnberger J, Kribben A, Opazo Saez A, Heusch G, Philipp T, Phillips CL. The Invs gene encodes a microtubule-associated protein. J Am Soc Nephrol 2004; 15:1700 - 10; http://dx.doi.org/10.1097/01.ASN.0000128291.30249.D0; PMID: 15213257
- Spéder P, Adám G, Noselli S. Type ID unconventional myosin controls left-right asymmetry in Drosophila.. Nature 2006; 440:803 - 7; http://dx.doi.org/10.1038/nature04623; PMID: 16598259
- Aw S, Koster JC, Pearson W, Nichols CG, Shi NQ, Carneiro K, Levin M. The ATP-sensitive K(+)-channel (K(ATP)) controls early left-right patterning in Xenopus and chick embryos. Dev Biol 2010; 346:39 - 53; http://dx.doi.org/10.1016/j.ydbio.2010.07.011; PMID: 20643119
- Fukumoto T, Blakely R, Levin M. Serotonin transporter function is an early step in left-right patterning in chick and frog embryos. Dev Neurosci 2005; 27:349 - 63; http://dx.doi.org/10.1159/000088451; PMID: 16280633
- Hibino T, Ishii Y, Levin M, Nishino A. Ion flow regulates left-right asymmetry in sea urchin development. Dev Genes Evol 2006; 216:265 - 76; http://dx.doi.org/10.1007/s00427-005-0051-6; PMID: 16534626
- Levin M, Johnson RL, Stern CD, Kuehn M, Tabin C. A molecular pathway determining left-right asymmetry in chick embryogenesis. Cell 1995; 82:803 - 14; http://dx.doi.org/10.1016/0092-8674(95)90477-8; PMID: 7671308
- Vandenberg LN, Lemire JM, Levin M. Serotonin has early, cilia-independent roles in Xenopus left-right patterning. Dis Model Mech 2013; 6:261 - 8; http://dx.doi.org/10.1242/dmm.010256; PMID: 22899856
- Yost HJ. Development of the left-right axis in amphibians. Ciba Found Symp 1991; 162:165 - 76, discussion 176-81; PMID: 1802642
- Vladar EK, Bayly RD, Sangoram AM, Scott MP, Axelrod JD. Microtubules enable the planar cell polarity of airway cilia. Curr Biol 2012; 22:2203 - 12; http://dx.doi.org/10.1016/j.cub.2012.09.046; PMID: 23122850
- Antic D, Stubbs JL, Suyama K, Kintner C, Scott MP, Axelrod JD. Planar cell polarity enables posterior localization of nodal cilia and left-right axis determination during mouse and Xenopus embryogenesis. PLoS One 2010; 5:e8999; http://dx.doi.org/10.1371/journal.pone.0008999; PMID: 20126399
- Zhang Y, Levin M. Left-right asymmetry in the chick embryo requires core planar cell polarity protein Vangl2. Genesis 2009; 47:719 - 28; http://dx.doi.org/10.1002/dvg.20551; PMID: 19621439
- Shulman JM, St Johnston D. Pattern formation in single cells. Trends Cell Biol 1999; 9:M60 - 4; http://dx.doi.org/10.1016/S0962-8924(99)01680-3; PMID: 10611685
- Vandenberg LN, Morrie RD, Seebohm G, Lemire JM, Levin M. Rab GTPases are required for early orientation of the left-right axis in Xenopus.. Mech Dev 2013; 130:254 - 71; http://dx.doi.org/10.1016/j.mod.2012.11.007; PMID: 23354119
- Taniguchi K, Maeda R, Ando T, Okumura T, Nakazawa N, Hatori R, Nakamura M, Hozumi S, Fujiwara H, Matsuno K. Chirality in planar cell shape contributes to left-right asymmetric epithelial morphogenesis. Science 2011; 333:339 - 41; http://dx.doi.org/10.1126/science.1200940; PMID: 21764746
- Frankel J. Intracellular handedness in ciliates. Ciba Found Symp 1991; 162:73 - 88, discussion 88-93; PMID: 1802651
- Wood WB, Kershaw D. Handed asymmetry, handedness reversal and mechanisms of cell fate determination in nematode embryos. CIBA Found Symposium: Biological Asymmetry and Handedness, 1991:143-59; discussion 59-64.
- Shibazaki Y, Shimizu M, Kuroda R. Body handedness is directed by genetically determined cytoskeletal dynamics in the early embryo. Curr Biol 2004; 14:1462 - 7; http://dx.doi.org/10.1016/j.cub.2004.08.018; PMID: 15324662
- Dale L, Slack JM. Fate map for the 32-cell stage of Xenopus laevis.. Development 1987; 99:527 - 51; PMID: 3665770
- Moody SA, Kline MJ. Segregation of fate during cleavage of frog (Xenopus laevis) blastomeres. Anat Embryol (Berl) 1990; 182:347 - 62; http://dx.doi.org/10.1007/BF02433495; PMID: 2252221
- Blum M, Beyer T, Weber T, Vick P, Andre P, Bitzer E, Schweickert A. Xenopus, an ideal model system to study vertebrate left-right asymmetry. Dev Dyn 2009; 238:1215 - 25; http://dx.doi.org/10.1002/dvdy.21855; PMID: 19208433
- Koga M, Nakashima T, Matsuo S, Takeya R, Sumimoto H, Sakai M, Kageura H. High cell-autonomy of the anterior endomesoderm viewed in blastomere fate shift during regulative development in the isolated right halves of four-cell stage Xenopus embryos. Dev Growth Differ 2012; 54:717 - 29; http://dx.doi.org/10.1111/j.1440-169X.2012.01372.x; PMID: 22994797
- Vick P, Schweickert A, Weber T, Eberhardt M, Mencl S, Shcherbakov D, Beyer T, Blum M. Flow on the right side of the gastrocoel roof plate is dispensable for symmetry breakage in the frog Xenopus laevis. Dev Biol 2009; 331:281 - 91; http://dx.doi.org/10.1016/j.ydbio.2009.05.547; PMID: 19450574
- Amack JD, Yost HJ. The T box transcription factor no tail in ciliated cells controls zebrafish left-right asymmetry. Curr Biol 2004; 14:685 - 90; http://dx.doi.org/10.1016/j.cub.2004.04.002; PMID: 15084283
- Bisgrove BW, Makova S, Yost HJ, Brueckner M. RFX2 is essential in the ciliated organ of asymmetry and an RFX2 transgene identifies a population of ciliated cells sufficient for fluid flow. Dev Biol 2012; 363:166 - 78; http://dx.doi.org/10.1016/j.ydbio.2011.12.030; PMID: 22233545
- Cha SW, Tadjuidje E, Wylie C, Heasman J. The roles of maternal Vangl2 and aPKC in Xenopus oocyte and embryo patterning. Development 2011; 138:3989 - 4000; http://dx.doi.org/10.1242/dev.068866; PMID: 21813572
- Hyatt BA, Lohr JL, Yost HJ. Initiation of vertebrate left-right axis formation by maternal Vg1. Nature 1996; 384:62 - 5; http://dx.doi.org/10.1038/384062a0; PMID: 8900277
- Adams DS, Levin M. Inverse drug screens: a rapid and inexpensive method for implicating molecular targets. Genesis 2006; 44:530 - 40; http://dx.doi.org/10.1002/dvg.20246; PMID: 17078061
- Adams DS, Levin M. Strategies and techniques for investigation of biophysical signals in patterning. In: Whitman M, Sater AK, eds. Analysis of Growth Factor Signaling in Embryos: Taylor and Francis Books, 2006:177-262.
- Baffet AD, Benoit B, Januschke J, Audo J, Gourhand V, Roth S, Guichet A. Drosophila tubulin-binding cofactor B is required for microtubule network formation and for cell polarity. Mol Biol Cell 2012; 23:3591 - 601; http://dx.doi.org/10.1091/mbc.E11-07-0633; PMID: 22855530
- Cau J, Hall A. Cdc42 controls the polarity of the actin and microtubule cytoskeletons through two distinct signal transduction pathways. J Cell Sci 2005; 118:2579 - 87; http://dx.doi.org/10.1242/jcs.02385; PMID: 15928049
- Marszalek JR, Ruiz-Lozano P, Roberts E, Chien KR, Goldstein LS. Situs inversus and embryonic ciliary morphogenesis defects in mouse mutants lacking the KIF3A subunit of kinesin-II. Proc Natl Acad Sci U S A 1999; 96:5043 - 8; http://dx.doi.org/10.1073/pnas.96.9.5043; PMID: 10220415
- Happle R. Dohi Memorial Lecture. New aspects of cutaneous mosaicism. J Dermatol 2002; 29:681 - 92; PMID: 12484429
- Happle R. X-chromosome inactivation: role in skin disease expression. Acta Paediatr Suppl 2006; 95:16 - 23; http://dx.doi.org/10.1080/08035320600618775; PMID: 16720460
- König A, Happle R, Bornholdt D, Engel H, Grzeschik KH. Mutations in the NSDHL gene, encoding a 3beta-hydroxysteroid dehydrogenase, cause CHILD syndrome. Am J Med Genet 2000; 90:339 - 46; http://dx.doi.org/10.1002/(SICI)1096-8628(20000214)90:4<339::AID-AJMG15>3.0.CO;2-5; PMID: 10710235
- Liptau H, Viebahn C. Expression patterns of gap junctional proteins connexin 32 and 43 suggest new communication compartments in the gastrulating rabbit embryo. Differentiation 1999; 65:209 - 19; http://dx.doi.org/10.1046/j.1432-0436.1999.6540209.x; PMID: 10653357
- Viebahn C, Mayer B, Hrabé de Angelis M. Signs of the principle body axes prior to primitive streak formation in the rabbit embryo. Anat Embryol (Berl) 1995; 192:159 - 69; http://dx.doi.org/10.1007/BF00186004; PMID: 7486012
- Pohl C. Left-right patterning in the C. elegans embryo: Unique mechanisms and common principles. Commun Integr Biol 2011; 4:34 - 40; PMID: 21509174
- Kuroda R, Endo B, Abe M, Shimizu M. Chiral blastomere arrangement dictates zygotic left-right asymmetry pathway in snails. Nature 2009; 462:790 - 4; http://dx.doi.org/10.1038/nature08597; PMID: 19940849
- McManus C. Reversed bodies, reversed brains, and (some) reversed behaviors: of zebrafish and men. Dev Cell 2005; 8:796 - 7; http://dx.doi.org/10.1016/j.devcel.2005.05.006; PMID: 15935767
- Afzelius BA. A human syndrome caused by immotile cilia. Science 1976; 193:317 - 9; http://dx.doi.org/10.1126/science.1084576; PMID: 1084576
- Schneider H, Brueckner M. Of mice and men: dissecting the genetic pathway that controls left-right asymmetry in mice and humans. Am J Med Genet 2000; 97:258 - 70; http://dx.doi.org/10.1002/1096-8628(200024)97:4<258::AID-AJMG1276>3.0.CO;2-8; PMID: 11376437
- Danilchik M, Williams M, Brown E. Blastocoel-spanning filopodia in cleavage-stage Xenopus laevis: Potential roles in morphogen distribution and detection. Dev Biol 2013; 382:70 - 81; http://dx.doi.org/10.1016/j.ydbio.2013.07.024; PMID: 23916849
- Vandenberg LN. Laterality defects are influenced by timing of treatments and animal model. Differentiation 2012; 83:26 - 37; http://dx.doi.org/10.1016/j.diff.2011.08.004; PMID: 22099174
- Yost HJ. Left-right development in Xenopus and zebrafish. Semin Cell Dev Biol 1998; 9:61 - 6; http://dx.doi.org/10.1006/scdb.1997.0191; PMID: 9572115
- Vandenberg LN, Lemire JM, Levin M. Serotonin has early, cilia-independent roles in Xenopus left-right patterning. Dis Model Mech 2012; PMID: 22899856
- Carneiro K, Donnet C, Rejtar T, Karger BL, Barisone GA, Díaz E, Kortagere S, Lemire JM, Levin M. Histone deacetylase activity is necessary for left-right patterning during vertebrate development. BMC Dev Biol 2011; 11:29; http://dx.doi.org/10.1186/1471-213X-11-29; PMID: 21599922
- Levin M, Buznikov GA, Lauder JM. Of minds and embryos: left-right asymmetry and the serotonergic controls of pre-neural morphogenesis. Dev Neurosci 2006; 28:171 - 85; http://dx.doi.org/10.1159/000091915; PMID: 16679764
- Esser AT, Smith KC, Weaver JC, Levin M. Mathematical model of morphogen electrophoresis through gap junctions. Dev Dyn 2006; 235:2144 - 59; http://dx.doi.org/10.1002/dvdy.20870; PMID: 16786594
- Freeman G, Lundelius JW. The Developmental Genetics of Dextrality and Sinistrality in the Gastropod Lymnaea-Peregra. Wilhelm Rouxs Archives of Developmental Biology 1982; 191:69 - 83; http://dx.doi.org/10.1007/BF00848443
- Bessodes N, Haillot E, Duboc V, Röttinger E, Lahaye F, Lepage T. Reciprocal signaling between the ectoderm and a mesendodermal left-right organizer directs left-right determination in the sea urchin embryo. PLoS Genet 2012; 8:e1003121; http://dx.doi.org/10.1371/journal.pgen.1003121; PMID: 23271979
- Shimeld SM, Levin M. Evidence for the regulation of left-right asymmetry in Ciona intestinalis by ion flux. Dev Dyn 2006; 235:1543 - 53; http://dx.doi.org/10.1002/dvdy.20792; PMID: 16586445
- Thompson H, Shaw MK, Dawe HR, Shimeld SM. The formation and positioning of cilia in Ciona intestinalis embryos in relation to the generation and evolution of chordate left-right asymmetry. Dev Biol 2012; 364:214 - 23; http://dx.doi.org/10.1016/j.ydbio.2012.02.002; PMID: 22342242
- Essner JJ, Amack JD, Nyholm MK, Harris EB, Yost HJ. Kupffer’s vesicle is a ciliated organ of asymmetry in the zebrafish embryo that initiates left-right development of the brain, heart and gut. Development 2005; 132:1247 - 60; http://dx.doi.org/10.1242/dev.01663; PMID: 15716348
- Francescatto L, Rothschild SC, Myers AL, Tombes RM. The activation of membrane targeted CaMK-II in the zebrafish Kupffer’s vesicle is required for left-right asymmetry. Development 2010; 137:2753 - 62; http://dx.doi.org/10.1242/dev.049627; PMID: 20630945
- Qiu D, Cheng SM, Wozniak L, McSweeney M, Perrone E, Levin M. Localization and Loss-of-function Suggest Early, Cytoplasmic Roles for “ciliary” Proteins in Embryonic Left-Right Asymmetry. Developmental Dynamics 2005; http://dx.doi.org/10.1002/dvdy.20509
- Levin M, Mercola M. Gap junctions are involved in the early generation of left-right asymmetry. Dev Biol 1998; 203:90 - 105; http://dx.doi.org/10.1006/dbio.1998.9024; PMID: 9806775
- Schweickert A, Weber T, Beyer T, Vick P, Bogusch S, Feistel K, Blum M. Cilia-driven leftward flow determines laterality in Xenopus.. Curr Biol 2007; 17:60 - 6; http://dx.doi.org/10.1016/j.cub.2006.10.067; PMID: 17208188
- Levin M, Mercola M. Gap junction-mediated transfer of left-right patterning signals in the early chick blastoderm is upstream of Shh asymmetry in the node. Development 1999; 126:4703 - 14; PMID: 10518488
- Muders K, Fischer A, Blum M. Gap junctions mediate asymmetric gene expression in rabbit embryos. Dev Biol 2006; 295:450 - 1; http://dx.doi.org/10.1016/j.ydbio.2006.04.389
- Nonaka S, Shiratori H, Saijoh Y, Hamada H. Determination of left-right patterning of the mouse embryo by artificial nodal flow. Nature 2002; 418:96 - 9; http://dx.doi.org/10.1038/nature00849; PMID: 12097914