Abstract
The destruction of normal synaptic architecture is the main pathogenetic substrate in HIV-associated neurocognitive disorder (HAND), but the sequence of cellular events underlying this outcome is not completely understood. Our recent work in a mouse model of HAND using a single intraparenchymal injection of the HIV-1 regulatory protein trans-activator of transcription revealed increased microglial phagocytosis that was accompanied by an increased release of pro-inflammatory cytokines and elimination of dendritic spines in vivo, thus suggesting that microglia-synapse interactions could be dysregulated in HAND. Here, we further examine the relationships between microglia and synaptic structures in our mouse model, at high spatial resolution using immunocytochemical electron microscopy. Our ultrastructural analysis reveals the prevalence of putative microglial filopodial protrusions, which are targeting excitatory and inhibitory synapses, some of which contain phagocytic inclusions at various distances from their distal extremities to the microglial cell bodies. These observations thus suggest that cell-to-cell contacts mediated by microglial filopodia might be a crucial preliminary step in the elimination of synaptic structures in a neuroinflammatory milieu that occurs in HAND.
These past few years, a series of discoveries have challenged our view of microglia, the resident immune cells of the brain, by revealing their contribution to normal brain functions.Citation1-Citation6 In particular, microglial contacts with pre-synaptic axon terminals and post-synaptic dendritic spines were observed throughout normal lifespan,Citation7-Citation9 sometimes accompanied by the structural remodeling, engulfment and phagocytic elimination of synaptic structures.Citation7,Citation10,Citation11 Importantly, compromising this microglial ability to eliminate axon terminals during postnatal development resulted in a sustained deficit of synaptic connectivity into adulthood.Citation11 These observations demonstrated that microglia could be involved in synaptic plasticity, in addition to preserving the integrity of neuronal circuits.Citation2,Citation12 In contrast, considerably less is known about the early sequence of events that lead to synaptic destruction by microglia during neurodegenerative disease with a neuroinflammatory component.
Destruction of neurons and synapses could be directly caused by microglial phagocytosis, as suggested by our recent observations in a mouse model of HAND.Citation13,Citation14 HAND affects as many as half of all patients living with HIV-1, despite the fact that combination antiretroviral treatment can reduce viral burden to nearly undetectable levels in cerebrospinal fluid and serum. There is growing consensus that destruction of normal synaptic architecture is the main pathogenic mechanism in HAND,Citation15-Citation17 but the sequence of cellular events leading to this outcome are not completely understood. In our mouse model,Citation13,Citation14 we found that intra-cortical injection of the viral protein trans-activator of transcription (Tat1–72) increases the brain levels of pro-inflammatory cytokines in vivo, the prevalence of phagocytic inclusions inside of microglial cell bodies and processes in situ, and the loss of dendritic spines in vivo,Citation13 thus suggesting that microglia-synapse interactions could be dysregulated in HAND.
To further investigate these findings, here we examined the ultrastructural changes in microglia-synapse interactions in our mouse model of HAND using immunocytochemical electron microscopy. The animals were perfused with a solution of acrolein and paraformaldehyde, 7 d following injection of Tat or saline (n = 3 adult CX3CR1-GFP+/− mice in each group). The brains were afterwards cut with a vibratome to yield 50 μm of sections. These sections were stained freely-floating for ionized calcium binding adaptor molecule 1 (IBA1) using diaminobenzidine (DAB), which produces an electron-dense precipitate, in order to visualize microglial cell bodies and processes, and to analyze their ultrastructural interactions with all the surrounding elements of neuropil including synapses.Citation7,Citation18 Using IBA1 in this paradigm cannot exclusively identify microglia, because Tat can induce a chemotactic gradient resulting in the influx of inflammatory leukocytes,Citation14 including monocyte-derived macrophages that are also capable of phagocytosis and can be labeled by IBA1. While microglia can be further differentiated from perivascular macrophages and peripheral inflammatory leukocytes trafficking into the inflamed CNS based on the relative levels of CD45 expression by flow cytometry,Citation19,Citation20 this is not a methodological strategy that is compatible with the present ultrastructural study. Therefore, we have elected to refer to IBA1-positive cells as “putative” microglia. In each animal, 2000 μm2 of neuropil was randomly sampled in the superficial cortical layers, i.e., between 2 and 3 mm from the injection site, and analyzed using the same series of pictures and criteria for ultrastructural identification as in our recent study.Citation13
This additional analysis revealed that putative microglial cell bodies are generally enlarged following Tat-injection (which was noted at the light level using two photon microscopy of cortical windows, but did not reach significance – see Figure 7A from our recent studyCitation13), and they often extend filopodial protrusions contacting with dendritic spines, axon terminals, and asymmetrical synapses between axon terminals and dendritic spines (), but also dendrites () and symmetrical synapses between axon terminals and dendrites (). Electron micrographs showing examples of putative microglial cells in the saline-injected controls are also provided in . In both Tat- and saline-injected animals, the putative microglial cells were typically surrounded by pockets of extracellular space ( and ), as during normal conditions,Citation7 thus suggesting the release of extracellular proteases, such as metalloproteinases, plasminogens and cathepsinsCitation21 which could influence the structural remodeling and subsequent elimination of dendritic spines.Citation22,Citation23 Filopodia are considered to be cytoplasmic “microspikes” on the leading edge of lamellipodia and they are well-known for their roles in cell-to-cell interactions.Citation24 Similarly, microglial filopodia were previously described in autism postmortem brains, but only at the light microscopic level.Citation25 Microglial interactions with symmetrical synapses (which are considered to be inhibitory) have not been described yet, but the notion of “synaptic stripping” already suggested that microglia could remove inhibitory synapses from neuronal cell bodies and dendrites by intervening processes between them.Citation26,Citation27
Figure 1. Putative microglial filopodial protrusions contacting synaptic elements in a mouse model of HIV-associated neurocognitive disorder (HAND). A-B: Filopodia from the same cell (shown by the arrows in A) are simultaneously targeting three asymmetrical synapses between axon terminals (t) and dendritic spines (s). C: A putative microglial process that contains a vacuole (v) is simultaneously touching a dendritic spine and a symmetrical synapse between an axon terminal and a dendrite (d). D: Another process juxtaposing a neuronal cell body (n) is contacting an axon terminal and an astrocytic process (a). m, putative microglia; ma, myelinated axon. Pockets of extracellular space are shown by the asterisks (*). Scale bars: 1μm.
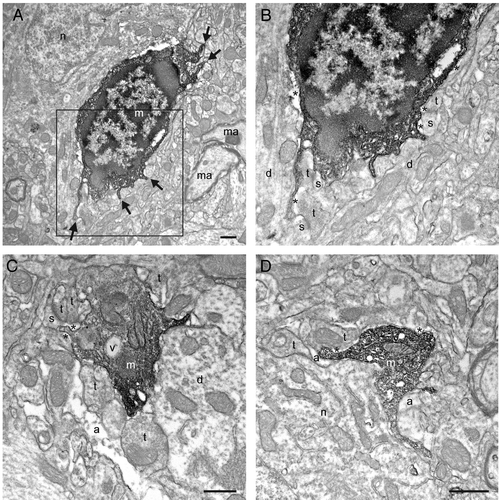
Figure 2. Additional examples of putative microglial filopodial protrusions contacting synaptic elements (A-D), proximal dendrites (B-C), a blood vessel (bv; C), and myelinated axons (D). Note that B and Care showing a magnified view of the putative microglia in A. Annotations as in . Scale bars: 1μm.
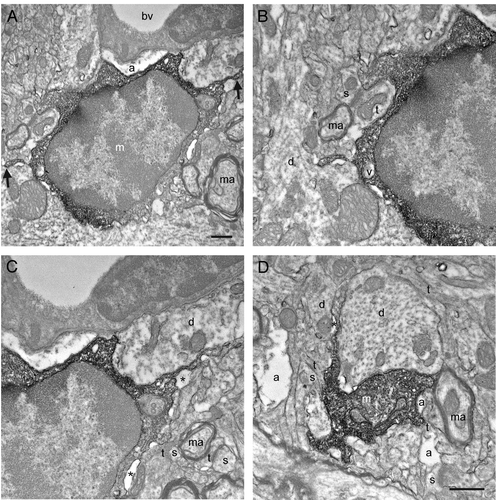
Figure 3. Examples of putative microglial cell body, large and small processes in the saline-injected controls (A-D) observed in direct juxtaposition with synaptic elements like dendritic spines (s) and axon terminals (t), as well as perisynaptic astrocytic processes (a). Note the absence of filopodial protrusions from the cell body in A, as during normal physiological conditions. Annotations as in and . Scale bars: 1μm.
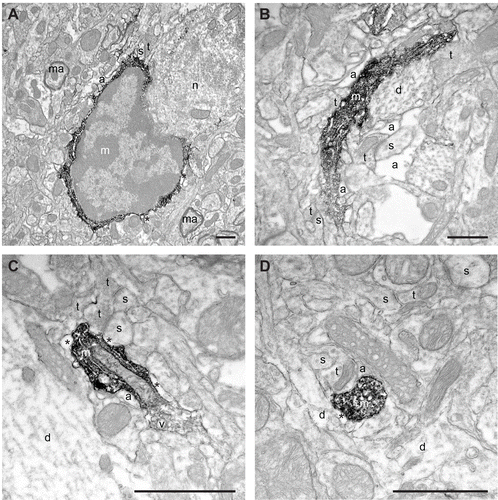
Specifically in the Tat-injected animals, we also observed putative microglial filopodia containing phagocytic inclusions at various distances between their distal extremities () and their cell bodies (see for an example of proximal process containing several inclusions). Some of these phagocytic inclusions displayed ultrastructural features of axon terminals and dendritic spines, such as clearly visible synaptic vesicles (see for examples), post-synaptic densities and spine apparatuses (see ), typically surrounded by extracellular space, thus suggesting their ongoing proteolytic degradation. In support of this finding, macrophage filopodia can act as phagocytic tentacles taking up cellular materials to be digested into the cell bodies.Citation28 Microglial engulfment of tissue components subsequently transported toward the cell bodies were also observed in vivo during normal conditions.Citation29 Therefore, our ultrastructural observations suggest that cell-to-cell contacts mediated by microglial filopodia might be a crucial preliminary step in the elimination of synaptic structures in vulnerable synapses in a neuroinflammatory milieu caused by HIV-1 Tat, which can be released even in the absence of productive viral replication. The identification of this key step in synaptic phagocytosis is likely to advance our understanding of crucial molecular species that mediate these interactions, leading to HAND.
Figure 4. Filopodial protrusions displaying phagocytic inclusions (in) nearby their extremity (A) and closer to the cell body (B). In C, a putative proximal microglial process is containing several cellular inclusions, including two axon terminals (t), a dendritic spine (s) and two vacuoles (v). Note that the engulfed elements are typically surrounded by extracellular space, which suggests their ongoing proteolytic degradation. Other annotations as in , and . Scale bars: 1μm.
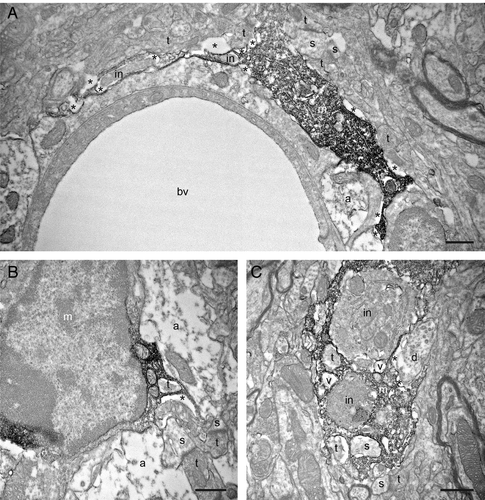
Disclosure of Potential Conflicts of Interest
No potential conflicts of interest were disclosed.
Acknowledgment
This work was supported by grants from the Banting Research Foundation and the Scottish Rite Charitable Foundation of Canada to M.-È.T.; and NIH grants MH64570 and MH56838 to H.A.G.
The authors sincerely thank Karen Bentley and Gayle Schneider at the University of Rochester EM Core Facility for their technical assistance.
References
- Hellwig S, Heinrich A, Biber K. The brain’s best friend: microglial neurotoxicity revisited. Front Cell Neurosci 2013; 7:71; http://dx.doi.org/10.3389/fncel.2013.00071; PMID: 23734099
- Kettenmann H, Kirchhoff F, Verkhratsky A. Microglia: new roles for the synaptic stripper. Neuron 2013; 77:10 - 8; http://dx.doi.org/10.1016/j.neuron.2012.12.023; PMID: 23312512
- Wake H, Moorhouse AJ, Miyamoto A, Nabekura J. Microglia: actively surveying and shaping neuronal circuit structure and function. Trends Neurosci 2013; 36:209 - 17; http://dx.doi.org/10.1016/j.tins.2012.11.007; PMID: 23260014
- Miyamoto A, Wake H, Moorhouse AJ, Nabekura J. Microglia and synapse interactions: fine tuning neural circuits and candidate molecules. Front Cell Neurosci 2013; 7:70; http://dx.doi.org/10.3389/fncel.2013.00070; PMID: 23720611
- Schafer DP, Stevens B. Phagocytic glial cells: sculpting synaptic circuits in the developing nervous system. Curr Opin Neurobiol 2013; 23:1034 - 40; http://dx.doi.org/10.1016/j.conb.2013.09.012; PMID: 24157239
- Tremblay ME, Stevens B, Sierra A, Wake H, Bessis A, Nimmerjahn A. The role of microglia in the healthy brain. J Neurosci 2011; 31:16064 - 9; http://dx.doi.org/10.1523/JNEUROSCI.4158-11.2011; PMID: 22072657
- Tremblay ME, Lowery RL, Majewska AK. Microglial interactions with synapses are modulated by visual experience. PLoS Biol 2010; 8:e1000527; http://dx.doi.org/10.1371/journal.pbio.1000527; PMID: 21072242
- Tremblay ME, Zettel ML, Ison JR, Allen PD, Majewska AK. Effects of aging and sensory loss on glial cells in mouse visual and auditory cortices. Glia 2012; 60:541 - 58; http://dx.doi.org/10.1002/glia.22287; PMID: 22223464
- Wake H, Moorhouse AJ, Jinno S, Kohsaka S, Nabekura J. Resting microglia directly monitor the functional state of synapses in vivo and determine the fate of ischemic terminals. J Neurosci 2009; 29:3974 - 80; http://dx.doi.org/10.1523/JNEUROSCI.4363-08.2009; PMID: 19339593
- Paolicelli RC, Bolasco G, Pagani F, Maggi L, Scianni M, Panzanelli P, Giustetto M, Ferreira TA, Guiducci E, Dumas L, et al. Synaptic pruning by microglia is necessary for normal brain development. Science 2011; 333:1456 - 8; http://dx.doi.org/10.1126/science.1202529; PMID: 21778362
- Schafer DP, Lehrman EK, Kautzman AG, Koyama R, Mardinly AR, Yamasaki R, Ransohoff RM, Greenberg ME, Barres BA, Stevens B. Microglia sculpt postnatal neural circuits in an activity and complement-dependent manner. Neuron 2012; 74:691 - 705; http://dx.doi.org/10.1016/j.neuron.2012.03.026; PMID: 22632727
- Tremblay ME, Majewska AK. A role for microglia in synaptic plasticity?. Commun Integr Biol 2011; 4:220 - 2; http://dx.doi.org/10.4161/cib.4.2.14506; PMID: 21655446
- Marker DF, Tremblay ME, Puccini JM, Barbieri J, Gantz Marker MA, Loweth CJ, Muly EC, Lu SM, Goodfellow VS, Dewhurst S, et al. The new small-molecule mixed-lineage kinase 3 inhibitor URMC-099 is neuroprotective and anti-inflammatory in models of human immunodeficiency virus-associated neurocognitive disorders. J Neurosci 2013; 33:9998 - 10010; http://dx.doi.org/10.1523/JNEUROSCI.0598-13.2013; PMID: 23761895
- Lu SM, Tremblay ME, King IL, Qi J, Reynolds HM, Marker DF, Varrone JJ, Majewska AK, Dewhurst S, Gelbard HA. HIV-1 Tat-induced microgliosis and synaptic damage via interactions between peripheral and central myeloid cells. PLoS One 2011; 6:e23915; http://dx.doi.org/10.1371/journal.pone.0023915; PMID: 21912650
- Masliah E, Heaton RK, Marcotte TD, Ellis RJ, Wiley CA, Mallory M, Achim CL, McCutchan JA, Nelson JA, Atkinson JH, et al, HNRC Group. The HIV Neurobehavioral Research Center. Dendritic injury is a pathological substrate for human immunodeficiency virus-related cognitive disorders. Ann Neurol 1997; 42:963 - 72; http://dx.doi.org/10.1002/ana.410420618; PMID: 9403489
- Glass JD, Wesselingh SL, Selnes OA, McArthur JC. Clinical-neuropathologic correlation in HIV-associated dementia. Neurology 1993; 43:2230 - 7; http://dx.doi.org/10.1212/WNL.43.11.2230; PMID: 8232935
- Everall IP. Neuropsychiatric aspects of HIV infection. J Neurol Neurosurg Psychiatry 1995; 58:399 - 402; http://dx.doi.org/10.1136/jnnp.58.4.399; PMID: 7738542
- Tremblay ME, Riad M, Majewska A. Preparation of mouse brain tissue for immunoelectron microscopy. J Vis Exp 2010; http://dx.doi.org/10.3791/2021; PMID: 20689505
- Sedgwick JD, Schwender S, Imrich H, Dörries R, Butcher GW, ter Meulen V. Isolation and direct characterization of resident microglial cells from the normal and inflamed central nervous system. Proc Natl Acad Sci U S A 1991; 88:7438 - 42; http://dx.doi.org/10.1073/pnas.88.16.7438; PMID: 1651506
- Zhang GX, Li J, Ventura E, Rostami A. Parenchymal microglia of naïve adult C57BL/6J mice express high levels of B7.1, B7.2, and MHC class II. Exp Mol Pathol 2002; 73:35 - 45; http://dx.doi.org/10.1006/exmp.2002.2441; PMID: 12127052
- Nakanishi H. Microglial functions and proteases. Mol Neurobiol 2003; 27:163 - 76; http://dx.doi.org/10.1385/MN:27:2:163; PMID: 12777686
- Tremblay ME, Majewska AK. A role for microglia in synaptic plasticity?. Commun Integr Biol 2011; 4:220 - 2; http://dx.doi.org/10.4161/cib.4.2.14506; PMID: 21655446
- Tremblay ME. The role of microglia at synapses in the healthy CNS: novel insights from recent imaging studies. Neuron Glia Biol 2011; 7:67 - 76; http://dx.doi.org/10.1017/S1740925X12000038; PMID: 22418067
- Mattila PK, Lappalainen P. Filopodia: molecular architecture and cellular functions. Nat Rev Mol Cell Biol 2008; 9:446 - 54; http://dx.doi.org/10.1038/nrm2406; PMID: 18464790
- Morgan JT, Chana G, Pardo CA, Achim C, Semendeferi K, Buckwalter J, Courchesne E, Everall IP. Microglial activation and increased microglial density observed in the dorsolateral prefrontal cortex in autism. Biol Psychiatry 2010; 68:368 - 76; http://dx.doi.org/10.1016/j.biopsych.2010.05.024; PMID: 20674603
- Trapp BD, Wujek JR, Criste GA, Jalabi W, Yin X, Kidd GJ, Stohlman S, Ransohoff R. Evidence for synaptic stripping by cortical microglia. Glia 2007; 55:360 - 8; http://dx.doi.org/10.1002/glia.20462; PMID: 17136771
- Blinzinger K, Kreutzberg G. Displacement of synaptic terminals from regenerating motoneurons by microglial cells. Z Zellforsch Mikrosk Anat 1968; 85:145 - 57; http://dx.doi.org/10.1007/BF00325030; PMID: 5706753
- Kress H, Stelzer EH, Holzer D, Buss F, Griffiths G, Rohrbach A. Filopodia act as phagocytic tentacles and pull with discrete steps and a load-dependent velocity. Proc Natl Acad Sci U S A 2007; 104:11633 - 8; http://dx.doi.org/10.1073/pnas.0702449104; PMID: 17620618
- Nimmerjahn A, Kirchhoff F, Helmchen F. Resting microglial cells are highly dynamic surveillants of brain parenchyma in vivo. Science 2005; 308:1314 - 8; http://dx.doi.org/10.1126/science.1110647; PMID: 15831717