Abstract
The F-BAR domain is emerging as an important player in membrane remodeling pathways. F-BAR domain proteins couple membrane remodeling with actin dynamics associated with endocytic pathways and filopodium formation. Here, we provide a comprehensive analysis of F-BAR domain proteins in terms of their evolutionary relationships and protein function. F-BAR domain containing proteins can be categorized into five subfamilies based on their phylogeny which is consistent with the additional protein domains they possess, for example, RhoGAP domains, Cdc42 binding sites, SH3 domains, and tyrosine kinase domains. We derive a protein-protein interaction network suggesting that dynamin1/2, N-WASP, Huntingtin, intersectin and Cdc42 are central nodes influencing F-BAR domain protein function.
Introduction
All eukaryotic cells are surrounded by a plasma membrane, and they also contain multiple membrane-based organelles and structures inside cells. Thus membrane remodeling is likely to be important for most cellular activities and development. Recently, the Bin-Amphiphysin-Rvs (BAR) domain superfamily of proteins have been found to play a major role in remodeling cellular membranes linked with organelle biogenesis, membrane trafficking, cell division, cell morphology and cell migration.Citation1 The BAR domain superfamily of proteins is evolutionarily conserved with representative members present from yeast to man.
BAR Domains
Currently there are three distinct families of BAR domain proteins: classical BAR, F-BAR (FCH-BAR e.g., Fes/CIP4 homology BAR e.g., Toca-1) and I-BAR (inverse-BAR e.g., IRSp53). Structural studies have revealed common elements of the various BAR domains which include dimerisation modules that possess curvature and positively charged surfaces.Citation1 Two basic residues in the BAR domain interact with the negatively charged phospholipids in the membrane. BAR domain dimers form a banana-shaped structure, with each dimer possessing a distinct curvature. BAR domains can deform membranes in vitro2 but may also detect curvature in membranes. The classical BAR domain family includes N-BAR and other additional lipid-binding domains. The N-BAR domain is distinguished from BAR domains by an amphipathic helix in front of the classical BAR domain, which enhances its ability to bind liposomes with no effect on tubulation. 2 BAR domains are sometimes coupled with phosphoinositide binding motifs, phox (PX) or PH domains, which enable these BAR proteins to associate specifically with certain types of membranes.Citation1
The I-BAR domain is “cigar shaped” and exists as a six-helix bundle dimer. Positively charged amino acids are located at the end of the I-BAR domainCitation3–Citation6 and are the binding sites for the membrane and actin. The I-BAR domain binds to phosphatidylinositol-rich membranes and has membrane tubulation activity.Citation4–Citation7 Saarikangas et al.Citation8 showed that clustering of PIP2 occurred after I-BAR binding. The membrane was deformed by electrostatic interactions and the I-BAR domain was still dynamically associated with the inner side of the deformed membrane tubules.Citation8 I-BAR domains bind to lipid bilayers through a convex surface and promote the formation of outward protrusions of membranes.Citation4 The I-BAR domain containing protein IRSp53 induces filopodia by coupling membrane protrusion and actin dynamics.Citation9
The fission yeast ‘Pombe Cdc15 homology’ (PCH) family proteins are adaptor proteins involved in cytokinesis and actin dynamics.Citation10 PCH family members are expressed in diverse eukaryotic species with limited sequence similarity, but they do have similar domain structures—an N-terminal Fes/CIP4 homology (FCH) domain, followed by a coiled coil (CC) region and one or more SH3 domains at the C-terminal.Citation10,Citation11
The F-BAR domain, also known as the extended FCH domain (EFC) in PCH family proteins includes the FCH domain and CC region and is only weakly similar to the BAR domain.Citation12,Citation13 By sequence alignment, structural, biochemical and cell biological studies, it has been shown that the EFC is actually an extended BAR domain, and that F-BAR domains share similar properties with BAR domains. The F-BAR domain plays a role in dimerization and membrane phospholipid binding.Citation13 Three pairs of basic residues are conserved in the F-BAR domain, which bind lipids and mediate membrane tubulation activity.Citation13 The F-BAR domain binds specifically to certain kinds of lipids. This is in contrast to the BAR domain, which does not have such lipid preferences. The F-BAR domain has high affinity for PIP2,Citation13,Citation14 moderate affinity for PIP3 and phosphatidylserine,Citation13 and does not bind lysophosphatidic acid, lysophosphocholine or sphingosine-1-phosphate.Citation13 The F-BAR domain tubulates the membrane in vitro and in vivo.Citation12,Citation13 The F-BAR domain induces a gently curved helical-bundle dimer with a length ∼200 ∼600 Å tubulated membrane.Citation15 End to end oligomerisation of the F-BAR domain of formin binding protein 17 (FBP17) and thyroid hormone receptor interactor 10 (TRIP10) form filaments that can induce cell membrane invagination.Citation15,Citation16
F-BAR Domain Protein Families
To provide a comprehensive overview of the repertoire of F-BAR domain proteins and to study their evolutionary relationship, we first had to create a proper F-BAR domain alignment since the existing FCH domain model in PFAM covered only the N-terminal part (about one third) of the F-BAR globular domain, as seen in the superimposition of crystal structures of three different F-BAR proteins. Using this structural alignment as seed, we created a new alignment of the whole F-BAR domain (see Suppl. Material for details) and used a derived hidden Markov model to identify a total of 1287 F-BAR domain-containing proteins in the UniRef100 database. Finally, focusing on nine representative species, we carried out a phylogenetic analysis of 305 F-BAR domain proteins (). The phylogenetic subgrouping in the resulting tree clusters proteins with similar domain composition and architecture together which indicates robustness of the analysis as the tree structure itself is based solely on the sequence similarity distances within the F-BAR domain regions. From this analysis, five subfamilies of F-BAR domain proteins can be distinguished. Subfamily 1, the Toca family (FBP17, TRIP10 or CIP4, and Toca-1 or FBP1). Both family 1 and 4 are involved in endocytosis. The Toca family are characterized by the presence of a Cdc42 binding site (homology region 1 (HR1) domain) which is absent in the Pacsin family. Subfamily 2 is the Fps/Fes and Fer subfamily of non-receptor protein tyrosine kinases, including Fps (Fujinami poultry sarcoma) and Fes (feline sarcoma), which are oncogenes identified from tumorforming retroviruses that encode non-receptor tyrosine kinases, and FCHSD1/2. Subfamily 3 contains the Rho GTPase activating protein (RhoGAP) domain, which regulates the functions of these Rho GTPases and includes SLIT-ROBO Rho GTPase activating protein (SrGAP) 1-3. Subfamily 4 includes syndapins/ pacsins and members of this subfamily have a domain structure and function similar to those in Subfamily 5 consisting of growth arrest-specific protein (GAS7), proline-serine-threonine phosphatase-interacting protein 1 (PSTPIP1), cell division cycle 15 (CDC15), FCH domain only 2 (FCHO2) and Nostrin.
F-BAR Domain Protein Function
srGAP1-3.
srGAP1-3 contain an F-BAR domain at the N-terminal, a RhoGAP domain in the middle and an SH3 domain at the C-terminal. WAVE associated Rac GTPase activating protein (WRP) or SrGAP1 was first identified by Soderling et al.Citation17 as a scaffolding protein that inhibited Rac function with a potential link to mental retardation.Citation18 Guerrier et al.Citation19 showed that srGAP2-induced filopodium formation was dependent on its F-BAR domain and that this activity was associated with neurite outgrowth and branching. Furthermore, srGAP2 inhibited neuronal migration. Thus srGAP2 contributes to neuronal morphogenesis. These data suggest that there are functional similarities between the F-BAR domain and I-BAR domain in that both can generate membrane protrusions. Thus F-BAR domains have diverse structure and membrane remodeling activities.
Fes/Fer.
The Fps/Fes and Fer proteins are a distinct family of non-receptor tyrosine kinases with prominent roles in inflammation and immunity (reviewed in ref. Citation11). Fer localises to microtubule ends and can phosphorylate the adhesion molecule platelet/endothelial cell adhesion molecule 1 (PECAM-1).Citation20 Fer has also been linked to actin dynamics through cortactin and the phosholipase D-phosphatidic acid (PLD-PA) pathway.Citation21,Citation22 Interestingly, Fer is implicated in growth cone collapse downstream of semaphorin 3A in dorsal root ganglion neuronsCitation23 and in dorsal closure in DrosophilaCitation24 by influencing the stability of adherens junctions.
Syndapins/Pacsins.
Syndapins (synaptic dynamin-associated proteins), also known as pacsins, are a group of F-BAR domain proteins that have been studied in detail.Citation25 Syndapins have three isoforms and they are expressed in a tissue-specific manner and have been implicated in the regulation of the endocytic events.Citation26–Citation31 Syndapins contain an N-terminal F-BAR domain and a C-terminal SH3 domain. There are no Cdc42 binding sites in syndapins. Overexpression of the SH3 domain of syndapins inhibits receptor-mediated endocytosis, while overexpression of the full-length protein generates microspikes and lamellipodia-like structures. It would be interesting to examine the dynamics of the microspikes generated by syndapins to see if these structures are filopodia, as has been found to be the case for IRSp53.Citation32 Syndapins can oligomerise through their N-terminal CC region.Citation28,Citation32 This oligomerisation is thought to increase the concentration of the SH3 domain for the recruitment of N-WASP and dynamin. Syndapins bind to synaptojanin and sos, which are important proteins in endocytosis.Citation25 Other functions of syndapins include regulation of the membrane trafficking events at the trans-Golgi networkCitation32 and endosomal recycling.Citation30 Recently, the function of syndapin in the formation of a postsynaptic membrane system in Drosophila has been documented.Citation33 The structural study by Wang et al.Citation34 revealed that the specific characteristics of the F-BAR domain of syndapins contributed to its ability to generate small tubules and tubule constriction, in contrast to the wide tubules generated by other F-BAR domains. In additional, full length syndapin lacks membrane tubulation activity and thus probably exists in an autoinhibited state.
Toca-1 (FBP1), -2 (FBP17) and -3 (TRIP10/CIP4)
A subset of F-BAR domain proteins including Toca-1, FBP17, TRIP10/CIP4 (Toca-1-3) contain an F-BAR domain at the N-terminal, an HR1 domain (Cdc42 binding site) in the middle and a C-terminal SH3 domain. Toca-1 was identified as an essential component of Cdc42-mediated actin polymerisationCitation35 and it directly or indirectly activates N-WASP. Using an in vitro system, Takano et al.Citation36 investigated the mechanism of Toca-1/FBP17-mediated actin polymerisation. They showed that Toca-1/FBP17 recruited N-WASP-WASP interacting protein (WIP) to the membrane, and the membrane curvature generated by Toca-1/FBP17 activated N-WASP-WIP-mediated actin polymerisation.Citation36 F-BAR domain proteins bind to lipids and deform the membrane through the F-BAR domain.Citation12,Citation13 The membrane deformation activity of the F-BAR domain is enhanced by disruption of the actin cytoskeleton.Citation12,Citation13 These proteins can also induce actin bundling.Citation37 Overexpression and RNAi knockdown of FBP17 and CIP4 affect endocytosis.Citation12,Citation13 Toca-1, FBP17 and CIP4 possess Cdc42 binding sites as well as an SH3 domain that binds N-WASP. These three proteins can regulate the activation of N-WASP.Citation12,Citation13,Citation35,Citation38 Disruption of the actin cytoskeleton enhances F-BAR domain-induced tubular membrane invaginations.Citation12 The SH3 domain of FBP17 and CIP4 binds to dynamin, which antagonizes the tubulation activity of FBP17 and CIP4.Citation12 RNAi results suggest that there is functional redundancy between Toca-1, FBP17 and CIP4.Citation12 Recently, Fricke et al.Citation39 investigated the in vivo function of CIP4/Toca-1 in Drosophila and found that CIP4/Toca-1 acted downstream of Cdc42 to control wing hair formation through WASP/SCAR/WAVE. In C. elegans, Toca-1 and Toca-2 were found to regulate oocyte growth and embryonic epidermal morphology through regulating clathrin-mediated endocytosis.Citation40 Toca-1 has been shown to induce filopodia and neurite formation when in a complex with N-WASP.Citation41 The Toca-1/N-WASP complex is involved in endocytosisCitation41 and CdcCitation42 is implicated in regulation of the formation of this complex (Bu W and Ahmed S, unpublished data). This data suggests that the Toca family coordinates the membrane-cytoskeleton events associated with endocytosis and that this is linked to filopodium formation. The physiological role of linking endocytosis and filopodium formation may be to facilitate the coordination of membrane trafficking and morphology pathways.Citation41
GAS7, PSTPIP1 and FCHO1.
GAS7 has been shown to play a role in neuronal development.Citation42 GAS7 can induce cell protrusions resembling early neurites in cell lines such as PC12.Citation43,Citation44 This neurite outgrowth activity of GAS7 is linked to its ability to cross-link actin and modulate actin dynamics.Citation45 PSTPIP1 was first identified as a tyrosine phosphorylated protein associated with F-actin.Citation46 PSTPIP1 interacts with WASP via its SH3 domain and a tyrosine phosphatase through its CC region.Citation47 More recently, PSTPIP has been linked to filopodium formation through its putative F-actin bundling activity.Citation37 The role of its F-BAR domain in filopodium formation has yet to be investigated. In contrast to GAS7 and PSTPIP1/2, FCHO1 seems to be involved in clathrin coated vesicle formation and endocytosis.Citation48
F-BAR Domain Subfamilies Protein-Protein Interaction Map
To generate an overview of how the five F-BAR domain protein subfamilies may relate to one another functionally we carried out an analysis of their protein-protein interactions using Ingenuity pathway analysis software (). From this analysis, the following central nodes emerge: (1) Dnm1/2 (dynamin1 and 2), WASL (N-WASP), ITSN1 (intersectin) and HTT (Huntingtin) represent important links for F-BAR domain proteins, (2) Cdc42 may affect F-BAR domain subfamily function indirectly via the Toca family, (3) YWHAZ (14-3-3 family member zeta) may play a role in masking the activity of srGAP1/2 and FCHSD1 and (4) trafficking of the EGFR is a potential target for these proteins.
Conclusion
The F-BAR domain proteins couple membrane remodeling and actin dynamics associated with endocytic pathways and filopodium formation. Further analysis of the F-BAR domain subfamilies and their interacting partners is likely to give us mechanistic insight into membrane trafficking and morphology pathways.
Abbreviations
BAR | = | Bin-Amphiphysin-Rvs |
F-BAR | = | Fes/CIP4 homology-BAR |
I-BAR | = | inverse-BAR |
PX | = | phox |
CC | = | coiled coil |
PCH | = | Pombe Cdc15 homology |
EFC | = | extended FCH |
RhoGAPs | = | Rho GTPase activating proteins |
Fps | = | Fujinami poultry sarcoma |
Fes | = | feline sarcoma |
WRP | = | WAVE associated Rac GTPase activating protein |
Syndapins | = | synaptic dynamin-associated proteins |
PIP2 | = | phosphatidylinositol 4,5-bisphosphate |
PIP3 | = | phosphatidylinositol 3,4,5-trisphosphate |
Figures and Tables
Figure 1 Evolutionary relationships of F-BAR domain-containing proteins (tree generated with MEGA4,Citation49). Structural alignment of three available crystal structures of FBP17, CIP4 and FCHO2 (PDBs: 2EFL, 2EFK and 2V0O) was created using MUSTANGCitation50 implemented in YASARA.Citation51 The resulting structure-based alignment was used as seed to align orthologues, retrieved from OMA,Citation52 and each of the three seed proteins (seed-driven alignment was generated by MAFFT using EINSI settings for multidomain proteins).Citation53 Fragmentary sequences were removed and the resulting alignment was trimmed at the conserved borders of the structural seed alignment in JalviewCitation54 and using Cd-hit,Citation55 and overall redundancy was removed by allowing a maximal sequence identity of 90%. This alignment was converted to HMM, calibrated (seed = 0) and searched with HMMerCitation56 against UniRef100,Citation57 (Release 15.9 which consists of 10,011,983 entries). The resulting 744 hits with E-values below 0.01 were then added to the alignment (as before with seed and borders from structural alignment and redundancy removal). This alignment was then used after conversion to HMM for a second iteration of the search against UniRef100. This HMMer search returned 1287 proteins with E-values below 0.01. For clarity of the representation of phylogenetic relationships we selected only proteins from nine representative species (Saccharomyces cerevisiae, Schizosaccharomyces pombe, Caenorhabditis elegans, Drosophila melanogaster, Xenopus tropicalis, Gallus gallus, Danio rerio, Mus musculus and Homo sapiens) which reduced the 1287 proteins to 305 for further analysis. Next, we generated a neighbour joining treeCitation58 based only on the curated alignment of the F-BAR domain regions with pairwise gap deletion, JTT as distance measure and 200 bootstrapCitation59 replicates (generated within MEGA4,Citation49). Sequence and domain analysis of the full length proteins was carried out using the ANNIE resourceCitation60 and CD-Search.Citation61 Domain architecture images were created using the MyDomains image creator (http://www.expasy.ch/tools/mydomains/). Paralogous relationships were inferred for two proteins from the same organism by using T-BLASTN against the organism’s genome and confirming non-overlapping genomic/chromosomal location as mapped with the Map Viewer tool from NCBI.Citation62,Citation63 Additional files that are provided as supplementary material include the sequence accession numbers and names of the 305 F-BAR proteins used for the phylogenetic tree, as well as the key to the gene names used in the interaction network. Alignments and HMMs are available on request from the authors.
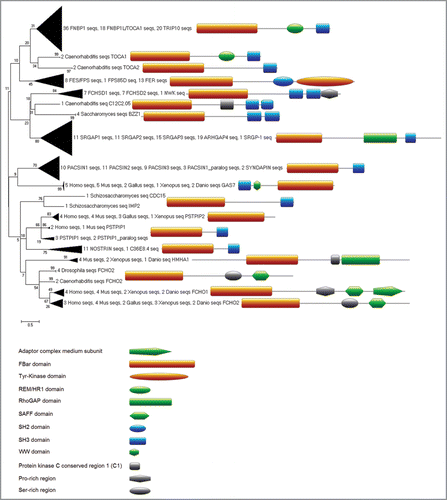
Figure 2 An interaction network between several F-BAR domain-containing proteins generated using Ingenuity Pathway Analysis (Ingenuity® Systems, www.ingenuity.com). Genes or gene products are represented as nodes, and the biological relationship between two nodes is represented as a line. All lines are supported by at least one reference from the literature, from a textbook, or from canonical information stored in the Ingenuity Pathways Knowledge Base. Human, mouse and rat orthologues of a gene are stored as separate objects in the Ingenuity Pathways Knowledge Base, but are represented as a single node in the network. The F-BAR domain-containing proteins are colored according to their categorization into one of five subfamilies. Subfamilies (colour): 1, pink; 2, olive; 3, red; 4, blue; 5, green, see text for details. Network Generation. A data set containing identifiers for the F-BAR domain-containing proteins was uploaded into Ingenuity Pathway Analysis (Ingenuity Systems, www.ingenuity.com). The identifiers were mapped to their corresponding gene objects in the Ingenuity Pathways Knowledge Base. Networks of these genes were then algorithmically generated based on their connectivity. All five generated networks were merged and the resulting network was grown to form a larger network, using the all molecules option. Molecules that do not directly interact with F-BAR domain-containing proteins were removed. From the 20 F-BAR domain-containing genes that were found in the merged networks, PSTPIP2, FCHO2, FCHO1 and ARHGAP4 were removed as they were linked to the rest of the network by only a single line via genes that are non-specifically connected to several hundreds of other genes.
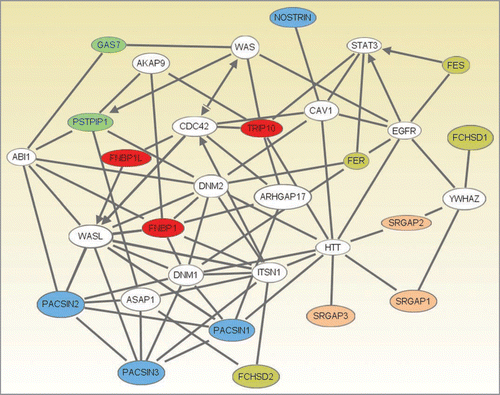
Additional material
Download Zip (87 KB)References
- Frost A, Unger VM, de CP. The BAR domain superfamily: membrane-molding macromolecules. Cell 2009; 137:191 - 196
- Peter BJ, Kent HM, Mills IG, Vallis Y, Butler PJ, Evans PR, et al. BAR domains as sensors of membrane curvature: the amphiphysin BAR structure. Science 2004; 303:495 - 499
- Lee SH, Kerff F, Chereau D, Ferron F, Klug A, Dominguez R. Structural basis for the actin-binding function of missing-in-metastasis. Structure 2007; 15:145 - 155
- Mattila PK, Pykalainen A, Saarikangas J, Paavilainen VO, Vihinen H, Jokitalo E, et al. Missing-inmetastasis and IRSp53 deform PI(4,5)P2-rich membranes by an inverse BAR domain-like mechanism. JCell Biol 2007; 176:953 - 964
- Millard TH, Bompard G, Heung MY, Dafforn TR, Scott DJ, Machesky LM, et al. Structural basis of filopodia formation induced by the IRSp53/MIM homology domain of human IRSp53. EMBO J 2005; 24:240 - 250
- Suetsugu S, Murayama K, Sakamoto A, Hanawa-Suetsugu K, Seto A, Oikawa T, et al. The RAC binding domain/IRSp53-MIM homology domain of IRSp53 induces RAC-dependent membrane deformation. J Biol Chem 2006; 281:35347 - 35358
- Yamagishi A, Masuda M, Ohki T, Onishi H, Mochizuki N. A novel actin bundling/filopodium-forming domain conserved in insulin receptor tyrosine kinase substrate p53 and missing in metastasis protein. J Biol Chem 2004; 279:14929 - 14936
- Saarikangas J, Zhao H, Pykalainen A, Laurinmaki P, Mattila PK, Kinnunen PK, et al. Molecular mechanisms of membrane deformation by I-BAR domain proteins. Curr Biol 2009; 19:95 - 107
- Lim KB, Bu W, Goh WI, Koh E, Ong SH, Pawson T, et al. The Cdc42 effector IRSp53 generates filopodia by coupling membrane protrusion with actin dynamics. J Biol Chem 2008; 283:20454 - 20472
- Lippincott J, Li R. Involvement of PCH family proteins in cytokinesis and actin distribution. MicroscRes Tech 2000; 49:168 - 172
- Greer P. Closing in on the biological functions of Fps/Fes and Fer. Nat Rev Mol Cell Biol 2002; 3:278 - 289
- Itoh T, Erdmann KS, Roux A, Habermann B, Werner H, De Camilli P. Dynamin and the actin cytoskeleton cooperatively regulate plasma membrane invagination by BAR and F-BAR proteins. Dev Cell 2005; 9:791 - 804
- Tsujita K, Suetsugu S, Sasaki N, Furutani M, Oikawa T, Takenawa T. Coordination between the actin cytoskeleton and membrane deformation by a novel membrane tubulation domain of PCH proteins is involved in endocytosis. J Cell Biol 2006; 172:269 - 279
- Kovar DR. Molecular details of formin-mediated actin assembly. Curr Opin Cell Biol 2006; 18:11 - 17
- Shimada A, Niwa H, Tsujita K, Suetsugu S, Nitta K, Hanawa-Suetsugu K, et al. Curved EFC/F-BARdomain dimers are joined end to end into a filament for membrane invagination in endocytosis. Cell 2007; 129:761 - 772
- Frost A, Perera R, Roux A, Spasov K, Destaing O, Egelman EH, et al. Structural basis of membrane invagination by F-BAR domains. Cell 2008; 132:807 - 817
- Soderling SH, Binns KL, Wayman GA, Davee SM, Ong SH, Pawson T, et al. The WRP component of the WAVE-1 complex attenuates Rac-mediated signalling. Nat Cell Biol 2002; 4:970 - 975
- Soderling SH, Guire ES, Kaech S, White J, Zhang F, Schutz K, et al. A WAVE-1 and WRP signaling complex regulates spine density, synaptic plasticity and memory. J Neurosci 2007; 27:355 - 365
- Guerrier S, Coutinho-Budd J, Sassa T, Gresset A, Jordan NV, Chen K, et al. The F-BAR domain of srGAP2 induces membrane protrusions required for neuronal migration and morphogenesis. Cell 2009; 138:990 - 1004
- Kogata N, Masuda M, Kamioka Y, Yamagishi A, Endo A, Okada M, et al. Identification of Fer tyrosine kinase localized on microtubules as a platelet endothelial cell adhesion molecule-1 phosphorylating kinase in vascular endothelial cells. Mol Biol Cell 2003; 14:3553 - 3564
- Fan L, Di Ciano-Oliveira C, Weed SA, Craig AW, Greer PA, Rotstein OD, et al. Actin depolymerization-induced tyrosine phosphorylation of cortactin: the role of Fer kinase. Biochem J 2004; 380:581 - 591
- Itoh T, Hasegawa J, Tsujita K, Kanaho Y, Takenawa T. The tyrosine kinase Fer is a downstream target of the PLD-PA pathway that regulates cell migration. Sci Signal 2009; 2:52
- Shapovalova Z, Tabunshchyk K, Greer PA. The Fer tyrosine kinase regulates an axon retraction response to Semaphorin 3A in dorsal root ganglion neurons. BMC Dev Biol 2007; 7:133
- Murray MJ, Davidson CM, Hayward NM, Brand AH. The Fes/Fer non-receptor tyrosine kinase cooperates with Src42A to regulate dorsal closure in Drosophila. Development 2006; 133:3063 - 3073
- Kessels MM, Qualmann B. The syndapin protein family: linking membrane trafficking with the cytoskeleton. J Cell Sci 2004; 117:3077 - 3086
- Modregger J, Ritter B, Witter B, Paulsson M, Plomann M. All three PACSIN isoforms bind to endocytic proteins and inhibit endocytosis. J Cell Sci 2000; 113:4511 - 4521
- Anggono V, Smillie KJ, Graham ME, Valova VA, Cousin MA, Robinson PJ. Syndapin I is the phosphorylation-regulated dynamin I partner in synaptic vesicle endocytosis. Nat Neurosci 2006; 9:752 - 760
- Kessels MM, Qualmann B. Syndapin oligomers interconnect the machineries for endocytic vesicle formation and actin polymerization. J Biol Chem 2006; 281:13285 - 13299
- Kessels MM, Dong J, Leibig W, Westermann P, Qualmann B. Complexes of syndapin II with dynamin II promote vesicle formation at the trans-Golgi network. J Cell Sci 2006; 119:1504 - 1516
- Braun A, Pinyol R, Dahlhaus R, Koch D, Fonarev P, Grant BD, et al. EHD proteins associate with syndapin I and II and such interactions play a crucial role in endosomal recycling. Mol Biol Cell 2005; 16:3642 - 3658
- Kessels MM, Qualmann B. Syndapins integrate N-WASP in receptor-mediated endocytosis. EMBO J 2002; 21:6083 - 6094
- Qualmann B, Kelly RB. Syndapin isoforms participate in receptor-mediated endocytosis and actin organization. J Cell Biol 2000; 148:1047 - 1062
- Kumar V, Fricke R, Bhar D, Reddy-Alla S, Krishnan KS, Bogdan S, et al. Syndapin promotes formation of a postsynaptic membrane system in Drosophila. Mol Biol Cell 2009; 20:2254 - 2264
- Wang Q, Navarro MV, Peng G, Molinelli E, Lin GS, Judson BL, et al. Molecular mechanism of membrane constriction and tubulation mediated by the F-BAR protein Pacsin/Syndapin. Proc Natl Acad Sci USA 2009; 106:12700 - 12705
- Ho HY, Rohatgi R, Lebensohn AM, Le M, Li J, Gygi SP, et al. Toca-1 mediates Cdc42-dependent actin nucleation by activating the N-WASP-WIP complex. Cell 2004; 118:203 - 216
- Takano K, Toyooka K, Suetsugu S. EFC/F-BAR proteins and the N-WASP-WIP complex induce membrane curvature-dependent actin polymerization. EMBO J 2008;
- Chitu V, Pixley FJ, Macaluso F, Larson DR, Condeelis J, Yeung YG, et al. The PCH family member MAYP/ PSTPIP2 directly regulates F-actin bundling and enhances filopodia formation and motility in macrophages. Mol Biol Cell 2005; 16:2947 - 2959
- Tian L, Nelson DL, Stewart DM. Cdc42-interacting protein 4 mediates binding of the Wiskott-Aldrich syndrome protein to microtubules. J Biol Chem 2000; 275:7854 - 7861
- Fricke R, Gohl C, Dharmalingam E, Grevelhorster A, Zahedi B, et al. Drosophila Cip4/Toca-1 Integrates Membrane Trafficking and Actin Dynamics through WASP and SCAR/WAVE. Curr Biol 2009; 19:1429 - 1437
- Giuliani C, Troglio F, Bai Z, Patel FB, Zucconi A, Malabarba MG, et al. Requirements for F-BAR proteins TOCA-1 and TOCA-2 in actin dynamics and membrane trafficking during Caenorhabditis elegans oocyte growth and embryonic epidermal morphogenesis. PLoS Genet 2009; 5:1000675
- Bu W, Chou AM, Lim KB, Sudhaharan T, Ahmed S. The Toca-1-N-WASP complex links filopodial formation to endocytosis. J Biol Chem 2009; 284:11622 - 11636
- Ju YT, Chang AC, She BR, Tsaur ML, Hwang HM, Chao CC, et al. gas7: A gene expressed preferentially in growth-arrested fibroblasts and terminally differentiated Purkinje neurons affects neurite formation. Proc Natl Acad Sci USA 1998; 95:11423 - 11428
- Lortie K, Huang D, Chakravarthy B, Comas T, Hou ST, Lin-Chao S, et al. The gas7 protein potentiates NGF-mediated differentiation of PC12 cells. Brain Res 2005; 1036:27 - 34
- Chao CC, Chang PY, Lu HH. Human Gas7 isoforms homologous to mouse transcripts differentially induce neurite outgrowth. J Neurosci Res 2005; 81:153 - 162
- She BR, Liou GG, Lin-Chao S. Association of the growth-arrest-specific protein Gas7 with F-actin induces reorganization of microfilaments and promotes membrane outgrowth. Exp Cell Res 2002; 273:34 - 44
- Yeung YG, Soldera S, Stanley ER. A novel macrophage actin-associated protein (MAYP) is tyrosinephosphorylated following colony stimulating factor-1 stimulation. J Biol Chem 1998; 273:30638 - 30642
- Wu Y, Spencer SD, Lasky LA. Tyrosine phosphorylation regulates the SH3-mediated binding of the Wiskott-Aldrich syndrome protein to PSTPIP, a cytoskeletal-associated protein. J Biol Chem 1998; 273:5765 - 5770
- Sakaushi S, Inoue K, Zushi H, Senda-Murata K, Fukada T, Oka S, et al. Dynamic behavior of FCHO1 revealed by live-cell imaging microscopy: its possible involvement in clathrin-coated vesicle formation. Biosci Biotechnol Biochem 2007; 71:1764 - 1768
- Tamura K, Dudley J, Nei M, Kumar S. MEGA4: Molecular Evolutionary Genetics Analysis (MEGA) software version 4.0. Mol Biol Evol 2007; 24:1596 - 1599
- Konagurthu AS, Whisstock JC, Stuckey PJ, Lesk AM. MUSTANG: a multiple structural alignment algorithm. Proteins 2006; 64:559 - 574
- Krieger E, Koraimann G, Vriend G. Increasing the precision of comparative models with YASARA NOVA—a self-parameterizing force field. Proteins 2002; 47:393 - 402
- Schneider A, Dessimoz C, Gonnet GH. OMA Browser—exploring orthologous relations across 352 complete genomes. Bioinformatics 2007; 23:2180 - 2182
- Katoh K, Kuma K, Toh H, Miyata T. MAFFT version 5: improvement in accuracy of multiple sequence alignment. Nucleic Acids Res 2005; 33:511 - 518
- Waterhouse AM, Procter JB, Martin DM, Clamp M, Barton GJ. Jalview Version 2—a multiple sequence alignment editor and analysis workbench. Bioinformatics 2009; 25:1189 - 1191
- Li W, Godzik A. Cd-hit: a fast program for clustering and comparing large sets of protein or nucleotide sequences. Bioinformatics 2006; 22:1658 - 1659
- Eddy SR. Profile hidden Markov models. Bioinformatics 1998; 14:755 - 763
- The universal protein resource (UniProt). Nucleic Acids Res 2008; 36:190 - 195
- Saitou N, Nei M. The neighbor-joining method: a new method for reconstructing phylogenetic trees. Mol Biol Evol 1987; 4:406 - 425
- Felsenstein J. Confidence limits on phylogenies: an approach using the bootstrap. Evolution 1985; 783 - 791
- Ooi HS, Kwo CY, Wildpaner M, Sirota FL, Eisenhaber B, Maurer-Stroh S, et al. ANNIE: integrated de novo protein sequence annotation. Nucleic Acids Res 2009; 37:435 - 440
- Marchler-Bauer A, Bryant SH. CD-Search: protein domain annotations on the fly. Nucleic Acids Res 2004; 32:327 - 331
- Altschul SF, Gish W, Miller W, Myers EW, Lipman DJ. Basic local alignment search tool. J Mol Biol 1990; 215:403 - 410
- Wheeler DL, Barrett T, Benson DA, Bryant SH, Canese K, Chetvernin V, et al. Database resources of the National Center for Biotechnology Information. Nucleic Acids Res 2006; 34:173 - 180