Abstract
Recent work on G-protein coupled receptors (GPCRs) has highlighted the importance of homo- and heterodimerization in all areas of GPCR function, including trafficking, signaling and desensitization. Novel GPCR dimers and even high-order oligomers are constantly being discovered. Advances in techniques such as fluorescent microscopy have improved our ability to detect these interactions. As GPCRs represent the largest class of transmembrane signaling molecules in biology, these new insights into their function could vastly improve our understanding of the complex physiological role GPCRs play in cellular signaling. Utilizing a combination of classic biochemical approaches and newer techniques such as fluorescence resonance energy transfer (FRET) and total internal reflection fluorescence microscopy (TIRF), we recently demonstrated a novel interaction between M2 muscarinic receptors and GABAB receptors. In this addendum, we address technical aspects of combining FRET and TIRF to study GPCR interactions and further discuss the physiological implications of the M2-GABAB heterodimer.
G-protein coupled receptors (GPCRs) represent the largest, most diverse class of transmembrane signaling molecules in biology, and are a major drug discovery target. All GPCRs share a similar structure, consisting of seven transmembrane domains with an extracellular amino terminus and an intracellular carboxy terminus. Within this general framework, however, there are large variations in both form and function. Originally thought to function only as monomers, recent work suggests that GPCRs can also function as dimers or larger oligomers. Yet despite over a decade of research into GPCR dimerization, these interactions remain increasingly complex and any simple rules governing them elusive. Do all GPCRs function as dimers, or even higher-order oligomers? What factors determine the specificity or promiscuity of these interactions? And how do these interactions affect trafficking, ligand binding or G-protein signaling?
The classical example of GPCR dimerization is the GABAB receptor, which has a functional requirement for dimerization.Citation1,Citation2 The GABAB1 subunit contains the ligand binding site, while the GABAB2 subunit contains the G-protein signaling site and allows forward trafficking of the heterodimer.Citation1,Citation2 The GABAB receptor has also been shown to bind to an extracellular calcium sensing receptor, altering trafficking.Citation3 This suggests a certain level of promiscuity in binding for GABAB. The M2 muscarinic receptor, on the other hand, has previously been shown to dimerize with itself and certain other muscarinic receptors, but not different classes of GPCRs such as opioid receptors.Citation4,Citation5 M2 receptors undergo agonist-induced endocytosis in PC12 cells, a neuronal-like cell line, due to endogenous release of acetylcholine.Citation6,Citation7 Surprisingly, expression of GABAB receptors can rescue muscarinic signaling in PC12 cells, despite high levels of agonist.Citation8 To investigate the putative interaction between M2 and GABAB receptors, we used a combination of classical approaches (i.e., co-immunoprecipitation, biochemistry) and newer, more novel approaches (fluorescence resonance energy transfer combined with total internal reflection fluorescence microscopy).
FRET & TIRF for Evaluating GPCR Interactions
Classic techniques for detecting proteinprotein interactions often rely on a number of assumptions that may not translate in vivo. Newer fluorescent microscopy techniques have increasingly been used to study protein interactions on a nanometer scale, such as fluorescent resonance energy transfer (FRET). Other techniques, like total internal reflection fluorescence (TIRF) microscopy allow visualization of proteins on or near the plasma membrane. TIRF microscopy alters the angle at which collimated laser light contacts the specimen. At a critical angle, most light will reflect off the coverslip, where the refractive index changes, creating an evanescent wave that penetrates approximately 100 nanometers into the cell.Citation9 Measuring FRET using TIRF microscopy provides a powerful tool for visualizing direct interactions between proteins at the plasma membrane in a live cell.
compares FRET measured under TIRF versus standard epifluorescence microscopy. Any standard FRET techniques can be used with TIRF (acceptor photobleaching, three cube method, etc.). We used the acceptor photobleach method to study the novel interaction of M2 muscarinic receptors and GABAB receptors, each fused to a cyan fluorescent protein (CFP) or yellow fluorescent protein (YFP). CFP and YFP have overlapping spectral properties, such that excitation of CFP results in YFP emission when the two fluorophores are within 100 aligned (i.e., not orthogonal). To measure percentage FRET efficiency (%FRET), the acceptor fluorophore (YFP) is directly photobleached, which dequenches the CFP and reveals a stronger emission for the donor molecule CFP. This technique has the advantage of not needing correction of cross-talk and bleed-through between fluorophores, and also allows direct comparisons between studies by relying solely on changes in donor fluorescence.Citation10 Using YFP or CFP tagged M2 and GABAB receptors, we showed for the first time a direct interaction between M2 and GABAB2 subunits in PC12 and HEK cells.
Technical Issues for FRET Studies Using TIRF Microscopy
We used the acceptor photobleach method to minimize the technical issues involved with other assays, such as bleedthrough, as well as to allow direct comparisons between studies.Citation1 With the acceptor photobleach method, the FRET efficiency (%FRET) is directly calculated by measuring the changes in CFP emission before and after photobleaching. In order to achieve the highest FRET levels with minimal background, there were several technical variables that needed to be addressed. Background levels were measured by expressing a CFP-tagged protein alone (no YFP) and using our photobleaching protocol. We occasionally found, however, that even non-FRET pairs produced background levels slightly higher than CFP alone, likely due to random interactions within the cell as a result of protein overexpression, particularly with HEK cells. In order to minimize background and prevent movement of the fluorophores during the photobleaching period, we found it necessary to ‘fix’ the cells prior to assaying, effectively taking a snapshot at a fixed point in time. Immediately after removal from the incubator, cells were fixed for five minutes in ice-cold methanol, and allowed to return to room temperature in the dark before beginning the assay. This method, effectively dehydrating cells while preventing endocytosis or trafficking, produced less background than a true fixation by protein crosslinking using paraformaldehyde. Another variable was the length of time necessary to photobleach the YFP fluorophore. Because the changes in the donor fluorophore are dependent on the amount of photodestruction of the acceptor, it is crucial to photobleach the YFP as completely as possible without photobleaching CFP. We found that 45–60 seconds of direct YFP photobleaching produced >90% reduction in YFP fluorescence with minimal changes in CFP fluorescence, when expressed alone, over the same length of photobleaching time. To further ensure that the FRET changes seen were true non-random interactions, we plotted the change in CFP emission as a function of YFP emission before photobleaching. Because changes in CFP emissions are dependent on YFP expression, true FRET pairs will show a hyperbolic increase in FRET as function of YFP expression, giving an asymptote at high YFP expression, while random interactions show a linear dependence.Citation11 Lastly, it has been reported that photobleaching YFP can photoconvert YFP to CFP, appearing as an increase in CFP emission.Citation12 Appropriate controls, such as other YFP-tagged proteins/GPCRs, helped minimize this potential problem. Using these methods and controls, we consistently measured %FRET with minimal background.
Combining Approaches to Get a More Complete Picture
Correlating interactions between proteins at the plasma membrane with intracellular signaling assays provides a spatial and functional picture of GPCR interactions. For example, to assay the physiological consequences of the M2-GABAB2 interaction, we used electrophysiological measurements of signaling to G-protein coupled inwardly rectifying channels (GIRKs), a Gβγ-dependent membranedelimited process. To address whether other Gα-dependent, downstream processes were affected, we also assayed changes in intracellular cAMP levels upon muscarinic stimulation. In both experiments, we demonstrated that GABAB2 receptors rescued muscarinic receptors from a downregulated state, leading to muscarinic-dependent activation of GIRK channels or inhibition of cAMP. Thus, GABAB receptors not only physically interact with M2 receptors at the plasma membrane but also cause a change in the functional signaling properties of M2 receptors.
Furthermore, classic biochemical techniques for evaluating protein interactions and mutagenesis of the receptors of interest can provide a more complete picture of where and how such interactions occur. For instance, analysis of overlapping receptor distributions can provide spatial clues as to where these receptors might interact. GABAB2 and M2 are both highly expressed in several brain regions, including cortex, thalamus and hypothalamus. Importantly, we showed that M2 and GABAB2 receptors co-immunoprecipitate from adult mouse brains, suggesting the interaction occurs in vivo8. Lastly, we provided corroborating evidence for the association of GABAB2 and M2 receptors using a combination of in vitro binding assays and mutagenesis combined with our FRET/TIRF assayCitation8. Both chimeric studies and C-terminal deletions implicated the C-terminal domains, which was confirmed by the demonstration of direct binding between the M2 and GABAB2 C-terminal domains in a protein-protein overlay assayCitation8. Taken together, these results demonstrate a novel interaction between M2 and GABAB2 receptors that likely occurs in vivo and alters the signaling properties of M2 muscarinic receptors.
Physiological Consequences of M2 and GABAB2 Receptor Interaction
Dimerization between GPCRs can have widely varied consequences, including ligand-binding specificity, G-protein signaling specificity, desensitization and trafficking.Citation13 M2 muscarinic receptors normally show agonist-induced densensitization in neuronal PC12 cells due to endogenous release of acetylcholine, leading to reduced M2 signaling.Citation6,Citation7 Because co-expression of GABAB2 rescued M2 receptor from this downregulated state, we hypothesized that GABAB2 altered trafficking of M2 receptors. Studies with Brefeldin-A, a fungal metabolite that inhibits insertion of proteins into the plasma membrane,Citation14 supported this idea. Furthermore, disrupting GABAB2 signaling did not affect the rescue of M2 signaling, suggesting a lack of cross-talk between the two receptors. In the GABAB heterodimer, the GABAB2 subunit plays a role in forward trafficking. We hypothesize that GABAB2 plays a similar role with M2 receptor, shuttling it to the plasma membrane despite continuous agonistinduced endocytosis. This chaperoning allows continued muscarinic signaling through M2 despite elevated levels of agonist.
As noted above, M2 and GABAB2 receptors show overlapping distributions in several cortical regions and co-IP from mouse brain. These may represent small interneurons in the cerebral cortex containing M2 receptor, GABA and inhibitory neuropeptides.Citation15 Small changes in cholinergic projections to these regions could affect cognition and working memory.Citation16 Interestingly, muscarinic modulation of GABAergic transmission is impaired in mouse models of Alzheimer’s disease.Citation17 GABAB and M2 receptors are also present on presynaptic terminals in the spinal cord where they control release of glutamate.Citation18 Thus, interactions between M2 and GABAB2 receptors could also have implications for spinally-mediated analgesia. The precise physiological consequences of GABAB2-mediated upregulation of M2 cholinergic signaling remain an exciting area for future discovery.
Figures and Tables
Figure 1 FRET in epifluorescence vs. TIRF. (A) A cell illuminated by direct epifluorescence. Y—YFP flourophore; C—CFP fluorophore. Shaded blue areas represent regions illuminated by laser light. In epifluorescence, fluorophores throughout the whole cell, including intracellular compartments, are illuminated. (B) The same cell illuminated under TIRF, where θ represents the critical angle. Only flourophores within ∼100 nm of the plasma membrane are illuminated.
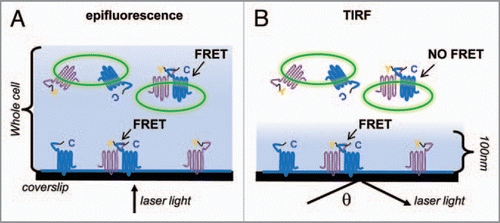
Acknowledgements
We acknowledge funding support from the Chapman Charitable Trust at the Salk Institute (S.B.B.), the National Institute of Neurological Disorders and Stroke (NS37682; P.A.S.) and the National Institute on Drug Abuse (DA019022; P.A.S.). The content is solely the responsibility of the authors and does not necessarily represent the official views of the NIDA, NINDS or the National Institutes of Health.
Addendum to:
References
- Margeta-Mitrovic M, Jan YN, Jan LY. A trafficking checkpoint controls GABA(B) receptor heterodimerization. Neuron 2000; 27:97 - 106
- Robbins MJ, Calver AR, Filippov AK, Hirst WD, Russell RB, Wood MD, et al. GABA(B2) is essential for G-protein coupling of the GABA(B) receptor heterodimer. J Neurosci 2001; 21:8043 - 8052
- Chang W, Tu C, Cheng Z, Rodriguez L, Chen TH, Gassmann M, et al. Complex formation with the Type Bgamma-aminobutyric acid receptor affects the expression and signal transduction of the extracellular calcium-sensing receptor. Studies with HEK-293 cells and neurons. J Biol Chem 2007; 282:25030 - 25040
- van Koppen CJ, Kaiser B. Regulation of muscarinic acetylcholine receptor signaling. Pharmacol Ther 2003; 98:197 - 220
- Wang D, Sun X, Bohn LM, Sadee W. Opioid receptor homo- and heterodimerization in living cells by quantitative bioluminescence resonance energy transfer. Mol Pharmacol 2005; 67:2173 - 2184
- Clancy SM, Boyer SB, Slesinger PA. Coregulation of natively expressed pertussis toxin-sensitive muscarinic receptors with G-protein-activated potassium channels. J Neurosci 2007; 27:6388 - 6399
- Greene LA, Rein G. Synthesis, storage and release of acetylcholine by a noradrenergic pheochromocytoma cell line. Nature 1977; 268:349 - 351
- Boyer SB, Clancy SM, Terunuma M, Revilla-Sanchez R, Thomas SM, Moss SJ, et al. Direct interaction of GABAB receptors with M2 muscarinic receptors enhances muscarinic signaling. J Neurosci 2009; 29:15796 - 15809
- Axelrod D, Thompson NL, Burghardt TP. Total internal inflection fluorescent microscopy. J Microsc 1983; 129:19 - 28
- Vogel SS, Thaler C, Koushik SV. Fanciful FRET. Sci STKE 2006; 331:2
- Kenworthy AK, Edidin M. Distribution of a glycosylphosphatidylinositol-anchored protein at the apical surface of MDCK cells examined at a resolution of <100 energy transfer. J Cell Bio 1998; 142:69 - 84
- Valentin G, Verheggen C, Piolot T, Neel H, Coppey-Moisan M, Bertrand E. Photoconversion of YFP into a CFP-like species during acceptor photobleaching FRET experiments. Nat Methods 2005; 2:801
- Franco R, Casado V, Cortes A, Ferrada C, Mallol J, Woods A, et al. Basic concepts in G-protein-coupled receptor homo- and heterodimerization. Scientific World J 2007; 7:48 - 57
- Chardin P, McCormick F. Brefeldin A: the advantage of being uncompetitive. Cell 1999; 97:153 - 155
- Mufson EJ, Ginsberg SD, Ikonomovic MD, DeKosky ST. Human cholinergic basal forebrain: chemoanatomy and neurologic dysfunction. J Chem Neuroanat 2003; 26:233 - 242
- Youdim MB, Buccafusco JJ. CNS Targets for multifunctional drugs in the treatment of Alzheimer’s and Parkinson’s diseases. J Neural Transm 2005; 112:519 - 537
- Zhong P, Gu Z, Wang X, Jiang H, Feng J, Yan Z. Impaired modulation of GABAergic transmission by muscarinic receptors in a mouse transgenic model of Alzheimer’s disease. J Biol Chem 2003; 278:26888 - 26896
- Chen SR, Pan HL. Activation of muscarinic receptors inhibits spinal dorsal horn projection neurons: role of GABAB receptors. Neuroscience 2004; 125:141 - 148