Abstract
Plastids are the central orchestrators of the early and late responses to wounding and herbivory in plants. This organelle houses some of the most important enzymes involved in the biogenesis of intra and extracellular signals that mediate defense responses against these stresses. Among these enzymes are the ones initiating the biosynthesis of oxylipins (e.g., jasmonic acid (JA) and C6 volatiles), terpenoid volatiles and phenolic compounds, including both volatile (e.g., methyl-salicylate (MeSA)) and non-volatile compounds (e.g., salicylic acid (SA)). Plastids also play a major role in orchestrating changes in primary metabolism during herbivory and thereby in the reallocation of carbon and nitrogen to different functions in response to herbivory. How the primary stress signals generated by mechanical damage and herbivory reach the plastid to activate the rapid synthesis of these signal molecules is at present largely unknown.
Perception of Insect Herbivory and Induction of Plant Defense Responses
Plants have evolved elaborate defense systems to defend against or tolerate insect herbivores and some of these systems are constitutive whereas others are inducible. The induced defense responses are generally classified as direct and indirect. Direct defenses consist in the production of metabolites with are repellent or anti-digestive to the herbivore and those include (among others) glucosinolates, cyanogenic glucosides, alkaloids, phenolics and proteinase inhibitors. Which metabolites are produced depends largely on the plant species. Indirect defense responses include (among others) the production of organic volatiles (e.g., C6 compounds and terpenoids) and extrafloral nectar. These compounds play an important function as attractants of the herbivore’s natural enemies (e.g., predators or parasitoids).Citation1,Citation2 Because the induction of defense responses is energetically costly, plants use sophisticated regulatory mechanisms to perceive and trigger defense responses only when required. Insect herbivory is generally associated with tissue wounding, in particular for foraging insects, and therefore the plant’s responses to wounding overlap with those against herbivores. However, some responses are specific for a particular stimuli.Citation3
One well-defined mechanism by which plants can perceive herbivore feeding is through the perception of components present in the oral secretion (OS) of the insect’s larvae. These factors can enhance some of the wound-induced response whereas others can suppress them. For example, N-linolenoyl-glutamate (18:3-Glu), a fatty acid amino acid conjugate (FAC) found in the OS of several lepidopteran larvae, can (among other responses) heighten the production of jasmonic acid (JA) and ethylene (ET) in Nicotiana attenuata.Citation4 High glucose oxidase (GOX) activity in Spodoptera exigua OS is sufficient to elicit an enhanced SA burst in N. attenuata plants that can attenuate the FAC-mediated production of JA and ET in this plant species.Citation5 Another example is the FAC volicitin [N-(17-hydroxy-linolenoyl)-Gln] originally found in the OS of Spodoptera exigua and which induces the differential production of volatiles in corn (Zea mays) plants.Citation6 Thus, insect-derived compounds can modify the initial wound-associated cellular events resulting in a reprogramming of the wound response that will ultimately have a strong impact on the differential activation of plastidial enzymes. However, not all plant species perceive FACs and therefore this mechanism for insect perception is not universal. For example, when lima beans (Phaseolus lunatus) are mechanically damaged by a robot (MecWorm) that mimics herbivore-caused tissue damage, they emit a volatile organic compound blend which is similar to that induced by herbivore feeding.Citation7 Moreover, the level of leaf damage is linearly correlated with the accumulation of C6 volatiles and monoterpenes.Citation7 Thus, in this case, mechanical damage alone is sufficient to mimic the emission of volatiles induced by insect herbivory. Recently, it has been proposed that plants can perceive ‘damaged-self recognition’, the perception of molecules derived from the plant and modified to indicate disintegrated plant cells.Citation8 One example is the perception of inceptins, digestive fragments of an ATP synthase.Citation9
The Plastid Reacts Rapidly to Wounding and Herbivory by Generating a Broad Range of Different Chemical Signals
In green tissue, induced defense responses against insects and wounding relay largely on chloroplasts, which react almost immediately (within seconds) to these stimuli by the rapid generation of diverse chemical signals targeted to induce these responses both locally and systemically ().
Among the first and most critical chemical signals to be generated by the plastids are the oxylipins. Oxylipins are lipid metabolites derived from the oxidation of unsaturated fatty acids [e.g., linoleic acid (18:2), α-linolenic acid (18:3) or roughanic acid (16:3)] and they include among others hydroperoxi-, hydroxy-, oxo- and keto-fatty acids, divinyl ethers, short-chain aldehydes and the phytohormone JA. The first step of oxylipin biosynthesis is the formation of fatty acid hydroperoxides, which can occur enzymatically (e.g., by lipoxygenases) or non-enzymatically by chemical oxidation of the fatty acid double bonds. Enzymatic conversion of hydroperoxides can occur by various pathways, including those initiated by allene oxide synthase (AOS), divinyl ether synthase (DES), hydroperoxide lyases (HPL), peroxygenases (PXG) or epoxy alcohol synthase (EAS) among others.Citation10 The signaling roles of some of the products of these pathways in defense responses against pathogens and herbivores have been extensively documented.Citation10 As a result of biotic stresses, intracellular levels of reactive oxygen species (ROS) may rise rapidly. These ROS can induce the nonenzymatic oxidation of fatty acids to generate biologically active oxylipins.Citation11 The plastid also produces the bulk of fatty acids in the cell and supplies these molecules for diverse biosynthetic and regulatory pathways.Citation12 In addition to oxylipins, recent evidence indicates that structural fatty acids (e.g., 18:0, 18:1, 18:2 and 18:3) can also modulate defense-related responses in plants. For example, in Arabidopsis, a mutation in the major 18:0-ACP desaturase (FAB2, SSI2) alters defense signaling and resistance via mechanisms associated with the levels of 18:1.Citation13,Citation14 Plastid-derived C6 volatiles are released from mechanically and herbivore damaged leaves immediately (0–5 min) after the stimulus.Citation1 HPL catalyzes the cleavage of fatty acid hydroperoxides into ω-oxo fatty acids and (3Z)-alkenals, generating C6 volatiles that include (2E)-hexenal, (2E)-hexenol, (3Z)-hexenal, hexanal, hexanol and some of their esters and C12 derivatives including traumatin and traumatic acid. The role of C12 ω-oxo fatty acids in the regulation of defense mechanisms requires further attention.
Plastids produce isoprenoids via the methyl-D-erythritol 4-phosphate (MEP) pathway and it is proposed that C10 precursors of monoterpenes and C20 precursors of diterpenes are predominantly synthesized within this organelle.Citation15 Some monoterpenes are de novo synthesized after herbivore damage and released systemically from the whole plant.Citation2 In contrast to the rapid release of C6 volatiles, herbivore-induced monoterpenes start to be released in a diurnal cycle that is decoupled from short-term insect damage. It has been demonstrated that 1-deoxy-D-xylulose-5-phosphate synthase (DXS), the first enzyme of the MEP pathway, plays a major role in the regulation of the pathway.Citation16 However, how plastids control the changes in substrate fluxes for the activation of monoterpene and diterpene biosynthesis and release after herbivory remains at present unclear.
Finally, the plastid reacts to some biotic stresses by the production of the 2-hydroxyl derivative of benzoic acid (BA), salicylic acid (SA). Two main SA biosynthetic pathways have been described in plants, one derived from trans-cinnamate and benzoic acid and one from chorismate via isochorismate synthase (ICS).Citation17 The exact contribution of these two pathways is apparently species-specific. MeSA is emitted as a volatile and it can act as a defence compound against insects.Citation18
Mechanisms Conveying Primary Stress Signals to the Plastid
How the stresses of mechanical damage or herbivory are translated into changes in plastidial metabolic processes remains largely unknown. Recent studies performed with lima bean (Phaseolus lunatus) showed that among the early events occurring during herbivore attack and wounding are a strong depolarization of the plasmamembrane (ΔVm), an enhanced influx of Ca2+, and hydrogen peroxide (H2O2) generation.Citation19,Citation20 These studies suggest that early cellular signals in response to mechanical damage and herbivory are generated in the plasmamembrane environment. These early cellular mechanisms may induce the activation of downstream components such as protein MAPKs or calcium-dependent protein kinases (CDPKs) that will subsequently transduce the primary stress down into the choloroplast, where the targeted enzymes reside (). For example, several extra-plastidial regulatory factors with a potential function upstream of JA biosynthesis have been identified in different plant species. Wounding and herbivory in tobacco species (Nicotina ssp) and tomato (Solanum lycopersicum) activate the mitogen activated protein kinases SIPK (salicylate-induced protein kinase) and WIPK (wound-induced protein kinase).Citation21–Citation23 The silencing of SIPK and WIPK expression in tobacco plants results in a substantial reduction of JA production after wounding and OS elicitation.Citation21,Citation23 The effect of SIPK on JA biosynthesis in N. attenuata leaves occurs most likely at the level of substrate supply, by stimulating the release of α-linolenic acid (18:3) via activation of GLA1 (glycereolipase 1; ).Citation24 In contrast, the effect of WIPK is at the level of conversion of 13-hydroperoxylinolenic acid into 12-phytodienoic acid (OPDA) and therefore WIPK may affect the activity of AOS (allene oxide synthase) and/or AOC (allene oxide cyclase; ).Citation24 Another regulatory component that affects JA production in N. attenuata is NPR1 (Nonexpressor of PR-1), an essential component of the SA signal transduction pathway first identified in Arabidopsis.Citation25 N. attenuata NPR1-silenced plants accumulate substantially lower JA levels after elicitation than WT and similar to SIPK, NPR1 most likely affect the release of 18:3 via GLA1 activation ().Citation24,Citation26 Other extraplastidial regulatory factors that affect JA accumulation in different plant species include the wound-induced receptor-like protein kinase (WRK), calcium-dependent protein kinases (CDPKs), MAPK KINASE 3-MAPK 6 and protein phosphatase 2C (AP2C1).Citation27–Citation30 How these factors affect JA formation is unknown however this diverse set of regulators suggests that a complex network of signals integrated by multiple transduction pathways convey the primary stress signal to the plastid to regulate JA biosynthesis.
N. attenuata SIPK- and WIPK-silenced plants produce similar levels of C6 volatiles within the first hours after M. sexta OS elicitation.Citation31 However, C6 volatile levels are reduced several hours after elicitation in these silenced plants, indicating that SIPK and WIPK may have a long-term effect on C6 production by affecting the expression of the respective biosynthetic genes.Citation31 The JA and C6 volatile biosynthetic pathways share similar initial enzymatic steps, namely the release of free 18:2 and 18:3 from membranes and their hydroperoxidation by lipoxygenases. Interestingly, the plastids of several plant species harbor specific isoforms of lipoxygenases that specifically channel fatty acid hydroperoxides to JA or C6 volatile production and independent mechanisms may affect their activities. Likewise, several lipase isoforms can specifically regulate the JA or C6 volatile biosynthetic pathways.
Genetic evidence for the participation of cytosolic-vacuolar changes in ion fluxes in the regulation of JA biosynthesis comes from the isolation of the fou2 mutant in Arabidopsis, carrying a gain-of-function allele of the Two Pore Channel 1 (TPC1) gene.Citation32 In this mutant, the activity of TPC1 is deregulated in the tonoplast and the production of JA is enhanced several fold after wounding. Thus, one possibility is that changes in ion fluxes induced by deregulated TPC1 activity in the tonoplast are translated into the activation of cytosolic factors affecting JA biosynthesis ().
In the case of SA biosynthesis, genetic evidence indicates that ENHANCED DISEASE SUSCEPTIBILITY 1 (EDS1), NONSPECIFIC DISEASE RESISTANCE 1 (NDR1) and PHYTOALEXIN DEFICIENT 4 (PAD4) are components acting upstream of SA biosynthesis in basal resistance to biotrophic pathogens and that Ca2+/calmodulin-dependent signaling also regulates SA production.Citation17 However, similar to JA biosynthesis, how these regulators signal the plastid to activate SA biosynthesis is at present also unclear ().
Conclusions
Although we understand the biosynthetic pathways leading to the formation of the signal molecules described in this review, the regulatory mechanisms underlying the activation of these plastidial pathways upon wounding and herbivory remain largely unknown. Thus, understanding how the primary stress signals generated by these stimuli are translated into the activation of specific plastidial enzymes controlling the flux of metabolites through signal-molecule-generating pathways is essential if we are to understand how plants control this important signaling system for defense responses.
Figures and Tables
Figure 1 Plastids play a central role in the generation of wounding and herbivory induced signals. After wounding and herbivory, several different cellular events take place at the plasmamembrane [e.g., increased ROS production, changes in ion fluxes and plasmamembrane potential, recognition of insect elicitors (e.g., FACs)]. The perception of pathogen elicitors by specific membrane receptors also activates some of these cellular processes. These events lead to the activation of downstream components in the cytosol (arrow 1) that transduce the primary stress signals to activate enzymes located in the plastid (arrow 2). Changes in ion fluxes between the cytosol and the tonoplast may also influence the activity of plastidial enzymes (arrow 3). The plastid reacts to these stimuli by activating the synthesis of fatty acid-derived signals (e.g., oxylipins and structural fatty acids), SA, and isoprenoid volatiles that regulate direct and indirect defense responses (dashed arrows). FAC, fatty-acid amino-acid conjugate; FAS, fatty acid synthase; ACP, acyl carrier protein; ROS, reactive oxygen species; GLA, glycerolipase A; LOX, lipoxygenase; AOS, allene oxide synthase; AOC, allene oxide cyclase; HPL, hydroperoxide lyase; SKP, shikimate pathway; ICS, isochorismate synthase; BA, benzoic acid (* formation of BA requires one cycle of β-oxidation that most likely takes place in the peroxisome); G3P, glyceraldehyde-3-phosphate; DXS, 1-deoxy-D-xylulose-5-phosphate synthase; DXP, 1-deoxy-D-xylulose-5-phosphate; DXR, 1-deoxy-D-xylulose-5-phosphate reductoisomerase; MEP, methyl-D-erythritol 4-phosphate; MT, monoterpenes; DT, diterpenes.
![Figure 1 Plastids play a central role in the generation of wounding and herbivory induced signals. After wounding and herbivory, several different cellular events take place at the plasmamembrane [e.g., increased ROS production, changes in ion fluxes and plasmamembrane potential, recognition of insect elicitors (e.g., FACs)]. The perception of pathogen elicitors by specific membrane receptors also activates some of these cellular processes. These events lead to the activation of downstream components in the cytosol (arrow 1) that transduce the primary stress signals to activate enzymes located in the plastid (arrow 2). Changes in ion fluxes between the cytosol and the tonoplast may also influence the activity of plastidial enzymes (arrow 3). The plastid reacts to these stimuli by activating the synthesis of fatty acid-derived signals (e.g., oxylipins and structural fatty acids), SA, and isoprenoid volatiles that regulate direct and indirect defense responses (dashed arrows). FAC, fatty-acid amino-acid conjugate; FAS, fatty acid synthase; ACP, acyl carrier protein; ROS, reactive oxygen species; GLA, glycerolipase A; LOX, lipoxygenase; AOS, allene oxide synthase; AOC, allene oxide cyclase; HPL, hydroperoxide lyase; SKP, shikimate pathway; ICS, isochorismate synthase; BA, benzoic acid (* formation of BA requires one cycle of β-oxidation that most likely takes place in the peroxisome); G3P, glyceraldehyde-3-phosphate; DXS, 1-deoxy-D-xylulose-5-phosphate synthase; DXP, 1-deoxy-D-xylulose-5-phosphate; DXR, 1-deoxy-D-xylulose-5-phosphate reductoisomerase; MEP, methyl-D-erythritol 4-phosphate; MT, monoterpenes; DT, diterpenes.](/cms/asset/b8734303-e15e-48d9-97d6-0c4623060aec/kcib_a_10911834_f0001.gif)
Figure 2 Early enzymatic steps in the JA biosynthesis pathway regulated by SIPK, WIPK and NPR1 after wounding and FAC elicitation. The perception of FACs and/or wounding by the leaf elicits signaling mechanisms that activate the release of 18:3 from membrane glycerolipids. This activation is mediated by SIPK and NPR1 in N. attenuata leaves most likely via the activation of glycerolipase A1 (GLA1). N. attenuata lipoxygenase 3 (LOX3) catalyzes the conversion of the free 18:3 into 13-OOH-18:3 which it is rapidly utilized for the synthesis of OPDA by allene oxide synthase (AOS) and allene oxide cyclase (AOC). WIPK-mediated mechanisms target the activation of OPDA biosynthesis via the regulation of AOS and/or AOC. EOT, 12,13-epoxy-18:3; OPDA, (9S,13S)-12-oxo-phytodienoic acid; JA, jasmonic acid.
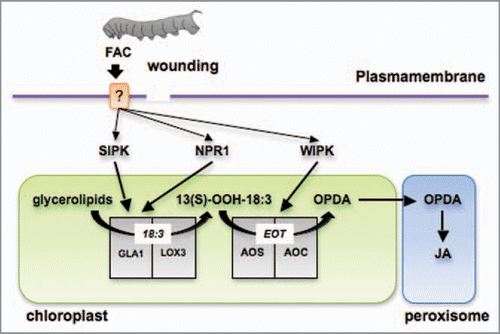
References
- Holopainen JK. Multiple functions of inducible plant volatiles. Trends Plant Sci 2004; 9:529 - 533
- Degenhardt J, Gershenzon J, Baldwin IT, Kessler A. Attracting friends to feast on foes: engineering terpene emission to make crop plants more attractive to herbivore enemies. Curr Opin Biotechnol 2003; 14:169 - 176
- Mithöfer A, Boland W. Recognition of herbivory-associated molecular patterns. Plant Physiol 2008; 146:825 - 831
- Halitschke R, Schittko U, Pohnert G, Boland W, Baldwin IT. Molecular interactions between the specialist herbivore Manduca sexta (Lepidoptera, Sphingidae) and its natural host Nicotiana attenuata III. Fatty acid-amino acid conjugates in herbivore oral secretions are necessary and sufficient for herbivore-specific plant responses. Plant Physiol 2001; 125:711 - 777
- Diezel C, von Dahl CC, Gaquerel E, Baldwin IT. Different lepidopteran elicitors account for cross-talk in herbivory-induced phytohormone signaling. Plant Physiol 2009; 150:1576 - 1586
- Turlings TCJ, Alborn HT, Loughrin JH, Tumlinson JH. Volicitin, an elicitor of maize volatiles in oral secretion of spodoptera exigua: Isolation and bioactivity. J Chem Ecol 2000; 26:189 - 202
- Mithöfer A, Wanner G, Boland W. Effects of feeding Spodoptera littoralis on lima bean leaves II. Continuous mechanical wounding resembling insect feeding is sufficient to elicit herbivory-related volatile emission. Plant Physiol 2005; 137:1160 - 1168
- Heil M. Damaged-self recognition in plant herbivore defence. Trends Plant Sci 2009; 14:356 - 363
- Schmelz EA, Carroll MJ, LeClere S, Phipps SM, Meredith J, Chourey PS, et al. Fragments of ATP synthase mediate plant perception of insect attack. Proc Natl Acad Sci USA 2006; 103:8894 - 8899
- Andreou A, Brodhun F, Feussner I. Biosynthesis of oxylipins in non-mammals. Prog Lipid Res 2009; 48:148 - 170
- Müller MJ. Archetype signals in plants: the phytoprostanes. Curr Opin Plant Biol 2004; 7:441 - 448
- Bonaventure G, Salas JJ, Pollard MR, Ohlrogge JB. Disruption of the FATB gene in Arabidopsis demonstrates an essential role of saturated fatty acids in plant growth. Plant Cell 2003; 15:1020 - 1033
- Kachroo A, Shanklin J, Whittle E, Lapchyk L, Hildebrand D, Kachroo P. The Arabidopsis stearoylacyl carrier protein-desaturase family and the contribution of leaf isoforms to oleic acid synthesis. Plant Mol Biol 2007; 63:257 - 271
- Kachroo A, Venugopal SC, Lapchyk L, Falcone D, Hildebrand D, Kachroo P. Oleic acid levels regulated by glycerolipid metabolism modulate defense gene expression in Arabidopsis. Proc Natl Acad Sci USA 2004; 101:5152 - 5157
- Sharkey TD, Wiberley AE, Donohue AR. Isoprene emission from plants: why and how. Ann Bot 2008; 101:5 - 18
- Cordoba E, Salmi M, León P. Unravelling the regulatory mechanisms that modulate the MEP pathway in higher plants. J Exp Bot 2009; 60:2933 - 2943
- Vlot AC, Dempsey DA, Klessig DF. Salicylic Acid, a multifaceted hormone to combat disease. Annu Rev Phytopathol 2009; 47:177 - 206
- Ament K, Krasikov V, Allmann S, Rep M, Takken FL, Schuurink RC. Methyl salicylate production in tomato affects biotic interactions. Plant J 2010; 62:124 - 134
- Maffei M, Bossi S, Spiteller D, Mithöfer A, Boland W. Effects of feeding Spodoptera littoralis on lima bean leaves I. Membrane potentials, intracellular calcium variations, oral secretions and regurgitate components. Plant Physiol 2004; 134:1752 - 1762
- Maffei ME, Mithöfer A, Arimura G, Uchtenhagen H, Bossi S, Bertea CM, et al. Effects of feeding Spodoptera littoralis on lima bean leaves III. Membrane depolarization and involvement of hydrogen peroxide. Plant Physiol 2006; 140:1022 - 1035
- Seo S, Sano H, Ohashi Y. Jasmonate-based wound signal transduction requires activation of WIPK, a tobacco mitogen-activated protein kinase. Plant Cell 1999; 11:289 - 298
- Kandoth PK, Ranf S, Pancholi SS, Jayanty S, Walla MD, Miller W, et al. Tomato MAPKs LeMPK1, LeMPK2 and LeMPK3 function in the systemin-mediated defense response against herbivorous insects. Proc Natl Acad Sci USA 2007; 104:12205 - 12210
- Wu JQ, Hettenhausen C, Meldau S, Baldwin IT. Herbivory rapidly activates MAPK signaling in attacked and unattacked leaf regions but not between leaves of Nicotiana attenuata. Plant Cell 2007; 19:1096 - 1122
- Kallenbach M, Alagna F, Baldwin IT, Bonaventure G. Nicotiana attenuata SIPK, WIPK, NPR1 and fatty acid-amino acid conjugates participate in the induction of JA biosynthesis by affecting early enzymatic steps in the pathway. Plant Physiol 2010; 52:96 - 106
- Cao H, Bowling SA, Gordon AS, Dong X. Characterization of an Arabidopsis mutant that is nonresponsive to inducers of systemic acquired resistance. Plant Cell 1994; 6:1583 - 1592
- Rayapuram C, Baldwin IT. Increased SA in NPR1-silenced plants antagonizes JA and JA-dependent direct and indirect defenses in herbivore-attacked Nicotiana attenuata in nature. Plant J 2007; 52:700 - 715
- Takabatake R, Seo S, Ito N, Gotoh Y, Mitsuhara I, Ohashi Y. Involvement of wound-induced receptor-like protein kinase in wound signal transduction in tobacco plants. Plant J 2006; 47:249 - 257
- Ludwig AA, Saitoh H, Felix G, Freymark G, Miersch O, Wasternack C, et al. Ethylene-mediated cross-talk between calcium-dependent protein kinase and MAPK signaling controls stress responses in plants. Proc Nat Acad Sci USA 2005; 102:10736 - 10741
- Takahashi F, Yoshida R, Ichimura K, Mizoguchi T, Seo S, Yonezawa M, et al. The mitogen-activated protein kinase cascade MKK3-MPK6 is an important part of the jasmonate signal transduction pathway in Arabidopsis. Plant Cell 2007; 19:805 - 818
- Schweighofer A, Kazanaviciute V, Scheikl E, Teige M, Doczi R, Hirt H, et al. The PP2C-type phosphatase AP2C1, which negatively regulates MPK4 and MPK6, modulates innate immunity, jasmonic acid and ethylene levels in Arabidopsis. Plant Cell 2007; 19:2213 - 2224
- Meldau S, Wu J, Baldwin IT. Silencing two herbivory-activated MAP kinases, SIPK and WIPK, does not increase Nicotiana attenuata’s susceptibility to herbivores in the glasshouse and in nature. New Phytol 2009; 181:161 - 173
- Bonaventure G, Gfeller A, Proebsting WM, Hortensteiner S, Chetelat A, Martinoia E, et al. A gain-of-function allele of TPC1 activates oxylipin biogenesis after leaf wounding in Arabidopsis. Plant J 2007; 49:889 - 898