Abstract
Different types of endosomal vesicles show distinct distribution patterns within cells. While early endosomes can be found throughout the cell, recycling endosomal vesicles and tubules tend to cluster near the microtubule organizing center in the perinuclear region in most cell types. The molecular mechanisms underlying the steady-state distribution and dynamics of various types of endosomal vesicles has long remained enigmatic. However, during the past decade it has become evident that microtubule-based motor proteins of the kinesin family play a pivotal role in the positioning of endosomes. Early endosomes were shown to cluster in the perinuclear area in the absence of KIF16B,1 KIF3A is required for the steady-state distribution of late endosomes/lysosomes,2,and KIF13A directs M6PR-containing vesicles from the TGN to the plasma membrane3 to name only a few examples. In the case of Tf-containing recycling endosomes antibody-injection experiments implicated kinesin-1, a heteromer comprised of KIF5 heavy and KLC light chains, as a motor for their transport towards the cell periphery.4 Indeed, KIF5B knockdown experiments confirmed that kinesin-1 is necessary to maintain the peripheral pool of recycling endosomes.5 But how is kinesin-1 linked to endosomal vesicles? Work from our own laboratory has identified the AP-1-binding protein Gadkin as a molecular link between AP-1-mediated traffic and kinesin-1-based transport along microtubules.5 This work as well as hypothetical models for kinesin-dependent endosomal membrane traffic will be discussed here.
Gadkin has been originally found in a screen for proteins localizing to the Golgi/TGN area. Immunofluorescence microscopy of HeLa cells showed a strong overlap between Gadkin and AP-1, a clathrin adaptor complex involved in vesicle formation at the TGN and/or on endosomes.Citation6–Citation8 The precise mechanisms of action of AP-1 and its partitioning between endosomes and the TGN is not yet fully understood.Citation7,Citation8 However, the ear domain of the γ1-adaptin subunit (γ-ear) of the heterotetrameric AP-1 complex binds to a variety of accessory proteins such as aftiphilin, epsinR/enthoprotin/Clint,Citation9–Citation12 γ-synergin,Citation13 eps15, Rabaptin-5, p56 and NECAPs.Citation14 These factors most likely contribute to the specific targeting of AP-1. Accessory proteins interact with the AP-1 γ-ear via ØG[P/D/E][Ø/L/M] peptide motifs (with Ø being a large hydrophobic amino acid).Citation13,Citation15,Citation16 Gadkin which binds to the γ-earCitation17 contains three sequences (FGLL, FENL, YSGF) that partially conform to this consensus. Surprisingly, mutating all three motifs did not abrogate binding,Citation17 suggesting the existence of other AP-1-binding determinants. Indeed, subsequent Pepscan analysis revealed a fourth binding motif (WENDF)Citation18 within Gadkin. Analytical ultracentrifugation identified this novel subtype of AP-1 γ-ear binding motif as the major high affinity AP-1-binding site within Gadkin. Mutating aromatic amino acids W and F within this motif strongly inhibits Gadkin binding to γ-ear, but mutation of all four motifs is necessary to completely abrogate complex formation between AP-1 and Gadkin.Citation18 NMR spectroscopy demonstrated that Gadkin uses a conserved surface on the γ-ear, a site that is also targeted by other AP-1-binding accessory proteins like γ-synergin and aftiphilin via conventional AP-1 γ-ear binding motifs.Citation18 We speculate that the observed high nanomolar affinity of the novel AP-1 binding motif might provide Gadkin and potentially other factors including GGAs and epsinR with privileged access to the AP-1 γ-ear. Gadkin mutants that are unable to associate with AP-1 localize to the plasmalemma, a phenotype that is also seen upon siRNA-mediated knockdown of AP-1. Hence, AP-1 appears to be an essential determinant for the recruitment of Gadkin to the TGN and/or endosomal vesicles.
As often seen for components of the same complex or pathway, localization of Gadkin and AP-1 is reciprocally interdependent. Although Gadkin does not harbor transmembrane domains, membrane recruitment per se does not depend on functional interactions with AP-1. Mutational analysis and biochemical experiments revealed that three aminoterminal cysteines within Gadkin serve as acceptor sites for its S-palmitoylation, thereby providing Gadkin with a stable membrane anchor.Citation18 This stable membrane association might explain why overexpressed Gadkin increases the fraction of membrane-bound AP-1 and in contrast to other γ-ear associated accessory proteins is able to protect AP-1 from brefeldin A-mediated membrane dissociationCitation17 (AP-1 is recruited to membranes by ARF-1, a process that is blocked by brefeldin A), a function originally referred to in previous nomenclature as “γ1-adaptin brefeldin A resistance” (γ-BAR).
The intimate link between AP-1 and Gadkin is further underscored by overexpression and knockdown experiments. Loss of AP-1 is known to cause defective sorting of lysosomal enzymes such as cathepsin D via missorting of mannose 6-phosphate receptors (M6PRs) from endosomes. Similar to AP-1 μ1A-knockout fibroblasts cells over- or underexpressing Gadkin display a partial redistribution of M6PRs to endosomes and increased secretion of cathepsin D. Thus, Gadkin modulates AP-1 dependent membrane traffic at the TGN/endosomal boundary.Citation17
A striking phenotype was observed upon overexpression of Gadkin in HeLa cells: Gadkin-eGFP positive cells displayed a near complete redistribution of the perinuclear Gadkin/AP-1 positive compartment to the cell periphery. Further analyses demonstrated that the shifted compartment contained the perinuclear endosomal marker proteins Tf, TfR, Rab11, Rab4 and a fraction of cycling TGN/endosomal proteins such as TGN46 and M6PRs, while non-cycling TGN markers like p230 and Golgin-97 remained largely unaffected. Early endosomal, late endosomal/lysosomal, or mitochondrial proteins were absent from peripheral Gadkin accumulations.Citation5 This indicated that Gadkin specifically affects the distribution of perinuclear, most likely TGN-derived endosomal recycling vesicles (REVs). The observed outward redistribution of those vesicles would be best compatible with enhanced transport by a plus-end directed microtubule motor. This idea is further supported by the observation that Gadkin-positive vesicles align with microtubules (). Consistent with microtubule-based transport, proteomic approaches using GST-Gadkin and brain extract tracked down the plus-end directed microtubule motor protein kinesin-1 as a putative Gadkin binding protein. Further biochemical experiments showed that Gadkin directly binds to kinesin-lightchain (KLC), a subunit of the heterotetrameric kinesin-1 complex, via a conserved tryptophan-based acidic amino acid motif.Citation5 Mutating W210 to alanine completely abolished Gadkin binding to kinesin-1. Similar acidic cluster/tryptophan motifs have been shown previously to associate with kinesin light chains in other proteins, an interaction that may involve the KLC TPR repeats.
Gadkin mutants that were defective in either AP-1 or kinesin-1 binding were then used to dissect the importance of either interaction in microtubule-based outward transport of REVs. Depleting cells of AP-1, kinesin-1, or assembled microtubules prevented the Gadkin-driven translocation of Tf-positive REVs to the cell periphery.Citation5 Consistently, AP-1- or kinesin-1-binding defective Gadkin was unable to support plus-end-directed movement of REVs. Thus, Gadkin targets AP-1 positive REVs for plus-end directed movement along microtubule tracks and thereby constitutes a physical link between AP-1 and kinesin-1.
Plus-end directed movement of Gadkin-positive REVs along microtubular tracks is also supported by additional observations. Overexpressed Gadkin decorates microtubular plus-ends in the distal part of growing axons in primary neurons and is localized to the center of the midzone of dividing cells (), another site where microtubule plus-ends are found. Endogenous Gadkin displays a similar localization to the midzone during cytokinesis ().
These data thus suggest a model whereby an AP-1/Gadkin/kinesin-1 complex controls the positioning and/or dynamics of perinuclear REVs. Consistent with this, knockdown of kinesin-1 causes a striking perinuclear concentration of Tf-positive REVs. Suprisingly, depletion of Gadkin did not display a similarly striking effect on REV positioning.Citation5 This suggests that Gadkin is involved in positioning of only a specific subset of REVs and/or that other, partially redundant factors contribute to kinesin-1-mediated perinuclear endosome dynamics. This idea is supported by Gadkin depletion experiments using Tf/TfR sorting and recycling as a functional model. In Gadkin-depleted cells Tf recycling was significantly accelerated by 20%,Citation5 a phenotype also seen in Rab4-depleted cells.Citation19 Following its internalization into peripheral early endosomes Tf can recycle either directly by a fast actin-dependent pathway or by a slow route involving sorting to Rab4-/Rab11-positive perinuclear endosomes.Citation20–Citation22 Knockdown of Gadkin specifically appears to affect the latter pathway and knockdown/rescue experiments suggest that the function of Gadkin in facilitating sorting to Rab4/Rab11-positive endosomes critically depends on its interactions with both AP-1 and kinesin-1. Based on these data we propose the following hypothetical model (): Gadkin is recruited by AP-1 to a subpopulation of perinuclear TfR-, Rab4-, Rab11-positive REVs in close apposition to the TGN. The stability of Gadkin and AP-1 at perinuclear endosomes is reciprocally interdependent on high affinity complex formation between both proteins and on S-palmitoylation of Gadkin. Gadkin binding to kinesin-1 targets Rab4-/Tf-positive REVs to microtubule tracks for plus-end directed transport. This outward-directed microtubular transport may act synergistically with short-range actin- and myosin Vb-dependent movement of REVsCitation23 and most likely is counterbalanced by ASAP1/Rab11Fip3,Citation24 and Rab11-Fip5/Kif3BCitation25 which facilitate pericentrosomal localization of recycling endosomal tubules. Overexpression of Gadkin offsets this intricate balance causing REVs to accumulate in the cell periphery. Conversely, depletion of Gadkin may prevent access of endocytosed Tf to REVs, causing a traffic jam that shunts Tf into the fast direct recycling pathway, resulting in accelerated Tf recycling to the cell surface.
In summary, these collective data identify Gadkin as a novel molecular link between AP-1 mediated vesicular traffic and kinesin-1-dependent REV dynamics, thereby modulating cargo flux through the TGN/endosomal system. Our findings strenghten the emerging paradigm that a plethora of different adaptor/motor combinations are used in the cell to achieve the differential positioning and dynamics of functionally distinct endosomal vesicles.
Previous studies have implicated REV dynamics in a variety of processes including recycling of nutrient (i.e., TfR) and signaling receptors to the cell surface,Citation26 polarized trafficking of cell adhesion molecules during morphogenesis and development,Citation27 plasticity-induced growth of dendritic spines during long-term potentiation in the nervous system,Citation28 as well as the regulation of plasma membrane area during mitosis.Citation29 It remains a challenge for future studies to unravel the precise physiological role of the AP-1/Gadkin/kinesin-1 complex at the organismic level in any one or more of these emerging functions of REVs.
Abbreviations
AP-1 | = | adaptor protein complex 1 |
EGFP | = | enhanced green fluorescent protein |
Gadkin | = | γ1-adaptin & kinesin interactor |
M6PR | = | mannose-6-phosphate receptor |
REV | = | recycling endosomal vesicle |
TGN | = | trans-golgi-network |
Tf | = | transferrin |
TfR | = | transferrin receptor |
Figures and Tables
Figure 1 Role of Gadkin and its partners AP-1 and kinesin-1 in trafficking of TGN-derived endosomal vesicles along microtubule tracks. (A and B) HeLa cells expressing Gadkin WT (shown in red) were fixed 24 h post-transfection and analyzed by indirect immunofluorescence microscopy with antibodies against Gadkin and endogenous tubulin (shown in green). Blue, DAPI-stained nuclei. Insets, 4–5× magnified view of boxed areas. Scale bar, 2 µm. (C) HeLa cells were fixed and analyzed by indirect immunofluorescence microscopy with antibodies against endogenous Gadkin (shown in green) and endogenous tubulin (shown in red). Blue, DAPI-stained nuclei. Inset, 4× magnified view of boxed area. Scale bar, 2 µm. (D) Hypothetical model for the role of Gadkin in the regulation of recycling endosomal membrane traffic. See text for further details.
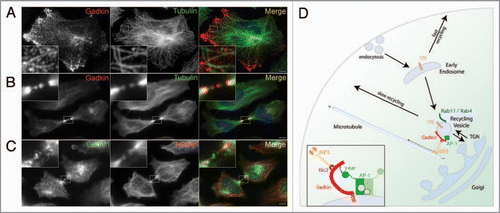
References
- Hoepfner S, Severin F, Cabezas A, Habermann B, Runge A, Gillooly D, et al. Modulation of receptor recycling and degradation by the endosomal kinesin KIF16B. Cell 2005; 121:437 - 450
- Brown CL, Maier KC, Stauber T, Ginkel LM, Wordeman L, Vernos I, et al. Kinesin-2 is a motor for late endosomes and lysosomes. Traffic 2005; 6:1114 - 1124
- Nakagawa T, Setou M, Seog D, Ogasawara K, Dohmae N, Takio K, et al. A novel motor, KIF13A, transports mannose-6-phosphate receptor to plasma membrane through direct interaction with AP-1 complex. Cell 2000; 103:569 - 581
- Lin SX, Gundersen GG, Maxfield FR. Export from pericentriolar endocytic recycling compartment to cell surface depends on stable, detyrosinated (glu) microtubules and kinesin. Mol Biol Cell 2002; 13:96 - 109
- Schmidt MR, Maritzen T, Kukhtina V, Higman VA, Doglio L, Barak NN, et al. Regulation of endosomal membrane traffic by a Gadkin/AP-1/kinesin KIF5 complex. Proc Natl Acad Sci USA 2009; 106:15344 - 15349
- Folsch H, Pypaert M, Maday S, Pelletier L, Mellman I. The AP-1A and AP-1B clathrin adaptor complexes define biochemically and functionally distinct membrane domains. J Cell Biol 2003; 163:351 - 362
- Hinners I, Tooze SA. Changing directions: clathrinmediated transport between the Golgi and endosomes. J Cell Sci 2003; 116:763 - 771
- Meyer C, Zizioli D, Lausmann S, Eskelinen EL, Hamann J, Saftig P, et al. mu1A-adaptin-deficient mice: lethality, loss of AP-1 binding and rerouting of mannose 6-phosphate receptors. EMBO J 2000; 19:2193 - 2203
- Kalthoff C, Groos S, Kohl R, Mahrhold S, Ungewickell EJ. Clint: a novel clathrin-binding ENTH-domain protein at the Golgi. Mol Biol Cell 2002; 13:4060 - 4073
- Hirst J, Motley A, Harasaki K, Peak Chew SY, Robinson MS. EpsinR: an ENTH domain-containing protein that interacts with AP-1. Mol Biol Cell 2003; 14:625 - 641
- Mills IG, Praefcke GJ, Vallis Y, Peter BJ, Olesen LE, Gallop JL, et al. EpsinR: an AP1/clathrin interacting protein involved in vesicle trafficking. J Cell Biol 2003; 160:213 - 222
- Wasiak S, Legendre-Guillemin V, Puertollano R, Blondeau F, Girard M, de Heuvel E, et al. Enthoprotin: a novel clathrin-associated protein identified through subcellular proteomics. J Cell Biol 2002; 158:855 - 862
- Hirst J, Borner GH, Harbour M, Robinson MS. The aftiphilin/p200/gamma-synergin complex. Mol Biol Cell 2005; 16:2554 - 2565
- Ritter B, Philie J, Girard M, Tung EC, Blondeau F, McPherson PS. Identification of a family of endocytic proteins that define a new alpha-adaptin ear-binding motif. EMBO Rep 2003; 4:1089 - 1095
- Mattera R, Ritter B, Sidhu SS, McPherson PS, Bonifacino JS. Definition of the consensus motif recognized by gamma-adaptin ear domains. J Biol Chem 2004; 279:8018 - 8028
- Wasiak S, Denisov AY, Han Z, Leventis PA, de Heuvel E, Boulianne GL, et al. Characterization of a gammaadaptin ear-binding motif in enthoprotin. FEBS Lett 2003; 555:437 - 442
- Neubrand VE, Will RD, Mobius W, Poustka A, Wiemann S, Schu P, et al. Gamma-BAR, a novel AP-1-interacting protein involved in post-Golgi trafficking. EMBO J 2005; 24:1122 - 1133
- Maritzen T, Schmidt MR, Kukhtina V, Higman VA, Strauss H, Volkmer R, et al. A novel subtype of AP-1-binding motif within the palmitoylated trans-Golgi network/endosomal accessory protein Gadkin/gamma-BAR. J Biol Chem 2009; 285:4074 - 4086
- Deneka M, Neeft M, Popa I, van Oort M, Sprong H, Oorschot V, et al. Rabaptin-5alpha/rabaptin-4 serves as a linker between rab4 and gamma(1)-adaptin in membrane recycling from endosomes. EMBO J 2003; 22:2645 - 2657
- Maxfield FR, McGraw TE. Endocytic recycling. Nat Rev Mol Cell Biol 2004; 5:121 - 132
- Sheff DR, Daro EA, Hull M, Mellman I. The receptor recycling pathway contains two distinct populations of early endosomes with different sorting functions. J Cell Biol 1999; 145:123 - 139
- van Dam EM, Ten Broeke T, Jansen K, Spijkers P, Stoorvogel W. Endocytosed transferrin receptors recycle via distinct dynamin and phosphatidylinositol 3-kinase-dependent pathways. J Biol Chem 2002; 277:48876 - 48883
- Hales CM, Vaerman JP, Goldenring JR. Rab11 family interacting protein 2 associates with Myosin Vb and regulates plasma membrane recycling. J Biol Chem 2002; 277:50415 - 50421
- Inoue H, Ha VL, Prekeris R, Randazzo PA. Arf GTPase-activating protein ASAP1 interacts with Rab11 effector FIP3 and regulates pericentrosomal localization of transferrin receptor-positive recycling endosome. Mol Biol Cell 2008; 19:4224 - 4237
- Schonteich E, Wilson GM, Burden J, Hopkins CR, Anderson K, Goldenring JR, et al. The Rip11/Rab11-FIP5 and kinesin II complex regulates endocytic protein recycling. J Cell Sci 2008; 121:3824 - 3833
- Miaczynska M, Pelkmans L, Zerial M. Not just a sink: endosomes in control of signal transduction. Curr Opin Cell Biol 2004; 16:400 - 406
- Pelissier A, Chauvin JP, Lecuit T. Trafficking through Rab11 endosomes is required for cellularization during Drosophila embryogenesis. Curr Biol 2003; 13:1848 - 1857
- Park M, Salgado JM, Ostroff L, Helton TD, Robinson CG, Harris KM, et al. Plasticity-induced growth of dendritic spines by exocytic trafficking from recycling endosomes. Neuron 2006; 52:817 - 830
- Boucrot E, Kirchhausen T. Endosomal recycling controls plasma membrane area during mitosis. Proc Natl Acad Sci USA 2007; 104:7939 - 7944