Abstract
Ciliated protozoa sensing pheromones secreted from nonself mating types engage in preconjugal “courtship” dances and contacts. Using simulated “social” trials, I recently showed the heterotrich ciliate, Spirostomum ambiguum, can learn to advertise degrees of mating fitness to “suitors” and “rivals” when serially contracting or (ciliary) reversing at variable rates. Conspicuous consumers signal higher quality reproductive status by playing “harder-to-get” via metabolically wasteful avoidance displays that hinder the exchange of preconjugal touches between “courting” couples. Conversely, prudent savers conserve energy pending situations more favorable for conjugating a partner. These ciliates reply with lower avoidance frequencies, guaranteeing nearby conspecifics of being “easier-to-get”. By deciding to switch from behavioral strategies signaling conspicuous consumption to those signaling prudent savings, fitter ciliates learn to altruistically sacrifice net payoffs and persuade suitors to participate in paired reproduction. Less fit ciliates, unable to sustain long periods of high response rates, switch their behavioral strategies of prudent savings to briefly emit conspicuous consumption and thus learn to opportunistically cheat superior rivals. Mating competency depends, in part, on the efficiency of heuristics formed from recursive strategy searches and use. Heuristics represent stored patterns of action which evolve into ordered computational networks supporting entire courting repertoires. As ciliates expand signaling skills over many trials, the connectivity between strategies strengthens from Hebbian-like learning, leading to faster decisions about the appropriateness of courting messages and replies. The best experts master signaling decisions at efficiencies comparable to finding target solutions from superposed states with Grover’s quantum search algorithm. I here append these findings with a critique on the feasibility of serial behavioral strategies to perfect ciliate mate selection via classical repetition and quantum bit-flip error-correction codes that safeguard transmitted social information from noise and might be exploited for signal encryption.
Ciliated protozoa can end their lifecycle with sexual-like conjugation capable of yielding eight daughter cells per reproductive pair.Citation1 Conjugation happens between nonself mating types attracted by released pheromones.Citation2,Citation3 “Courtship rituals” preceding conjugation depict trends in cooperation and competition usually attributed to phylogenetically more recent organisms of the Animal Kingdom. Pheromones evoke complex courtship cell-cell contacts and dances patterned by recursive, stereotypic movements associated with avoidance reactions, such as contractions and ciliary reversals, and exploration, such as probing.Citation4–Citation6 Dancers will reset their choreography to insure partner compatibility when interrupted by mating rivals or bystanders. Some genera of heterotrich ciliates, such as Spirostomum,Citation7 even partake in orgy-like mating acts that envelop great numbers of microbes. In the circulating gradients of pheromones secreted by a mixed population of mating types, skilled intra- and intermate selection can give ciliates tremendous advantages in perceiving the fitness of presumptive suitors and rivals as well as in broadcasting their own reproductive status. Honest two-player signaling games often model this kind of ambiguous social setting for animal sexual behavior.Citation8 One player, the sender, communicates a risky, reliable message of sexual prowess not intended to deceive, such as ornate or prudent morphology and behavior. Observing these signals with incomplete knowledge of the sender, the second player, termed the receiver, appreciates the metabolic and predation costs of the message for the sender, makes a judgment about the high quality of the sender referenced to those costs, and picks a behavior in reply. The type of sender, its message, and the receiver’s reply figure into the payoffs expected and/or procured by both parties.
In a recent article,Citation9 I report my findings of the signaling behavior of the large contractile ciliate, Spirostomum ambiguum, after experiencing artificially produced vibration trains that mimic honest mating signals sent by an ambiguous source characterizing suitors and/or rivals. Vibrations are an essential component of courting contacts and dances. Probing pressures and drag turbulence of avoidance reactions may activate mechanosensitive Ca2+ conductances that contribute to motility production in proximal ciliates. S. ambiguum receives these signals, interprets them as “aggressive” or “friendly”, then chooses learned serial actions composed of contractions and ciliary reversals that are themselves honest blind replies to the sender. A payoff schedule determines content of messages and replies based on combinations of potential reproductive effort, calculated as different probabilities of offspring count, and responsiveness, calculated as different probabilities of behavioral preparedness or perceptual bias. These potential payoffs are structured to meet the four requirements of a game beginning in one of two forms of perfect Bayesian equilibria, known as pooling and separating equilibria.Citation10 A pooling equilibrium is where a signaling game between two players of different type, such as mating types, stabilizes on the same signal for message and reply. Whereas, separating equilibrium stabilizes on polar signals for message and reply from players of different type. For both cases of equilibrium, messages and replies become irrelevant or cheap talk for actionable decisions,Citation11 making cheating or cooperation incidental unless equilibrium is broken by learning or other means, including color noise. In my experiment, messages emitted in vibrations from their ambiguous source, always deemed with equal probability the same and opposite mating type of each ciliate tested, express net interest in reproductive effort. Ciliates “believing” the signals to be honest, but unable to discriminate the vibration source’s type, initially reply with matched or opposing signals falling between ideal conspicuous consumption and ideal prudent savings. Ciliates beginning the game (i.e., testing) at high responsiveness display initial conspicuous consumption. Conspicuous consumers advertise their reproductive fitness by playing harder-to-get through the extravagant rate at which they signal escape. By being fit enough to squander resources, these ciliates should be perceived by the ambiguous vibration source to be of higher quality than inferior repliers incapable of both wastefulness and survival. Initial inferior repliers, such as ciliates with initial low responsiveness, higher costs, and poorer net payoffs, announce meager fitness by prudently saving resources and signaling escape at lower rates. They begin play as easier-to-get, but can maximize individual potential net payoffs and reproductive value by increasing responsiveness.
S. ambiguum controls signaling of preferences for social interactions in ambiguous settings by modifying their escape behaviors and potential net reproductive payoffs via simple learning, such as nonassociative habituation and sensitization. After starting in pooling or separating equilibrium, learning breaks game equilibrium to make talk less cheap for initial prudent savers trying to compete with better repliers and for initial conspicuous consumers willing to sacrifice payoffs to cooperate. Furthermore, ciliates, very similar to “higher” eukaryotes, learn via Hebbian-like feedbackCitation12 to organize their behavioral strategies into multistable heuristics founded on internal cues that prepare ciliates to make certain kinds of perceptions in ambiguous contexts (see ). Heuristics help ciliates facilitate planning and execution of serial strategies signaling different levels of mating fitness by reducing combinatorial complexity and strategy search times to quantum levels with growing expertise. Initial prudent savers or conspicuous consumers capable of faster, complete behavioral strategy searches easily learn to switch their replies between playing harder-to-get and easier-to-get over brief intervals. As a consequence, they display greater flexibility for adapting replies and the expected net reproductive payoffs accompanying those replies to putative social settings which might demand either selfishness or selflessness to encourage successful courtship relationships. Importantly, the bit string configuration of courting messages and replies (see ) allows for error-correction codes to be utilized by ciliates to protect the degradation of signal content, and therefore courting strategies, from noise. Both color and white noise can affect a signal. For instance, nonrandom color noise in the form of vibrations created by mating rivals can collide with those emitted by mating suitors, physically modulating signal amplitude and frequency and perhaps purposefully interfering with courtship rituals. Alternately, random ecological white noise, such as elevated viscosity of an aqueous habitat due to climate changes or local disturbances in habitat currents caused by motion of floating organic debris and additional obstacles, may also dampen, annihilate, or otherwise distort signals. From an information transmission perspective, it thus makes good sense for ciliates to have evolved mechanisms of error-correction to guarantee specificity of cell-cell communications during mate selection.
One simple error-correction technique potentially used by ciliates, known as repetition coding, incorporates redundant key information in a coded bit string. If part of the coded string becomes corrupted, the key information maintains a high probability of being retrievable from the original message or reply in a process called “majority voting” decoding. This type of error-correction only works for classical digital information passing through a noisy communication channel, such as the binary symmetric channel having normalized probabilities p > 0 and 1 − p > 0 of transmitting information with and without error, respectively (see ).Citation14 Repetition coding is evident in my previous analyses of heuristic-guided social commitments advertised by ciliates. Sequences of all-or-none contractions and all-or-none ciliary reversals are stored by ciliates as three-bit signaling strategies, which obey Takens' embedding theorem,Citation15 in separate topologically invariant computational networks.Citation9 Strategy 000, signaling ideal prudent savings, and strategy 111, signaling ideal conspicuous consumption, appear to function as orthonormal logical 0 and logical 1 codes with additional copies of unit basis states 0 or 1 completing the bit string. Bit copies permit a message or reply of 0 (i.e., no response), signaling prudent savings, or 1 (i.e., response), signaling conspicuous consumption, to be transmitted between sender and receiver with a bit-flip error corrupting the code. As long as no more than one bit flip occurs in the sequence of three contractions or ciliary reversals, the message or reply is delivered and decoded successfully at probability p < 1/2. When p exceeds this value, the probability of error becomes pE = 3p2 − 2p3 > p and signal transmission loses its improved reliability over single-bit coding. Ciliates tend to exploit the improved reliability of repetition coding, as modal serial behavioral strategies (i.e., those strategies most frequently employed by ciliates) signal ideal prudent savings or ideal conspicuous consumption.
Another plausible means of error-correction used during ciliate mate selection is the quantum bit-flip code. This code belongs to a family of codes generally termed quantum error-correction coding that enlists quantum computation and information theory to safeguard information against noise. In quantum information theory, the classical bit is replaced with the quantum bit or qubit. A single qubit is the orthonormal unit vectors or basis states |0〉 and |1〉 for a two dimensional vector space put into linear combination or superposition: |Ψ〉 = αβ0〉 + β|1〉, where variables α and β are complex numbers called vector amplitudes. Measuring a qubit after communication transmission yields a single pure state of 0 or 1, such as that denoting no response or response for a courting ciliate, with respective probabilities |α|2 and |β|2, so that |α|2 + |β|2 = 1. Now suppose that ciliates code their signaling behavior as contraction-reversal qubits in a manner similar to repetition coding. S. ambiguum exhibits a modest negative correlation between the use of mating signals conveyed by serial all-ornone contractions and serial all-or-none ciliary reversals, indicating an intracellular bottleneck exists for “sensorimotor” resources involving free cytosolic and stored calcium.Citation9 In other words, the contraction or ciliary reversal strategy 000 signaling ideal prudent savings accompanies, with few exceptions, the respective orthonormal ciliary reversal or contraction strategy 111 signaling ideal conspicuous consumption. Since serial strategies are built from individual responses, an “anticorrelation” between serial contraction and ciliary reversal signals might be perfectly converted, for example, from a superposed single contraction-reversal qubit state, |Ψ〉 = α|0〉 + α|0〉, into a superposed logical |Ψ〉 and logical |1〉 coding scheme, |Ψ〉L = α|000〉 + β|111〉, with three encoded qubits. This sort of coding scheme, known as the quantum bit-flip code, upholds the no-cloning theorem and has respective probabilities p and 1 − p of being transmitted through a noisy quantum bit-flip channel with and without error (see ).Citation14 The three-qubit message or reply, like classical repetition coding, raises signal reliability over that of a single qubit message or reply, provided no more than one quantum bit-flip error occurs in the encoded sequence of qubits. A message or reply containing additional quantum bit-flips in a three-qubit code has an error probability of: pE = 3p2 − 2p3 > p > 1/2.
By coding courtship signals with classical and quantum error-correction procedures, ciliates may powerfully increase the reliability of finding and choosing a mate under noisy social conditions, such as those introduced by self-interested rivals. Classical repetition coding offers the most straightforward behavioral and cell biological ways for suitors and recipients to accomplish this goal. Suitors simply need to repeat a message over brief intervals and recipients need to perceive those messages with established sensory transduction systems, such as two-component response regulators, which implement summation integration or possibly coincidence detection to evaluate errors in transmitted messages.Citation16,Citation17 The detection sensitivity of such systems tend to be highly modifiable due to feedback control, leading to traditionally learned quantum-level changes in the planning and execution of a recipient’s replies.Citation9,Citation13,Citation18 Despite the feasibility of classical error-correction codes in ciliate mate selection, analogous quantum bit-flip methods require as of yet unknown errordetection, termed “syndrome diagnosis”, and code-recovery mechanismsCitation14 at the scale of single cells that will not perturb the signal’s quantum state during measurement. Until such mechanisms become identified, quantum bit-flip codes will continue to be speculative for the information processing of ciliates as well as all living biological systems, including plants, bacteria, and animals, which exchange signals. If or when mechanisms for quantum error-detection and code-recovery are found, non-naïve senders and receivers at any systematics level might use these processes to also communicate encrypted signals to one another based on principles of quantum teleportation.Citation19
Figures and Tables
Figure 1 Ciliates build heuristics from grouped serial behavioral strategies used to signal courting assurances. (A) Serial contraction or reversal strategies containing three consecutive behavioral events, symbolized by binary strings composed of basis bit 1 (response) and/or 0 (no response), organize into several types of homology groups. Serial strategies with one or fewer responses convey prudent savings. those with two or more responses convey conspicuous consumption. topologically invariant groups of tetrahedral-like (T) (top row) and pyritohedral-like (Th) (second row) symmetry store different signals of uniform mating fitness. Groups storing different signals of mixed mating fitness make transitions between strategies of prudent savings and conspicuous consumption (third row). An incomplete heuristic used by a ciliate transitioning from prudent savings to conspicuous consumption during sensitization learning (bottom row). (B and C) examples of multistable contraction and ciliary reversal heuristics evolving over time, sn, into 3d computational networks with eight different strategies. the same ciliate built, searched, and used both heuristics. It began testing by signaling prudent contraction savings and conspicuous reversal consumption, then sensitized its contraction output to signal conspicuous consumption and habituated its reversal output to signal prudent savings. edges between vertices of computational structures in (A) through (C) indicate a ciliate's choice to switch from one signal to another. recurrent decisions overlap and might not be visible in heuristic representations. Figure 1 reproduced fromCitation13 with permission.
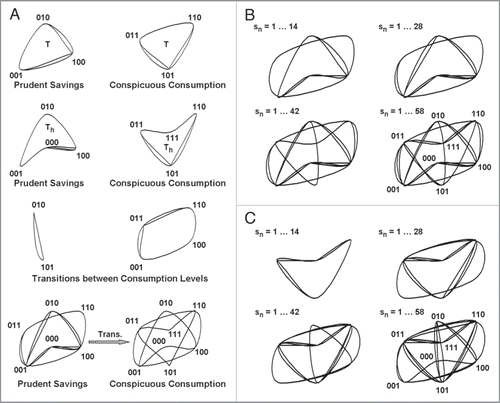
Figure 2 Examples of noisy binary symmetric and quantum bit flip channels interfering with suitor messages. (A) With a binary symmetric channel, an S. ambiguum suitor either contracts (1) or remains fully extended (0) with probability 1 − p of respectively sending or not sending a single courting vibration without error to a recipient S. ambiguum. A noisy S. ambiguum rival positioned between suitor and recipient emits a single contraction with a probability p of corrupting the suitor’s message. Vibration from rival’s contraction may collide with suitor’s vibration, annihilating both signals and flipping the courting signal from 1 to 0. Or, vibration from rival’s contraction may pass to the recipient when suitor remains fully extended, flipping the courting signal from 0 to 1. The rival’s behavior is a form of social cheating, where it takes advantage of its closer physical proximity to the recipient to attract (e.g., bit flip from 0 to 1) and conjugate (e.g., bit flip from 1 to 0) a mating partner already “aroused” by the suitor's previous actions. (B) With a quantum bit flip channel, the suitor codes its courting message as a single contraction (C)-reversal (R) qubit. A ciliary reversing suitor typically does not contract, so it sends a courting message (gray): |Ψ〉 = α|1 + β|0〉, with probabilities 1 − p and p of being delivered and decoded with or without perfect fidelity, respectively. The corrupted message (gray), |Ψ〉E = α|0〉 + β|1〉, is flipped. Likewise, a contracting suitor usually does not ciliary reverse. It projects a courting message (black): |Ψ〉 = α|0 + β|1〉, with the same probabilities of reliability noted above. The corrupted message (black), |Ψ〉E = α|1〉 + β|0〉, is flipped. Suitor contractions and ciliay reversals create quantitatively and qualitatively dissimilar vibrations from drag turbulence, redirecting or pulling local microcurrents surrounding the recipient toward the suitor. Contraction and reversal courting messages differ in that distances traveled by the suitor during contraction are shorter and that “body” re-extension follows contraction, increasing the opportunity for suitor and recipient to touch after avoidance. A noisy rival juxtaposed between suitor and recipient tries to disrupt the message and any further relations between suitor and recipient.
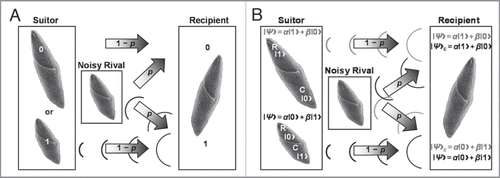
Addendum to:
References
- Lynn DH. The ciliated protozoa: characterization, classification and guide to the literature 2008; Berlin Springer,
- Stock C, Krüppel T, Key G, Lueken W. Sexual behavior in Euplotes raikovi is accompanied by pheromoneinduced modifications of ionic currents. J Exp Biol 1 1999; 202:475 - 483
- Vallesi A, Giuli G, Bradshaw RA, Luporini P. Autocrine mitogenic activity of pheromones produced by the protozoan ciliate Eupotes raikovi. Nature 1995; 367:522 - 524
- Cronkite DL. A suppressor gene involved in chemical induction of conjugation in Paramecium Aurelia. Genetics 1975; 80:13 - 21
- Ricci N. The behavior of ciliated protozoa. Anim Behav 1990; 40:1048 - 1069
- Seshachar BR, Padmavathi PB. Conjugation in Spirostomum. Nature 1959; 184:1510 - 1511
- Bishop A. Some observations on Spirostomum ambiguum (Ehrenberg). Q J Microbiol Sci 1923; 67:391 - 434
- Johnstone RA. Sexual selection, honest advertisement and the handicap principle: reviewing the evidence. Biol Rev 1995; 70:1 - 65
- Clark KB. Origins of learned reciprocity in solitary ciliates searching grouped ‘courting’ assurances at quantum efficiencies. BioSystems 20 2010; 99:27 - 41
- Osborne MJ, Rubenstein A. A course in game theory 1984; Cambridge MIT Press,
- Farrell J, Rabin M. Cheap talk. J Econ Perspect 1996; 10:103 - 118
- Hebb DO. The organization of behavior: a neuropsychological theory 1949; New York Wiley,
- Clark KB. Bose-Einstein condensates form in heuristics learned by ciliates deciding to signal ‘social’ commitments. BioSystems 2010; 99:167 - 178
- Nielsen MA, Chuang IL. Quantum computation and quantum information 2000; Cambridge Cambridge University Press,
- Takens F. Rand DA, Yong LS. Detecting strange attractors in turbulence. Dynamical systems and turbulence 1981; Berlin Springer, 366 - 381
- Eisenstein EM, Brunder DG, Blair HJ. Habituation and sensitization in an aneural cell: some comparative and theoretical considerations. Neurosci Biobehav Rev 1982; 6:183 - 194
- Mok KC, Wingreen NS, Bassler BL. Vibrio harveyi quorum sensing: a coincidence detector for two autoinducers controls gene expression. EMBO 2003; 22:870 - 881
- Clark KB. Quantum ‘social’ intelligences expressed by microbes. SciTopics 2010; www.scitopics.com/Quantum_Social_Intelligences_Expressed_by_Microbes.html
- Bennett CH, Brassard G, Crépeau C, Jozsa R, Peres A, Wootters W. Teleporting an unknown quantum state via dual classical and EPR channels. Phys Rev Lett 1993; 70:1895 - 1899