Abstract
Animals that lack rigid structures often employ pressurization to maintain body form and posture. Structural stability is then provided by incompressible fluids or tissues and the inflated morphology is called a hydrostatic skeleton. However, new ground reaction force data from the caterpillar, Manduca sexta suggest an alternate strategy for large soft animals moving in complex three dimensional structures. When crawling, Manduca can keep its body primarily in tension and transmit compressive deformation forces through the substrate. This effectively allows the caterpillar to minimize reliance on a hydrostatic skeleton and helps it conform to the environment. We call this alternative strategy an "environmental skeleton".
Caterpillar Locomotion
The primary role of lepidopteron larvae is to collect nutrients for future reproduction. Finding and maintaining access to resources by foraging requires locomotion. For many caterpillars there is no need to run away from predators (caterpillars are usually cryptic or toxic) so locomotion can be relatively slow, trading speed and agility for stability and a large carrying capacity. As with all animals it is presumably important to conserve energy whenever possible so caterpillars are expected to exploit efficient mechanical strategies.
In addition to the six true legs present in adult insects, many larvae have extra limbs to support their large abdomen or to perform stage specific behaviors. In caterpillars these abdominal appendages are called “prolegs.” Studies of gene expression patterns suggest that they can develop in any abdominal segment.Citation1,Citation2 Consequently, different species have evolved different proleg configurationsCitation3,Citation4 and a variety of gaits.Citation5–Citation7 Most caterpillars initiate a body contraction from the posterior end and propagate the deformation forward:Citation8 this anterior-grade wave resembles either crawling or inching depending on the configuration of the prolegs and the length of body that is lifted in each step ().
Inching is probably one of the most primitive modes of locomotion due to its mechanical simplicity. In contrast to articulated legged systems where body masses are pivoted over the legs at the footholds,Citation9 an inching strategy benefits animals that cannot take compressive loads. Many legless animals can exploit this gait including leeches, snakes and earthworms. Even though they have legs, caterpillars use variations of inching to locomote because their stubby soft legs can neither transmit compressive load nor produce significant leverage. Large caterpillars such as Manduca employ a crawling gait that has more complex loading characteristics but much is still reminiscent of inching. The progressive wave of movements can be regarded as segmental inching with the substrate as a skeletal support (instead of the inherent hydrostatic skeleton). By supporting its body with an array of prolegs the caterpillar can afford to be much larger without sacrificing mobility or stability.
The Role of Prolegs in the Context of Locomotion
Lepidopteran prologs can be specialized for many functions,Citation10 and their morphologies can vary dramatically.Citation11 In locomotion, however, they are body anchors that lock sections of the abdomen to the substrate to counteract muscle contraction. In addition to providing dependable grip a key feature of the prolegs is that they must detach cleanly on demand. In Manduca the hooking strength of even a single plantar is sufficient to support the entire body weight so release must be completely reliable. This is borne out by the ground reaction forces measured from horizontal crawling caterpillars which were always positive (weight loading) and never negative (pulling upwards) in the vertical direction indicating clean detachment from each step.Citation12 As expected, most locomotor deformations are along the direction of travel (or the animal body axis). The vertical loading on the prolegs during horizontal crawling resembles simple weight placement. The body center of mass does not vary over 10% the body length, and the overall body length never changes more than 5%. This implies that the animal rarely attempt to force any body curvature during its locomotion. The body simply takes on the curvature of the substrate. The major forces are directed along the animal body axis and probably generated by the large longitudinal muscles in the body.
Body Tension and Crawling
A worm-like cylinder with only fluid inside tends to collapse under its own weight unless the internal pressure is sufficient to keep the body wall in tension. This sagging phenomenon becomes more significant as the structure scales up in size (Lin et al. unpublished data) but it can be counteracted with additional support along the body. This seems to be the trend in caterpillars because larger species typically have more prolegs and small ones appear to have lost mid abdominal prolegs through evolution.Citation13 However, locomotion requires the release of prolegs from the substrate at least temporarily thus large caterpillars need to be able to maintain body posture during foothold exchange. Let us consider a scenario where a caterpillar is crawling on a branch upside down in the horizontal orientation, something very common in nature (). The caterpillar has two ways to control body sagging under gravity whenever the mid prologs are disengaged. The first one involves increasing the body pressure to activate the hydrostatic skeleton. The body wall will be loaded against the incompressible body fluid, entering the classical hydrostatic support paradigm (). To counter the gravitational pull on any suspended segments, the caterpillar might need to further tighten the dorsal muscles. An alternative method to prevent sagging is to simply load the body against the substrate, using the prolegs as anchors against the body contraction. This overall body tension will keep the body stable in much the same way as a laundry line can be pulled straight even under the load of the laundry (). The ground reaction force data suggest that large caterpillars such as Manduca prefer this tension strategy whenever they are attached to a substrate.
Of course it would be oversimplifying to suggest that the hydrostatic skeleton plays no role in caterpillar locomotion. During the first stage of a crawl cycle, Manduca must pick up the three most posterior body segments to advance the footholds. A certain amount of body rigidity is necessary, and the muscle contractions associated with lifting must help to pressurize the body. However, once the terminal prolegs are planted, the body immediately transits into a tension loaded condition in which mid body contractions are checked by the advancing thoracic legs and the locked terminal segments.
Thoracic Legs and Hydrostatic Posture Control
Although we treat caterpillars as soft-bodied animals, they still have some rigid components. Beside the big head capsule which houses the massive mandibular muscles, they also have the six articulated true legs in the thorax. These legs can push and pull especially with claws at the metatarsal. This results in a continuous positive ground reaction force in the thorax. The ability to articulate these appendages also enables caterpillars to climb up their own silk threads (lifelines) when they drop from the trees.Citation5 In some caterpillars such as the case-bearing species only the thoracic legs are used for locomotion leaving the reduced prolegs to hold on to their protective shells.Citation14,Citation15 To explore the functional contrast between thoracic legs and prolegs, we suspended Manduca from its head capsule and dorsal horn. When a stiff substrate was placed under the thoracic legs it was passed along to the abdominal prolegs and then backward as the caterpillar performed regular crawling kinematics. If this stiff wooden dowel was replaced with a flexible and curved silicon tube that could not be easily crumpled the crawling behavior was normal and the tube was “walked” backwards along the prolegs (). This demonstrates that body posture is not an essential parameter in caterpillar crawling. In contrast, when Manduca was given a flexible and compressible substrate such as a silk braid the thoracic legs still progressed along the thread (as in silk climbing) but abdominal segments that rely on compressing the substrate could not be stretched out sufficiently. As an interesting consequence, Manduca becomes stuck in the extension phase of the crawl cycle. In this phase, the posterior prolegs are brought forward and planted and the thoracic legs progress forward to stretch out the body. Since the silk braid does not offer any extension force between the thorax and the posterior abdomen, the animal simply kept spooling the substrate from the thoracic legs or simply abandoned the substrate altogether. This is distinct from a typical hydrostatic mechanism in which an animal straightens its body without the aid of any substrate. Because Manduca can perform “reaching” movements that involve stiff counter-levering of the anterior segments, this failure to crawl normally on a soft substrate might be a motor pattern constraint or a behavioral preference. The overall body tension might be an important cue for the crawling gait, and such information could come from any stretch sensors since the same tension is felt across multiple segments in series.
Concluding Remarks
The compliance of biological structures varies in a continuum, and depends on the relative scale. We can say tree branches are stiff because they maintain a configuration under their own weight. At other times, they are compliant under the aerodynamic loads of wind. A worm may not have any rigid structures to support itself. Yet when the body pressurizes to perform a task, the whole body becomes sufficiently stiff relative to the functional load. Caterpillars are soft because they give in to the “posture” of the substrate in most situations. This strategy not only ensures a good match between the caterpillar body curvature and that of the substrate but also create a robust animal-substrate system. With the prolegs as ultimate anchors, caterpillars can use any shape of substrate as their external skeleton. Staying sufficiently soft allows them to navigate complex 3-D structures and blend into the contours of their environment.
Figures and Tables
Figure 1 Two dominant modes of locomotion in caterpillars: crawling and inching. Some caterpillars with selective proleg reduction develop unique intermediate inch-crawl gaits which will be reported later in a full research article.
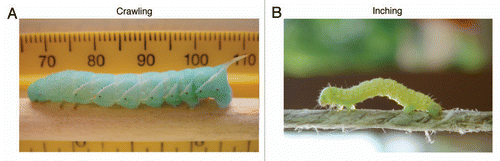
Figure 2 A simple scenario illustrates the difference between using hydrostatic skeleton and environmental skeleton for posture control. (A) A doubly supported soft beam will buckle dramatically under the influence of gravity. (B) Pressurization is one way to increase stiffness and therefore maintaining the linear configuration. The buckling side (dorsal) will experience greater tensile stress according to the beam theory. (C) Alternatively, and overall increase of axial tension can straighten the body without pressurization. Yet the substrate needs to withstand substantial compressive load from the animal without buckling.
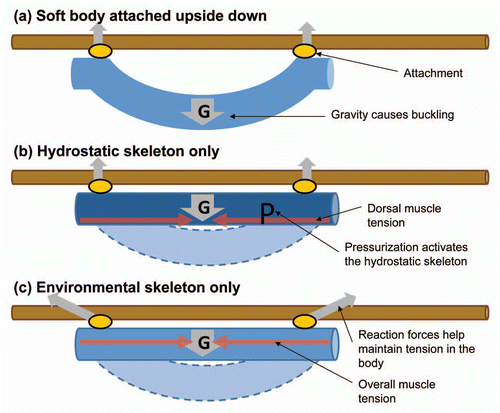
Figure 3 Substrate stiffness greatly affects the body control of Manduca caterpillars as demonstrated in the suspension experiment. (A) There are no observable kinematics differences when the caterpillar is walking a stiff substrate. (B) Flexible substrate has variable curvature which the caterpillar simply adapts to it. (C) Soft substrate that cannot support bending load nor compression leads to gait confusion in the caterpillar's normal locomotion since the substrate fails to maintain the axial tension in the caterpillar body.
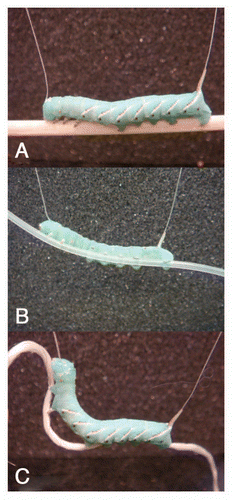
Addendum to:
References
- Suzuki Y, Palopoli MF. Evolution of insect abdominal appendages: are prolegs homologous or convergent traits?. Dev Genes Evol 2001; 211:486 - 492
- Warren RW, Nagy L, Selegue J, Gates J, Carroll S. Evolution of homeotic gene regulation and function in flies and butterflies. Nature 1994; 372:458 - 461
- Hall BK, Wake MH. The origin and evolution of larval forms 1999; Boston Academic Press
- Miller JC, Janzen DH, Hallwachs W. 100 Caterpillars 2006; Belknap Press of Harvard University Press
- Brackenbury J. Novel locomotory mechanisms in caterpillars: life-line climbing in Epinotia abbreviana (Tortricidae) and Yponomeuta padella (Yponomeutidae). Physiol Entomol 1996; 21:7 - 14
- Brackenbury J. Caterpillar kinematics. Nature 1997; 390:453
- Brackenbury J. Fast locomotion in caterpillars. J Insect Physiol 1999; 45:525 - 533
- Trimmer BA, Issberner J. Kinematics of soft-bodied, legged locomotion in Manduca sexta larvae. Biol Bull 2007; 212:130
- Holmes P, Robert J, Koditschek DE, Guckenheimer J. The dynamics of legged locomotion: models, analyses and challenges. SIAM Rev 2006; 48:207 - 304
- Scott JL, et al. The evolutionary origins of ritualized acoustic signals in caterpillars. Nature Commun 2009; 1:1 - 9
- Snodgrass RE. Principles of insect morphology 1993; Cornel University Press
- Lin HT, Trimmer BA. The substrate as a skeleton: ground reaction forces from a soft-bodied legged animal. J Exp Biol 2010; 213:1133 - 1142
- Wagner DL. Caterpillars of eastern North America: a Guide to Identification and Natural History 2005; Princeton NJ Princeton University Press
- Rubinoff D, Haines WP. Web-spinning caterpillar stalks snails. Science 2005; 309:575
- Gaston KJ, Reavey D, Valladares GR. Changes in feeding habit as caterpillars grow. Ecol Entomol 1991; 16:339 - 344