Abstract
Synchronized spikes prevail in cortical networks, and their modulations are associated with attention, sensory processing, and motor behaviors, yet it remained unclear how spikes can synchronize at the millisecond precision in a noisy network. Our new evidence shows, however, that neurons do not easily synchronize; rather, in order to make them generate synchronous spikes, "parent" presynaptic neurons commonly shared by them are required to synchronize in advance. Therefore, the conventional style of neurophysiological research is unable to reach the true origin of synchronization.
Neurons transmit spikes through a web of neurites and synaptic junctions, in which segregation and integration of multiple spike flow contribute to information processing. Individual spikes are usually orchestrated into synchronized events consisting of various sizes of neuron populations. The strength of synchronization between any two neurons is often referred to as a ‘functional’ connection, and their large-scale network topology has been examined by graph-theoretic studies.Citation1–Citation4 On the other hand, anatomical studies have addressed the ‘structural’ connectivity of synaptic wiring among neurons.Citation5–Citation9 A fundamental question, however, is how the functional and structural connectivity are mutually correlated?
Unfortunately, due to a lack of appropriate experimental techniques, spiking patterns and synaptic architectures have not been directly compared in identical neuronal networks and information is still lacking about their relationship. The only possible approach is mathematical modeling of “in-silico” neuronal networks. The numerical simulation can infer how patterned activities emerge from specifically structured circuits and how they in turn lead to network reorganization, but this conjecture has to be experimentally verified. To do this, it is essential to record the spatiotemporal pattern of spikes from neuronal networks in which the synaptic connectivity was identified.
In our recent paper, we conducted simultaneous patch-clamp recordings from two or more CA3 pyramidal neurons in organotypic cultures of hippocampal slices.Citation10 The CA3 region includes an autoassociative network in which pyramidal cells are unidirectionally or bidirectionally synapsed with a connection probability of 20–30%, and thus, we sometimes encountered synaptic coupling between randomly selected pairs of pyramidal cells. Importantly, the connected pairs exhibited higher rates of synchronous firing than uncoupled pairs did; in other words, the functional connectivity becomes stronger when two neurons are anatomically connected. Thus, the functional connectivity is a relatively good reflection of network wiring topology.
Can the presence of synaptic connections fully explain spike synchronization between the two neurons? The answer is, of course, no. In general, cortical excitatory synapses are weak and unreliable, and a single synapse is insufficient to induce a suprathreshold depolarization to emit an action potential of a postsynaptic neuron (). To make it fire, dozens (or even hundreds) of presynaptic neurons have to send synaptic inputs to it within a narrow time window such as tens of milliseconds. But does this truly happen? The most likely explanation seems that neurons that emit more synchronized spikes share more common presynaptic neurons and receive correlated synaptic inputs from them.
To address this, we conducted functional multineuron calcium imaging (fMCI). This optical technique can probe the pattern of spiking activity and synaptic wiring in a large neuron population,Citation11,Citation12 providing a unique opportunity to access the functional and anatomical relationship with a single-cell resolution. We have improved fMCI so as to image from about 100 neurons at frame rates of 500–2,000 Hz. We also devised fMCI for thoroughly mapping the synaptic connections among them. Then we compared synchrony-based networks with synaptically defined networks. As we expected, we indeed found that more highly synchronized pairs of neurons share more numbers of common presynaptic neurons. Furthermore, targeted patch-clamp recordings from highly synchronized neurons revealed that they received highly correlated synaptic inputs.
Then, are these correlated inputs responsible for synchronized spike output? The story is not straightforward. We found that in silico generated correlated inputs were unable to efficiently elicit synchronized spikes even though their correlation efficient was increased to a realistic extent. This implies that spontaneously occurring synaptic inputs convey more information that cannot be captured by the widely used cross-correlation measurement, thereby efficiently producing synchronized spikes in postsynaptic neurons (). Then what is the true source of synchronization?
During further careful comparisons between functional and anatomical connectivity, we realized that common parent neurons per se already synchronized in a power-law scale, sending highly coherent synaptic inputs to postsynaptic neurons. Consistent with this, when in silico correlated synaptic inputs were generated from power law-scaled presynaptic spike trains and injected into two pyramidal neurons, we now found that they exhibited strongly synchronized spikes, as comparable to actual synchronization ().
Overall, what our study finally unveiled is that synchronized spike outputs require highly synchronized synaptic inputs arising from “parent” presynaptic neurons, but also vice versa. Moreover, the synchronized parents naturally require synaptic inputs from highly synchronized spikes of “grandparent” neurons, which must also be synchronized by further upstream synchronized neurons, and so on. Now we must return to the fact that CA3 neurons are densely interconnected through a recurrent network. Our journey for searching the origin of synchronization will eventually come back to the starting point of synchronization. This functional recursive loop, a feature often found in the self-organizing complex system, can be interpreted as a “recurrent network” version of synfire chain.Citation13–Citation15
In the brain, the degree of synchronization is known to vary depending on the behavioral state. Under awake conditions, the network tends to be more asynchronous.Citation16,Citation17 Thus, a next-step study will be required to elucidate the modulation of the synchrony level.
Figures and Tables
Figure 1 Synchronization of presynaptic neuron layer is required to synchronous spikes of postsynaptic neuron pair. (A) Synaptic connection between two neurons by itself cannot synchronize them. (B) Common synaptic inputs from the presynaptic neuron layer contribute to postsynaptic spike synchronization to some extent but cannot fully account for the degree of naturally occurring synchronization. (C) Highly synchronized presynaptic neurons efficiently lead to postsynaptic synchronization.
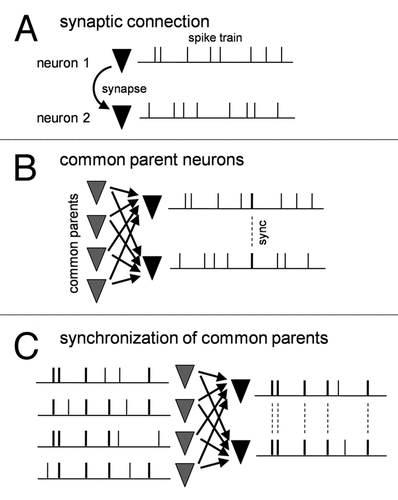
Addendum to:
References
- Beggs JM, Plenz D. Neuronal avalanches are diverse and precise activity patterns that are stable for many hours in cortical slice cultures. J Neurosci 2004; 24:5216 - 5229
- Eytan D, Marom S. Dynamics and effective topology underlying synchronization in networks of cortical neurons. J Neurosci 2006; 26:8465 - 8476
- Sasaki T, Matsuki N, Ikegaya Y. Metastability of active CA3 networks. J Neurosci 2007; 27:517 - 528
- Yu S, Huang D, Singer W, Nikolic D. A small world of neuronal synchrony. Cereb Cortex 2008; 18:2891 - 2901
- Holmgren C, Harkany T, Svennenfors B, Zilberter Y. Pyramidal cell communication within local networks in layer 2/3 of rat neocortex. J Physiol 2003; 551:139 - 153
- Kampa BM, Letzkus JJ, Stuart GJ. Cortical feed-forward networks for binding different streams of sensory information. Nat Neurosci 2006; 9:1472 - 1473
- Shepherd GM, Stepanyants A, Bureau I, Chklovskii D, Svoboda K. Geometric and functional organization of cortical circuits. Nat Neurosci 2005; 8:782 - 790
- Song S, Sjostrom PJ, Reigl M, Nelson S, Chklovskii DB. Highly nonrandom features of synaptic connectivity in local cortical circuits. PLoS Biol 2005; 3:68
- Yoshimura Y, Dantzker JL, Callaway EM. Excitatory cortical neurons form fine-scale functional networks. Nature 2005; 433:868 - 873
- Takahashi N, Sasaki T, Matsumoto W, Matsuki N, Ikegaya Y. Circuit topology for synchronizing neurons in spontaneously active networks. Proc Natl Acad Sci USA 2010; 107:10244 - 10249
- Takahashi N, Sasaki T, Usami A, Matsuki N, Ikegaya Y. Watching neuronal circuit dynamics through functional multineuron calcium imaging (fMCI). Neurosci Res 2007; 58:219 - 225
- Sasaki T, Minamisawa G, Takahashi N, Matsuki N, Ikegaya Y. Reverse optical trawling for synaptic connections in situ. J Neurophysiol 2009; 102:636 - 643
- Abeles M. Corticonics: Neural Circuits of the Cerebral Cortex 1991; Cambridge Cambridge University Press
- Diesmann M, Gewaltig MO, Aertsen A. Stable propagation of synchronous spiking in cortical neural networks. Nature 1999; 402:529 - 533
- Ikegaya Y, Aaron G, Cossart R, Aronov D, Lampl I, et al. Synfire chains and cortical songs: temporal modules of cortical activity. Science 2004; 304:559 - 564
- Poulet JF, Petersen CC. Internal brain state regulates membrane potential synchrony in barrel cortex of behaving mice. Nature 2008; 454:881 - 885
- Greenberg DS, Houweling AR, Kerr JN. Population imaging of ongoing neuronal activity in the visual cortex of awake rats. Nat Neurosci 2008; 11:749 - 751