Abstract
Information between neurons and the target cells they innervated passes through sites of functional contact called synapses. How synapses form and are altered by sensory or cognitive experience is central to understand nervous system function. Studies of synapse formation and plasticity have concentrated on a few "model" synapses. The vertebrate neuromuscular junction (NMJ), the synapse between a motoneuron in the spinal cord and a skeletal muscle fiber, is one such model synapse. The extracellular matrix proteoglycan agrin plays an essential organizing role at the NMJ. Agrin is also present at some synapses in the brain and in other organs in the periphery, but its function outside the NMJ is unclear. The core signaling pathway for agrin at the NMJ, which is still incompletely defined, includes molecules specifically involved in this cascade and molecules used in other signaling pathways in many cells. Mitogen-activated protein kinases (MAPKs) are evolutionarily conserved components of intracellular signaling modules that control a myriad of cellular processes. This article reviews emerging evidence that suggests that MAPKs are involved in agrin signaling at the NMJ and in the putative functions of agrin in the formation of a subset of synapses in the brain.
Agrin
All chemical synapses exhibit a high concentration of neurotransmitter receptors in the postsynaptic membrane. This ensures that receptor concentration is not limiting for neurotransmission. Perhaps no other synapse possesses as high a density of receptors as the mature vertebrate neuromuscular junction (NMJ), the synapse between a motoneuron and a skeletal muscle fiber. At the NMJ, acetylcholine receptors (AChRs) are clustered at about 10,000/µm2.Citation1 Studies on regeneration of adult neuromuscular synapses suggested that signals in the extracellular matrix (ECM) in the synaptic cleft, the narrow space that separates the nerve terminal from the synaptic sarcolemma, direct the clustering of AChRs at the NMJ.Citation2,Citation3 Agrin was purified from ECM extracts of Torpedo electric organ on the basis of its ability to induce aggregation of AChRs on cultured myotubes.Citation4 Agrin is a large (∼400–600 kDa) heparan sulfate proteoglycan that accumulates at the synaptic basal lamina through its binding to laminin.Citation5,Citation6 Agrin is expressed by motoneurons and muscle fibers. However, cell-specific alternative splicing of exons encoding selective amino acid sequences in the C-terminal domain of the protein, generates isoforms with very different AChR clustering activities.Citation7 The isoforms produced by motoneurons, called agrin-z+ in mammals,Citation8 are endowed with clustering activity. Isoforms made by muscle cells (agrin-z−) essentially lack aggregating activity. Agrin-z− is widely expressed in the periphery, particularly in lung, kidney and immune cells.Citation9 Agrin-z− is also a major structural component of the basement membrane in the blood-brain barrier.Citation10 Agrin-z+ is restricted to neurons in the brain and spinal cord.Citation9
Mitogen-activated Protein kinases
Mitogen-activated protein kinases (MAPKs) are components of intracellular signaling modules that control a myriad of cellular processes.Citation11 MAPK modules have three critical protein kinase components. The most downstream component, the actual MAPK, is the S/T kinase that phosphorylates the various cellular proteins, such as transcription factors, cytoskeletal elements or other protein kinases, that are the targets of regulation by signaling cascades initiated at the cell surface. A MAPK is activated by an upstream MAPK kinase (MAP2K). MAP2Ks are unusual in that they phosphorylate both T and Y residues on MAPKs to activate them. A MAP2K is in turn activated by an MAP2K kinase (MAP3K). MAP3Ks are generally at the receiving end of activating signals coming from small, monomeric GTPases such as the Ras family or by other more elaborate mechanisms.Citation12,Citation13 In mammalian cells the prototypical MAPK module consists of the MAPKs ERK1/2 (extracellular-signal regulated kinases 1 and 2), the MAP2Ks MEK1/2 and the MAP3K Raf. Parallel MAPK modules assemble upstream of the MAPKs JNK (c-Jun NH2-terminal kinase) and p38.Citation11,Citation13,Citation14 ERK1/2 is implicated in the regulation of normal cellular responses to multiple growth factors and cytokines during proliferation and differentiation. In general, JNK and p38 play essential roles in the cellular responses to stress. In this review, I will concentrate on the role of ERK1/2, JNK and p38 in agrin signaling at the NMJ and in the brain.
MAPKs in Agrin Signaling at the NMJ
Gain- and loss-of-function manipulations of the agrin gene have established the essential role of agrin-z+ in the formation of the NMJ in vivo.Citation15–Citation18 To induce AChR clusters and other aspects of the postsynaptic apparatus, agrin-z+ binds Lrp4 (LDL receptor related protein 4),Citation19,Citation20 and activates the receptor tyrosine kinase MuSK (muscle specific kinase),Citation21 on the sarcolemma. In addition, Rapsyn (receptor-associated protein at the synapse) and Dok-7 (docking protein-7) are two intracellular proteins that critically mediate agrin's synaptogenic activity in vitro and in vivo.Citation22,Citation23 Rapsyn is an intracellular peripheral membrane protein that binds to AChRs and links them to the actin cytoskeleton. Dok-7 is a phosphotyrosine-binding protein that binds to activated MuSK. Herbst and BurdenCitation24 were the first to report a weak ERK1/2 activation after 30 min of agrin-z+ treatment in cultured myotubes. The ERK activity returned to control levels by 60 min. We recently reported that agrin-z+-induced ERK1/2 activation is quite robust if examined within 10 min after agrin addition, also returning to baseline by 60 min.Citation25 Thus, agrin induces a transient ERK1/2 activation in myotubes. This activation is Lrp4/MuSK-dependent as it was not observed in myotubes deficient for either of these proteins following agrin-z+ treatment.Citation25 Blocking agrin-induced ERK1/2 activation using highly selective chemical MEK1/2 inhibitors (U0126 and PD0325901,Citation26) failed to prevent AChR clustering. However, it led to a potentiation of agrin-induced AChR clustering.Citation25 This result suggested that agrin-induced ERK1/2 activation is dispensable for AChR clustering, but is part of a negative feedback loop that regulates the activity of agrin-z+. This conclusion was supported by our finding that PMA (phorbol 12-myristate 13-acetate), a classical inhibitor of agrin-induced AChR clustering in myotubes,Citation27 requires ERK1/2 activity for this effect.Citation25 Thus, PMA appears to co-opt the negative feedback loop mediated by agrin-induced ERK1/2 activation to inhibit AChR clustering. The biological significance of this negative feedback loop remains to be tested in vivo, however, one can speculate that by restraining the clustering activity of agrin, agrin-induced ERK1/2 activation may help localize the synaptogenic signal in space and time to ensure precise apposition of pre- and postsynaptic components, which is critical for synapse structure and function. In the near future it will be important to determine the downstream substrates of agrin-induced ERK1/2 activation. AChRs, Lrp4, MuSK, Rapsyn and Dok-7 should be examined as potential targets of phosphorylation by agrin-activated ERK1/2. Neuregulin-1 and the neurotrophins BDNF (brain-derived neurotrophic factor) and NT-4 can inhibit agrin-induced AChR clustering in cultured myotubes.Citation28,Citation29 All of these factors are known to engage MAPK pathways in many cell types. However, neuregulin-1 does not require ERK1/2 activation to inhibit agrin-induced AChR clustering,Citation25 while it remains to be investigated whether BDNF and NT-4 use MAPKs to exert their effect on AChR clustering.
Transcription of postsynaptic genes such as those encoding AChR subunits and MuSK is highly enriched at synaptic myonuclei. This mechanism supports the high density of postsynaptic proteins at the NMJ. The agrin-Lrp4-MuSK-Dok-7 pathway provides a signal that drives this synapse-specific expression. Prives and colleagues first showed that agrin could activate JNK transiently in cultured myotubes in a Rac/Cdc42-dependent fashion.Citation30 Although the Rho family GTPases Rac and Cdc42 appear to play important roles in agrin-induced AChR clustering in cultured myotubes,Citation30 the JNK activation they stimulate was shown dispensable for it.Citation31 However, Brenner and co-workers showed that JNK activation downstream of agrin-MuSK-Rac/Cdc42 is necessary for transcription of MuSK and AChR encoding genes both in myotubes and adult muscle fibers.Citation32 In this cascade, Rac/Cdc42 seem to activate the JNK-selective MAP2K7.Citation32 Thus, agrin-induced JNK activation plays a role in synapse-specific gene expression.
MAPKs in Agrin Signaling in the Brain
In the central nervous system, MAPKs have been implicated in regulating molecular mechanisms of synaptic plasticity underlying learning and memory.Citation33 The precise function of agrin in the CNS remains elusive. Agrin does not appear as indispensable for general interneuronal synapse formation as it is for neuromuscular synaptogenesis. However, agrin immunostaining colocalizes to a subset of excitatory synapses in the adult mouse brain, and most importantly, there is a selective 30% reduction in the number of excitatory synapses in the adult cortex in mice deficient for agrin in brain and other tissues but not in spinal cord (NMJ-rescued agrin-deficient mice).Citation34 In contrast to standard agrin-deficient mice that are stillborn,Citation17 these mice survive into early adulthood because they express agrin-z+ selectively in motoneurons.Citation34
The molecular mechanisms underpinning the brain phenotype of the rescued mice are unclear. An increase in the synaptic GTPase-activating protein SynGAP was the most significant change detected in these mice by western blot analysis of various excitatory synaptic components.Citation34 SynGAP is a negative regulator of ERK1/2 activation at excitatory synapses,Citation35 which suggests that synaptic levels of active ERK1/2 may be decreased in NMJ-rescued agrin-deficient mice. In addition, microarray gene expression analysis showed that the JNK-selective MAP2K7 was reduced in NMJ-rescued agrin-deficient brains,Citation34 which might also reduce JNK signaling. Thus, the reduction in synapses in the cortex of NMJ-rescued agrin-deficient mice correlated with a putative decrease in MAPK signaling.
In NMJ-rescued agrin-deficient mice, there were significant reductions in dendrite length, spine number per dendrite and spine density.Citation34 Spines are the sites on dendrites where postsynaptic specializations of most excitatory synapses reside. Filopodia—actin-rich, thin cytoplasmic projections—are thought of as the precursors of spines during excitatory interneuronal synaptogenesis.Citation36,Citation37 A reduction in dendritic filopodia during development would be expected to impact on the number of synapses that form. Agrin promotes formation of filopodia by a mechanism that involves MAPK activation.Citation38 Alternative promoter usage generates two agrin isoforms with different N-terminal domains encoded by two distinct first exons. One form is secreted and has an N-terminal domain that binds to laminin in the ECM,Citation5 while the other has a hydrophobic N-terminal domain that makes agrin into a type II transmembrane protein,Citation39 enriched in dendrites and axons. Antibody-induced clustering or overexpression of transmembrane agrin in neuronal and non-neuronal cells induces filopodia.Citation40–Citation43 This activity depends on domains in the N-terminal third of the agrin protein,Citation43,Citation44 not overlapping with the C-terminal domains responsible for AChR clustering. ERK1/2 is likely to mediate filopodia induction by transmembrane agrin as PD98059, a MEK1/2 inhibitor,Citation45 blocked this activity.Citation38 The ability to induce filopodia on neurons is not limited to transmembrane agrin. Sonderegger and colleagues have reported that secreted agrin bound to the brain ECM can be cleaved by the synaptic serine protease neurotrypsin in an electrical activity-dependent fashion. A neurotrypsin-released, 22-kDa fragment containing the C-terminal domain of agrin induced dendritic filopodia in hippocampal slices from juvenile mice. 22-kDa fragments from both secreted agrin-z+ and agrin-z− have filopodia-inducing activity.Citation46 Although Lrp4 and MuSK are expressed in the brain,Citation34,Citation47,Citation48 the evidence above suggests that these receptors do not mediate the ability of transmembrane or neurotrypsin-cleaved agrin to induce filopodia on dendrites. Smith and colleagues have shown that the α3 subunit of the Na+/K+-ATPase is a high-affinity receptor for C-terminal fragments of agrin-z+ and agrin-z− in neurons.Citation49 Thus, α3 Na+/K+-ATPase is a good candidate to mediate the filopodia-inducing activity of secreted agrin in neurons. However, this remains to be tested directly. Interestingly, this agrin receptor appears to engage ERK1/2 to induce c-fos expression in cultured cortical neurons.Citation50
In cultured hippocampal neurons, agrin-z+ has also been shown to stimulate the phosphorylation of the transcription factor CREB (cAMP-response element binding protein),Citation51 in an ERK1/2-dependent fashion.Citation52 Phospho-CREB would then stimulate the expression of downstream genes with direct roles in synaptogenesis and/or genes that regulate dendrite length or branching, which would affect synapse numbers indirectly. Agrin-z+-induced CREB phosphorylation may be Lrp4/MuSK-dependent because knockdown of MuSK expression reduces CREB phosphorylation in hippocampus.Citation47 However, further tests of this possibility are needed.
Soluble agrin-z+ inhibited axonal elongation in cultured hippocampal neurons via simultaneous activation of ERK1/2 and p38.Citation53 The role of MAPK activation in this process in vivo is unclear, as it appears in conflict with the phenotype of the NMJ-rescued agrin-deficient mice.Citation34
Conclusions and Perspectives
In summary, results to date indicate that agrin-z+ activates MAPK signaling through Lrp4/MuSK at the NMJ to negatively regulate its AChR clustering activity via ERK1/2, and to stimulate synapse specific transcription of postsynaptic genes via JNK (). In neurons, agrin activates ERK1/2 signaling to stimulate filopodial generation and to activate gene expression via CREB (). A role for agrin-induced JNK activation in the brain is possible but remains undefined. Transmembrane agrin may act as a receptor of a yet unknown ligand to mediate filopodial formation, while secreted agrin may use α3 Na+/K+-ATPase for this purpose. These activities directly or indirectly contribute to the formation of some excitatory synapses in the adult cortex during development, and may also mediate experience-dependent plasticity in the young adult hippocampus. Because PD98059 was the only MEK1/2 inhibitor used to support the notion that the induction of filopodia by transmembrane agrin,Citation38 and the phosphorylation of CREB by secreted agrin are ERK1/2-dependent,Citation52 it is possible that these depend on ERK5 instead, as this less studied MAPK can also be inhibited by PD98059.Citation54 It is expected that the next few years will yield research approaches that more directly test the above functions in vivo, both at the NMJ and in the brain. A big challenge will be to devise strategies that specifically address the involvement of MAPKs in agrin signaling for these evolutionarily conserved molecules participate in multiple other cellular events and signaling cascades.
Abbreviations
AChR | = | acetylcholine receptor |
BDNF | = | brain-derived neurotrophic factor |
CREB | = | cAMP-response element binding protein |
Dok-7 | = | docking protein-7 |
ECM | = | extracellular matrix |
ERK1/2 | = | extracellular-signal regulated kinases 1 and 2 |
JNK | = | c-jun NH2-terminal kinase |
Lrp4 | = | LDL receptor related protein 4 |
MAPK | = | mitogen-activated protein kinase |
MAP2K | = | MAPK kinase |
MAP3K | = | MAPK kinase kinase |
MuSK | = | muscle-specific kinase |
NMJ | = | neuromuscular junction |
PMA | = | phorbol 12-myristate 13-acetate |
Rapsyn | = | receptor-associated protein at the synapse |
Figures and Tables
Figure 1 Simplified diagrams for agrin-induced signaling. (A) At the NMJ secreted agrin-z+ binds to Lrp4 and activates (arrows) MuSK and Dok-7. Rac/Cdc42 is activated dowstream of Dok-7, which leads to AChR clustering, to activation of MAP2K7, JNK and ultimately to transcriptional stimulation of postsynaptic genes such as MuSK and AChR subunits. Agrin-z+-induced Lrp4/MuSK activation also stimulates MEK1/2 and ERK1/2 transiently (thinner arrows), which activates a negative feedback loop that inhibits AChR clustering (T-shape line). For much more detailed diagrams of agrin signaling at the NMJ please see reference Citation55. (B) In cortical and/or hippocampal neurons that bear excitatory synapses, soluble agrin-z+ may bind to Lrp4/MuSK, which might activate ERK1/2, which in turn would phosphorylate CREB. Phosphorylated CREB would stimulate transcription of genes that directly or indirectly influence synaptogenesis or synapse stability (i.e., “synaptic” genes). Electrical activity-dependent cleavage of full-length agrin (N-agrin-C) in the ECM by neurotrypsin releases a 22-kDa C-terminal fragment (agrin-C) that may bind α3 Na+/K+-ATPase (NKA), to stimulate filopodial formation. The involvement of MAPKs in this pathway is unknown (MAPKs?). An unknown ligand may bind to transmembrane agrin (TM agrin), leading to formation of dendritic filopodia via activation of ERK1/2 dowstream of the Src-family tyrosine kinase Fyn. (?) These steps have not been confirmed experimentally. For references see text.
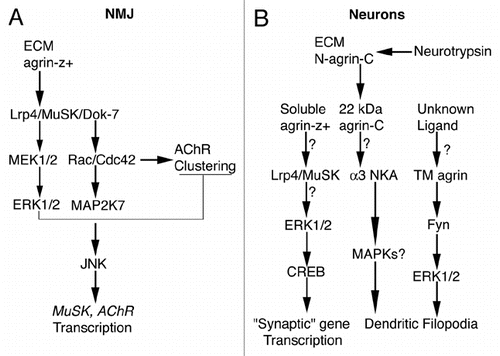
Acknowledgements
I thank Earl Godfrey for critically reading the manuscript. Supported by Texas A&M Health Science Center start-up funds.
References
- Salpeter MM, Loring RH. Nicotinic acetylcholine receptors in vertebrate muscle: properties, distribution and neural control. Prog Neurobiol 1985; 25:297 - 325
- Burden SJ, Sargent PB, McMahan UJ. Acetylcholine receptors in regenerating muscle accumulate at original synaptic sites in the absence of the nerve. J Cell Biol 1979; 82:412 - 425
- McMahan UJ, Slater CR. The influence of basal lamina on the accumulation of acetylcholine receptors at synaptic sites in regenerating muscle. J Cell Biol 1984; 98:1453 - 1473
- Nitkin RM, Smith MA, Magill C, Fallon JR, Yao YM, Wallace BG, et al. Identification of agrin, a synaptic organizing protein from Torpedo electric organ. J Cell Biol 1987; 105:2471 - 2478
- Denzer AJ, Brandenberger R, Gesemann M, Chiquet M, Ruegg MA. Agrin binds to the nerve-muscle basal lamina via laminin. J Cell Biol 1997; 137:671 - 683
- Burgess RW, Skarnes WC, Sanes JR. Agrin Isoforms with Distinct Amino Termini. Differential expression, localization and function. J Cell Biol 2000; 151:41 - 52
- Ruegg MA, Tsim KW, Horton SE, Kroger S, Escher G, Gensch EM, et al. The agrin gene codes for a family of basal lamina proteins that differ in function and distribution. Neuron 1992; 8:691 - 699
- Ferns MJ, Campanelli JT, Hoch W, Scheller RH, Hall Z. The ability of agrin to cluster AChRs depends on alternative splicing and on cell surface proteoglycans. Neuron 1993; 11:491 - 502
- Bezakova G, Ruegg MA. New insights into the roles of agrin. Nat Rev Mol Cell Biol 2003; 4:295 - 308
- Barber AJ, Lieth E. Agrin accumulates in the brain microvascular basal lamina during development of the blood-brain barrier. Dev Dyn 1997; 208:62 - 74
- Lawrence MC, Jivan A, Shao C, Duan L, Goad D, Zaganjor E, et al. The roles of MAPKs in disease. Cell Res 2008; 18:436 - 442
- Yoon S, Seger R. The extracellular signal-regulated kinase: multiple substrates regulate diverse cellular functions. Growth Factors 2006; 24:21 - 44
- Cuadrado A, Nebreda AR. Mechanisms and functions of p38 MAPK signalling. Biochem J 2010; 429:403 - 417
- Weston CR, Davis RJ. The JNK signal transduction pathway. Curr Opin Cell Biol 2007; 19:142 - 149
- Jones G, Meier T, Lichtsteiner M, Witzemann V, Sakmann B, Brenner HR. Induction by agrin of ectopic and functional postsynaptic-like membrane in innervated muscle. Proc Natl Acad Sci USA 1997; 94:2654 - 2659
- Cohen I, Rimer M, Lomo T, McMahan UJ. Agrin-induced postsynaptic-like apparatus in skeletal muscle fibers in vivo. Mol Cell Neurosci 1997; 9:237 - 253
- Gautam M, Noakes PG, Moscoso L, Rupp F, Scheller RH, Merlie JP, et al. Defective neuromuscular synaptogenesis in agrin-deficient mutant mice. Cell 1996; 85:525 - 535
- Burgess RW, Nguyen QT, Son YJ, Lichtman JW, Sanes JR. Alternatively spliced isoforms of nerve- and muscle-derived agrin: their roles at the neuromuscular junction. Neuron 1999; 23:33 - 44
- Kim N, Stiegler AL, Cameron TO, Hallock PT, Gomez AM, Huang JH, et al. Lrp4 is a receptor for Agrin and forms a complex with MuSK. Cell 2008; 135:334 - 342
- Zhang B, Luo S, Wang Q, Suzuki T, Xiong WC, Mei L. LRP4 serves as a coreceptor of agrin. Neuron 2008; 60:285 - 297
- Glass DJ, Bowen DC, Stitt TN, Radziejewski C, Bruno J, Ryan TE, et al. Agrin acts via a MuSK receptor complex. Cell 1996; 85:513 - 523
- Gautam M, Noakes PG, Mudd J, Nichol M, Chu GC, Sanes JR, et al. Failure of postsynaptic specialization to develop at neuromuscular junctions of rapsyn-deficient mice. Nature 1995; 377:232 - 236
- Okada K, Inoue A, Okada M, Murata Y, Kakuta S, Jigami T, et al. The muscle protein Dok-7 is essential for neuromuscular synaptogenesis. Science 2006; 312:1802 - 1805
- Herbst R, Burden SJ. The juxtamembrane region of MuSK has a critical role in agrin-mediated signaling. EMBO J 2000; 19:67 - 77
- Rimer M. Modulation of agrin-induced acetylcholine receptor clustering by extracellular signal-regulated kinases 1 and 2 in cultured myotubes. J Biol Chem 2010; 285:32370 - 32377
- Bain J, Plater L, Elliott M, Shpiro N, Hastie CJ, McLauchlan H, et al. The selectivity of protein kinase inhibitors: a further update. Biochem J 2007; 408:297 - 315
- Wallace BG. Regulation of agrin-induced acetylcholine receptor aggregation by Ca++ and phorbol ester. J Cell Biol 1988; 107:267 - 278
- Trinidad JC, Cohen JB. Neuregulin inhibits acetylcholine receptor aggregation in myotubes. J Biol Chem 2004; 279:31622 - 31628
- Wells DG, McKechnie BA, Kelkar S, Fallon JR. Neurotrophins regulate agrin-induced postsynaptic differentiation. Proc Natl Acad Sci USA 1999; 96:1112 - 1117
- Weston C, Yee B, Hod E, Prives J. Agrin-induced acetylcholine receptor clustering is mediated by the small guanosine triphosphatases Rac and Cdc42. J Cell Biol 2000; 150:205 - 212
- Luo ZG, Wang Q, Zhou JZ, Wang J, Luo Z, Liu M, et al. Regulation of AChR clustering by Dishevelled interacting with MuSK and PAK1. Neuron 2002; 35:489 - 505
- Lacazette E, Le Calvez S, Gajendra N, Brenner HR. A novel pathway for MuSK to induce key genes in neuromuscular synapse formation. J Cell Biol 2003; 161:727 - 736
- Ye X, Carew TJ. Small G protein signaling in neuronal plasticity and memory formation: the specific role of ras family proteins. Neuron 2010; 68:340 - 361
- Ksiazek I, Burkhardt C, Lin S, Seddik R, Maj M, Bezakova G, et al. Synapse loss in cortex of agrin-deficient mice after genetic rescue of perinatal death. J Neurosci 2007; 27:7183 - 7195
- Rumbaugh G, Adams JP, Kim JH, Huganir RL. SynGAP regulates synaptic strength and mitogen-activated protein kinases in cultured neurons. Proc Natl Acad Sci USA 2006; 103:4344 - 4351
- Ziv NE, Smith SJ. Evidence for a role of dendritic filopodia in synaptogenesis and spine formation. Neuron 1996; 17:91 - 102
- Trachtenberg JT, Chen BE, Knott GW, Feng G, Sanes JR, Welker E, et al. Long-term in vivo imaging of experience-dependent synaptic plasticity in adult cortex. Nature 2002; 420:788 - 794
- Ramseger R, White R, Kroger S. Transmembrane form agrin-induced process formation requires lipid rafts and the activation of Fyn and MAPK. J Biol Chem 2009; 284:7697 - 7705
- Neumann FR, Bittcher G, Annies M, Schumacher B, Kroger S, Ruegg MA. An alternative amino-terminus expressed in the central nervous system converts agrin to a type II transmembrane protein. Mol Cell Neurosci 2001; 17:208 - 225
- Uhm CS, Neuhuber B, Lowe B, Crocker V, Daniels MP. Synapse-forming axons and recombinant agrin induce microprocess formation on myotubes. J Neurosci 2001; 21:9678 - 9689
- Annies M, Bittcher G, Ramseger R, Loschinger J, Woll S, Porten E, et al. Clustering transmembrane-agrin induces filopodia-like processes on axons and dendrites. Mol Cell Neurosci 2006; 31:515 - 524
- McCroskery S, Chaudhry A, Lin L, Daniels MP. Transmembrane agrin regulates filopodia in rat hippocampal neurons in culture. Mol Cell Neurosci 2006; 33:15 - 28
- Porten E, Seliger B, Schneider VA, Woll S, Stangel D, Ramseger R, et al. The process-inducing activity of transmembrane agrin requires follistatin-like domains. J Biol Chem 2010; 285:3114 - 3125
- Lin L, McCroskery S, Ross JM, Chak Y, Neuhuber B, Daniels MP. Induction of filopodia-like protrusions by transmembrane agrin: role of agrin glycosaminoglycan chains and Rho-family GTPases. Exp Cell Res 2010; 316:2260 - 2277
- Alessi DR, Cuenda A, Cohen P, Dudley DT, Saltiel AR. PD 098059 is a specific inhibitor of the activation of mitogen-activated protein kinase kinase in vitro and in vivo. J Biol Chem 1995; 270:27489 - 27494
- Matsumoto-Miyai K, Sokolowska E, Zurlinden A, Gee CE, Luscher D, Hettwer S, et al. Coincident pre- and postsynaptic activation induces dendritic filopodia via neurotrypsin-dependent agrin cleavage. Cell 2009; 136:1161 - 1171
- Garcia-Osta A, Tsokas P, Pollonini G, Landau EM, Blitzer R, Alberini CM. MuSK expressed in the brain mediates cholinergic responses, synaptic plasticity and memory formation. J Neurosci 2006; 26:7919 - 7932
- Weatherbee SD, Anderson KV, Niswander LA. LDL-receptor-related protein 4 is crucial for formation of the neuromuscular junction. Development 2006; 133:4993 - 5000
- Hilgenberg LG, Su H, Gu H, O'Dowd DK, Smith MA. Alpha3Na+/K+-ATPase is a neuronal receptor for agrin. Cell 2006; 125:359 - 369
- Hilgenberg LG, Smith MA. Agrin signaling in cortical neurons is mediated by a tyrosine kinase-dependent increase in intracellular Ca2+ that engages both CaMKII and MAPK signal pathways. J Neurobiol 2004; 61:289 - 300
- Ji RR, Bose CM, Lesuisse C, Qiu D, Huang JC, Zhang Q, et al. Specific agrin isoforms induce cAMP response element binding protein phosphorylation in hippocampal neurons. J Neurosci 1998; 18:9695 - 9702
- Karasewski L, Ferreira A. MAPK signal transduction pathway mediates agrin effects on neurite elongation in cultured hippocampal neurons. J Neurobiol 2003; 55:14 - 24
- Ferreira A. Abnormal synapse formation in agrin-depleted hippocampal neurons. J Cell Sci 1999; 112:4729 - 4738
- Nishimoto S, Nishida E. MAPK signalling: ERK5 versus ERK1/2. EMBO Rep 2006; 7:782 - 786
- Wu H, Xiong WC, Mei L. To build a synapse: signaling pathways in neuromuscular junction assembly. Development 2010; 137:1017 - 1033