Abstract
Glycosaminoglycans, a major component of the extracellular matrix molecules in animal tissues, play important roles in various physiological events. Glycosaminoglycans are found in not only vertebrates but also many invertebrates, implying a conserved function in the animal kingdom. Here, we discuss the analysis of glycosaminoglycans in 11 invertebrate phyla focusing on structure as well as physiological functions elucidated in model organisms. Various sulfated structures of heparan sulfate are widely distributed from very primitive organisms to humans, indicating an involvement in fundamental biological processes. By contrast, chondroitin/dermatan sulfate from lower organisms is limited in its structural complexity and often associated with a particular function. The presence of hyaluronic acid outside of vertebrates has been reported only in a mollusk.
Glycosaminoglycans (GAGs) are complex polysaccharides with important roles in cell growth, differentiation, morphogenesis, cell migration and bacterial/viral infections.Citation1–Citation5 Major GAGs in vertebrates include heparin (Hep)/heparan sulfate (HS), chondroitin sulfate (CS)/dermatan sulfate (DS) and hyaluronic acid (HA). These GAG chains are composed of alternating units of an amino sugar, either N-acetyl-D-glucosamine (GlcNAc) or N-acetyl-D-galactosamine (GalNAc) and a hexuronic acid (HexA), either glucuronic acid (GlcA) or iduronic acid (IdoA). Although the polysaccharide backbones of GAGs are simple, repetitive linear chains, Hep/HS and CS/DS acquire a considerable degree of structural variability by extensive modifications involving sulfation and uronate epimerization (), which are the basis for the wide variety of domain structures with biological activities.
The presence of GAGs in various invertebrates as well as vertebrates has been well documented. Conversely, GAGs have never been reported in plants. Some bacterial strains synthesize polysaccharides with the same backbone structure as GAGs; however, these GAG-like polysaccharides are not attached to core proteins and are produced on the inner surface of the cytoplasmic membrane.Citation6 Thus, the origin of the bacterial polysaccharides is most likely different from that of the GAGs in metazoans.
Many reports have revealed the structural diversity in vertebrate GAGs. From fish and amphibians to mammals, the structure of vertebrate GAGs is extremely heterogeneous.Citation5,Citation7–Citation13 The biosynthesis of structurally complex GAGs is regulated and the diverse sulfation patterns are formed in an organ- and tissue-specific as well as temporally specific manner during growth and development.Citation8–Citation13 Mutational defects in most genes of GAG-biosynthetic enzymes in vertebrates lead to severe consequences,Citation1,Citation14 indicating that the expression of specific sulfated structures in GAGs plays an important role in life.
In the 1970s, Dietrich et al. performed the first systematic study on the distribution of GAGs in all classes of invertebrates.Citation15 However, it was difficult to quantitate GAGs or determine their disaccharide composition using analytical techniques of the time, and the saccharide structure of invertebrate GAGs had not been completely confirmed to be similar to that of vertebrate GAGs. Notably, nonsulfated chondroitin (Chn) and HA were almost indistinguishable, since the sugar stereo configuration, the substitution pattern of the backbone hydroxy groups and glycosidic linkages are identical between them. The only difference in structure between Chn and HA is the configuration at the C4-position of the hexosamine residue. Even the enzymes used for the depolymerization of these GAGs including CS lyases as well as testicular hyaluronidase act on both Chn/CS and HA.
Recently, sensitive microanalytical methods using fluorescent tagging and HPLCCitation16,Citation17 or capillary electrophoresisCitation18,Citation19 have been established and applied to the quantitative analysis of GAG disaccharides at low picomole levels. These methods can separate the enzyme digest of HA from that of Chn. The distribution of various types of GAGs has been elucidated in not only vertebrates but also invertebrates, from Platyhelminthes to Insecta. A wide variety of biological functions of GAGs have also been indicated (). Here we review the analysis of GAGs in 11 invertebrate phyla focusing on structure as well as physiological functions elucidated in model organisms ( and ).
Acidic Glycans in Porifera (no GAGs)
Marine sponges belong to the most basal metazoans. Investigations of GAGs in Porifera have proved the presence of acidic glycans, which mediate cellular interaction through multivalent carbohydrate-carbohydrate binding.Citation20,Citation21 This unique cell recognition system has been well studied,Citation22,Citation23 and the acidic glycans were found to have a novel structure distinct from known GAGs. To date, the classical GAGs, Hep/HS, CS/DS, Chn and HA, have not been found in Porifera.
GAGs in Platyhelminthes (CS and HS)
Planarians are an excellent model for studying the processes of regeneration. Autoradiography indicated the synthesis and deposition of GAGs in the basal lamina of the regenerating planarian in 1980.Citation24 However, due to a lack of analytical techniques to characterize the structure of the compounds, the presence of GAGs was not sufficiently demonstrated and no characterization was accomplished. Our preliminary structural analysis of planarian GAGs indicated that planarians synthesize HS with diverse sulfation patterns as well as CS composed mostly of 4-O-sulfated GalNAc-containing disaccharide units, followed by 6-O-sulfated and nonsulfated GalNAc-containing disaccharide units (Li F, Sengottuvelan M, Nandini CD and Sugahara K, unpublished data).
GAGs in Cnidaria (CS and HS)
Hydra is the most basal animal reported to contain GAGs. A considerable amount of CS and three orders of magnitude less HS were found in Hydra magnipapillata.Citation25 Neither DS nor HA has been detected. The nematode Caenorhabditis elegans contains only nonsulfated Chn chains (see “GAGs in Nematoda”), and CS chains of the fruit fly Drosophila melanogaster are nonsulfated or sulfated predominantly at the C4-position of GalNAc residues (see “GAGs in Hexapoda”) as shown below. Since D. melanogaster and C. elegans are considered higher forms of life, H. magnipapillata had been expected to have no CS chains or only nonsulfated Chn chains. However, Hydra CS turned out to be more heterogeneous in terms of sulfation than C. elegans Chn and D. melanpogaster CS. H. magnipapillata HS is modified by diverse sulfation including GlcN(2-N-sulfate), GlcN(6-O-sulfate) and HexA(2-O-sulfate) as found in vertebrates,Citation25 indicating that the highly sulfated structural characteristics of HS are maintained throughout evolution.
Recently, we have demonstrated an essential role for proteoglycans in the tubulation of the cnidarian nematocyst vesicle.Citation26 Nematocysts are the characteristic stinging organelles of the phylum Cnidaria, involved in prey capture, defense and locomotion. The morphogenesis of this extrusive organelle takes place inside a giant post-Golgi vesicle, which topologically represents the extracellular space. This process includes the formation of a complex collagenous capsule structure that elongates into a long tubule, which invaginates after its completion. We have shown that a nonsulfated Chn appears as a scaffold early in the morphogenesis of all nematocyst types in Hydra and Nematostella. Although CS isolated from whole hydra tissue was analyzed and found to contain nonsulfated as well as 4-O-sulfated and 6-O-sulfated GalNAc residues, only an unusual nonsulfated form of Chn could be detected in nematocysts, indicating that sulfated forms are present in the cellular tissue but not in nematocysts and that nonsulfated Chn plays a specific and indispensable role during nematocyst development.
HS could also be detected in the starlet sea anemone Nematostella vectensis.Citation27 It was demonstrated that members of the enzyme families necessary for the biosynthesis and modification of HS are present in Nematostella. In addition, biochemical analysis showed that the sulfation pattern of HS chains in Nematostella is unique and distinct from that in vertebrates.
GAGs in Nematoda (Chn and HS)
A considerable amount of nonsulfated Chn as well as a far less yet appreciable amount of HS were found in adult worms of C. elegans.Citation28 However, neither HA nor DS has been detected. 1H NMR spectroscopic analysis confirmed the fine structure of Chn to be distinct from that of HA.
The putative genes encoding a few HS glycosyltransferases as well as several HS sulfotransferases have been identified in the C. elegans genome, consistent with the observation that the existing HS is extensively sulfated.Citation28 Homologous genes of human and murine hyaluronan synthase as well as hyaluronidase are found in the C. elegans genome despite that C. elegans does not synthesize HA. Homologues of hyaluronan synthase may be associated with chitin synthesis as assumed from its homology to chitin synthase. The gene homologous to human hyaluronidase encodes a Chn-specific hydrolase, which does not depolymerize HA.Citation29,Citation30
The indispensable role of Chn in C. elegans has been well characterized.Citation31–Citation33 Chn, accounting for approximately 0.2% of dried nematodes, is crucial for both cytokinesis and morphogenesis during the development of C. elegans. The blocking of Chn synthesis with RNAi of a biosynthetic enzyme or by treatment with CS lyase, results in cytokinetic defects in early embryogenesis. Cytokinesis of the Chn-depleted embryos reverses and eventually cell division stops after the oscillation of cytokinesis and cell division, leading to early embryonic death. Mutations in the genes involved in the biosynthesis of GAGs in C. elegans have also been well investigated. A group of mutants with defects in vulval organogenesis, called squashed vulva or sqv, have been established. Most of the eight sqv genes mediate the biosynthesis of GAG chains.Citation34 Defective GAG formation caused by the mutations leads to a collapse of the vulval structure in C. elegans.
GAGs in Echinodermata (CS, DS and HS)
The structure and function of GAGs in sea urchin embryos have been characterized. CS/DS proteoglycans in the sea urchin embryo play an indispensable role in migration the of primary mesenchyme cells, according to observations following treatment with CS lyase or exposure to exogenous β-D-xyloside.Citation35 GAGs synthesized by embryos of the sea urchin Strongylocentrotus purpuratus contain a large amount of DS at the mesenchyme blastula-early gastrula stage.Citation36 The number of 4-O- and 6-O-disulfated GalNAc units in the DS is high, and chain length is considerably longer than that of vertebrate DS. Although adult sea urchin tissues also contain high concentrations of sulfated polysaccharides, DS is restricted to the body wall and its sulfation at the C4-position decreases markedly. The presence of HS in sea urchin embryos was demonstrated in 1982 based on sensitivity toward HS lyases.Citation37 However, its disaccharide composition has not been determined. Recently, the sea urchin (Hemicentrotus pulcherrimus) homolog of Sulf, a HS 6-O-endosulfatase, has been demonstrated to be involved in the regulation of vascular endothelial growth factor signaling and necessary for the early developmental process.Citation38 These reports suggest the important functions of both DS and HS during sea urchin development.
CS from the body wall of the sea cucumber Ludwigothurea grisea has a unique structural feature. It contains disaccharide units in which sulfated fucopyranoses are linked to the C3-position of the GlcA moiety.Citation39,Citation40 Besides the fucose branches, sea cucumber CS contains sulfate esters at the C3-position of GlcA. Nonsulfated, 6-O-sulfated and 4-O- and 6-O-disulfated disaccharide units are also present in the sea cucumber CS.Citation39 Strong anticoagulant activity has been observed in the fucosylated CS, and the sulfated α-L-fucose branches confer anticoagulant activity on the polysaccharide.Citation41 The effect of this unique CS on tumor metastasis and neutrophil recruitment has also been investigated.Citation42 The fucosylated CS inhibited colonization of adenocarcinoma cells in the lungs in an experimental model of metastasis as well as neutrophil recruitment during acute inflammation in experiments with mice. Both of these activities may depend on the inhibitory effect of the fucosylated CS on the function of P- and L-selectins. Removal of the sulfated fucose branches abolishes these inhibitory effects, indicating that the modifications on CS chains contribute to the biological activities. Clinical application of the CS from sea cucumber as an antithrombotic or antitumorous agent has been suggested.Citation43,Citation44
GAGs in Annelida (CS and HS)
Reports of GAGs in Annelida are limited to only a few papers. Recently, Amaral et al. have characterized the compartmental distribution of sulfated GAGs in the earthworm Eisenia andrei. HS and CS but not DS were purified from the whole body of adult earthworms. Although the composition of GAGs in juveniles is similar to that in adults, the newborn earthworms predominantly contained CS and no GAGs were detected in cocoons. The disaccharide composition of GAGs from earthworms was analyzed by Im et al. using liquid chromatography-electrospray ionization mass spectrometry.Citation46 The earthworm CS is composed of nonsulfated, 6-O-sulfated, and 2-O- and 4-O-disulfated disaccharide units, and its HS contained 2-N-sulfated, 2-N- and 6-O-disulfated, and 2-O-, 2-N-, and 6-O-trisulfated disaccharide units. The disaccharide composition of HS is very unusual, in that no N-acetyl group was observed.
GAGs in Tunicata (CS, DS and HS)
The major GAG reported in ascidians is DS, distinctive from mammalian DS in its sulfation at the C2-position of the IdoA residue and/or C6-position of the GalNAc residue. The predominant structure of DS from Ascidia nigra and Halocynthia pyriformis is “IdoA(2-O-sulfate)-GalNAc(6-O-sulfate)”Citation47 and “IdoA(2-O-sulfate)-GalNAc(4-O-sulfate),”Citation48 respectively. Although the former DS has no discernible anticoagulant activity, the latter DS is a potent anticoagulant that potentiates the inhibition of thrombin by heparin cofactor II, whose activity is approximately 10-fold higher than mammalian DS.Citation48 DS from Ascidia nigra was also demonstrated to bind and activate hepatocyte growth factor/scatter factor.Citation49 The presence of CS in ascidians has been reported by Xu et al. It was extracted from the ascidian tunic and shown to be composed of 6-O-sulfated (43%) and 4-O-disulfated (57%) disaccharide units. It showed anti-inflammatory activity by reducing the expression of inflammatory factors in a mouse model of inflammation of the skin. Recently, a set of genes homologous to the chondroitin/dermatan sulfotransferase genes has been identified in the genome of the ascidian Ciona intestinalis.Citation51 The number of sulfotransferase genes was 19, which is unexpectedly large, but consistent with the complex sulfation structure in ascidian DS.
HS was isolated from test cells of the ascidian Styela plicata and its structure was characterized.Citation52 The HS from the test cells was highly sulfated, and its major disaccharide unit was HexA(2-O-sulfate)-GlcN(2-N-, 6-O-disulfate) (approximately 53%), while the disulfated disaccharide, HexA(2-O-sulfate)-GlcN(2-N-sulfate), as well as the monosulfated disaccharides, HexA-GlcN(2-N-sulfate) and HexA-GlcNAc(6-O-sulfate), accounted for 22, 11 and 14%, respectively. In the developing larva, the test cells containing HS produced an external and hydrophilic layer for protection. In the adult ascidian, HS occurs as intracellular granules at the apical tip of epithelial cells surrounding the lumen of both the intestine and pharynx, in close contact with the external environment, a distribution similar to that of heparin in mammalian mast cells. The arrangement of HS in ascidians may reflect its participation in defensive mechanisms.Citation53
GAGs in Mollusca (CS, DS, HS and HA)
There are many reports on GAGs from Mollusca. This emphasizes the heterogeneity of GAGs in this phylum. The structural diversity of mollusk GAGs may even be greater than that of vertebrate GAGs.
An oversulfated CS from squid cartilage is available commercially and often utilized to study the biological activities of highly sulfated CS. The heavily modified CS also contains 3-O-sulfated GlcA as well as a β-D-Glc branch.Citation54–Citation58 CS preparations from various squid body parts have also been investigated,Citation58–Citation63 indicating not only a highly sulfated CS but also a nonsulfated Chn as a homogenous polymer.Citation59,Citation63 CS is more concentrated in the skin and fins than in other tissues in squid.Citation63
Highly sulfated HS preparations have also been isolated from various species of mollusks by Dietrich et al. and other groups.Citation64–Citation68 Like the mammalian HS, the mollusk HS is composed of blocks of low sulfated GlcNAc-containing disaccharides and highly sulfated GlcN(2-N-sulfate)-containing disaccharides. The structural analysis of a clam HS revealed the presence of a large amount of GlcN(3-O-sulfate)-containing saccharide, identified as a unique marker for the antithrombin-binding region of heparin/HS.Citation69 An HS preparation with a unique structure was isolated from the giant African snail Achatina fulica and named acharan sulfate.Citation70 The major repeating disaccharide sequence in acharan sulfate is IdoA(2-O-sulfate)-GlcNAc. Recently, a unique HS has been isolated from the bivalve Nodipecten nodosus (Linnaeus 1758).Citation71 The disaccharide units in the HS chain are composed of nonsulfated, 2-O-sulfated or 3-O-sulfated GlcA as well as 2-N-sulfated and/or 6-O-sulfated GlcN, and the anticoagulant activity of the mollusk HS was 5-fold lower than that of porcine heparin. Although the HS occurs at the basement membrane of the gill epithelium and throughout the extracellular matrix of the gills and mantle of the bivalve, its biological role in the mollusk has not been elucidated.
The presence of DS in a mollusk has also been proved. GAGs from the body of the marine clam Scapharca inaequivalvis are composed of 58% DS, 16% CS and 26% HS.Citation72 The major disaccharide units in DS from S. inaequivalvis are monosulfated at the C4-position of GalNAc [IdoA-GalNAc(4-O-sulfate) unit] and disulfated at the C2-position of IdoA as well as C4-position of GalNAc [IdoA(2-O-sulfate)-GalNAc(4-O-sulfate) unit].
The mollusk bivalve Mytilus galloprovincialis produces HA.Citation73 So far, the occurrence of HA is restricted to vertebrates, and no HA has been found in invertebrates except for this mollusk, although some pathogenic bacterial strains synthesize HA as a capsule polysaccharide to evade the immune system of their host.Citation74 Previously it was very difficult to demonstrate the presence of HA because it could not be distinguished from non-sulfated Chn due to its structural similarity. However, using modern techniques including NMR, HPLC and capillary electrophoresis, HA in the mollusk is clearly identifiable. The molecular weight of the mollusk HA was determined to be 200 kDa, which is much smaller than that of mammalian HA (103-104 kDa). The biological significance of HA in the mollusk has not been clarified yet.
GAGs in Chelicerata (CS and HS)
Chelicerata contains the classes Arachnida (spiders, scorpions, mites and ticks), Eurypterida (sea scorpions) and Xiphosura (horseshoe crabs). Reports on GAGs in Arachnida are limited. Although Cassaro and DietrichCitation15 showed the presence of HS and CS in the spider Nephila clavipes, their structure has not been characterized. GAGs in Eurypterida have never been reported to our knowledge. In contrast, CS in horseshoe crabs has been well investigated. The CS from horseshoe crab cartilage is designated CS-K, the horseshoe crab being also called the king crab. Unlike the cartilage CS from many other organisms, CS-K contains unique 3-O-sulfated GlcA residues. Seno and MurakamiCitation75 originally proposed the GlcA(3-O-sulfate) structure in the disulfated disaccharide unit isolated from CS-K after digestion with testicular hyaluronidase followed by chondroitinase ABC. The 3-O-sulfated GlcA structure was confirmed by solid chemical analysis with mass spectrometry and 1H NMR.Citation76 The 3-O-sulfated GlcA structure was later found in CS preparations from the body wall of sea cucumberCitation39 as well as the cartilage of squid.Citation55 CS-K also contains a novel 3-O-fucosylated GlcA residue,Citation77 which is observed in the CS preparation from connective tissues of sea cucumbers as well.Citation78
The study of GlcA(3-O-sulfate)-containing oligosaccharides revealed an unexpected enzymatic action of CS lyases. Digestion of CS containing GlcA(3-O-sulfate) with chondroitinase ABC destroyed the GlcA(3-O-sulfate)-containing disaccharide unit, though this structure is resistant to chondroitinase AC-II.Citation76 The degradation of GlcA(3-O-sulfate) by chondroitinase ABC was investigated in detail using electrospray ionization mass spectrometry.Citation79 The unsaturated disaccharide unit containing ΔHexA(3-O-sulfate) generated by the digestion is unstable because of the influence of the 3-O-sulfate group. The ΔHexA moiety is degraded in water and the end product is a GalNAc monosaccharide. The disaccharide composition of CS/DS from various sources has been analyzed after digestion with chondroitinase ABC. Since the GlcA(3-O-sulfate)-containing disaccharide in CS is decomposed into a monosaccharide by the action of chondroitinase ABC, disaccharide units containing a GlcA(3-O-sulfate) structure from various sources including mammals may have been overlooked.
HS chains have been found in the gills and endosternite cartilage of the king crab (Fongmoon D, Shetty AK and Sugahara K, unpublished data), although in much smaller amounts than CS.
GAGs in Crustacea (CS and HS)
Although HS and CS/DS are present in crabs, shrimps and lobsters, structural characterization is limited to the HS from shrimps.Citation80 The HS from the shrimp Penaeus brasiliensis is relatively small (8.5 kDa), although it is highly sulfated. Digestion of the shrimp HS with HS lyases yields di- and trisulfated disaccharides as major components. The shrimp HS inhibits the activity of factor Xa as well as thrombin mediated by heparin cofactor II to exhibit anticoagulant activity.
The influence of “habitat” on the structure of sulfated GAGs in Crustacea and Mollusca was investigated by Nader et al.Citation81 The amount and composition of sulfated GAGs were analyzed in species living with different levels of salinity. GAGs from the species living with high salt concentrations were more highly sulfated than those from the organisms living with low salt concentrations. The amount of the sulfated GAGs increased with the degree of salinity. Large amounts of highly sulfated GAGs may be needed for maintenance of the interaction between the sulfated GAGs and their targets in high salt environments.
GAGs in Hexapoda (CS and HS)
GAGs from D. melanogaster have been well characterized. Both CS and HS are detected in the organism, but not DS or HA. CS is composed of nonsulfated and 4-O-sulfated disaccharides, whereas the disaccharides from D. melanogaster HS bear 2-N-, 2-O- and/or 6-O-sulfate groups and include mono-, di- and trisulfated forms.Citation17 Toyoda et al. characterized the tissue- and stage-specific differences in both CS and HS composition as well as the GAGs from flies bearing mutations in the genes encoding GAG biosynthetic enzymes.Citation82 The findings demonstrated the utility of D. melanogaster as a model for studying the function and biosynthesis of GAGs in vivo.
GAGs from the mosquito Anopheles stephensi, known as a vector for Plasmodium parasites, the causative agents of malaria, have also been characterized.Citation83 CS as well as HS, but not DS or HA, are present in the mosquito. The disaccharide composition of HS and CS from salivary glands and midguts of the mosquito, which Plasmodium uses for invasion at different stages of the parasite's life cycle, has also been determined.
Concluding Remarks
HS is widely distributed from very primitive organisms to humans and diverse sulfation structures have been revealed in HS from lower animals such as H. magnipapillata and C. elegans. Since HS has maintained its structural features throughout evolution, it must be involved in fundamental biological processes.
In contrast, the structural complexity of CS/DS from lower organisms is limited. C. elegans synthesizes nonsulfated Chn but no CS/DS chains. CS might have complex functions in higher organisms. However, the Chn in C. elegans accounts for approximately 0.2% of the nematode (dry weight), and plays an indispensable role in cytokinesis during development. Only an unusual nonsulfated form of Chn in nematocysts of H. magnipapillata functions as a scaffold for the formation of nematocysts early in their morphogenesis, although CS isolated from whole hydra tissue contains a sulfated structure as well. DS, which is a CS isomer and epimerized at the C5-position of GlcA, has been reported in some invertebrates such as mollusks (Mollusca), ascidians (Tunicata) and sea urchins (Echinodermata), despite its absence in fruit flies (Insecta). Chn/CS/DS may have a specific role in a particular function of each organism. CS/DS which have unique biological functions attract much attention, because they are promising research seeds for developing medicines and potentially applicable to therapeutics.Citation5
Comparing the structural complexity of GAGs with evolutionary position reveals that higher animals do not necessarily produce more highly sulfated GAGs. It has been shown that GAGs from sharks, hagfish, king crabs, squid, mollusks, sea cucumbers and ascidians are characterized by oversulfated complex structures.Citation5,Citation39,Citation40,Citation47,Citation48,Citation64,Citation84 Generally, there is a tendency for aquatic species to contain more structural variety in their GAGs than terrestrial animals. GAGs from mollusks have particularly complicated structures with various modifications including high sulfation, uronate epimerizaton, sulfation at unusual positions, fucosylation and glucosidation. Further, their sulfation is influenced by the different “habitats” in which the organisms live. The degree of sulfation in GAG increases as a function of the salinity in the habitat of the organism. Since interactions between components of the extracellular matrix appear to occur at higher salt concentrations in marine invertebrates than in vertebrates, GAGs with increased charge density may be required.Citation36
Highly sulfated HS (heparin) may play a role in the protection of organisms against foreign bodies, such as bacteria and viruses.Citation85 In vertebrates, highly sulfated HS (heparin) is mainly found in tissues/organs that are in direct contact with the environment, for example skin, lung, and intestine. The highly sulfated and/or variously modified GAGs in mollusks may also have a role in defense against pathogens without involvement of the immune system. Fucose and glucose residues modified on the GAGs from mollusks and sea cucumbers may also be involved in the resistance to pathogens in organisms that lack an immune system. Further studies will help to elucidate how life has acquired the huge structural complexity found in GAGs during evolution.
Figures and Tables
Figure 1 The backbone structure of GAGs. CS or DS is composed of alternating disaccharide units of GlcA or IdoA and GalNAc, respectively. R1, R2, R3, R4 = −H or −SO3H. Modification with β-D-glucose at the R2 position has been found in squid CS. The presence of the α-L-fucose branch at the R4 position has been reported in the CS preparations from sea cucumber and king crab. HS is composed of alternating disaccharide units of GlcA or IdoA and GlcN. R1 = −COCH3 or −SO3H; R2, R3, R4, R5 = −H or −SO3H. Recently, sulfation at the R5 position was discovered in a bivalve HS.Citation71 HA is a linear polymer composed of GlcA and GlcNAc.
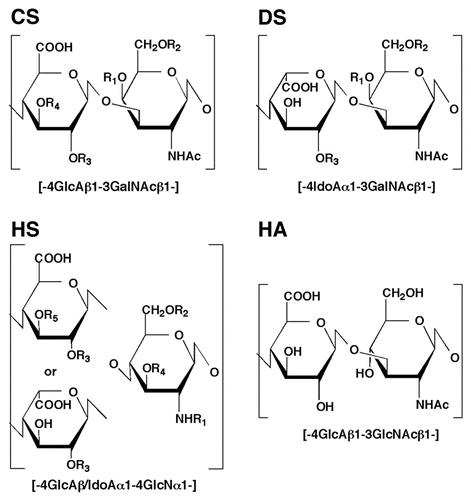
Table 1 Representative disaccharide composition of CD/DS from various species
Table 2 Representative disaccharide composition of HS from various species
Acknowledgements
This work was supported in part by Grants-in-aid for Scientific Research C-21590057 (to S.Y.), Scientific Research (B) 20390019 (to K.S.), and the Future Drug Discovery and Medical Care Innovation Program (to K.S.) from the Ministry of Education, Culture, Sports, Science and Technology of Japan (MEXT).
References
- Sugahara K, Kitagawa H. Recent advances in the study of the biosynthesis and functions of sulfated glycosaminoglycans. Curr Opin Struct Biol 2000; 10:518 - 527
- Esko JD, Selleck SB. Order out of chaos: assembly of ligand binding sites in heparan sulfate. Annu Rev Biochem 2001; 71:435 - 471
- Sugahara K, Mikami T, Uyama T, Mizuguchi S, Nomura K, Kitagawa H. Recent advances in the structural biology of chondroitin sulfate and dermatan sulfate. Curr Opin Struct Biol 2003; 13:612 - 620
- Rauch U, Kappler J. Chondroitin/dermatan sulfates in the central nervous system: their structures and functions in health and disease. Adv Pharmacol 2006; 53:337 - 356
- Yamada S, Sugahara K. Potential therapeutic application of chondroitin sulfate/dermatan sulfate. Curr Drug Discov Technol 2008; 5:289 - 301
- DeAngelis PL. Evolution of glycosaminoglycans and their glycosyltransferases: Implications for the extracellular matrices of animals and the capsules of pathogenic bacteria. Anat Rec 2002; 268:317 - 326
- Gomes PB, Dietrich CP. Distribution of heparin and other sulfated glycosaminoglycans in vertebrates. Comp Biochem Physiol B 1982; 73:857 - 863
- Maccarana M, Sakura Y, Tawada A, Yoshida K, Lindahl U. Domain structure of heparan sulfates from bovine organs. J Biol Chem 1996; 271:17804 - 17810
- Kitagawa H, Tsutsumi K, Tone Y, Sugahara K. Developmental regulation of the sulfation profile of chondroitin sulfate chains in the chicken embryo brain. J Biol Chem 1997; 272:31377 - 31381
- Warda M, Toida T, Zhang F, Sun P, Munoz E, Xie J, et al. Isolation and characterization of heparan sulfate from various murine tissues. Glycoconj J 2006; 23:555 - 563
- Zhang F, Zhang Z, Thistle R, McKeen L, Hosoyama S, Toida T, et al. Structural characterization of glycosaminoglycans from zebrafish in different ages. Glycoconj J 2009; 26:211 - 218
- Yamada S, Onishi M, Fujinawa R, Tadokoro Y, Okabayashi K, Asashima M, et al. Structural and functional changes of sulfated glycosaminoglycans in Xenopus laevis during embryogenesis. Glycobiology 2009; 19:488 - 498
- Ohtake-Niimi S, Kondo S, Ito T, Kakehi S, Ohta T, Habuchi H, et al. Mice deficient in N-acetylgalactosamine 4-sulfate 6-O-sulfotransferase are unable to synthesize chondroitin/dermatan sulfate containing N-acetylgalactosamine 4,6-bissulfate residues and exhibit decreased protease activity in bone marrow-derived mast cells. J Biol Chem 2010; 285:20793 - 20805
- Uyama T, Kitagawa H, Sugahara K. Kamerling JP. Biosynthesis of glycosaminoglycans and proteoglycans. Comprehensive Glycoscience 2007; 3:Amsterdam Elsevier 79 - 104
- Cássaro CMF, Dietrich CP. Distribution of sulfated mucopolysaccharides in invertebrates. J Biol Chem 1977; 252:2254 - 2261
- Kinoshita A, Sugahara K. Microanalysis of glycosaminoglycan- derived oligosaccharides labeled with a fluorophore-2-aminobenzamide by high-performance liquid chromatography: application to disaccharide composition analysis and exosequencing of oligosaccharides. Anal Biochem 1999; 269:367 - 378
- Toyoda H, Kinoshita-Toyoda A, Selleck SB. Structural analysis of glycosaminoglycans in Drosophila and Caenorhabditis elegans and demonstration that toutvelu, a Drosophila gene related to EXT tumor suppressors, affects heparan sulfate in vivo. J Biol Chem 2000; 275:2269 - 2275
- Karamanos NK, Axelsson S, Vanky P, Tzanakakis GN, Hjerpe A. Determination of hyaluronan and galactosaminoglycan disaccharides by high-performance capillary electrophoresis at the attomole level. Applications to analyses of tissue and cell culture proteoglycans. J Chromatogr A 1995; 696:295 - 305
- Kitagawa H, Kinoshita A, Sugahara K. Microanalysis of glycosaminoglycan-derived disaccharides labeled with the fluorophore-2-aminoacridone by capillary electrophoresis and high-performance liquid chromatography. Anal Biochem 1995; 232:114 - 121
- Coombe DR, Jakobsen KB, Parish CR. A role for sulfated polysaccharide recognition in sponge cell aggregation. Exp Cell Res 1987; 170:381 - 401
- Parish CR, Jakobsen KB, Coombe DR, Bacic A. Isolation and characterization of cell adhesion molecules from the marine sponge, Ophlitaspongia tenuis. Biochim Biophys Acta 1991; 1073:56 - 64
- Misevic GN, Burger MM. Carbohydrate-carbohydrate interactions of a novel acidic glycan can mediate sponge cell adhesion. J Biol Chem 1993; 268:4922 - 4929
- Fernàndez-Busquets X, Burger MM. Circular proteoglycans from sponges: first members of the spongican family. Cell Mol Life Sci 2003; 60:88 - 112
- Hori I. Localization of newly synthesized precursors of basal lamina in the regenerating planarian as revealed by autoradiography. Tissue Cell 1980; 12:513 - 521
- Yamada S, Morimoto H, Fujisawa T, Sugahara K. Glycosaminoglycans in Hydra magnipapillata (Hydrozoa, Cnidaria): demonstration of chondroitin in the developing nematocyst, the sting organelle and structural characterization of glycosaminoglycans. Glycobiology 2007; 17:886 - 894
- Adamczyk P, Zenkert C, Balasubramanian PG, Yamada S, Murakoshi S, Sugahara K, et al. A non-sulfated chondroitin stabilizes membrane tubulation in cnidarian organelles. J Biol Chem 2010; 285:25613 - 25623
- Feta A, Do AT, Rentzsch F, Technau U, Kusche-Gullberg M. Molecular analysis of heparan sulfate biosynthetic enzyme machinery and characterization of heparan sulfate structure in Nematostella vectensis. Biochem J 2009; 419:585 - 593
- Yamada S, Van Die I, Van den Eijnden DH, Yokota A, Kitagawa H, Sugahara K. Demonstration of glycosaminoglycans in Caenorhabditis elegans. FEBS Lett 1999; 459:327 - 331
- Kaneiwa T, Yamada S, Mizumoto S, Montaño AM, Mitani S, Sugahara K. Identification of a novel chondroitin hydrolase in Caenorhabditis elegans. J Biol Chem 2008; 283:14971 - 14979
- Yamada S, Mizumoto S, Sugahara K. Chondroitin hydrolase in Caenorhabditis elegans. Trends Glycosci Glycotechnol 2009; 21:149 - 162
- Hwang HY, Olson SK, Esko JD, Horvitz HR. Caenorhabditis elegans early embryogenesis and vulval morphogenesis require chondroitin biosynthesis. Nature 2003; 423:439 - 443
- Mizuguchi S, Uyama T, Kitagawa H, Nomura KH, Dejima K, Gengyo-Ando K, et al. Chondroitin proteoglycans are involved in cell division of Caenorhabditis elegans. Nature 2003; 423:443 - 448
- Izumikawa T, Kitagawa H, Mizuguchi S, Nomura KH, Nomura K, Tamura J, et al. Nematode chondroitin polymerizing factor showing cell-/organ-specific expression is indispensable for chondroitin synthesis and embryonic cell division. J Biol Chem 2004; 279:53755 - 53761
- Bulik DA, Robbins PW. The Caenorhabditis elegans sqv genes and functions of proteoglycans in development. Biochim Biophys Acta 2002; 1573:247 - 257
- Lane MC, Solursh M. Primary mesenchyme cell migration requires a chondroitin sulfate/dermatan sulfate proteoglycan. Dev Biol 1991; 143:389 - 397
- Vilela-Silva AC, Werneck CC, Valente AP, Vacquier VD, Mourão PA. Embryos of the sea urchin Strongylocentrotus purpuratus synthesize a dermatan sulfate enriched in 4-O- and 6-O-disulfated galactosamine units. Glycobiology 2001; 11:433 - 440
- Solursh M, Katow H. Initial characterization of sulfated macromolecules in the blastocoels of mesenchyme blastulae of Strongylocentrotus purpuratus and Lytechinus pictus. Dev Biol 1982; 94:326 - 336
- Fujita K, Takechi E, Sakamoto N, Sumiyoshi N, Izumi S, Miyamoto T, et al. HpSulf, a heparan sulfate 6-O-endosulfatase, is involved in the regulation of VEGF signaling during sea urchin development. Mech Dev 2010; 127:235 - 245
- Vieira RP, Mulloy B, Mourão PA. Structure of a fucose-branched chondroitin sulfate from sea cucumber. Evidence for the presence of 3-O-sulfo-beta-D-glucuronosyl residues. J Biol Chem 1991; 266:13530 - 13536
- Medeiros GF, Mendes A, Castro RA, Baú EC, Nader HB, Dietrich CP. Distribution of sulfated glycosaminoglycans in the animal kingdom: widespread occurrence of heparin-like compounds in invertebrates. Biochim Biophys Acta 2000; 1475:287 - 294
- Mourão PA, Pereira MS, Pavão MS, Mulloy B, Tollefsen DM, Mowinckel MC, et al. Structure and anticoagulant activity of a fucosylated chondroitin sulfate from echinoderm. Sulfated fucose branches on the polysaccharide account for its high anticoagulant action. J Biol Chem 1996; 271:23973 - 23984
- Borsig L, Wang L, Cavalcante MC, Cardilo-Reis L, Ferreira PL, Mourão PA, et al. Selectin blocking activity of a fucosylated chondroitin sulfate glycosaminoglycan from sea cucumber. Effect on tumor metastasis and neutrophil recruitment. J Biol Chem 2007; 282:14984 - 14991
- Fonseca RJ, Mourão PA. Fucosylated chondroitin sulfate as a new oral antithrombotic agent. Thromb Haemost 2006; 96:822 - 829
- Lu Y, Wang BL. The research progress of antitumorous effectiveness of Stichopus japonicus acid mucopolysaccharide in north of China. Am J Med Sci 2009; 337:195 - 198
- Amaral HB, Mateus SH, Ferreira LC, Ribeiro CC, Palumbo-Junior A, Domingos MA, et al. Localization and characterization of sulfated glycosaminoglycans in the body of the earthworm Eisenia andrei (Oligochaeta, Annelida). Acta Histochem 2011; 113:442 - 452
- Im AR, Park Y, Sim JS, Zhang Z, Liu Z, Linhardt RJ, et al. Glycosaminoglycans from earthworms (Eisenia andrei). Glycoconj J 2010; 27:249 - 257
- Pavão MS, Mourão PA, Mulloy B, Tollefsen DM. A unique dermatan sulfate-like glycosaminoglycan from ascidian. Its structure and the effect of its unusual sulfation pattern on anticoagulant activity. J Biol Chem 1995; 270:31027 - 31036
- Pavão MS, Aiello KR, Werneck CC, Silva LC, Valente AP, Mulloy B, et al. Highly sulfated dermatan sulfates from Ascidians. Structure versus anticoagulant activity of these glycosaminoglycans. J Biol Chem 1998; 273:27848 - 27857
- Catlow KR, Deakin JA, Wei Z, Delehedde M, Fernig DG, Gherardi E, et al. Interactions of hepatocyte growth factor/scatter factor with various glycosaminoglycans reveal an important interplay between the presence of iduronate and sulfate density. J Biol Chem 2008; 283:5235 - 5248
- Xu CX, Jin H, Chung YS, Shin JY, Lee KH, Beck GR Jr, et al. Chondroitin sulfate extracted from ascidian tunic inhibits phorbol ester-induced expression of Inflammatory factors VCAM-1 and COX-2 by blocking NFkappaB activation in mouse skin. J Agric Food Chem 2008; 56:9667 - 9675
- Tetsukawa A, Nakamura J, Fujiwara S. Identification of chondroitin/dermatan sulfotransferases in the protochordate, Ciona intestinalis. Comp Biochem Physiol B Biochem Mol Biol 2010; 157:205 - 212
- Cavalcante MC, Mourão PA, Pavão MS. Isolation and characterization of a highly sulfated heparan sulfate from ascidian test cells. Biochim Biophys Acta 1999; 1428:77 - 87
- Cavalcante MC, Allodi S, Valente AP, Straus AH, Takahashi HK, Mourão PA, et al. Occurrence of heparin in the invertebrate Styela plicata (Tunicata) is restricted to cell layers facing the outside environment. An ancient role in defense?. J Biol Chem 2000; 275:36189 - 36196
- Habuchi O, Sugiura K, Kawai N. Glucose branches in chondroitin sulfates from squid cartilage. J Biol Chem 1977; 252:4570 - 4576
- Kinoshita A, Yamada S, Haslam SM, Morris HR, Dell A, Sugahara K. Novel tetrasaccharides isolated from squid cartilage chondroitin sulfate E contain unusual sulfated disaccharide units GlcA(3-O-sulfate)beta1-3GalNAc(6-O-sulfate) or GlcA(3-O-sulfate)beta1-3GalNAc. J Biol Chem 1997; 272:19656 - 19665
- Kinoshita A, Yamada S, Haslam SM, Morris HR, Dell A, Sugahara K. Isolation and structural determination of novel sulfated hexasaccharides from squid cartilage chondroitin sulfate E that exhibits neuroregulatory activities. Biochemistry 2001; 40:12654 - 12665
- Kinoshita-Toyoda A, Yamada S, Haslam SM, Khoo KH, Sugiura M, Morris HR, et al. Structural determination of five novel tetrasaccharides containing 3-O-sulfated D-glucuronic acid and two rare oligosaccharides containing a beta-D-glucose branch isolated from squid cartilage chondroitin sulfate E. Biochemistry 2004; 43:11063 - 11074
- Shetty AK, Kobayashi T, Mizumoto S, Narumi M, Kudo Y, Yamada S, et al. Isolation and characterization of a novel chondroitin sulfate from squid liver integument rich in N-acetylgalactosamine(4,6-disulfate) and glucuronate(3-sulfate) residues. Carbohydr Res 2009; 344:1526 - 1532
- Karamanos NK, Aletras AJ, Antonopoulos CA, Tsegenidis T, Tsiganos CP, Vynios DH. Extraction and fractionation of proteoglycans from squid skin. Biochim Biophys Acta 1988; 966:36 - 43
- Karamanos KK, Aletras AJ, Antonopoulos CA, Hjerpe A, Tsiganos CP. Chondroitin proteoglycans from squid skin. Isolation, characterization and immunological studies. Eur J Biochem 1990; 192:33 - 38
- Vynios DH, Tsiganos CP. Squid proteoglycans: isolation and characterization of three populations from cranial cartilage. Biochim Biophys Acta 1990; 1033:139 - 147
- Karamanos NK, Manouras A, Tsegenidis T, Antonopoulos CA. Isolation and chemical study of the glycosaminoglycans from squid cornea. Int J Biochem 1991; 23:67 - 72
- Tamura J, Arima K, Imazu A, Tsutsumishita N, Fujita H, Yamane M, et al. Sulfation patterns and the amounts of chondroitin sulfate in the diamond squid, Thysanoteuthis rhombus. Biosci Biotechnol Biochem 2009; 73:1387 - 1391
- Dietrich CP, Nader HB, de Paiva JF, Santos EA, Holme KR, Perlin AS. Heparin in molluscs: chemical, enzymatic degradation and 13C and 1H NMR spectroscopical evidence for the maintenance of the structure through evolution. Int J Biol Macromol 1989; 11:361 - 366
- Ferreira TM, Medeiros MG, Dietrich CP, Nader HB. Structure of heparan sulfate from the fresh water mollusc Anomantidae sp: sequencing of its disaccharide units. Int J Biochem 1993; 25:1219 - 1225
- Kim YS, Ahn MY, Wu SJ, Kim DH, Toida T, Teesch LM, et al. Determination of the structure of oligosaccharides prepared from acharan sulfate. Glycobiology 1998; 8:869 - 877
- Luppi E, Cesaretti M, Volpi N. Purification and characterization of heparin from the Italian clam Callista chione. Biomacromolecules 2005; 6:1672 - 1678
- Volpi N, Maccari F. Glycosaminoglycan composition of the large freshwater mollusc bivalve Anodonta anodonta. Biomacromolecules 2005; 6:3174 - 3180
- Pejler G, Danielsson A, Björk I, Lindahl U, Nader HB, Dietrich CP. Structure and antithrombin-binding properties of heparin isolated from the clams Anomalocardia brasiliana and Tivela mactroides. J Biol Chem 1987; 262:11413 - 11421
- Chi L, Munoz EM, Choi HS, Ha YW, Kim YS, Toida T, et al. Preparation and structural determination of large oligosaccharides derived from acharan sulfate. Carbohydr Res 2006; 341:864 - 869
- Gomes AM, Kozlowski EO, Pomin VH, de Barros CM, Zaganeli JL, Pavão MS. Unique extracellular matrix heparan sulfate from the bivalve Nodipecten nodosus (Linnaeus 1758) safely inhibits arterial thrombosis after photochemically induced endothelial lesion. J Biol Chem 2010; 285:7312 - 7323
- Volpi N, Maccari F. Structural characterization and antithrombin activity of dermatan sulfate purified from marine clam Scapharca inaequivalvis. Glycobiology 2009; 19:356 - 367
- Volpi N, Maccari F. Purification and characterization of hyaluronic acid from the mollusc bivalve Mytilus galloprovincialis. Biochimie 2003; 85:619 - 625
- Wessels MR, Moses AE, Goldberg JB, DiCesare TJ. Hyaluronic acid capsule is a virulence factor for mucoid group A streptococci. Proc Natl Acad Sci USA 1991; 88:8317 - 8321
- Seno N, Murakami K. Structure of disulfated disaccharides from chondroitin polysulfates, chondroitin sulfate D and K. Carbohydr Res 1982; 103:190 - 194
- Sugahara K, Tanaka Y, Yamada S, Seno N, Kitagawa H, Haslam SM, et al. Novel sulfated oligosaccharides containing 3-O-sulfated glucuronic acid from king crab cartilage chondroitin sulfate K. Unexpected degradation by chondroitinase ABC. J Biol Chem 1996; 271:26745 - 26754
- Kitagawa H, Tanaka Y, Yamada S, Seno N, Haslam SM, Morris HR, et al. A novel pentasaccharide sequence GlcA(3-sulfate)(beta1-3)GalNAc(4-sulfate)(beta1-4) (Fucalpha1-3)GlcA(beta1-3)GalNAc(4-sulfate) in the oligosaccharides isolated from king crab cartilage chondroitin sulfate K and its differential susceptibility to chondroitinases and hyaluronidase. Biochemistry 1997; 36:3998 - 4008
- Vieira RP, Mourão PA. Occurrence of a unique fucosebranched chondroitin sulfate in the body wall of a sea cucumber. J Biol Chem 1988; 263:18176 - 18183
- Fongmoon D, Shetty AK, Basappa, Yamad S, Sugiura M, Kongtawelert P, et al. Chondroitinase-mediated degradation of rare 3-O-sulfated glucuronic acid in functional oversulfated chondroitin sulfate K and E. J Biol Chem 2007; 282:36895 - 36904
- Dietrich CP, Paiva JF, Castro RA, Chavante SF, Jeske W, Fareed J, et al. Structural features and anticoagulant activities of a novel natural low molecular weight heparin from the shrimp Penaeus brasiliensis. Biochim Biophys Acta 1999; 1428:273 - 283
- Nader HB, Medeiros MGL, Paiva JF, Paiva VMP, Jerônimo SMB, Ferreira TMPC, et al. A correlation between the sulfated glycosaminoglycan concentration and degree of salinity of the “habitat” in fifteen species of the classes Crustacea, Pelecypoda and Gastropoda. Comp Biochem Physiol 1983; 76:433 - 436
- Toyoda H, Kinoshita-Toyoda A, Fox B, Selleck SB. Structural analysis of glycosaminoglycans in animals bearing mutations in sugarless, sulfateless and tout-velu. Drosophila homologues of vertebrate genes encoding glycosaminoglycan biosynthetic enzymes. J Biol Chem 2000; 275:21856 - 21861
- Sinnis P, Coppi A, Toida T, Toyoda H, Kinoshita-Toyoda A, Xie J, et al. Mosquito heparan sulfate and its potential role in malaria infection and transmission. J Biol Chem 2007; 282:25376 - 25384
- Sugahara K, Yamada S. Structure and function of oversulfated chondroitin sulfate variants: unique sulfation patterns and neuroregulatory activities. Trends Glycosci. Glycotechnol 2000; 12:321 - 349
- Nader HB, Dietrich CP. Lane DA, Lindahl U. Natural occurrence and possible biological role of heparin. Heparin 1989; London Edward Arnold 81 - 96
- Karamanos NK, Manouras A, Tsegenidis T, Antonopoulos CA. Isolation and chemical study of the glycosaminoglycans from squid cornea. Int J Biochem 1991; 23:67 - 72
- Lee KB, Kim JS, Kwak ST, Sim W, Kwak JH, Kim YS. Isolation and identification of chondroitin sulfates from the mud snail. Arch Pharm Res 1998; 21:555 - 558
- Volpi N, Mucci A. Characterization of a low-sulfated chondroitin sulfate from the body of Viviparus ater (mollusca gastropoda). Modification of its structure by lead pollution. Glycoconj J 1998; 15:1071 - 1078
- Cesaretti M, Luppi E, Maccari F, Volpi N. Isolation and characterization of a heparin with high anticoagulant activity from the clam Tapes phylippinarum: evidence for the presence of a high content of antithrombin III binding site. Glycobiology 2004; 14:1275 - 1284