Abstract
During cerebral cortical development, post-mitotic neurons exhibit a multi-step migration. The locomotion mode covers most of the neuronal migration path. Although for many decades, locomoting neurons have been known to migrate along radial glial fibers, how the cortical locomoting neurons attach to and migrate along radial glial fibers was largely unknown. We recently reported that N-cadherin is required for cortical neuronal migration in vivo. Knockdown or dominant negative inhibition of N-cadherin results in severe neuronal migration defects. Furthermore, suppression of Rab5-dependent endocytosis increases cell surface levels of N-cadherin and perturbs neuronal migration. We showed here that N-cadherin overexpression, which would mimic Rab5 suppression, weakly suppressed neuronal migration, suggesting that excess N-cadherin also disturbs neuronal migration. Interestingly, however, N-cadherin-knockdown and overexpression in neurons resulted in different morphologies. While N-cadherin-overexpressing cells closely attached to the radial glial fibers similar to control or Rab5-knockdown cells, N-cadherin knockdown weakened the attachment as the average distance between the soma and radial glial fibers was significantly increased. Taken together, these findings suggest that N-cadherin controls the neuronal attachment to radial glial fibers and that N-cadherin-mediated adhesion complexes are reconstituted through Rab GTPases-dependent endocytic pathways to maintain the proper surface N-cadherin level and to promote neuronal migration.
During the development of the mammalian cerebral cortex, post-mitotic neurons, generated near the ventricle, migrate radially toward the pial surface to form the six-layered cortical structure whose disruption leads to several neurological disorders, such as lissencephaly and periventricular heterotopia.Citation1–Citation4 Neuronal migration consists of several steps with complex morphological changes: multipolar mode, locomotion mode and terminal translocation mode.Citation5,Citation6 After exhibiting multipolar morphologies, neurons form an axon and a thick process extending toward the pial surface, called a leading process, in the intermediate zone. The polarized bipolar-shaped neurons with a leading process, called locomoting neurons, migrate over a long distance from the intermediate zone to reach near the top of the cortical plate. At the final phase of neuronal migration, the locomoting neurons change from their migration mode into the terminal translocation mode. Among the migration modes, the locomotion mode is the main contributor to neuronal migration and cortical layer formation because it covers most of the migration route.Citation7 It has been known for many decades that locomoting neurons migrate long distances along the radial glial fibers, pia-directed long processes of neural progenitors.Citation8 However, how the locomoting neurons attach to and migrate along the radial glial fibers remained largely unknown.
Previous in vitro analyses using cultured cerebellar neurons and Bergmann glia showed that astrotactin (Astn1) is required for Bergmann glial fiber-dependent migration of cerebellar granule neurons.Citation9–Citation11 Furthermore, the cerebellum of Astn1-deficient mice is approximately 10% smaller than that of wild type and delayed migration of cerebellar granule neurons is found in vivo.Citation12 On the other hand, neuronal migration defects in the cerebral cortex have not been reported in mice lacking Astn1. In addition, Astn2, which regulates the cell surface expression of Astn1, is predominantly expressed in the cerebellum.Citation13 These observations suggest that the interaction between the locomoting neurons and radial glial fibers in the developing cerebral cortex are mediated by other cell adhesion molecule(s).
Recently we reported that N-cadherin is expressed in both migrating neurons and radial glial fibers and that in vivo electroporation-mediated RNA interference (in vivo RNAi)Citation14 for N-cadherin, a cell-to-cell adhesion molecule, inhibited pia-directed neuronal migration in the mouse developing cerebral cortex.Citation15 Although previous studies indicated roles for N-cadherin in neural progenitors,Citation16–Citation18 the expression of a dominant negative form of N-cadherin driven by a neuronspecific promoter as well as a ubiquitous promoter also perturbed neuronal migration. These data indicate that N-cadherin is required for cortical neuronal migration, independent of its functions in neural progenitors. In this Addendum paper, we focus on the roles of N-cadherin in the interaction between locomoting neurons and radial glial fibers.
Using in utero electroporation,Citation19 an N-cadherin-knockdown vector (NC-sh1023) in conjunction with an EGFP-expressing vector were electroporated into mouse developing cerebral cortices at embryonic day 14 (E14), and the electroporated brains were fixed at E17 and stained with anti-EGFP and anti-Nestin (a marker for radial glial fibers) antibodies. Consistent with our recent findings,Citation15 some N-cadherin-knockdown cells had a short and irregular leading process even after the acquirement of neuronal polarity (), whereas control scrambled RNAi (sh-scr4)-electroporated cells had a normal thick leading process (). In addition, the N-cadherinknockdown cells did not tightly attach to the radial glial fibers. Measurement of the length between the center of the soma of N-cadherin-knockdown bipolar cells with a leading process in the intermediate zone and the radial glial fibers they attached to revealed that compared with control cells [average distance was 2.03 ± 0.07 µm (n = 76 cells)], the average distance between N-cadherin-knockdown cells and the radial glial fibers was significantly increased [2.92 ± 0.19 µm (n = 63 cells), p < 0.0001, t test] (). These data suggest that N-cadherin is involved in the tight interaction between the locomoting neurons and radial glial fibers.
To carry out cell migration, adhesion complexes may be continuously and dynamically reconstituted. Previous electron microscopic analyses using cultured migrating neurons indicate that many coated vesicles are observed in the proximal regions of the leading processes (dilation regions)Citation20 and near the junctions between migrating neurons and glial fibers (interstitial densities)Citation21 raising the possibility that cell adhesion molecules may be internalized into coated vesicles and reconstituted. Consistent with this idea, we have showed that N-cadherin is internalized and recycled to the plasma membrane through Rab5- and Rab11-dependent endocytic pathways and that knockdown or dominant negative suppression of Rab5 or Rab11 perturbed neuronal migration.Citation15 Furthermore, it has been reported that dominant negative-Exo70, a component of the exocyst complex, also disturbs neuronal migration,Citation22 suggesting that both endocytosis and exocytosis are essential for proper neuronal migration.
Since knockdown of Rab5 increases the cell surface levels of N-cadherin, we examined whether the increased N-cadherin levels affect neuronal migration. A strong CAG promoter-driven N-cadherin-expressing vectorCitation23 was electroporated into E14 cerebral cortices, followed by fixation at postnatal day 0 (P0). While most of the control vector-electroporated cells had reached the superficial layer of the cortical plate at P0 (–F), some of the N-cadherin-overexpressing cells were still located in the lower part of the cortical plate (–I). To deduce the transfection efficiency in each migrating neuron, we visualized the EGFP fluorescence intensities with different colors ( and H). As indicated by the color bar in , blue, green and red colors represent strong, moderate and weak fluorescence intensities, respectively. Most of delayed neurons in the lower cortical plate displayed a strong blue intensity whereas cells in the superficial layer of the cortical plate were green or red in the N-cadherin-overexpressed cortices (). These data suggest that excess N-cadherin expression also disturbed neuronal migration. It is consistent with previous in vitro studies that excessive integrin-mediated adhesion inhibits non-neuronal cell migrationCitation24 and that stationary neurons form strong adhesions to radial glial fibers, such as desmosomes, rather than interstitial junctions.Citation21
Although both knockdown and overexpression of N-cadherin suppressed neuronal migration, their phenotypes were somewhat different. A portion of the N-cadherin-overexpressing cells exhibited abnormally branched leading processes (arrows in ), similar to Rab5-knockdown cells.Citation15 The ratio of cells with a branched leading process in the CP was significantly increased (control: 3.31 ± 2.49%, N-cadherin overexpression: 15.01 ± 2.83%, p < 0.02, t-test). In many cases, the branched processes of N-cadherin-overexpressing or Rab5-suppressing cells were elongated along radial glial fibers (data not shown). In addition, unlike N-cadherin-knockdown cells, N-cadherin-overexpressing cells seemed to closely attach to the radial glial fibers (–L). The average distance between N-cadherin-overexpressing cells and the radial glial fibers was slightly but significantly decreased, compared with control (). Although the percentage of cells exhibiting more than 2 µm of the distances was higher in control (control: 36.8%, N-cadherin overexpression: 19.4%), the minimum and median, but not maximum, values were similar in both conditions (Control maximum, median and minimum values: 3.60 µm, 1.90 µm and 1.16 µm, N-cadherin overexpression: 2.58 µm, 1.73 µm and 1.00 µm), suggesting that the breadth of the distribution of the measured distances was decreased in N-cadherin overexpression. These data suggest that N-cadherin-overexpressing cells attach to the radial glial fibers normally or slightly stronger.
Taken together with our recent report,Citation15 these findings suggest that N-cadherin is required for the proper attachment of the locomoting neurons to the radial glial fibers. Knockdown or overexpression of N-cadherin disturbed the neuronal attachment to or migration along the radial glial fibers, respectively (), although the neuronal positioning was eventually disrupted in both cases. Interestingly, overexpression of N-cadherin resulted in phenotypes resembling Rab5 knockdown. Our previous findings suggest that N-cadherin is endocytosed and recycled in the locomoting neurons in a Rab5-and Rab11-dependent manner, which are also essential for neuronal migration ().Citation15,Citation26,Citation27 Together with the fact that knockdown of Rab5 increases cell surface N-cadherin levels, our N-cadherin-overexpression experiments suggest that the major cause of the migration defects in Rab5-knockdown neurons is too much N-cadherin on the cell surface. Consistent with this, the migration defects in Rab5-knockdown neurons can be rescued by co-expression of low concentrations of the N-cadherin-knockdown vector.Citation15 These data suggest that N-cadherin is required for the radial glial fiber-dependent neuronal migration, but that the N-cadherin-mediated adhesion complexes should be actively reconstituted through Rab GTPases-dependent endocytic pathways to maintain proper cell surface levels of N-cadherin and to promote the piadirected migration of the locomoting neurons ().
Figures and Tables
Figure 1 Effects on N-cadherin knockdown or overexpression on the locomotion mode of neuronal migration. (A, B, J and K) The morphologies of N-cadherin knockdown or overexpressing locomoting neurons in the intermediate zone of E17 cerebral cortices. The neurons were electroporated with the following plasmids plus pCAG-EGFPCitation19 at E14; Neurons in (A, B, J and K) were electroporated with control scrambled short hairpin RNA (sh-scr4: the negative control vector of pSilencer3.1-H1-neo, Ambion), short hairpin RNA for N-cadherin (NC-sh1023),Citation15 control vector (CAG-MCS2, 10 µg/µl),Citation25 or N-cadherin-expressing vector (CAG-human N-cadherin, 10 µg/µl),Citation23 respectively. Green and red represent immunostaining signals for EGFP or Nestin, a marker for radial glial fibers, respectively. Arrows in (K) indicate branched leading processes. In utero electroporation and immunohistochemistry were performed as described previously in references Citation15 and Citation19. (C and L) The distance between the center of the cell soma of the cells with one thick process in the intermediate zone and the nestin-positive radial glial fiber to which they attach were deduced by Leica SP5 confocal microscopy. Each bar represents the average distance ± SEM. The average distance was significantly increased in N-cadherin-knockdown conditions (sh-scr4: 2.03 ± 0.07 µm, NC-sh1023: 2.92 ± 0.19 µm, p < 0.0001, t-test), whereas it was slightly but significantly decreased in N-cadherin-overexpression (control vector: 1.95 ± 0.04 µm, CAG-N-cadherin: 1.75 ± 0.05 µm, p < 0.01, t-test). (D–I) Cerebral cortices at P0, electroporated with control vector (CAG-MCS2, 10 µg/µl)Citation25 or N-cadherin-expressing vector (CAG-human N-cadherin, 10 µg/µl),Citation23 plus pCAG-EGFP at E14. EGFP fluorescent signals were shown as green in (D and G) or different colors as indicated by the color bar in (E and H). The graphs in (F and I) show the estimation of cell migration, which was carried out by recording fluorescence intensities of EGFP in distinct regions of the cerebral cortices using Leica SP5 software. Each bar represents the mean percentage of relative intensity ± SEM. n = 4 brains. The quantification method was described previously in references Citation14 and Citation19. II–IV, layers II–IV of the cortical plate; V–VI, layers V–VI of the cortical plate; IZ, intermediate zone; WM, white matter; SVZ/VZ, subventricular zone/ventricular zone. Scale bars: (in A) A, B, 10 µm; (in D) D, E, G and H, 200 µm; (in J) J and K, 10 µm.
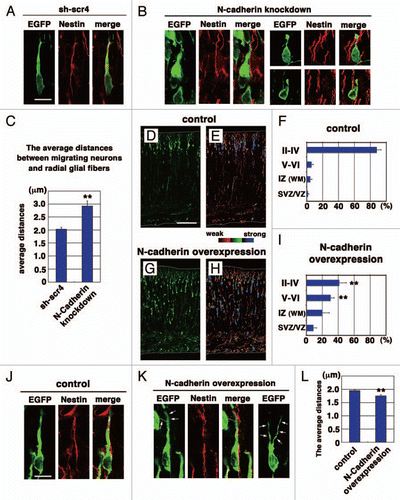
Figure 2 N-cadherin mediates the interaction between migrating neurons and radial glial fibers and radial glial-guided locomotion mode of neuronal migration. (A) Multipolar cells form a thick leading process, retract the other processes and attach to the radial glial fibers in order to change into the locomoting neurons. Knockdown of N-cadherin weakens the interaction between the polarized locomoting neurons and radial glial fibers. Subsequently, the locomoting neurons migrate toward the pial surface along the radial glial fibers. Similar to Rab5 suppression, increased levels of N-cadherin disturb the migration. (B) In the locomoting neurons, a portion of the cell surface N-cadherin is internalized into early endosomes (EE) and recycled back to the plasma membrane via recycling endosomes (RE). The intracellular trafficking of N-cadherin is essential for the piadirected migration of locomoting neurons. N-cadherin endocytosis and recycling are regulated by Rab family small GTPases, Rab5 and Rab11, respectively.
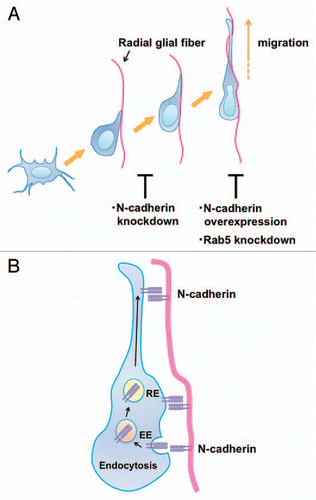
Acknowledgements
We thank Dr. Ozawa for providing us with N-cadherin-expressing vector, and Dr. Ruth T. Yu for helpful comments. We also thank the Core Instrumentation Facility, Keio University School of Medicine for help with Leica SP5 confocal microscopy. This work was supported by Grants-in-Aid from the Ministry of Education, Culture, Sports and Science and Technology, Japan and by grants from the JST PRESTO program and GCOE.
Addendum to:
References
- Marin O, Valiente M, Ge X, Tsai LH. Guiding neuronal cell migrations. Cold Spring Harb Perspect Biol 2010; 2:1834
- Kawauchi T, Hoshino M. Molecular pathways regulating cytoskeletal organization and morphological changes in migrating neurons. Dev Neurosci 2008; 30:36 - 46
- Rakic P. A century of progress in corticoneurogenesis: from silver impregnation to genetic engineering. Cereb Cortex 2006; 16:3 - 17
- Gleeson JG, Walsh CA. Neuronal migration disorders: from genetic diseases to developmental mechanisms. Trends Neurosci 2000; 23:352 - 359
- Nadarajah B, Parnavelas JG. Modes of neuronal migration in the developing cerebral cortex. Nat Rev Neurosci 2002; 3:423 - 432
- Barnes AP, Polleux F. Establishment of axon-dendrite polarity in developing neurons. Annu Rev Neurosci 2009; 32:347 - 381
- Nishimura YV, Sekine K, Chihama K, Nakajima K, Hoshino M, Nabeshima Y, et al. Dissecting the factors involved in the locomotion mode of neuronal migration in the developing cerebral cortex. J Biol Chem 2010; 285:5878 - 5887
- Rakic P. Mode of cell migration to the superficial layers of fetal monkey neocortex. J Comp Neurol 1972; 145:61 - 83
- Edmondson JC, Liem RK, Kuster JE, Hatten ME. Astrotactin: a novel neuronal cell surface antigen that mediates neuron-astroglial interactions in cerebellar microcultures. J Cell Biol 1988; 106:505 - 517
- Fishell G, Hatten ME. Astrotactin provides a receptor system for CNS neuronal migration. Development 1991; 113:755 - 765
- Zheng C, Heintz N, Hatten ME. CNS gene encoding astrotactin, which supports neuronal migration along glial fibers. Science 1996; 272:417 - 419
- Adams NC, Tomoda T, Cooper M, Dietz G, Hatten ME. Mice that lack astrotactin have slowed neuronal migration. Development 2002; 129:965 - 972
- Wilson PM, Fryer RH, Fang Y, Hatten ME. Astn2, a novel member of the astrotactin gene family, regulates the trafficking of ASTN1 during glial-guided neuronal migration. J Neurosci 2010; 30:8529 - 8540
- Kawauchi T, Chihama K, Nabeshima Y, Hoshino M. Cdk5 phosphorylates and stabilizes p27kip1 contributing to actin organization and cortical neuronal migration. Nat Cell Biol 2006; 8:17 - 26
- Kawauchi T, Sekine K, Shikanai M, Chihama K, Tomita K, Kubo K, et al. Rab GTPases-dependent endocytic pathways regulate neuronal migration and maturation through N-cadherin trafficking. Neuron 2010; 67:588 - 602
- Zhang J, Woodhead GJ, Swaminathan SK, Noles SR, McQuinn ER, Pisarek AJ, et al. Cortical neural precursors inhibit their own differentiation via N-cadherin maintenance of beta-catenin signaling. Dev Cell 2010; 18:472 - 479
- Kadowaki M, Nakamura S, Machon O, Krauss S, Radice GL, Takeichi M. N-cadherin mediates cortical organization in the mouse brain. Dev Biol 2007; 304:22 - 33
- Chenn A, Zhang YA, Chang BT, McConnell SK. Intrinsic polarity of mammalian neuroepithelial cells. Mol Cell Neurosci 1998; 11:183 - 193
- Kawauchi T, Chihama K, Nabeshima Y, Hoshino M. The in vivo roles of STEF/Tiam1, Rac1 and JNK in cortical neuronal migration. EMBO J 2003; 22:4190 - 4201
- Schaar BT, McConnell SK. Cytoskeletal coordination during neuronal migration. Proc Natl Acad Sci USA 2005; 102:13652 - 13657
- Gregory WA, Edmondson JC, Hatten ME, Mason CA. Cytology and neuron-glial apposition of migrating cerebellar granule cells in vitro. J Neurosci 1988; 8:1728 - 1738
- Letinic K, Sebastian R, Toomre D, Rakic P. Exocyst is involved in polarized cell migration and cerebral cortical development. Proc Natl Acad Sci USA 2009; 106:11342 - 11347
- Tsutsui J, Moriyama M, Arima N, Ohtsubo H, Tanaka H, Ozawa M. Expression of cadherin-catenin complexes in human leukemia cell lines. J Biochem 1996; 120:1034 - 1039
- Cox EA, Huttenlocher A. Regulation of integrinmediated adhesion during cell migration. Microsc Res Tech 1998; 43:412 - 419
- Kawauchi T, Chihama K, Nishimura YV, Nabeshima Y, Hoshino M. MAP1B phosphorylation is differentially regulated by Cdk5/p35, Cdk5/p25 and JNK. Biochem Biophys Res Commun 2005; 331:50 - 55
- Kawauchi T. Regulation of cell adhesion and migration in cortical neurons: Not only Rho but also Rab family small GTPases. Small GTPases 2011; 2:36 - 40
- Shieh JC, Schaar BT, Srinivasan K, Brodsky FM, McConnell SK. Endocytosis regulates cell soma translocation and the distribution of adhesion proteins in migrating neurons. PLoS One 2011; 6:e17082