Abstract
Cells respond to changes in osmolality with compensatory adaptations that re-establish ion homeostasis and repair disturbed aspects of cell structure and function. These physiologically complex processes can be separated into two functionally distinct cellular phases. The first phase operates to temporarily minimize cellular damage and stabilize critical cell functions necessary for survival. This phase is contingent upon the ability to generate a rapid adaptive response. For this reason, it occurs largely in the absence of de novo protein synthesis and instead relies upon modifying the activity of existing cellular proteins through protein-protein interactions and post-translational modifications. The second phase of the osmotic stress response is centered upon adjusting the expression of specific effector proteins required to re-establish cellular homeostasis. This phase is dependent on the completion of signal transduction events, as well the transcription and translation of target genes, and is therefore characterized by a significant temporal delay and not detected until several hours post exposure. Osmotic effector proteins central to the second phase, such as ion transporting proteins and organic osmolyte generating enzymes, have been studied in considerable detail. However, knowledge surrounding the first phase of the osmotic stress response is limited. This article focuses on recent insights into the players and interactions governing the first phase of the osmotic stress response with specific emphasis on protein-protein interactions.
Introduction
Osmolality is a pervasive abiotic factor that has a strong influence on cellular and organismal function. The effects of osmotic stress can be severe, damaging cellular macromolecules, disturbing cell volume, altering intracellular macromolecular density and modifying stringent intracellular inorganic ion concentrations.Citation1 Consequently, cells have evolved mechanisms to retain critical cell functions and re-establish cellular homeostasis in response to changes in osmolality. These physiological processes are highly complex and extend across all levels of biological organization from molecules to behavior.Citation2 At the molecular level, the osmotic stress response appears to occur in two distinct phases.Citation1,Citation3,Citation4 Because adaptive processes like cell volume regulation and ion transport are not instantaneous, the first phase is aimed at temporarily increasing tolerance limits towards osmotic stress. This phase is predicated by speed, and as a result is characterized by changes in the activity of existing cellular proteins via protein-protein interactions and post-translational modifications.Citation5 This first phase affords time for a subsequent response that involves the de novo synthesis of a separate set of osmotic stress specific effector proteins that work synergistically to re-establish cellular homeostasis.Citation1,Citation3,Citation4 The completion of this second phase is dependent upon osmosensing and signal transduction events, as well as protein synthesis. Therefore, this phase involves a considerable time requirement, and changes in the abundance of effector proteins are not generally detected until 12–18 hours of osmotic stress exposure ().Citation5,Citation6 The function of effector proteins during osmotic stress, such as ion transporters and organic osmolyte generating enzymes, has been studied in great detail.Citation7 However, our knowledge of the molecular mechanisms governing the first phase of the osmotic stress response is much more uncertain. In the proceeding sections we discuss recent developments surrounding the identity and expression of proteins involved in the first phase of the osmotic stress response in fish, with emphasis placed on protein-protein interactions that may drive early adaptive responses prior to changes in gene expression.
FK506-Binding Protein 51
FK506-binding protein 51 (FKBP-51) is a molecular chaperone comprising one component of a heterocomplex of proteins maintaining the glucocorticoid receptor (GR) in a constitutively inactive state.Citation8,Citation9 Recent data suggest that FKBP-51 may be an important component of early adaptive responses to osmotic stress.Citation5 During osmotic stress, unfolded proteins may recruit the chaperone activity of FKBP-51 away from the GR, relieving its inhibitory functions and promoting GR ligand binding and subsequent signaling events. Such a scenario is especially appealing given the prominent role for GR-mediated processes during osmoregulation. Cortisol, the major corticosteroid in fish, is considered a master regulator of gill chloride cell differentiation and function during osmoregulation.Citation10 The physiological actions of cortisol are exerted through the glucocorticoid receptor, which acts as a ligand-dependent transcription factor to control the expression of specific genes.Citation11 These actions are relatively rapid and take approximately 30 to 60 minutes. FKBP-51 mRNA is rapidly upregulated beginning at 2 hours post exposure during hyper-osmotic stress in fish gills.Citation5 Given the rapid actions of the activated GR, it is plausible that FKBP-51 was recruited and the GR activated by cortisol during the first 60 minutes of hyper-osmotic stress. GR activity may become subsequently repressed as FKBP-51 mRNA levels increase through hour 12 (). This mechanism of GR regulation is consistent with current knowledge regarding the regulation of the GR by chaperones,Citation12 and reminiscent of heat shock factor regulation, an environmental stress-induced transcription factor responsible for the rapid synthesis of heat shock proteins.Citation13
Translationally Controlled Tumor Protein
Translationally controlled tumor protein (TCTP) is a highly conserved, transcriptionally-regulated protein widely expressed in eukaryotic cells, and implicated in a variety of cellular processes.Citation14 Interestingly, the protein structure of TCTP in yeast reveals homology with a family of small chaperone proteins.Citation15 Recent data have shown that TCTP interacts with the cytoplasmic domain of the catalytic α-subunit of Na+, K+-ATPase, acting as a repressor of activity.Citation16 In a mechanism similar to that described for FKBP-51, protein damage incurred by osmotic stress may recruit TCTP away from the Na+, K+-ATPase, relieving inhibition and promoting Na+, K+-ATPase dependent osmoregulatory processes ().Citation5
Insulin like Growth Factor Binding Proteins
Exogenous application of IGF-1 increases salinity tolerance in rainbow trout, Atlantic salmon, killifish and brown trout.Citation17,Citation18 The majority of IGFs in extracellular fluids are found complexed with specific high affinity binding proteins (IGFBPs). IGFBPs have been suggested to mediate the efflux of IGFs from the vascular space to the cell surface, thereby modulating interactions between ligands and receptors, and to prevent proteolytic degradation of IGFs, prolonging their half-lives.Citation19 The rapid induction of IGFBP-1 mRNA in hyper-osmotically stressed gill tissue is consistent with a role in early osmoregulatory events ().Citation5 Given the prominent role for IGFs in osmoregulatory processes, it is likely that IGFBPs serve to efficiently transduce IGF driven signaling events.
Concluding Remarks
At the molecular level, achieving ion homeostasis during osmotic stress is contingent upon the cell's ability to recognize and quantify environmental osmolality and arrange an appropriate response. Integral are the coordinated activities of osmosensors, which activate appropriate signal transduction pathways; signal transducers, which relay molecular messages to specific target molecules; and effectors, which work in concert to actively restore homeostasis.Citation2 Effector mechanisms involved in osmotic acclimation of a great many fish species have been identified and characterized in detail. In contrast, only modest attention has been directed towards identifying the molecular osmosensing and signal transduction events leading up to the activation of these effector proteins. However, recent efforts have begun to shed light on the identity and expression of molecules that function during the earliest osmoregulatory events. These data have shown that multiple major signaling pathways work in concert to modify diverse effectors, and that these pathways operate within a framework of regulatory proteins such as FKBP-51, TCTP and IGFBPs.Citation5 These regulatory proteins likely operate independent of de novo protein synthesis and may enable the earliest adaptive responses to osmotic stress.
Abbreviations
FKBP-51 | = | FK506 binding protein 51 |
GR | = | glucocorticoid receptor |
TCTP | = | translationally controlled tumor protein |
IGF | = | insulin like growth factor |
IGFBP | = | insulin like growth factor binding protein |
Figures and Tables
Figure 1 Expression profiles of osmotically-regulated genes illustrating the two-phase osmotic stress response (shaded areas). Genes mediating protein-protein interactions (open bars; FKBP-51, TCTP, IGFBP from left to right) and putatively involved in the first phase of the osmotic stress response (darkly shaded area) are significantly differentially expressed earlier than effector proteins (filled bars; Na+, K+ ATPase alpha subunit, Na+ channel IX alpha subunit, organic osmolyte catalyzing enzymes: cysteine sulfinic acid decarboxylase (taurine), glutamate decarboxylase (GABA) and inositol monophosphatase (inositol) (from left to right)) involved in the second phase (lightly shaded area). Because the completion of the second phase is dependent upon osmosensing and signal transduction events, as well as de novo protein synthesis, changes in the abundance of effector proteins are not detected until 12 hours of osmotic stress exposure. Protein-protein interactions, which enable rapid adaptive responses in the absence of changes in gene expression, may therefore underlie the first phase of the osmotic stress response. Expression and significance values were extracted from Evans and Somero,Citation5 and are illustrated as the absolute value of the log fold change. Open asterisks denote earliest time point of significant expression for phase 1 genes. Closed asterisks denote earliest time point of significant expression during of phase 2 effector proteins.
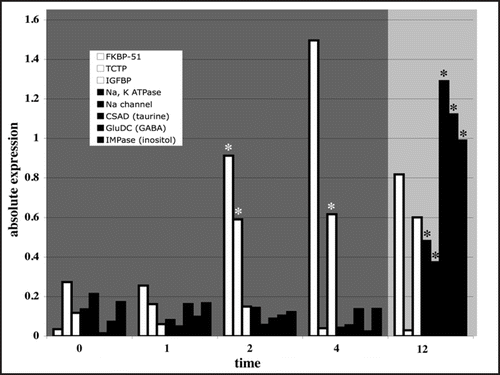
Addendum to:
References
- Kültz D, Burg M. Evolution of osmotic stress signaling via MAP kinase cascades. J Exp Biol 1998; 201:3015 - 3021
- Fiol DF, Kültz D. Osmotic stress sensing and signaling in fishes. FEBS J 2007; 274:5790 - 5798
- Kültz D. Molecular and evolutionary basis of the cellular stress response. Annu Rev Physiol 2005; 67:225 - 257
- Kültz D. Evolution of the cellular stress proteome: from monophyletic origin to ubiquitous function. J Exp Biol 2003; 206:3119 - 3124
- Evans TG, Somero GN. A microarray-based transcriptomic time-course of hyper- and hypo-osmotic stress signaling events in the euryhaline fish Gillichthys mirabilis: osmosensors to effectors. J Exp Biol 2008; 211:3636 - 3649
- Fiol DF, Kültz D. Rapid hyperosmotic coinduciton of two tilapia (Oreochromis mossambicus) transcription factors in gill cells. Proc Natl Acad Sci USA 2005; 102:927 - 932
- Evans DH. Cell signaling and ion transport across the fish gill epithelium. J Exp Zool 2002; 293:336 - 347
- Westberry JM, Sadosky PW, Hubler TR, Gross KL, Scammell JG. Glucocorticoid resistance in squirrel monkeys results from a combination of a transcriptionally incompetent glucocorticoid receptor and overexpression of the glucocortcoid receptor co-chaperone FKBP51. J Steroid Biochem Mol Biol 2006; 100:34 - 41
- Zhang X, Clark AF, Yorio T. FK506-binding protein 51 regulates nuclear transport of the glucocorticoid receptor beta and glucocorticoid responsiveness. Invest Opthalmol Vis Sci 2008; 49:1037 - 1047
- McCormick SD. Endocrine control of osmoregulation in teleost fish. Am Zool 2001; 41:781 - 794
- Cato ACB, Nestl A, Mink S. Rapid actions of steroid receptors in cellular signaling pathways. Science's STKE 2002; 138:9
- Grad I, Picard D. The glucocorticoid responses are shaped by molecular chaperones. Mol Cell Endocrinol 2007; 275:2 - 12
- Voellmy R. On the mechanisms that control heat shock transcription factor activity in metazoan cells. Cell Stress Chaper 2004; 9:122 - 133
- Bommer UA, Thiele BJ. The translationally controlled tumor protein (TCTP). Int J Biochem Cell Biol 2004; 36:379 - 385
- Thaw P, Baxter NJ, Hounslow AM, Price C, Waltho JP, Craven CJ. Structure of TCTP reveals an unexpected relationship with guanine nucleotide-free chaperones. Nat Struct Biol 2001; 8:701 - 704
- Kim MJ, Kwon JS, Suh SH, Suh JK, Jung J, Lee SN, et al. Transgenic overexpression of translationally controlled tumor protein induces systemic hypertension via repression of Na+/K+-ATPase. J Mol Cell Cardiol 2008; 44:151 - 159
- Mancera JM, McCormick SD. Osmoregulatory actions of the GH/IGF axis in nonsalmonid teleosts. Comp Biochem Physiol B 1998; 121:43 - 48
- Seidelin M, Madsen SS, Byrialsen A, Kristiansen K. Effects of insulin like growth factor-1 and cortisol on Na+/K+ ATPase expression in osmoregulatory tissues of brown trout (Salmo trutta). Gen Comp Endocrinol 1999; 113:331 - 342
- Wood AW, Duan C, Bern HA. Insulin-like growth factor signaling in fish. Int Rev Cytol 2005; 243:215 - 285