Abstract
In a recent paper, we described the orientation behavior of two passserine migrants under dim red light: the birds headed westward in spring as well as in autumn, displaying a 'fixed direction'-response. 'Fixed direction'-responses in other directions were observed under abnormal light regimes. Here, we point out the characteristic features of the 'fixed direction'-responses, in particular their differences to normal compass orientation, and discuss their implications. The conditions under which they are observed suggest complex interactions between magnetoreception and the visual system.
Compass Orientation and ‘Fixed Direction’-Responses
Magnetoreception in birds has been analyzed by using migratory orientation—the spontaneous tendency of migratory birds to head southward in autumn and northward in spring produces reliable directional tendencies that provide a stable baseline for orientation tests. As long as the birds are able to derive meaningful directional information from the magnetic field, they head in their seasonally appropriate migratory direction. The directional tendencies recorded in suitable orientation cages agree well with the direction of natural migration as indicated by ringing recoveries. Using the orientation of European Robin, Erithacus rubecula (Turdidae) in migratory direction as a criterion, the characteristics of the avian magnetic compass were analyzed already in the 1970s. They were found to differ markedly from those e.g., of the technical compass, with the most important difference beings that the avian compass works as an ‘inclination compass’—it is based on the axial course of the field lines and their inclination rather than on the polarity of the magnetic field.Citation1
Recently, however, another type of directional response has been described in two passerine migrants, the European Robin and the Australian Silvereyes, Zosterops l. lateralis (Zosteropidae). This response, observed under dim 645 nm red light of only 1 mW/m2, involves a westerly tendency that differs significantly from the migratory direction and, most surprisingly, it was the same in spring and in autumn, not showing the normal seasonal shift. This characterizes it as a so-called ‘fixed direction’-response. A detailed analysis revealed that it depends on the ambient magnetic field, but does not involve the inclination compass.Citation2 It thus represents a polar response—a property that contrasts sharply with the normal compass that controls migratory orientation.
The westerly tendency under dim red light, already described by Muheim et al. but not recognized as ‘fixed direction’-response,Citation3 is not the only one observed so far (). The first was found in silvereyes under bright monochromatic 565 nm green light (15 mW/m2),Citation4 where it was also identified as a polar response to the magnetic field, not involving the inclinations compass.Citation5 In robins, more ‘fixed direction’-responses have been described under various light regimes, one under bright monochromatic 502 nm turquoise light (17 mW/m2),Citation6 others under a combination of blue, turquoise and green light with 590 nm yellow light,Citation7–Citation9 and yet another recently in total darkness,Citation10 the latter possibly identical with that under dim red light.Citation2 All of them turned out to be polar responses.Citation6,Citation8,Citation9 These findings indicate a fundamental difference between the magnetic compass used for locating the migratory direction and the mechanisms leading to ‘fixed direction’-responses.
The Underlying Physical Mechanisms
The physical principles underlying the avian magnetic compass have been identified as radical pair processes by the disorienting effect of oscillating magnetic fields in the MHz range.Citation11,Citation12 This is consistent with the model forwarded by Ritz and colleagues:Citation13 Photon absorption leads to the formation of radical pairs, with the singlet—triplet ratio depending on the alignment of the receptor molecule in the ambient magnetic field. Since this effect is not sensitive to the polarity of the field, the model provides an explanation for the functional mode of the inclination compass. The first step—photon absorption—makes magnetoreception light dependent. This is in agreement with the observation that magnetic compass orientation in birds requires light from the short wavelength end of the spectrum up to 565 nm green; beyond that, birds were found to be disoriented.Citation14,Citation15 The right eye is indicated as site of the magnetoreceptors mediating compass information.Citation16
The mechanisms underlying the ‘fixed direction’-responses differ from those of the compass in several aspects, summarized in . ‘Fixed direction’-responses are also observed in total darkness.Citation10 The same oscillating fields that disrupt compass orientationCitation11,Citation12 have no effect.Citation6,Citation9,Citation10 Instead, ‘fixed direction’-responses are disrupted by local anesthesia of the upper beak, a treatment that does not affect orientation in migratoryCitation2,Citation9 and other compass directions.Citation15 In the skin of the upper beak, iron rich-structures containing superparamagnetic magnetite have been identified and discussed as putative magnetoreceptors in pigeons and other birds;Citation17–Citation20 the findings mentioned above suggest these receptors as origin of the directional information underlying the ‘fixed direction’-responses.
Interactions with the Visual System
These findings raise a number of questions. If the directional information for the ‘fixed direction’-responses originates in the magnetite-based receptors in the beak, why does the manifestation of a specific ‘fixed direction’ depend on the light regime? One tends to assume that any information provided by the receptors in the beak would be independent from light. Yet very different ‘fixed directions’ are observed under light of different wavelengths and combinations of wavelengths (). These findings seem to suggest interactions between directing information from the upper beak and the visual system at higher levels where the respective information is combined and processed.
This leads to the question about the conditions under which ‘fixed direction’-responses are observed. Darkness and dim red light probably interfere with the normal magnetic compass because they do not allow the formation of the crucial radical pairs. The occurrence of ‘fixed direction’-responses under bright monochromatic light (quantal flux about 36 quanta/s m2) and under bichromatic light (both components with a quantal flux of 6 quanta/s m2) is less easy to explain. Normal compass orientation in migratory direction is observed under dim monochromatic light with a quantal flux up to about 8 quanta/s m2. When the light intensity was increased above a certain level, the birds were no longer oriented in their migratory direction, but showed ‘fixed direction’-responses or axial preferences.Citation7,Citation21 This seems to imply that these light conditions also interfere with the perception of magnetic directional information. It is hard to see how they could disrupt the radical pair mechanism, as the light levels are still rather low; they correspond to light conditions before sunrise or after sunset and are by far lower than the light on a bright sunny day, where the magnetic compass works without problems.Citation22 Hence saturation of the receptors can be excluded. The difference between the test situation in the laboratory and the natural situation outside is that the test lights had been monochromatic or bichromatic, i.e., they would activate one or two of the color cones, but not the others. This is a totally unnatural situation, as natural light is always ‘white’ in the sense that it is composed of a multitude of wavelengths—‘fixed direction’-responses are only observed under conditions that do not occur in nature.
The levels of the monochromatic light where the change in the type of response takes place appears to depend on the wavelengths: under UV, the change occurs at lower intensities than under e.g., green.Citation21 The sensitivity of the color cones decreases with increasing wavelengths; hence it seems possible that the change in behavior is associated with an activation of the cones above a certain level, leading to an imbalance between their output. This may happen already at the level of the ganglion cells in the retina or at higher levels where visual information is processed; details are yet unknown. The imbalance, in turn, seems to somehow disrupt the magnetoreception system providing compass information. The same might also apply to the bichromatic lights leading to ‘fixed direction’-responses. Together, the conditions under which ‘fixed direction’-responses are observed also imply a complex relationship between magnetoreception and color vision in birds.
‘Fixed Direction’-Responses vs. Disorientation?
A third question concerns the observation that under certain conditions, the birds are disoriented in the geomagnetic field where magnetic information should be available. This is e.g., the case when they are treated with oscillating fields in the MHz range, a treatment that disrupts radical pair processes,Citation11,Citation12,Citation15 but does not interfere with the magnetite-based receptors in the upper beak.Citation11 Why do these receptors not take over and make the birds head into a ‘fixed direction’? The same applies to the tests under long wavelength lightCitation15,Citation23,Citation24 and when the right eye is coveredCitation16—in both situations, the birds do not head into ‘fixed directions’, but are disoriented, although neither of the treatments affects the magnetite-based receptors in the beak.
In first case, the radical pair processes in the right eye are directly disrupted by the oscillating fields; in the latter two cases, they are probably suppressed because of lack of suitable light. In total darkness, however, birds show a westerly ‘fixed direction’-response.Citation10 This situation suppresses the radical pair processes, but, at the same time, it also deprives the birds of all visual input. The question why this should lead to a different response is still open. It implies, however, that an interference with the primary physical process at the periphery alone, as caused by oscillating fields or lack of suitable light, may be fundamentally different from interference at the level of transmitting or processing magnetic information where input from the visual system is involved.
Nature and Significance of ‘Fixed Direction’-Responses
Another question concerns the nature of the responses. The inclination compass based on radical pair processes is used as a true compass to locate compass courses. Migrating birds head into their migratory direction—south in autumn and north in spring; the inclination compass indicates where these directions lie. The magnetic compass of homing pigeons is used in a similar way to locate the home course determined by navigational processes,Citation22 wherever this course lies, with the specific course depending on the pigeons' present position with respect to their home loft. Domestic chickens also have a magnetic compass that shares all major properties with that of migratory birds.Citation15 It was demonstrated in conditioning experiments with a social reward, where the chicks were trained to head into directions selected by the experimenter.Citation25
The magnetic compass thus tell birds where the direction lies in which they want to head, and this can be any direction the birds (or the experimenter) chooses. ‘Fixed direction’-responses, in contrast, are ‘fixed’: under a specific light condition, only one heading seems possible. Here, the birds do not seem to have any choice; it looks as if the particular direction is forced upon them by the respective stimulus situation, possibly similar to alignments. Details on the emergence of ‘fixed direction’-responses, and why certain light regimes cause a specific ‘fixed direction’ are not yet known.
This leaves a final question, namely that about the significance of the ‘fixed direction’-responses. They originate in the magnetite-based receptors in the skin of the upper beak, a receptor system that normally seems to provide information on magnetic intensity as a component of the navigational ‘map’, as indicated by experimental evidence from behavioralCitation26–Citation28 as well as electrophysiological studies.Citation29 The finding that these receptors also provide the directional information underlying ‘fixed direction’-responses was rather surprising. The biological significance of this additional function is unclear, as the ‘fixed direction’-responses do not seem to be helpful to the birds in any way. They are also not harmful, however, as they do not occur under natural conditions—they are observed only in the laboratory under light conditions that seem to drive the normal compass mechanism beyond its limits.
Possibly, the directional input underlying the ‘fixed direction’-responses is some kind of phylogenetic relict. Magnetite is found in all vertebrate groups,Citation30 and e.g., in mammals, it is discussed as basis for a magnetic compass.Citation31 Hence the diretcional input could represent an ancient function of magnetite-based receptors that has lost its significance in today's birds. Here it has been superseded by the modern avian compass based on radical pair processes that birds nowadays use.
Figures and Tables
Figure 1 ‘Fixed direction’-responses observed in migratory birds. The arrows mark the mean vectors based on the individual mean headings of 10 or 12 birds; the symbols at the periphery of the circle mark the mean ‘fixed direction’ in Australian silvereyes (diamonds) and European robins (triangles). The wavelengths of the monochromatic or bichromatic lights are indicated; for the respective intensities, see text (reviewed in refs. Citation2, Citation4 and Citation6–Citation9).
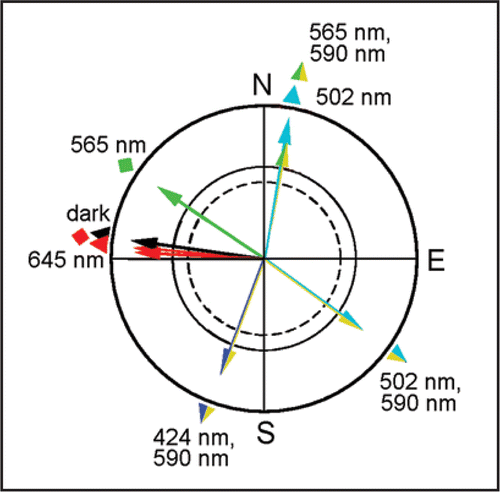
Table 1 Differences between compass orientation and fixed direction responses in birds
Addendum to:
References
- Wiltschko W, Wiltschko R. The magnetic compass of European robins. Science 1972; 176:62 - 64
- Wiltschko R, Munro U, Ford H, Stapput K, Wiltschko W. Light-dependent magnetoreception: orientation behaviour of migratory birds under dim red light. J Exp Biol 2008; 211:3344 - 3350
- Muheim R, Bäckman J, Akesson S. Magnetic compass orientation in European robins is dependent on both wavelength and intensity of light. J Exp Biol 2002; 205:3845 - 3856
- Wiltschko W, Wiltschko R, Munro U. Light-dependent magnetoreception in birds: the effect of intensity of 565-nm green light. Naturwissenschaften 2000; 87:366 - 369
- Wiltschko W, Munro U, Ford H, Wiltschko R. Magnetic orientation in birds: non-compass responses under monochromatic light of increased intensity. Proc R Soc Lond B 2003; 270:2133 - 2140
- Wiltschko R, Ritz T, Stapput K, Thalau P, Wiltschko W. Two different types of light-dependent responses to magnetic fields in birds. Curr Biol 2005; 15:1518 - 1523
- Wiltschko W, Gesson M, Stapput K, Wiltschko R. Light-dependent magnetoreception in birds; interaction of at least two different receptors. Naturwissenschaften 2004; 91:130 - 134
- Stapput K, Gesson M, Wiltschko R, Wiltschko W. Light-dependent magnetoreception: behavior of migratory birds under monochromatic and bichromatic lights. Orientation & Navigation—Birds, Humans and other Animals 2005; 16:Proc RIN conference Reading, England Royal Society of Navigation, Poster 1 - 7
- Wiltschko R, Stapput K, Ritz T, Thalau P, Wiltschko W. Magnetoreception in birds: different physical processes in different types of responses. HFSP J 2007; 1:41 - 48
- Stapput K, Thalau P, Wiltschko R, Wiltschko W. Orientation of birds in total darkness. Curr Biol 2008; 18:602 - 606
- Ritz T, Thalau P, Philllips JB, Wiltschko R, Wiltschko W. Resonance effects indicate a radical-pair mechanism for avian magnetic compass. Nature 2004; 429:177 - 180
- Thalau P, Ritz T, Stapput K, Wiltschko R, Wiltschko W. Magnetic compass orientation of migratory birds in the presence of a 1.315 MHz oscillating field. Naturwissenschaften 2005; 92:86 - 90
- Ritz T, Adem S, Schulten K. A Model for photoreceptor-based magnetoreception in birds. Biophys J 2000; 78:707 - 718
- Wiltschko W, Wiltschko R. Light-dependent magnetoreception in birds: the behavior of European robins, Erithacus rubecula, under monochromatic light of various wavelengths. J Exp Biol 2001; 204:3295 - 3302
- Wiltschko W, Freire R, Munro U, Ritz T, Rogers L, Thalau P, et al. The magnetic compass of domestic chickens, Gallus gallus. J Exp Bio 2007; 210:2300 - 2310
- Wiltschko W, Traudt J, Güntürkün O, Prior H, Wiltschko R. Lateralisation of magnetic compass orientation in a migratory birds. Nature 2002; 419:467 - 470
- Hanzlik M, Heunemann C, Holzkamp-Rötzler E, Winklhofer M, Petersen N, Fleissner G. Superparamagnetic magnetite in the upper beak tissue of homing pigeons. BioMetals 2000; 13:325 - 331
- Fleissner G, Holtkamp-Rötzler E, Hanzlik M, Winklhofer M, Fleissner G, Petersen N, et al. Ultrastructural analysis of a putative magnetoreceptor in the beak of homing pigeons. J Comp Neurol 2003; 458:350 - 360
- Fleissner G, Stahl B, Thalau P, Falkenberg G, Fleissner G. A novel concept of Fe-mineral-based magnetoreception: histological and physicochemical data from the upper beak of homing pigeons. Naturwissenschaften 2007; 94:631 - 642
- Tian L, Xiao B, Lin W, Zhang S, Zhu R, Pan Y. Testing for the presence of magnetite in the upper-beak skin of homing pigeons. BioMetals 2007; 20:197 - 203
- Wiltschko R, Stapput K, Bischof HJ, Wiltschko W. Light-dependent magnetoreception in birds: increasing intensity of monochromatic light changes the nature of the response. Front Zool 2007; 4:5
- Wiltschko R, Wiltschko W. Clock-shift experiments with homing pigeons: a compromise between solar and magnetic information?. Behav Ecol Sociobiol 2001; 49:393 - 400
- Wiltschko W, Munro U, Ford H, Wiltschko R. Red light disrupts magnetic orientation of migratory birds. Nature 1993; 364:525 - 527
- Wiltschko W, Wiltschko R. The effect of yellow and blue light on magnetic compass orientation in European Robins, Erithacus rubecula. J Comp Physiol A 1999; 184:295 - 299
- Freire R, Munro UH, Rogers LJ, Wiltschko R, Wiltschko W. Chickens orient using a magnetic compass. Curr Biol 2005; 15:620 - 621
- Munro U, Munro JA, Phillips JB, Wiltschko R, Wiltschko W. Evidence for a magnetite-based navigational ‘map’ in birds. Naturwissenschaften 1997; 84:26 - 28
- Beason RC, Semm P. Does the avian ophthalmic nerve carry magnetic navigational information?. J Exp Biol 1996; 198:141 - 146
- Wiltschko W, Munro U, Ford H, Wiltschko R. Bird navigation: what type of information does the magnetite-based receptor provide?. Proc R Soc B 2006; 273:2815 - 2850
- Semm P, Beason RC. Responses to small magnetic variations by the trigeminal system of the bobolink. Brain Res Bull 1990; 25:735 - 740
- Kirschvink JL, Jones DS, MacFadden BJ. Magnetite Biomineralization and Magnetoreception in Organisms 1985; New York Plenum Press
- Wegner RE, Begall S, Burda H. Magnetic compass in the cornea: local anaesthesia impairs orientation in a mammal. J Exp Biol 2006; 209:4747 - 4750