Abstract
The genetically tractable filamentous ascomycete fungus Aspergillus nidulans has been successfully exploited to gain major insight into the eukaryotic cell cycle. More recently, its amenability to in vivo multidimensional microscopy has fueled a potentially gilded second age of A. nidulans cell biology studies. This review specifically deals with studies on intracellular membrane traffic in A. nidulans. The cellular logistics are subordinated to the needs imposed by the polarized mode of growth of the multinucleated hyphal tip cells, whereas membrane traffic is adapted to the large intracellular distances. Recent work illustrates the usefulness of this fungus for morphological and biochemical studies on endosome and Golgi maturation, and on the role of microtubule-dependent motors in the long-distance movement of endosomes. The fungus is ideally suited for genetic studies on the secretory pathway, as mutations impairing secretion reduce apical extension rates, resulting in phenotypes detectable by visual inspection of colonies.
Keywords: :
Why A. nidulans Mitosis is Golden
The title of this review is in tribute to the monumental contributions that Ron Morris and his students and post-docs made to our understanding of mitosis. It derives from the title (“Mitotic gold in a mold”) of one of the reviews summarizing their work.Citation1 (A more recent review provides a comprehensive account of these findings.Citation2) The organism used in these groundbreaking studies was the haploid hyphal ascomycete Aspergillus nidulans, whose genetics had been developed by Guido Pontecorvo in the 1940s and 1950s.Citation3 “Ponte” exploited the “resolving power of genetic analysis” achievable with this fungus to conclude that genetic exchange (crossing over) can take place within a gene.Citation4 (Molecular techniques revealed that the resolving power of recombination can be narrowed down to as few as 12 bp.Citation5) The genetic tools that make A. nidulans a genetically tractable model organism have been recently reviewed.Citation6,Citation7
In his seminal screen for mitotic mutations,Citation8 Ron classified his conditional (ts) mutations in three different classes. Class I mutants, denoted nim (never in mitosis), arrested with both low spindle and low mitotic indices. Class II mutants (bim, blocked in mitosis) arrested with both high spindle and high mitotic indices. Class III mutants involved in nuclear distribution will be discussed below, as they are more directly related to this review. Characterization of bim and nim genes yielded golden data on mitosis. For example, they enabled the first report that a kinesin (BimC) is required for spindle formation.Citation9 BimE, a key component of what we know today as the anaphase-promoting complex/cyclosome (APC/C) was shown to be a master negative regulator of the mitosis-triggering maturation-promoting factor (MPF, today known as the Cdk1/cyclin B kinase).Citation10 NimA was shown to be another kinase required for mitosisCitation11 and the founding member of a family of protein kinases acting downstream of Cdk1.Citation12 As entry into mitosis involves protein phosphorylation, a popular supposition had it that mitotic exit might involve dephosphorylation. Characterization of BimG as a protein phosphatase provided the proof of principle that “dephosphorylation of a key protein(s) is required to complete mitosis.”Citation13
The Vegetative Phase, the Morphogenetic Switch
As in other fungi, the morphology of the cells is shaped by the cell wall. Thus, morphology is directly related to the direction and magnitude of secretion. During its vegetative phase, A. nidulans propagates by means of asexual spores (conidiospores) that form in specialized structures, the conidiophoresCitation14 ( and ). Wild-type A. nidulans conidiospores are green-colored and cover the surface of the colonies, giving them a characteristic appearance (). Conidiospores, which can be obtained in trillions from a single Petri dish, are nearly spherical. They are uninucleate and haploid, facilitating genetic analysis. Upon germination on an appropriate substrate, these spores initially grow isotropically (“swelling”) until they reach a certain diameter, after which a polarity axis is established (). At this point the whole biosynthetic machinery is focused toward the emerging germ-tube and from then onwards cell growth occurs exclusively by apical extension. Thus, unlike Saccharomyces cerevisiae, in which polarized growth is restricted to specific phases of the cell cycle,Citation15 A. nidulans, like many other filamentous fungi, represents an extreme example of persistently (and cell cycle independentCitation16) polarized growth.Citation17
Figure 1.Aspergillus nidulans. (A) Scanning micrograph of a conidiophore with nomenclature of the different specialized cells. (B) A hyphal tip cell. Nuclei were visualized by using a GFP-tagged version of the PacC zinc-finger transcription factor. (C) Progeny of a cross between a rabC+ strain carrying a yA2 “yellow spore” mutation and a rabCΔ strain with wild-type (green) conidiospores. Large colonies are rabC+ progeny whereas small colonies are rabCΔ progeny. As detailed in the text, RabCRab6 plays multiple roles in secretion and therefore its absence results in a conspicuous growth phenotype. The inset displays a sector of the Petri dish at double magnification.
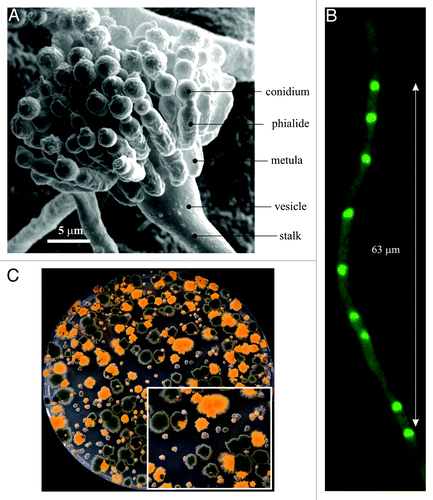
Figure 2. The vegetative growth cycle of A. nidulans. When an A. nidulans conidiospore germinates, it grows isotropically for a period of time, after which a polarity axis is selected (“polarity establishment”). This emerging polarity axis gives rise to a germ-tube, into which several nuclei migrate before the first septum is formed. This polarity axis can be maintained indefinitely, and subsequent septation events will take place as apical extension proceeds. Subapical cells may also establish new polarity axes (“branching”), which are also colonized by nuclei, giving rise to further hyphal tip cells. In the presence of appropriate stimuli, vegetative growth arrests and the developmental program starts with the emergence of a thick-walled “foot cell.” The tip of the foot cell undergoes a characteristic swelling, giving rise to a vesicle. Nuclei align in the periphery of the vesicle and migrate into cells that bud from the vesicle, denoted metulae. Metulae give rise to phialides (secondary sterigmata) and the latter produce conidiospores.Citation14,Citation97 Green arrows indicate the direction of polarized growth.
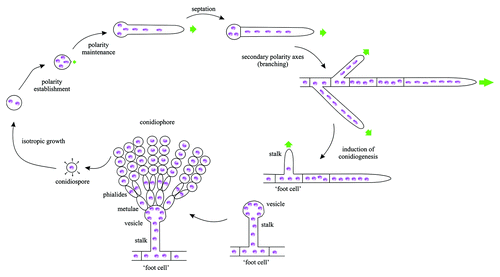
Size Matters
Rather than uninucleated, like the budding yeast cells, the vegetative tubular cells of A. nidulans are coenocytic (i.e., contain multiple nuclei per cell). Nuclei are exquisitely ordered, being evenly distributed across the whole hyphal length (), with an average distance between adjacent nuclei of 6.9 ± 0.7 μm.Citation18 The mean number of nuclei of the tip cells is 20 and the mean length of these cells is ~125 µm. Therefore, whereas the width of the hyphae (~2.5 µm) is below the length of the short axis of the S. cerevisiae cell (~3.8 µm), the distance between adjacent A. nidulans nuclei is larger, and the total cell length markedly larger than the longest axis of the yeast cell ellipsoid (ca. 4.5 µm). The larger size of the hyphal tip cell and the fact that the secretion machinery is at all times oriented toward the apex facilitate microscopy studies.
A Moving Story
The remarkably large intracellular distances existing within the hyphal tip cell calls for efficient organization of subcellular logistics. Long-distance delivery of cargoes necessitates tracks and efficient motors. Among these cargoes are the nuclei. Shortly after polarity is established, nuclei migrate into the nascent germ-tube and, after subsequent tip growth by apical extension, move forward with the growing tip, yet maintaining their ordered and even distribution (). Nuclear migration and positioning in A. nidulans is, as in S. cerevisiae, mediated by MTs and associated motors.Citation19 However, the conspicuous phenotypes displayed by A. nidulans mutants deficient in this process facilitate detection of mutations impairing these processes.
While the only non-mitotic role of MTs in S. cerevisiae is mediating nuclear migration, in A. nidulans MTs additionally play roles in intracellular traffic, resembling the roles of MTs in mammalian cells (see below). This feature has been exploited to study changes in organelle identity that are coupled to MT-dependent movement, such as endosomal maturation, enabling the use in studies on intracellular traffic of the arsenal of mutations derived from studies of nuclear migration that were instrumental for our current understanding of MT-dependent processes. A. nidulans was the first organism in which genetic analysis was applied to MTs, which led to the characterization of genes for α-Citation20 and β-tubulinCitation21 (the latter was another landmark achievement, representing the first ever characterization of a tubulin gene). Ten years later, Berl and Liz Oakley used suppressor analysis of tubulin mutations to identify, also for the first time, γ-tubulin.Citation22 Nuclear movement was shown to be β-tubulin-dependent.Citation23 The third category of mutants emerging from Morris' screens were the nud (nuclear distribution) mutants, which are able to establish polarity but are unable to move nuclei into nascent germ-tubes.Citation8 Characterization of nud genes revealed that they encode components of the dynein pathway. For example, nudA was shown to encode the heavy chain of cytoplasmic dyneinCitation24 whereas NudF,Citation25 the homolog of human LIS1 involved in lissencephaly, is a dynein regulatory factor (see below).
Secretion Predominates in the Tip
The apical extension rate of A. nidulans tip cells is remarkably high (of the order of 0.5–1 µm/min for hyphae cultured in microscopy chambers). This rate can only be maintained if the growing tip is provided, by way of the secretory pathway, with an appropriate supply of membrane, membrane fusion machinery proteins and enzymes/precursors involved in cell wall remodeling. One filamentous fungal-specific structure, denoted the Spitzenkörper (SPK), appears to contribute crucially to the directionality and efficiency of apical extension. The SPK is an actin-rich accumulation of vesicles of secretory nature adjacent to the apical plasma membrane.Citation26,Citation27 SepA, the A. nidulans formin, is a component of the SPK,Citation28 which reinforces the idea that this structure represents a microfilament organizing center that directs secretion to the growing apex in an F-actin-dependent manner. The fact that vesicles accumulate in the SPK implies that one or more limiting factors regulate fusogenicity with the plasma membrane. It is generally assumed that the clustering of vesicles at the SPK indicates that exocytosis is exclusively targeted to the apical dome. Indeed the A. nidulans exocyst component SecC (Sec3p in S. cerevisiae) localizes exclusively to the apex,Citation29 the synaptobrevin v-SNARE SynA distinctly labels the SPKCitation29,Citation30 and there is a conspicuous spot of the plasma membrane syntaxin SsoA at the apex.Citation29 However, besides this spot, SsoA is evenly distributed across the cell periphery and thus any locale of the plasma membrane is a priori competent to accept incoming carriers. Therefore, while the fact that the secretory traffic responsible for apical extension is focused at the apical dome is indisputable, it remains to be established whether other carriers (for example those delivering nutrient transporters to the plasma membrane) arrive at their destination away from the hyphal tip. This alternative pathway(s) would have considerable biotechnological importance, in view of the broad use of filamentous fungi for protein production. That secretion does not require the SPK is suggested by the fact that secreted enzymesCitation31 and each and every permease that has been tested can be delivered to the septae.
In common with many other filamentous fungi, A. nidulans hyphal tip cell growth (and thus secretion) is dependent on the actin and microtubule (MT) cytoskeletons.Citation29,Citation32-Citation34 In current models, MTs mediate the long-distance transport of secretory carriers to the tip region, where these carriers switch to F-actin, using the ‘umbrella’ of filaments emanating from the SPKCitation29,Citation35 (). Possibly the strongest evidence supporting this view has been only very recently obtained.Citation36 A. nidulans contains a single myosin V (MyoV), ortholog of S. cerevisiae Myo2p. GFP-MyoV localizes to the SPK, in agreement with the prediction that this motor runs the last relay in secretion. Cells deficient in MyoV form hyphae, although these are morphologically abnormal and display markedly reduced apical extension (both features consistent with a defect in secretion). However, MyoV becomes essential in the absence of microtubules, and the combination of MyoV deficiency and triple knockout of the single kinesin-1 (KinA) and the two kinesin-3 motors (UncA and UncB) leads to a lethality-causing severe polarity defectCitation36
Figure 3. Schematic representations of the subcellular organization of key components of the A. nidulans secretory pathway. The four cartoons display the distribution of different cytoskeletal structures and compartments involved in secretion. The plus-ends of MTs (dark green) arising from the spindle pole bodies reach the tip. The Spitzenkörper located under the apical plasma membrane contains vesicles awaiting fusion with the plasma membrane and appears to be the place from which actin cables (red) radiate. The position of the subapical endocytic ring where actin patches predominate is indicated in brown. Late Golgi elements are strongly polarized and excluded from the tip region. Early Golgi elements (as labeled with Sed5 t-SNARE) and ERESs (as labeled with Sec23) are less polarized and invade the tip region. ERESs are very abundant and more numerous than early Golgi elements.
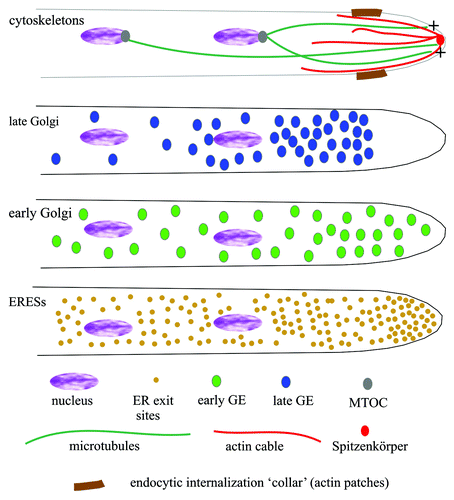
The Synergism of Genetic Studies and Microscopy
One practical consequence of the rapid apical extension of A. nidulans is that mutations impairing the efficiency of secretion lead to a detectable colony phenotype on agar plates (), making the fungus ideally suited for genetic studies on intracellular traffic. However, the potential that open-ended genome-wide (classical) genetic approaches have remains to be fully exploited. At the cellular level, the sharp decline in apical extension that results from genetic or drug-mediated impairment of secretion can be easily assessed at the level of individual tips, as growth arrest is often associated with tip swelling. This enables the observer to determine precisely, for example, the actual time at which the consequences of inactivation of a cellular function with a ts mutation become noticeable, and thus to restrict observations to an ‘informative’ time frame (see below). Another practical consequence concerns time-lapse epifluorescence studies. A. nidulans is ‘microscopy-friendly.’ As mentioned above, its cells are markedly larger than those of its yeast relatives. Importantly, hyphae cultured in microscopy chambers that are normally used for tissue cultivation become attached to the bottom glass coverslip, facilitating in vivo imaging using an inverted microscope. These microscopy chambers allow easy media exchange, enormously facilitating studies with inhibitors. Typical time-lapse studies involve 3D (x, y, z) and 4D (x, y, z, t) in one channel or in two simultaneously acquired channels, or in even more channels for the slower processes, when the time required for automated filter exchange becomes irrelevant. A recurrent concern in these studies is photo-toxicity resulting from repeated and persistent exposure to the excitation light. The fact that apical extension is highly sensitive to photo-damage allows the observer to draw conclusions only from hyphal tips that continuously undergo extension during the period of epifluorescence observation (example in and Vid. S1).
Figure 4. Time-lapse image acquisition. Three frames extracted from Video S2. Inverted contrast epifluorescence images of a hyphal tip cell stained with FM4–64, which labels the thread-like mitochondria of the tip region. The reason why, in many filamentous fungi, FM4-64 labels the membranes of mitochondria, in addition to labeling endocytic membranes, is unknown.
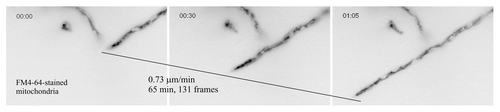
The Jewel in the Crown: The Golgi Apparatus
Efficient secretion immediately rings Golgi bells for cell biologists. Thus readers may be surprised that live microscopy studies on the Golgi and its dynamics started only very recently. In one of these founding studies, Breakspear et al.Citation37 used GFP-CopA (α-COP) to show that, as in most ascomycetes including S. cerevisiae, the A. nidulans Golgi is not stacked, being formed instead by discrete cytoplasmic bodies. Some of these bodies are ring-shaped, and thus almost certainly correspond to the ring structures that had previously been observed in electron micrographs of fungal hyphae.Citation38,Citation39 For reasons that will become apparent below, an important observation was that CopA-containing bodies are strongly polarized toward the tip. Another study reported that Arf1 (ArfA in A. nidulans) localizes to similar bodies and, importantly, that these bodies were aggregated by brefeldin A, a drug targeting Sec7, the GEF (GDP exchange factor) for Arf1.Citation40 Meanwhile our laboratory set out to study Golgi maturation by using ‘vigorous’ Golgi reporters that withstand 4D microscopy over relatively long periods of time. We initially used the fluorescently-tagged PH domain of human oxysterol binding protein (PHOSBP), a highly specific, largely PtdIns(4)P-dependent, probe of the late-Golgi that Levine and Munro had thoroughly characterized in S. cerevisiae.Citation41 Time-lapse observations of hyphae expressing this probe revealed an astonishingly dynamic network of ring-shaped cytosolic bodies (Golgi equivalents, GE) connected by transient, micron-long tubular structures. The absolute co-localization of GEs labeled with PHOSBP with the prototypical late Golgi resident Sec7 confirmed unambiguously that these cytoplasmic bodies represent late GEs. Moreover, image deconvolution revealed with outstanding degree of detail a network of fenestrated structures resembling the EM images cited aboveCitation34 (Vid. S2). As predicted by the fact that apical extension is not arrested during mitosis,Citation16,Citation32 late GEs did not disassemble during M-phase (the nuclei of the tip cell enter mitosis in a nearly synchronous manner). Late GEs are very strongly polarized, largely predominating between the two apex-proximal nuclei and the apex, but they are clearly excluded from the tip region (). This exclusion of late GEs from the tip enabled visualization of strings of Golgi-derived material detaching from the tip-proximal edge of GEs and arriving at the apical dome.Citation34 This material possibly consists of secretory carriers. Thus the technology was ripe for more ambitious objectives.
The Organization of the A. nidulans Golgi Apparatus
The non-stacked organization of the fungal Golgi allows the resolution by optical microscopy of early and late cisternae.Citation42 This was exploited by the Glick and Nakano laboratories for their groundbreaking in vivo microscopy analyses of S. cerevisiae Golgi dynamics,Citation43,Citation44 which provided strong support for the cisternal maturation model.Citation45-Citation47 A. nidulans PHOSBP-labeled late GEs are indeed ‘optically resolvable’ from cisternae containing the Golgi syntaxin Sed5.Citation48 Sed5 is a well-established early Golgi marker in yeast.Citation42,Citation44 A. nidulans early and late cisternae are intermingled across the apical tip cell (regretfully there is not a base-to-apex gradient of early-to-late cisternae!). However, a low yet detectable proportion of GEs contain both early and late Golgi reporters, which is consistent with these GEs representing cisternae captured at an intermediate step of maturation. Studies on cisternal maturation are currently underway. One notable observation though is that early Golgi cisternae invade the actual tip (), a territory from which late GEs are absent. This observation has an as yet unexplored implication: that cisternal maturation may proceed countercurrent to secretion in the tip region.
As noted above, the use of microscopy chambers facilitated studies with inhibitors. Initial emphasis was put on brefeldin A (BFA), a keystone of Golgi studies in mammalian cells. In agreement with data on ArfAArf1 mentioned above, late A. nidulans GEs aggregated upon addition of BFA, even though drug concentrations as high as 100–300 μg/ml were needed to observe an effect.Citation34 The need for such high concentrations was somewhat expected, as the natural producer of brefeldins is a Penicillium—a related ascomycete filamentous fungus—species [and strains did not carry ergosterol biosynthetic mutations to hypersensitize them to the drug (as used in S. cerevisiaeCitation49)]. What was unexpected is that, unlike in mammalian cells,Citation50 BFA does not lead to reabsorption of the A. nidulans Golgi into the ER.Citation34 Subsequent studies demonstrated that early Golgi bodies also aggregate with BFA,Citation48 opening up the possibility of studying the biogenesis of the normal Golgi network starting from these aggregates. Aggregates of early and late Golgi cisternae remain segregated from each other upon BFA treatment, implying that the Golgi maintains its asymmetry even under such disruptive conditions.Citation48
A further difference between early and late GEs is that the former are clearly less polarized than the latter.Citation34,Citation48 This is important because GFP-CopACop1-containing GEs are strongly polarized,Citation37 which appears to suggest that, in the steady-state, CopACop1 resides in the late Golgi. This possibility is supported by the finding that CopACop1 does not co-localize with the GDP-mannose transporter GmtA,Citation51 whose yeast Vrg4p ortholog is a demonstrated early Golgi resident.Citation43 The potential late Golgi localization of CopACop1 would need to be further investigated using other Golgi markers. CopACop1 is a component of the coat protein I (COPI) complex. In view of the demonstrated role of COPI in the formation of tubular Golgi connections,Citation52 it is tempting to speculate that CopACop1 may be mechanistically important for the formation of tubules networking A. nidulans GEs. Transient tubules connecting cisternae are more frequently observed in tip proximal regions than in posterior regions, where cisternae are more isolated. We suggest that A. nidulans may help to gain insight into the role of transient tubular connections interconnecting cisternae, an issue currently under debate.Citation53
An open question is what determines the strong polarization of late GEs. Early Golgi cisternae form in the proximity of ER exit sites (ERESs).Citation54,Citation55 Thus one possibility would be that ERESs and, consequently, early GEs are also strongly polarized themselves. However, microscopy of Sec23-GFP ERESsCitation34 and of GFP-Sed5 early GEsCitation48 showed that these markers display some polarization, but that this polarization is clearly less marked than that of late GEs (). (In passing, A. nidulans ERESs are markedly abundant, in agreement with the view that the non-stacked appearance of the Golgi of many fungi reflects the dispersion and multiplicity of ERESs.Citation56)
Given that the weaker polarization of ERESs and early GEs cannot account for the stronger late GE polarization, it seems that the latter might be actively promoted. Neither microtubule depolymerization nor the dynein nudA1ts mutation has any effect on the polarization or integrity of the GEs.Citation34,Citation37 However, anti-F-actin treatment had a dramatic effect. First, it promoted GE depolarization within 15–20 min, suggesting that the effect is direct [note that this treatment affect endocytosis as well as (also F-actin dependent) secretion]. In apparent agreement with a direct role for F-actin in determining the organization of the Golgi, we also found that the morphology of late GEs is markedly affected, and their size significantly reduced, by anti-actin treatment. All these changes revert when the anti-actin drug is washed out, correlating with migration of depolarized GEs into the secondary axes of polarity that emerge when actin polymerization is restored.Citation34 Thus, these results suggest that the highly tip-biased distribution of late GEs might involve actin cables.Citation34 Notably, another unexplored finding is that a rabCΔ mutation (rabC encodes the A. nidulans Rab6 ortholog, see below) resembles anti-actin treatment in that it also results in Golgi fragmentation.
RabCRab6, a Key Organizer of the Golgi and Beyond
RabCRab6, the only Rab6 homolog in A. nidulans, is not essential, but its absence results in markedly impaired apical extension leading to very small coloniesCitation48 (). Its clearly important physiological role is consistent with the multiplicity of roles that this Rab might play at the Golgi. One such role almost certainly involves the regulation of retrograde endosome-to-Golgi traffic, as in S. cerevisiaeCitation57,Citation58 (and in mammalian cells). This conclusion is based on the observations that rabCΔ results in a conspicuous reduction in the size of GEs, in a marked increase in the number of early endosomes and in mislocalization of the vacuolar hydrolase receptor VpsTVps10 (that recycles between the Golgi and the endosomes) to a cytosolic haze.
Another important observation was that in the absence of RabCRab6 early and late GEs become insensitive to BFA-induced aggregation, strongly indicating that RabCRab6 contributes to the dynamic organization of the Golgi network. The Golgi localization of RabCRab6 itself is remarkable. In tip-distal regions, it preferentially colocalizes with late GEs. In tip-proximal regions RabCRab6 preferentially colocalizes with Sed5 (thus with early GEs), although, in addition, it labels, more faintly, a highly dynamic network of tubular structures.Citation48 These position-dependent changes in RabCRab6 localization would be consistent with a scenario in which the Golgi dynamics change with the distance to the tip. We hypothesize that incoming and outgoing Golgi traffic may reach a different balance depending on the different secretory activities of tip-proximal and tip-distal regions. Thus, the preferential association of RabCRab6 with late GEs observed in tip-distal regions, where secretory activity is presumably low, might indicate that RabCRab6-mediated retrograde endosome-to-late Golgi traffic is more active in these locales. In contrast, its preferential association with early GEs near the tip might indicate that RabCRab6 plays a role in intra-Golgi traffic that is important to maintain the high secretory activity of the tip region. This highly speculative view is a ‘subcellular version’ of the different distribution and sizes that the Golgi shows in different mammalian cell types.Citation59 Of note, this ´ la carte position-dependent organization of the Golgi might be facilitated because within the same cell the multiplicity of ERESs and the dispersed organization of Golgi cisternae provide the necessary flexibility to the system as a whole.
An unexpected finding was that RabCRab6 is also present in the SPK, the apical structure where secretory carriers accumulate before fusing with the PM. rabCΔ results in a more prominent SPK, suggesting that the fusogenicity of these carriers is impaired. The possibility that a Rab6 protein regulates this fusion step has a precedent in mammalian cells, in which docking of secretory vesicles to the PM requires the Rab6 effector Rab6IP2/ELKS/CAST2.Citation60
Endocytosis and Exocytosis are Spatially Coupled in the Tip
Studies with FP-tagged components of the endocytic internalization machinery revealed that endocytic sites strongly predominate at the tip, concentrating in a subapical collar located immediately behind the apical dome.Citation29,Citation61,Citation62 This finding led to the suggestion that the persistently polarized growth of fungal hyphae requires the spatial coupling at the tip of secretion and endocytosis. In agreement, mutational inactivation of fimbrin (an F-actin cross-linking protein that plays a role in endocytic internalization) or ArfBArf6 (a small GTPase involved in the regulation of endocytosis) leads to polarity defects.Citation62,Citation63 Another strong case came from studies of SlaBSla2, a component of the endocytic internalization machinery that couples endocytic vesicle formation to actin polymerization.Citation64 slaBΔ leads to lethality: slaBΔ conidia are able to establish, but unable to maintain, polarity, arresting growth with a yeast-like morphotypeCitation61 This was convincingly documented with SlaB downregulation experiments in which normal polarity maintenance was permitted for a period of time before shutting SlaB synthesis off, which resulted in normal hyphal tips undergoing dramatic defects in apical extension.Citation65 Notably, abnormal hyphal tips downregulated for SlaBSla2 showed strings of F-actin material dangling from the cortex,Citation65 resembling those observed by Drubin’s group in S. cerevisiae sla2Δ cells.Citation64 This agrees with the view that Sla2p family proteins couple endocytic vesicle formation to actin polymerization.Citation64 Another remarkable observation was that the plasma membrane of the abnormal yeast-like A. nidulans cells formed by conidiospores germinated in the absence of SlaBSla2 contains deep, several microns wide invaginations, presumably resulting from aberrant, unrestrained endocytic pits. These deep invaginations might be exploited to study basic aspects of endocytic internalization (Vid. S3).Citation65
A prototypical cargo of the endocytic internalization ring is the v-SNARE SynA. Its S. cerevisiae Snc1p homolog is kinetically polarized by localized delivery to the plasma membrane followed by efficient endocytosis and recycling back to the plasma membrane.Citation66 SynA strongly predominates in the plasma membrane of the apical dome and, like Snc1p, is kinetically polarized, being one of the substrates of the subapical endocytic collar.Citation29,Citation30 Anti-actin treatment,Citation29 SlaBSla2 downregulationCitation65 or mutational inactivation of the SynA endocytic sorting motifCitation48 allows SynA to diffuse away from the tip, losing its polarized localization. The fact that membrane-associated proteins can kinetically polarize raises the attractive possibility that the spatial coupling of secretion and endocytosis at the hyphal tips might maintain the polarized distribution of proteins (and lipids) which determine the directionality of secretion/growth, such as the GTPases Cdc42 and Rac1Citation67 or the MesA transmembrane protein, which localizes to the tip and facilitates the recruitment of the SepA formin.Citation35 Obviously endocytic recycling may additionally improve the sustainability and efficiency of secretion toward the apex through efficient reutilization of membrane fusion machinery proteins, such as SynA itself.
Endosome Traffic: A Silver Mine
This is arguably the area where mining of A. nidulans intracellular traffic has provided most loot. Research on A. nidulans early endosomes was encouraged by the landmark achievements of Gero Steinberg’s group using Ustilago maydis.Citation68-Citation70
Rab GTPases are major determinants of membrane identity.Citation71-Citation73 A few years ago we undertook a systematic characterization of all 10 A. nidulans Rabs. These include two Rab5 paralogues, RabA and RabB, on early endosomes;Citation30,Citation74 one late endosome/vacuole RabSRab7 (ref. Citation74 and unpublished results); three Golgi Rabs, including Rab1 (under characterization), RabCRab6 (ref. Citation48) and Rab11 (ref. Citation74 and unpublished); one Sec4p homolog; two Rabs of uncertain role belonging to the Rab2/Rab4/Rab14 subgroup and, lastly, one completely unrelated to most other Rabs, that we have denoted RabX.
A. nidulans early endosomes containing the two Rab5 paralogues, RabA and RabB, undergo a characteristic long-distance bi-directional movement on MTs, powered by dynein and kinesins-3.Citation30,Citation69,Citation74-Citation77 Endosomes often move in one direction for some distance before arresting and shifting the direction of movement, giving a characteristic V-shaped pattern in kymographs (). Studies with U. maydis have shown that this behavior reflects a tug-of-war between kinesin-3 and dynein molecules bound to the same endosome.Citation68 At 25°C, the average speed of A. nidulans endosomes in either acropetal (toward the tip) or basipetal (away from the tip) direction is similar, in the order of 2.5 μm/sec. This characteristic motility of early endosomes can be exploited to differentiate them unmistakably from late/mature endosomes, which are essentially static within this time scale. It has also been exploited to understand key aspects of dynein function.
Figure 5. Kymographs of early endosomes. Analysis of endosomal movement using kymographs: High-density-of-frame time-lapse sequences in the GFP channel were acquired over the time indicated in the vertical axes. Fluorescent signals detected across a line traced along the cytoplasm of hyphae, wide enough to cover the complete width of the cell, were plotted against time. In this type of representation, vertical lines correspond to static signals, whereas moving structures appear as diagonals, whose slope indicates the speed and direction of movement. (A) Kymograph showing early endosomes visualized with GFP-RabB. The prominent endosome indicated with asterisks moves in one direction for 17 μm before abruptly shifting movement toward the opposite direction. (B) As above, using GFP-RabA. In one of the two examples shown (*), an endosome moves for 16 μm before becoming essentially static for the remaining period of observation. Another endosome (**) enters the region analyzed, moving in the opposite direction, and does not slow down over the remaining duration of the time-series.
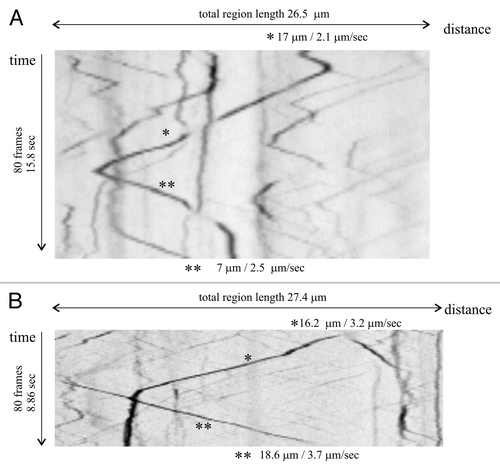
In A. nidulans (and in U. maydis) hyphae, MTs in the tip region are oriented with their plus ends toward the apexCitation78 (). Dynein is transported to these plus-ends by the conventional kinesin KinA.Citation77,Citation79 A fraction of acropetally moving endosomes actually reaches the tip before shifting the direction of movement, upon being loaded on dynein. In addition, endosomes originating from the highly active subapical endocytic ring are also loaded on dynein in this region, that has been labeled the “dynein loading zone.”Citation69 Thus, impairing either the function of dynein or its plus-end delivery by KinA prevents departure of early endosomes from the tip, resulting in a characteristic large aggregate of early endosomal membranes. This tip aggregate has been denoted the abnormal “NudA compartment” because it is never seen in the wild-type and because it is particularly conspicuous in cells carrying nudA1ts (a thermo-sensitive mutation isolated in the seminal nud screen) or nudAΔ alleles of the dynein heavy chain gene nudA.Citation30,Citation75,Citation77 The diagnostic value that this tip endosomal aggregate represents with regard to dynein function has been exploited to understand the role of other proteins in the dynein pathway. For example, deficiency of NudF/LIS1 also leads to the tip endosomal aggregate, indicating that NudF promotes the dynein-mediated basipetal transport of endosomes.Citation69,Citation77 Understanding this positive-acting role of NudF is crucial to understanding of the physiopathology of lissencephaly. NudF is not required to activate the ATPase cycle at the plus-ends, as this cycle appears to be active even in the molecules that are in the process of being transported to the plus ends. Instead, dynein isolated from NudF-deficient cells appears to have less affinity for MTs,Citation77 thus suggesting that NudF is required for the productive engagement of endosome-loaded dynein complexes with these cytoskeletal structures.
Possibly the largest gem extracted from the endosomal mine so far is Xiang’s lab findingCitation76 that the p25 subunit of dynactin (a dynein accessory complex) is specifically required for the interaction of endosomes with the dynein complex (the regulation of cytoplasmic dynein is reviewed in ref. Citation80). In contrast with loss-of-function mutations affecting the cytoplasmic dynein heavy chain NudA or the dynactin components p150, Arp1, Arp11, p62 and p50, all resulting in a nud phenotype, dynactin p25Δ does not affect nuclear distribution and shows a much milder colony growth phenotype than mutations affecting any of the above components. However, p25Δ resembles nudAΔ and nudFΔ in that it leads to a very prominent aggregate of endosomes in the tip region, indicative of major impairment of dynein function in early endosome transport.Citation76 In hyphal tips deficient in NudF, the NudA dynein heavy chain and the p150 component of dynactin accumulate with the typical tip aggregate of early endosomes, in agreement with the view that in this mutant background cytoplasmic dynein is unable to engage microtubules productively. This NudA and p150 accumulation was never seen in NudF-deficient cells also carrying the p25Δ mutation. Thus, NudA and p150 are retained with the aggregate of early endosomes in hyphal tips deficient in NudF, and this retention necessitates p25, strongly indicating that p25 links dynein to early endosomesCitation76
Whereas the basipetal dynein-mediated movement of endosomes appears to be required for maturation (see below), the physiological role of the anterograde movement is still in need of a satisfactory explanation. One attractive, although not the only, possibility is that anterograde movement of early endosomes facilitates recycling to the plasma membrane of cargo that arrives at the endosomal system but is not destined for degradation. (Even though endocytosis predominates in the tip, there are endocytic sites in more basal regions.) Redelivery of material undergoing recycling to the plasma membrane can plausibly be facilitated if endosomes move to the tip region where secretion predominates. In this model, endosomes arriving at the tip region would be subjected to sorting mechanisms by which endosomal domains/cargo destined to the PM would be segregated from endosomal domains/cargo destined for degradation, which would undergo dynein-mediated basipetal movement. The abnormal aggregation of endosomal membranes resulting from impairment of the dynein pathway would be expected to interfere with the sorting of these domains. Therefore, the fact that the prototypical recycling cargo SynA is stranded in the endosomal aggregates of nudA1ts tips is consistent with (although does not prove) this interpretation.Citation30
Pioneering work of Zerial's lab using mammalian cells strongly suggested that endosomal maturation occurs by Rab5-to-Rab7 conversion.Citation81 These studies were buttressed by the equally groundbreaking work of Ungermann’s lab on the biochemical characterization of oligomeric effectors of Vps21p (yeast Rab5) and Ypt7p (yeast Rab7).Citation82,Citation83 Although some details of the endosomal maturation model remain to be fully delineated,Citation72 the findings that metazoan SAND-1/Mon1 complexCitation84 or its yeast equivalent, the Mon1/Ccz1 complexCitation85 are Rab5 effectors that function as GEFs for Rab7 provided strong support to the view that endosomal maturation is driven by Rab conversion. An oversimplified version of the current models is that early endosomes containing the Rab5 oligomeric effector CORVET are ‘converted’ into late endosomes/vacuoles containing the Rab7 effector HOPS.Citation86 CORVET shares with HOPS the “core” subunits Vps11, Vps16, Vps18 and Vps33. CORVET and HOPS additionally contain two specific subunits each, Vps8 and Vps3 in CORVET and Vps41 and Vps39 in HOPS. Of relevance here, A. nidulans provided the second example in which CORVET was identified as a Rab5 effector: RabB and, to a lesser extent, RabA recruit to early endosomes the CORVET complex,Citation74 whereas RabSRab7 recruits HOPS (our unpublished results). Thus data in A. nidulans buttressed the conclusions obtained with yeast. In addition, studies on A. nidulans Rab5s provided new avenues for investigation: (1) the double rabAΔ rabBΔ mutation is lethal, as is the single vps8Δ mutation, indicating that endosomal maturation is essential for A. nidulans. (2) A. nidulans has a single syntaxin (Pep12) for prevacuolar endosomes and vacuoles. RabB is the main recruiter to endosomes of the SM protein Vps45 (also essential), which regulates Pep12 at prevacuolar endosomes to accept incoming Golgi traffic. Notably, Pep12 must be regulated by another SM protein, the CORVET and HOPS component Vps33, on both early and late endosomes. How the same syntaxin is regulated by different SM proteins, and at three different levels, is an important and as yet unresolved issue.
In A. nidulans, endosomal maturation appears to parallel a decrease in motility of the endosomes. This is strongly supported by experiments with the endocytic tracer FM4-64 that, after reaching the population of motile early endosomes, arrives at a class of punctate structures which are essentially immotile, almost certainly representing “late” endosomes/small vacuoles.Citation87 Thus it seems reasonable to assume that as endosomes undergo homotypic fusion, the resulting increase in size leads to a parallel reduction in motility (). Therefore motility (or lack of) can be used as a diagnostic feature to classify endosomes in studies of endosomal maturation. In addition to the size increase, a second factor that contributes to the loss of endosomal motility is, almost certainly, the substitution of RabB by RabSRab7, as RabB is specifically required for early endosome movement.Citation74 Thus, the process of endosomal maturation in A. nidulans resembles that of mammalian cells in that it involves dynein-dependent movement of early endosomes and in that motility of early endosomes is gradually lost as they increase in size, maturing into late endosomes,
RabB must play a key role in determining the identity of endosomal membrane domains destined to the vacuole, as it appears to be the only recruiter to endosomes of the PtdIns(3)P kinase Vps34. The lipid messenger PtdIns(3)P triggers the multivesicular body pathway and is therefore the landmark of endosomal degradative identity. This led us to conclude that multivesicular body sorting operates already at the level of early endosomes. Indeed motile early endosomes were shown to recruit the PtdIns(3)P-binding FYVE motif of Vps27 and contain ESCRT-III Vps32 and the “late” ESCRT-III accessory factor DidB.Citation30,Citation88,Citation89 The essential role of endosomal maturation extends to the multivesicular body pathway. Null mutations in ESCRT-0, -I, -II and -III components result in near-lethality.Citation90 However, their severely debilitating phenotype can be suppressed by loss-of-function mutations in a gene encoding an enigmatic zinc-finger transcription factor, denoted SltA, which regulates cation homeostasis.Citation90-Citation92 By itself, sltA loss-of-function leads to a very conspicuous hypertrophy of the vacuolar system.Citation91 By exploiting a conditional sltA allele, Calcagno-Pizarelli et al. showed that under sltA+ conditions ESCRT null mutations lead to very small vacuoles.Citation90 Subsequent inactivation of sltA, which rescues growth of ESCRT mutants, also rescues the normal vacuole phenotype.Citation90 These data suggest that the absence of endosomal maturation impairs the homotypic fusion of prevacuolar endosomes and that this fusion can be restored by mis-regulation of genes controlled by SltA. In view of the demonstrated role of calcium in homotypic vacuolar fusion,Citation93 it is highly suggestive that two vacuolar calcium pumps are upregulated by sltAΔ.Citation91 Of note, S. cerevisiae, in which ESCRT null mutants are viable, lacks a sltA homolog.
Then, Why Should I Buy Aspergillus for Trafficking Studies?
In a Dickensian world of fungal genetic models A. nidulans appears inevitably destined to be a poor relation to S. cerevisiae, whose wealth in genetic and biochemical tools and resources of all kinds cannot be matched by any other competitor. However, even in genetic terms, A. nidulans has certain specific advantages. A. nidulans has almost twice the number of genes as S. cerevisiae [AspGD (www.aspgd.org), a web facility modeled on the S. cerevisiae Genome Database (SGD) and the Broad Institute “Aspergillus Comparative Database” (www.broadinstitute.org/annotation/genome/aspergillus_group) provide the necessary backup of genomic information]. However, A. nidulans has an almost complete absence of genetic redundancy as, unlike the budding yeast, it has not undergone genome duplication. Second, A. nidulans grows by apical extension and does not undergo changes in polarity with progression through the nuclear division cycle. Third, mutations impaired in secretion, even to a minor extent, result in a detectable colony phenotype. Lastly, endocytosis cooperates with secretion to maintain high rates of apical extension, and thus mutations impairing endocytosis are also translatable into a colony phenotype.
Readers should also note that the level of similarity between human and A. nidulans orthologs is usually higher than that between budding yeast and humans. This greater similarity extends to overall aspects of cell physiology, such as metabolism. The characterization of historical “inborn errors of metabolism” using A. nidulans models of diseaseCitation94-Citation96 testifies to this similarity.
On the practical side, A. nidulans is very well suited for in vivo microscopy studies of secretion, not only because it has a natural adhesion to glass coverslips but, more importantly, because it allows direct correlation, at the single cell (hyphal tip) level of an experimental intervention (be it a thermo-sensitive mutation and temperature shift or an interfering drug addition/removal) with its consequences in intracellular traffic: hyphal tips arrested in apical extension present characteristic morphological abnormalities, permitting the researcher to determine, for example, the precise time point in which a ts mutation leads to a detectable cellular phenotype after a temperature shift. Additionally, the arrest in apical extension brought about by excessive cell irradiation in epifluorescence studies is a useful diagnostic tool to identify photo-damaged cells.
Irrespective of the value of these advantages, unquestionably the keyword that comes to mind when thinking on A. nidulans traffic is distance. The large size of the hyphal tip cell facilitates studies with conventional microscopy, as the organelles are less crowded than in the unicellular yeasts. A more fundamental advantage is that intracellular transport is highly dependent on the MT cytoskeleton, allowing studies of processes that involve MT-dependent transport. Thus it not surprising that the most important contributions of A. nidulans trafficking studies thus far come from the field of endosomal traffic, which is coupled to MT-dependent movement.
Conclusions: Time for Progress
In summary, some jewels have been extracted from mining A. nidulans intracellular traffic but only the future will tell whether the riches will rival those mined by Ron Morris and his colleagues in the analysis of mitosis. The system is sufficiently mature to study, for example, how Rab conversion across the endosomal and Golgi systems is orchestrated and how molecular order underlies the apparently chaotic morphological organization of the Golgi. However, the achievement of these ambitious objectives will require in-depth biochemical and genetic approaches, in addition to the full power of in vivo microscopy. Secretion and endocytosis are essential. Thus genetic approaches should necessarily rely on conditional or partial loss-of-function mutations, exploiting the fact that minor disruptions in anterograde and retrograde traffic must be reflected in impaired apical extension and, in turn, in a colony phenotype. One last yet important point is that other model fungi, most notably Neurospora crassa and U. maydis, which are only very briefly mentioned in this commentary, have been and will be important contributors to our understanding of intracellular traffic.
Abbreviations: | ||
BFA | = | brefeldin A |
ERE(s) | = | endoplasmic reticulum exit site(s) |
ESCRT | = | endosomal sorting complex required for transport |
FP | = | fluorescent protein |
GE | = | Golgi equivalent |
MPF | = | maturation promoting factor |
MT | = | microtubules |
PM | = | plasma membrane |
SPK | = | Spitzenkörper |
Additional material
Download Zip (3.7 MB)Acknowledgments
Work in the Madrid/London laboratories is currently supported by Spanish Ministerio de Ciencia y Tecnologi´a grant BIO2009–7281 (M.A.P.), Comunidad de Madrid grant SAL/0246/2006 (M.A.P.), Biotechnology and Biological Sciences Research Council grant BB/F01189X/1 (H.N.A. and Elaine Bignell) and Wellcome Trust grant 084660/Z/08/Z (H.N.A and Joan Tilburn).
References
- Morris NR, Enos AP. Mitotic gold in a mold: Aspergillus genetics and the biology of mitosis. Trends Genet 1992; 8:32 - 3; http://dx.doi.org/10.1016/0168-9525(92)90022-V; PMID: 1369734
- Osmani SA, Mirabito PM. The early impact of genetics on our understanding of cell cycle regulation in Aspergillus nidulans. Fungal Genet Biol 2004; 41:401 - 10; http://dx.doi.org/10.1016/j.fgb.2003.11.009; PMID: 14998523
- Pontecorvo G, Roper JA, Hemmons LM, MacDonald KD, Bufton AWJ. The genetics of Aspergillus nidulans. Adv Genet 1953; 5:141 - 238; http://dx.doi.org/10.1016/S0065-2660(08)60408-3; PMID: 13040135
- Cohen BL. Guido Pontecorvo (“Ponte”): a centenary memoir. Genetics 2007; 177:1439 - 44; PMID: 18039877
- Wilson RA, Arst HN Jr.. Mutational analysis of AREA, a transcriptional activator mediating nitrogen metabolite repression in Aspergillus nidulans and a member of the “streetwise” GATA family of transcription factors. Microbiol Mol Biol Rev 1998; 62:586 - 96; PMID: 9729601
- Todd RB, Davis MA, Hynes MJ. Genetic manipulation of Aspergillus nidulans: heterokaryons and diploids for dominance, complementation and haploidization analyses. Nat Protoc 2007; 2:822 - 30; http://dx.doi.org/10.1038/nprot.2007.113; PMID: 17446882
- Todd RB, Davis MA, Hynes MJ. Genetic manipulation of Aspergillus nidulans: meiotic progeny for genetic analysis and strain construction. Nat Protoc 2007; 2:811 - 21; http://dx.doi.org/10.1038/nprot.2007.112; PMID: 17446881
- Morris NR. Mitotic mutants of Aspergillus nidulans. Genet Res 1975; 26:237 - 54; http://dx.doi.org/10.1017/S0016672300016049; PMID: 773766
- Enos AP, Morris NR. Mutation of a gene that encodes a kinesin-like protein blocks nuclear division in A. nidulans. Cell 1990; 60:1019 - 27; http://dx.doi.org/10.1016/0092-8674(90)90350-N; PMID: 2138511
- Osmani SA, Engle DB, Doonan JH, Morris NR. Spindle formation and chromatin condensation in cells blocked at interphase by mutation of a negative cell cycle control gene. Cell 1988; 52:241 - 51; http://dx.doi.org/10.1016/0092-8674(88)90513-2; PMID: 3277718
- Osmani SA, Pu RT, Morris NR. Mitotic induction and maintenance by overexpression of a G2-specific gene that encodes a potential protein kinase. Cell 1988; 53:237 - 44; http://dx.doi.org/10.1016/0092-8674(88)90385-6; PMID: 3359487
- O’Connell MJ, Krien MJ, Hunter T. Never say never. The NIMA-related protein kinases in mitotic control. Trends Cell Biol 2003; 13:221 - 8; http://dx.doi.org/10.1016/S0962-8924(03)00056-4; PMID: 12742165
- Doonan JH, Morris NR. The bimG gene of Aspergillus nidulans, required for completion of anaphase, encodes a homolog of mammalian phosphoprotein phosphatase 1. Cell 1989; 57:987 - 96; http://dx.doi.org/10.1016/0092-8674(89)90337-1; PMID: 2544297
- Etxebeste O, Garzi´a A, Espeso EA, Ugalde U. Aspergillus nidulans asexual development: making the most of cellular modules. Trends Microbiol 2010; 18:569 - 76; http://dx.doi.org/10.1016/j.tim.2010.09.007; PMID: 21035346
- Pruyne D, Legesse-Miller A, Gao L, Dong Y, Bretscher A. Mechanisms of polarized growth and organelle segregation in yeast. Annu Rev Cell Dev Biol 2004; 20:559 - 91; http://dx.doi.org/10.1146/annurev.cellbio.20.010403.103108; PMID: 15473852
- Riquelme M, Fischer R, Bartnicki-Garci´a S. Apical growth and mitosis are independent processes in Aspergillus nidulans. Protoplasma 2003; 222:211 - 5; http://dx.doi.org/10.1007/s00709-003-0020-8; PMID: 14714210
- Momany M. Polarity in filamentous fungi: establishment, maintenance and new axes. Curr Opin Microbiol 2002; 5:580 - 5; http://dx.doi.org/10.1016/S1369-5274(02)00368-5; PMID: 12457701
- Fiddy C, Trinci AP. Mitosis, septation, branching and the duplication cycle in Aspergillus nidulans. J Gen Microbiol 1976; 97:169 - 84; PMID: 796408
- Fischer R. Nuclear movement in filamentous fungi. FEMS Microbiol Rev 1999; 23:39 - 68; http://dx.doi.org/10.1111/j.1574-6976.1999.tb00391.x; PMID: 10077853
- Morris NR, Lai MH, Oakley CE. Identification of a gene for α-tubulin in Aspergillus nidulans. Cell 1979; 16:437 - 42; http://dx.doi.org/10.1016/0092-8674(79)90019-9; PMID: 378391
- Sheir-Neiss G, Lai MH, Morris NR. Identification of a gene for β-tubulin in Aspergillus nidulans. Cell 1978; 15:639 - 47; http://dx.doi.org/10.1016/0092-8674(78)90032-6; PMID: 363278
- Oakley CE, Oakley BR. Identification of γ-tubulin, a new member of the tubulin superfamily encoded by mipA gene of Aspergillus nidulans. Nature 1989; 338:662 - 4; http://dx.doi.org/10.1038/338662a0; PMID: 2649796
- Oakley BR, Morris NR. Nuclear movement is β--tubulin-dependent in Aspergillus nidulans. Cell 1980; 19:255 - 62; http://dx.doi.org/10.1016/0092-8674(80)90407-9; PMID: 6986988
- Xiang X, Beckwith SM, Morris NR. Cytoplasmic dynein is involved in nuclear migration in Aspergillus nidulans. Proc Natl Acad Sci U S A 1994; 91:2100 - 4; http://dx.doi.org/10.1073/pnas.91.6.2100; PMID: 8134356
- Xiang X, Osmani AH, Osmani SA, Xin M, Morris NR. NudF, a nuclear migration gene in Aspergillus nidulans, is similar to the human LIS-1 gene required for neuronal migration. Mol Biol Cell 1995; 6:297 - 310; PMID: 7612965
- Harris SD, Read ND, Roberson RW, Shaw B, Seiler S, Plamann M, et al. Polarisome meets spitzenkörper: microscopy, genetics, and genomics converge. Eukaryot Cell 2005; 4:225 - 9; http://dx.doi.org/10.1128/EC.4.2.225-229.2005; PMID: 15701784
- Hohmann-Marriott MF, Uchida M, van de Meene AM, Garret M, Hjelm BE, Kokoori S, et al. Application of electron tomography to fungal ultrastructure studies. New Phytol 2006; 172:208 - 20; http://dx.doi.org/10.1111/j.1469-8137.2006.01868.x; PMID: 16995909
- Sharpless KE, Harris SD. Functional characterization and localization of the Aspergillus nidulans formin SEPA. Mol Biol Cell 2002; 13:469 - 79; http://dx.doi.org/10.1091/mbc.01-07-0356; PMID: 11854405
- Taheri-Talesh N, Horio T, Araujo-Baz´n L, Dou X, Espeso EA, Peñalva MA, et al. The tip growth apparatus of Aspergillus nidulans. Mol Biol Cell 2008; 19:1439 - 49; http://dx.doi.org/10.1091/mbc.E07-05-0464; PMID: 18216285
- Abenza JF, Pantazopoulou A, Rodri´guez JM, Galindo A, Peñalva MA. Long-distance movement of Aspergillus nidulans early endosomes on microtubule tracks. Traffic 2009; 10:57 - 75; http://dx.doi.org/10.1111/j.1600-0854.2008.00848.x; PMID: 19000168
- Hayakawa Y, Ishikawa E, Shoji JY, Nakano H, Kitamoto K. Septum-directed secretion in the filamentous fungus Aspergillus oryzae. Mol Microbiol 2011; 81:40 - 55; http://dx.doi.org/10.1111/j.1365-2958.2011.07700.x; PMID: 21564341
- Horio T, Oakley BR. The role of microtubules in rapid hyphal tip growth of Aspergillus nidulans. Mol Biol Cell 2005; 16:918 - 26; http://dx.doi.org/10.1091/mbc.E04-09-0798; PMID: 15548594
- Torralba S, Raudaskoski M, Pedregosa AM, Laborda F. Effect of cytochalasin A on apical growth, actin cytoskeleton organization and enzyme secretion in Aspergillus nidulans. Microbiology 1998; 144:45 - 53; http://dx.doi.org/10.1099/00221287-144-1-45; PMID: 9537763
- Pantazopoulou A, Peñalva MA. Organization and dynamics of the Aspergillus nidulans Golgi during apical extension and mitosis. Mol Biol Cell 2009; 20:4335 - 47; http://dx.doi.org/10.1091/mbc.E09-03-0254; PMID: 19692566
- Pearson CL, Xu K, Sharpless KE, Harris SD. MesA, a novel fungal protein required for the stabilization of polarity axes in Aspergillus nidulans. Mol Biol Cell 2004; 15:3658 - 72; http://dx.doi.org/10.1091/mbc.E03-11-0803; PMID: 15155805
- Zhang JT, Tan K, Wu X, Chen G, Sun J, Reck-Peterson SL, et al. Aspergillus Myosin-v supports polarized growth in the absence of microtubule-based transport. PLoS One 2011; 6:e28575; http://dx.doi.org/10.1371/journal.pone.0028575; PMID: 22194856
- Breakspear A, Langford KJ, Momany M, Assinder SJ. CopA:GFP localizes to putative Golgi equivalents in Aspergillus nidulans. FEMS Microbiol Lett 2007; 277:90 - 7; http://dx.doi.org/10.1111/j.1574-6968.2007.00945.x; PMID: 17986089
- Howard RJ. Ultrastructural analysis of hyphal tip cell growth in fungi: Spitzenkörper, cytoskeleton and endomembranes after freeze-substitution. J Cell Sci 1981; 48:89 - 103; PMID: 7196918
- Momany M, Richardson EA, Van Sickle C, Jedd G. Mapping Woronin body position in Aspergillus nidulans. Mycologia 2002; 94:260 - 6; http://dx.doi.org/10.2307/3761802; PMID: 21156495
- Peyroche A, Antonny B, Robineau S, Acker J, Cherfils J, Jackson CL. Brefeldin A acts to stabilize an abortive ARF-GDP-Sec7 domain protein complex: involvement of specific residues of the Sec7 domain. Mol Cell 1999; 3:275 - 85; http://dx.doi.org/10.1016/S1097-2765(00)80455-4; PMID: 10198630
- Levine TP, Munro S. Targeting of Golgi-specific pleckstrin homology domains involves both PtdIns 4-kinase-dependent and -independent components. Curr Biol 2002; 12:695 - 704; http://dx.doi.org/10.1016/S0960-9822(02)00779-0; PMID: 12007412
- Wooding S, Pelham HR. The dynamics of golgi protein traffic visualized in living yeast cells. Mol Biol Cell 1998; 9:2667 - 80; PMID: 9725919
- Losev E, Reinke CA, Jellen J, Strongin DE, Bevis BJ, Glick BS. Golgi maturation visualized in living yeast. Nature 2006; 441:1002 - 6; http://dx.doi.org/10.1038/nature04717; PMID: 16699524
- Matsuura-Tokita K, Takeuchi M, Ichihara A, Mikuriya K, Nakano A. Live imaging of yeast Golgi cisternal maturation. Nature 2006; 441:1007 - 10; http://dx.doi.org/10.1038/nature04737; PMID: 16699523
- Glick BS, Nakano A. Membrane traffic within the Golgi apparatus. Annu Rev Cell Dev Biol 2009; 25:113 - 32; http://dx.doi.org/10.1146/annurev.cellbio.24.110707.175421; PMID: 19575639
- Jackson CL. Mechanisms of transport through the Golgi complex. J Cell Sci 2009; 122:443 - 52; http://dx.doi.org/10.1242/jcs.032581; PMID: 19193869
- Luini A. A brief history of the cisternal progression-maturation model. Cell Logist 2011; 1:6 - 11; http://dx.doi.org/10.4161/cl.1.1.14693; PMID: 21686099
- Pantazopoulou A, Peñalva MA. Characterization of Aspergillus nidulans RabC/Rab6. Traffic 2011; 12:386 - 406; http://dx.doi.org/10.1111/j.1600-0854.2011.01164.x; PMID: 21226815
- Graham TR, Scott PA, Emr SD. Brefeldin A reversibly blocks early but not late protein transport steps in the yeast secretory pathway. EMBO J 1993; 12:869 - 77; PMID: 8458343
- Sciaky N, Presley J, Smith C, Zaal KJ, Cole N, Moreira JE, et al. Golgi tubule traffic and the effects of brefeldin A visualized in living cells. J Cell Biol 1997; 139:1137 - 55; http://dx.doi.org/10.1083/jcb.139.5.1137; PMID: 9382862
- Jackson-Hayes L, Hill TW, Loprete DM, Fay LM, Gordon BS, Nkashama SA, et al. Two GDP-mannose transporters contribute to hyphal form and cell wall integrity in Aspergillus nidulans. Microbiology 2008; 154:2037 - 47; http://dx.doi.org/10.1099/mic.0.2008/017483-0; PMID: 18599832
- Yang JS, Valente C, Polishchuk RS, Turacchio G, Layre E, Moody DB, et al. COPI acts in both vesicular and tubular transport. Nat Cell Biol 2011; 13:996 - 1003; http://dx.doi.org/10.1038/ncb2273; PMID: 21725317
- Emr S, Glick BS, Linstedt AD, Lippincott-Schwartz J, Luini A, Malhotra V, et al. Journeys through the Golgi--taking stock in a new era. J Cell Biol 2009; 187:449 - 53; http://dx.doi.org/10.1083/jcb.200909011; PMID: 19948493
- Mogelsvang S, Gomez-Ospina N, Soderholm J, Glick BS, Staehelin LA. Tomographic evidence for continuous turnover of Golgi cisternae in Pichia pastoris. Mol Biol Cell 2003; 14:2277 - 91; http://dx.doi.org/10.1091/mbc.E02-10-0697; PMID: 12808029
- Bevis BJ, Hammond AT, Reinke CA, Glick BS. De novo formation of transitional ER sites and Golgi structures in Pichia pastoris. Nat Cell Biol 2002; 4:750 - 6; http://dx.doi.org/10.1038/ncb852; PMID: 12360285
- Rossanese OW, Soderholm J, Bevis BJ, Sears IB, O’Connor J, Williamson EK, et al. Golgi structure correlates with transitional endoplasmic reticulum organization in Pichia pastoris and Saccharomyces cerevisiae. J Cell Biol 1999; 145:69 - 81; http://dx.doi.org/10.1083/jcb.145.1.69; PMID: 10189369
- Siniossoglou S, Pelham HR. An effector of Ypt6p binds the SNARE Tlg1p and mediates selective fusion of vesicles with late Golgi membranes. EMBO J 2001; 20:5991 - 8; http://dx.doi.org/10.1093/emboj/20.21.5991; PMID: 11689439
- Siniossoglou S, Peak-Chew SY, Pelham HR. Ric1p and Rgp1p form a complex that catalyses nucleotide exchange on Ypt6p. EMBO J 2000; 19:4885 - 94; http://dx.doi.org/10.1093/emboj/19.18.4885; PMID: 10990452
- Sengupta D, Linstedt AD. Control of organelle size: the Golgi complex. Annu Rev Cell Dev Biol 2011; 27:57 - 77; http://dx.doi.org/10.1146/annurev-cellbio-100109-104003; PMID: 21639798
- Grigoriev I, Splinter D, Keijzer N, Wulf PS, Demmers J, Ohtsuka T, et al. Rab6 regulates transport and targeting of exocytotic carriers. Dev Cell 2007; 13:305 - 14; http://dx.doi.org/10.1016/j.devcel.2007.06.010; PMID: 17681140
- Araujo-Baz´n L, Peñalva MA, Espeso EA. Preferential localization of the endocytic internalization machinery to hyphal tips underlies polarization of the actin cytoskeleton in Aspergillus nidulans. Mol Microbiol 2008; 67:891 - 905; http://dx.doi.org/10.1111/j.1365-2958.2007.06102.x; PMID: 18179595
- Upadhyay S, Shaw BD. The role of actin, fimbrin and endocytosis in growth of hyphae in Aspergillus nidulans. Mol Microbiol 2008; 68:690 - 705; http://dx.doi.org/10.1111/j.1365-2958.2008.06178.x; PMID: 18331474
- Lee SC, Schmidtke SN, Dangott LJ, Shaw BD. Aspergillus nidulans ArfB plays a role in endocytosis and polarized growth. Eukaryot Cell 2008; 7:1278 - 88; http://dx.doi.org/10.1128/EC.00039-08; PMID: 18539885
- Kaksonen M, Sun Y, Drubin DG. A pathway for association of receptors, adaptors, and actin during endocytic internalization. Cell 2003; 115:475 - 87; http://dx.doi.org/10.1016/S0092-8674(03)00883-3; PMID: 14622601
- Herv´s-Aguilar A, Peñalva MA. Endocytic machinery protein SlaB is dispensable for polarity establishment but necessary for polarity maintenance in hyphal tip cells of Aspergillus nidulans. Eukaryot Cell 2010; 9:1504 - 18; http://dx.doi.org/10.1128/EC.00119-10; PMID: 20693304
- Valdez-Taubas J, Pelham HR. Slow diffusion of proteins in the yeast plasma membrane allows polarity to be maintained by endocytic cycling. Curr Biol 2003; 13:1636 - 40; http://dx.doi.org/10.1016/j.cub.2003.09.001; PMID: 13678596
- Virag A, Lee MP, Si H, Harris SD. Regulation of hyphal morphogenesis by cdc42 and rac1 homologues in Aspergillus nidulans. Mol Microbiol 2007; 66:1579 - 96; PMID: 18005099
- Schuster M, Lipowsky R, Assmann MA, Lenz P, Steinberg G. Transient binding of dynein controls bidirectional long-range motility of early endosomes. Proc Natl Acad Sci U S A 2011; 108:3618 - 23; http://dx.doi.org/10.1073/pnas.1015839108; PMID: 21317367
- Lenz JH, Schuchardt I, Straube A, Steinberg G. A dynein loading zone for retrograde endosome motility at microtubule plus-ends. EMBO J 2006; 25:2275 - 86; http://dx.doi.org/10.1038/sj.emboj.7601119; PMID: 16688221
- Wedlich-Söldner R, Straube A, Friedrich MW, Steinberg G. A balance of KIF1A-like kinesin and dynein organizes early endosomes in the fungus Ustilago maydis. EMBO J 2002; 21:2946 - 57; http://dx.doi.org/10.1093/emboj/cdf296; PMID: 12065408
- Zerial M, McBride H. Rab proteins as membrane organizers. Nat Rev Mol Cell Biol 2001; 2:107 - 17; http://dx.doi.org/10.1038/35052055; PMID: 11252952
- Segev N. Coordination of intracellular transport steps by GTPases. Semin Cell Dev Biol 2011; 22:33 - 8; http://dx.doi.org/10.1016/j.semcdb.2010.11.005; PMID: 21130177
- Pfeffer S, Aivazian D. Targeting Rab GTPases to distinct membrane compartments. Nat Rev Mol Cell Biol 2004; 5:886 - 96; http://dx.doi.org/10.1038/nrm1500; PMID: 15520808
- Abenza JF, Galindo A, Pantazopoulou A, Gil C, de los Ri´os V, Peñalva MA. Aspergillus RabB Rab5 integrates acquisition of degradative identity with the long distance movement of early endosomes. Mol Biol Cell 2010; 21:2756 - 69; http://dx.doi.org/10.1091/mbc.E10-02-0119; PMID: 20534811
- Zekert N, Fischer R. The Aspergillus nidulans kinesin-3 UncA motor moves vesicles along a subpopulation of microtubules. Mol Biol Cell 2009; 20:673 - 84; http://dx.doi.org/10.1091/mbc.E08-07-0685; PMID: 19037104
- Zhang J, Yao X, Fischer L, Abenza JF, Peñalva MA, Xiang X. The p25 subunit of the dynactin complex is required for dynein-early endosome interaction. J Cell Biol 2011; 193:1245 - 55; http://dx.doi.org/10.1083/jcb.201011022; PMID: 21708978
- Zhang J, Zhuang L, Lee Y, Abenza JF, Peñalva MA, Xiang X. The microtubule plus-end localization of Aspergillus dynein is important for dynein-early-endosome interaction but not for dynein ATPase activation. J Cell Sci 2010; 123:3596 - 604; http://dx.doi.org/10.1242/jcs.075259; PMID: 20876661
- Konzack S, Rischitor PE, Enke C, Fischer R. The role of the kinesin motor KipA in microtubule organization and polarized growth of Aspergillus nidulans. Mol Biol Cell 2005; 16:497 - 506; http://dx.doi.org/10.1091/mbc.E04-02-0083; PMID: 15563609
- Zhang J, Li S, Fischer R, Xiang X. Accumulation of cytoplasmic dynein and dynactin at microtubule plus ends in Aspergillus nidulans is kinesin dependent. Mol Biol Cell 2003; 14:1479 - 88; http://dx.doi.org/10.1091/mbc.E02-08-0516; PMID: 12686603
- Kardon JR, Vale RD. Regulators of the cytoplasmic dynein motor. Nat Rev Mol Cell Biol 2009; 10:854 - 65; http://dx.doi.org/10.1038/nrm2804; PMID: 19935668
- Rink J, Ghigo E, Kalaidzidis Y, Zerial M. Rab conversion as a mechanism of progression from early to late endosomes. Cell 2005; 122:735 - 49; http://dx.doi.org/10.1016/j.cell.2005.06.043; PMID: 16143105
- Markgraf DF, Ahnert F, Arlt H, Mari M, Peplowska K, Epp N, et al. The CORVET subunit Vps8 cooperates with the Rab5 homolog Vps21 to induce clustering of late endosomal compartments. Mol Biol Cell 2009; 20:5276 - 89; http://dx.doi.org/10.1091/mbc.E09-06-0521; PMID: 19828734
- Peplowska K, Markgraf DF, Ostrowicz CW, Bange G, Ungermann C. The CORVET tethering complex interacts with the yeast Rab5 homolog Vps21 and is involved in endo-lysosomal biogenesis. Dev Cell 2007; 12:739 - 50; http://dx.doi.org/10.1016/j.devcel.2007.03.006; PMID: 17488625
- Poteryaev D, Datta S, Ackema K, Zerial M, Spang A. Identification of the switch in early-to-late endosome transition. Cell 2010; 141:497 - 508; http://dx.doi.org/10.1016/j.cell.2010.03.011; PMID: 20434987
- Nordmann M, Cabrera M, Perz A, Bröcker C, Ostrowicz C, Engelbrecht-Vandre´ S, et al. The Mon1-Ccz1 complex is the GEF of the late endosomal Rab7 homolog Ypt7. Curr Biol 2010; 20:1654 - 9; http://dx.doi.org/10.1016/j.cub.2010.08.002; PMID: 20797862
- Seals DF, Eitzen G, Margolis N, Wickner WT, Price AA. A Ypt/Rab effector complex containing the Sec1 homolog Vps33p is required for homotypic vacuole fusion. Proc Natl Acad Sci U S A 2000; 97:9402 - 7; http://dx.doi.org/10.1073/pnas.97.17.9402; PMID: 10944212
- Peñalva MA. Tracing the endocytic pathway of Aspergillus nidulans with FM4-64. Fungal Genet Biol 2005; 42:963 - 75; http://dx.doi.org/10.1016/j.fgb.2005.09.004; PMID: 16291501
- Herv´s-Aguilar A, Rodri´guez-Galán O, Galindo A, Abenza JF, Arst HN Jr., Peñalva MA. Characterization of Aspergillus nidulans DidB Did2, a non-essential component of the multivesicular body pathway. Fungal Genet Biol 2010; 47:636 - 46; http://dx.doi.org/10.1016/j.fgb.2010.03.010; PMID: 20362686
- Galindo A, Herv´s-Aguilar A, Rodri´guez-Galán O, Vincent O, Arst HN Jr., Tilburn J, et al. PalC, one of two Bro1 domain proteins in the fungal pH signalling pathway, localizes to cortical structures and binds Vps32. Traffic 2007; 8:1346 - 64; http://dx.doi.org/10.1111/j.1600-0854.2007.00620.x; PMID: 17696968
- Calcagno-Pizarelli AM, Herv´s-Aguilar A, Galindo A, Abenza JF, Peñalva MA, Arst HN Jr. Rescue of Aspergillus nidulans severely debilitating null mutations in ESCRT-0, I, II and III genes by inactivation of a salt-tolerance pathway allows examination of ESCRT gene roles in pH signalling. J Cell Sci 2011; 124:4064 - 76; http://dx.doi.org/10.1242/jcs.088344; PMID: 22135362
- Findon H, Calcagno-Pizarelli AM, Marti´nez JL, Spielvogel A, Markina-Iñarrairaegui A, Indrakumar T, et al. Analysis of a novel calcium auxotrophy in Aspergillus nidulans. Fungal Genet Biol 2010; 47:647 - 55; http://dx.doi.org/10.1016/j.fgb.2010.04.002; PMID: 20438880
- Spielvogel A, Findon H, Arst HN Jr., Araújo-Baz´n L, Hern´ndez-Orti´z P, Stahl U, et al. Two zinc finger transcription factors, CrzA and SltA, are involved in cation homoeostasis and detoxification in Aspergillus nidulans. Biochem J 2008; 414:419 - 29; http://dx.doi.org/10.1042/BJ20080344; PMID: 18471095
- Hay JC. Calcium: a fundamental regulator of intracellular membrane fusion?. EMBO Rep 2007; 8:236 - 40; http://dx.doi.org/10.1038/sj.embor.7400921; PMID: 17330068
- Fern´ndez-Cañón JM, Granadino B, Beltrán-Valero de Bernabe´ D, Renedo M, Fern´ndez-Ruiz E, Peñalva MA, et al. The molecular basis of alkaptonuria. Nat Genet 1996; 14:19 - 24; http://dx.doi.org/10.1038/ng0996-19; PMID: 8782815
- Gallardo ME, Desviat LR, Rodri´guez JM, Esparza-Gordillo J, Pe´rez-Cerdá C, Pe´rez B, et al. The molecular basis of 3-methylcrotonylglycinuria, a disorder of leucine catabolism. Am J Hum Genet 2001; 68:334 - 46; http://dx.doi.org/10.1086/318202; PMID: 11170888
- Peñalva MA. A fungal perspective on human inborn errors of metabolism: alkaptonuria and beyond. Fungal Genet Biol 2001; 34:1 - 10; http://dx.doi.org/10.1006/fgbi.2001.1284; PMID: 11567547
- Adams TH, Wieser JK, Yu JH. Asexual sporulation in Aspergillus nidulans. Microbiol Mol Biol Rev 1998; 62:35 - 54; PMID: 9529886