Abstract
Daily phagocytosis of outer segments (OS) places extraordinary demands on protein biosynthesis and trafficking in photoreceptor neurons. While the members and roles of the phototransduction pathway in the OS are well characterized, details about protein trafficking are just beginning to emerge. Phosphodiesterase6 (PDE6), the effector enzyme in phototransduction cascade, serves as an example of the steps multimeric proteins must pass through to achieve their functional state in the OS. Genetic model systems have recently provided snapshots of various steps in the pathway, as experimental difficulties such as an inability to maintain ciliated photoreceptor outer segments or express functional PDE6 holoenzyme in vitro necessitate in vivo studies. We will highlight the significant findings, their implications to blinding diseases, as well as discuss the gaps requiring further investigation.
Rod and cone photoreceptor cells of the retina are specialized neurons dedicated to the task of visual perception. They are polarized cells with three distinct regions. The outer segment (OS), a modified cilia specialized for photon absorption, the inner segment (IS), the location of protein biosynthesis, and the synaptic region, responsible for transmitting visual information to downstream neurons (). In the OS, a highly regulated signal transduction pathway converts light into an electrical signal and has been extensively reviewed elsewhere.Citation1,Citation2 In brief, the G-protein coupled receptor rhodopsin responds to light and activates transducin, the heterotrimeric G-protein in the visual system. Activated transducin relieves the inhibitory constraint on the effector enzyme phosphodiesterase6 (PDE6) to increase cyclic GMP (cGMP) hydrolysis. Lower levels of cGMP cause cyclic nucleotide gated channels to close initiating membrane hyperpolarization, resulting in synaptic transmission. Interestingly, all the proteins involved in phototransduction are synthesized in the IS and function within the confines, both integrally and peripherally, of the flattened membranous discs within the OS. To complicate this process, about 10% of the discs including the resident proteins are phagocytized by the adjacent retinal pigmented epithelium (RPE) every day.Citation3,Citation4 Biosynthetic demands on photoreceptor cells require rapid and efficient mechanisms to transport proteins synthesized in the IS along the narrow connecting cilia to replenish the OS. While our understanding of the phototransduction cascade and its regulation has progressed tremendously, gaps remain in our knowledge about phototransduction protein synthesis, assembly and trafficking to OS. Defects in any one of these steps can lead to blindness in humans illustrating the significance of this process.Citation5-Citation7
Figure 1. Model for biosynthesis and transport of PDE6 in rods. (A) Polarized rod photoreceptor cells have three distinct compartments. OS, outer segment; IS, inner segment and synaptic terminal; RER, rough endoplasmic reticulum; V, vesicle; CC, connecting cilia; RPE, retinal pigment epithelium. (B) Model depicting biosynthesis and trafficking of rod PDE6. (I) Folding of PDE6αβ subunits by AIPL1 and addition of the prenyl group by the prenyltransferase (PPTase) occurs in the cytosol before association with the ER (endoplasmic reticulum). (II) The last three amino acids (-AAX) are cleaved by the protease RAS-converting enzyme 1 (RCE1). (III) Isoprenylcysteine methyltransferase (ICMT) catalyzes the addition of a methyl group to the newly exposed prenylcysteine. Adenosyl methionine (AdoMet) serves as the methyl donor for the reaction. Assembly of the holoenzyme takes place in the inner segment either at the surface of the ER or after ER exit. We propose direct transport of PDE6 from ER to basal body, bypassing the Golgi as no Golgi-mediated modifications are known to occur to PDE6. Alternatively, PDE6 vesicles may fuse with the plasma membrane of the IS before transport through the CC (dashed arrow). Transport to the OS likely occurs by IFT, however mechanism is unknown. In the absence of RCE1, the final two steps of CAAX processing do not proceed (left side of dashed line). However, PDE6 holoenzyme assembles and is functional. Defective transport leads to accumulation of PDE6 containing vesicles in IS. AdoHcy, adenosyl homocysteine.
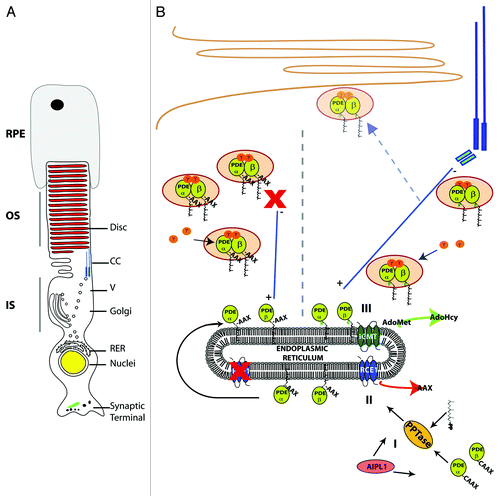
For simplicity, we broadly divide biosynthesis of proteins in the IS into three distinct phases: (1) initial synthesis, posttranslational modification and assembly of multimeric proteins, (2) targeting to the base of cilium and (3) transport and incorporation into photoreceptor disc membranes. Rhodopsin was the first phototransduction protein to be observed undergoing vesicular transport in vesicles termed rhodopsin transport carriers (RTC).Citation8,Citation9 Targeting sequences on the c-terminal tail of rhodopsin directs RTC’s from the Golgi to the base of the connecting cilium.Citation10,Citation11 The mechanism by which rhodopsin is transported from the basal body through the connecting cilia to the OS involves intraflagellar transport (IFT) machinery, however the exact details are still being elucidated.Citation12 Transducin trafficking is highly regulated as Tα and Tβγ subunits exit the OS under bright light and return in the dark as previously reviewed.Citation13,Citation14 Translocation in response to light is thought to occur by diffusion. In contrast, return of transducin subunits in the dark and assembly status of the heterotrimer is still not clear. Recent studies suggest diffusion as a possible mechanism behind this movement as well, but vesicular transport based mechanism cannot be ruled out.Citation15 In contrast to our knowledge of opsin and transducin trafficking, very little is known about transport of PDE6 to the OS. Recent studies based on novel animal models are shedding light on biosynthesis, assembly and transport of this crucial phototransduction protein and are the focus of this review.
In rod photoreceptor cells, PDE6 is composed of two catalytic subunits, PDE6α and PDE6β, and two inhibitory PDE6γ subunits.Citation16 Both catalytic subunits of PDE6 are lipidated by post-translational prenylation.Citation17 Prenylation involves covalent attachment of either a C15 farnesyl or C20 geranylgeranyl isoprenyl group to the C-terminal cysteine of proteins ending in a “CAAX” box (, Step I).Citation18 The “CAAX” box consists of a cysteine followed by two aliphatic (A) amino acids and the X amino acid designates the particular prenyl group to be attached.Citation19 Following addition of the prenyl groups in the cytosol, PDE6 is targeted to the endoplasmic reticulum (ER) for proteolysis of the last three amino acids (-AAX) by the protease, RAS-converting enzyme 1 (RCE1).Citation20 Subsequently, the newly prenylated cysteine group is methyl esterified by isoprenyl cysteine methyl transferase (ICMT).Citation21 Interestingly, among multimeric proteins, PDE6 is the only known holoenzyme, where one subunit is farnesylated and another is geranylgeranylated; however the contributions of differential prenylation to PDE6 function are not known.Citation22 We believe lipid modifications of PDE6 play a crucial role in its transport as well as retention in the OS disc membranes.Citation23 Additionally, differential lipid modification of PDE6 catalytic subunits may play a role in proper orientation of PDE6 with membranes aiding in efficient coupling to transducin for phototransduction (Singh R. and Ramamurthy V., unpublished studies).
Defects in PDE6 Lipidation and Retinal Degenerative Diseases
A patient mutation in the final amino acid of the PDE6β subunit provides clinical evidence of the importance of differential PDE6 lipid modifications.Citation24 Based on studies of prenyltransferase “CAAX” box specificity, the mutation is predicted to alter the prenyl group from a geranylgeranyl to a farnesyl group.Citation25 The patient was diagnosed with retinitis pigmentosa at age 12, had difficulty seeing at night, and no loss of visual acuity or color vision. These symptoms suggest PDE6 requires differential prenylation for function and/or stability. Animal models replicating a similar mutation in PDE6β show progressive rod cell degeneration and dysfunction (Singh R. and Ramamurthy V., unpublished studies).
Lipidation and Assembly of PDE6
Our interest in prenylation is due to our earlier work investigating the mechanism of a severe childhood blindness called Leber congenital amaurosis (LCA) caused by mutations in aryl-hydrocarbon interacting protein-like 1 (AIPL1).Citation6,Citation26 Yeast two hybrid (Y2H) analysis showed AIPL1 interacts with prenylated proteins.Citation27 In agreement with our Y2H results, mouse knockouts of AIPL1 affected the assembly, stability and function of prenylated phosphodiesterase 6 (PDE6).Citation6,Citation28 Further biochemical studies show that AIPL1 interacts with farnesylated PDE6α subunit.Citation29 Altogether, these studies suggest a model where AIPL1 acts as a chaperone for farnesylated PDE6α and promotes folding and proper assembly with its partner subunits. Absence or defects in AIPL1 affect the initial phase of biosynthesis of PDE6 leading to its degradation and subsequent loss in visual response.
Another chaperone involved in PDE6 membrane interaction is prenyl binding protein/δ (PrBP/δ) formerly known as PDE6/δ.Citation30 PrBP/δ interacts with farnesylated proteins by binding to the farnesylated/methylated cysteine and the proximal upstream amino acid residues.Citation31 PrBP/δ extracts PDE6 from rod OS membrane preparations and is thought to be important for PDE6 transport to the OS in cones.Citation32,Citation33
Postprenylation Processing and PDE6 Trafficking
The initial lipidation is thought to be the primary determinant for membrane attachment of PDE6.Citation34 The role of subsequent “CAAX” processing events in assembly, transport and function was recently elucidated from our studies in an animal model lacking RCE1 in retinal neurons. Conditional knockout of RCE1 eliminated both proteolysis and subsequent methylation of prenylated proteins including PDE6 at embryonic day 9.5 in the retina and forebrain.Citation23 Rods and cones formed normally but started to degenerate as the ciliated OS elaborated. Timing of degeneration corresponded to the requirement for efficient protein trafficking from IS to OS. Another key finding from conditional Rce1 animal model was that PDE6 requires RCE1-mediated proteolysis for transport to the OS while the other farnesylated proteins, transducin γ subunit and rhodopsin kinase are transported normally.Citation23 Additionally, lack of postprenylation processing did not affect the assembly or function of PDE6. Mislocalized PDE6 appeared as discrete vesicular structures at the distal end of the inner segment below the connecting cilia. Our preliminary ultrastructural studies confirm the presence of these vesicular structures in retina lacking Rce1. Animal models that exhibit impaired protein trafficking to the OS accumulate excess protein at photoreceptor synapses. For example, rod PDE6 is localized in the IS and synaptic region in addition to the OS in prenyl binding protein/δ (PrBP/δ) knockout mice.Citation33 In contrast, PDE6 was exclusively present in IS in retina lacking Rce1. Based on our findings, we propose the following models to explain the requirement for complete “CAAX” processing of PDE6 for proper trafficking to OS.
Model 1
PDE6 catalytic subunits exit the ER and form the holoenzyme on vesicles in the cytoplasm. Vesicular PDE6 trafficking has a unique pathway, that utilizes small GTPases or adaptor protein (akin to Rho GDI) that also require “CAAX” processing. In the absence of RCE1, the vesicles are not able to dock and release from the ciliary transport machinery for further transport to OS ().
Model 2
Assembly of the PDE6 holoenzyme occurs at the ER membrane and a putative prenyl binding protein specifically interacts with the methyl esterified isoprenyl cysteine group of PDE6 subunits. This prenyl binding protein is required for PDE6 extraction from ER membranes and further transport to OS (). We do not believe this prenyl binding protein to be PrBP/δ, because the majority of rod PDE6 is transported to the OS in the absence of PrBP/δ.Citation33
Model 3
PDE6 has a large phospholipid binding footprint with contributions from the prenyl groups, further methyl esterification and polybasic regions similar to Ras proteins.Citation35 In the absence of RCE1-mediated proteolysis, the presence of “-AAX” on PDE6 subunits creates steric hindrance impeding the ability of PDE6 to interact with transport vesicles. Investigating the composition and identity of proteins present in vesicular structures seen by light and electron microscopy will be crucial in differentiating these possibilities.
Unanswered Questions in Biosynthesis and Transport of PDE6
(1) The immediate question to be answered is whether the cause of rapid degeneration in Rce1 conditional knockout is strictly a PDE6 defect or a secondary defect such as a lack of processing of a small GTPase responsible for PDE6 vesicle docking. To answer this question, localization of PDE6 mutants with altered CAAX box similar to the one found in phosphorylase kinase unable to undergo proteolysis by RCE1 will need to be examined in retinal neurons expressing wild-type levels of RCE1.Citation36 This approach will also allow us to determine if the defects are specific to PDE6α, PDE6β or both. (2) If the defect is PDE6 specific, it is imperative to know if lack of proteolysis or methylation results in accumulation of PDE6 in the IS. We are at present pursuing a retina specific conditional knockout of Icmt, the methyl transferase. If PDE6 is not transported in the absence of ICMT, it would make a strong case for a quality control mechanism permitting only fully processed and methylated PDE6 to be transported to OS. (3) Cone PDE6 is a homodimer composed of two α’-subunits that are thought to be geranylgeranylated.Citation37 Unfortunately, we were not able to unequivocally determine the localization of cone PDE6 due to rapid degeneration of cone cells in the absence of RCE1. A cone-specific knockout of Rce1 will answer the importance of postprenylation processing of PDE6 in cones. (4) Differential prenylation of rod PDE6 may play a role in PDE6 specific transport by interacting with unique protein partners for each lipid group. Studies are underway to alter the lipid groups on individual subunits and test their ability to be transported to the OS.
Summary
It is important to note that several crucial proteins in the rod phototransduction cascade are farnesylated and undergo “CAAX” processing, including rhodopsin kinase (GRK1), transducin γ and PDE6.Citation17,Citation38,Citation39 We believe prenylation is critical for their interactions with other proteins and lipids, but rapid retinal degeneration inhibited our ability to investigate the effects of lack of “CAAX” processing on individual proteins. Previous studies suggest that PDE6 is transported to OS on vesicles also carrying guanylyl cyclase (GC).Citation40 However, transport of GC was unaffected in the absence of Rce1. Furthermore; absence of RD3 a protein important for stability of GC did not exhibit any defect in PDE6 transport.Citation41 This finding coupled with presence of discrete vesicular structures in our animal models suggest that there is a unique pathway for PDE6 trafficking in rod cells. We are in the early stages of deciphering how multimeric proteins such as PDE6 are assembled, targeted to transport vesicles, trafficked and integrated into photoreceptor discs in the OS. Now that there is in vivo evidence that “CAAX” processing is not required for assembly of PDE6 we can focus on resolving the transport mechanisms requiring proteolysis and or methylation of PDE6.
Acknowledgments
We thank our collaborator Drs Stephen Young and Martin Bergo for animal models, reagents and advice. This work was supported by National Institutes of Health grant RO1EY017035 (V.R.), West Virginia Lions, Lions Club International Foundation, and Research to Prevent Blindness (RPB) challenge grant to West Virginia University.
References
- Lamb TD, Pugh EN Jr. Dark adaptation and the retinoid cycle of vision. Prog Retin Eye Res 2004; 23:307 - 80; http://dx.doi.org/10.1016/j.preteyeres.2004.03.001; PMID: 15177205
- Burns ME, Arshavsky VY. Beyond counting photons: trials and trends in vertebrate visual transduction. Neuron 2005; 48:387 - 401; http://dx.doi.org/10.1016/j.neuron.2005.10.014; PMID: 16269358
- Besharse JC, Hollyfield JG. Turnover of mouse photoreceptor outer segments in constant light and darkness. Invest Ophthalmol Vis Sci 1979; 18:1019 - 24; PMID: 478775
- LaVail MM. Rod outer segment disk shedding in rat retina: relationship to cyclic lighting. Science 1976; 194:1071 - 4; http://dx.doi.org/10.1126/science.982063; PMID: 982063
- Liu X, Garriga P, Khorana HG. Structure and function in rhodopsin: correct folding and misfolding in two point mutants in the intradiscal domain of rhodopsin identified in retinitis pigmentosa. Proc Natl Acad Sci U S A 1996; 93:4554 - 9; http://dx.doi.org/10.1073/pnas.93.10.4554; PMID: 8643442
- Ramamurthy V, Niemi GA, Reh TA, Hurley JB. Leber congenital amaurosis linked to AIPL1: a mouse model reveals destabilization of cGMP phosphodiesterase. Proc Natl Acad Sci U S A 2004; 101:13897 - 902; http://dx.doi.org/10.1073/pnas.0404197101; PMID: 15365178
- Yamamoto S, Sippel KC, Berson EL, Dryja TP. Defects in the rhodopsin kinase gene in the Oguchi form of stationary night blindness. Nat Genet 1997; 15:175 - 8; http://dx.doi.org/10.1038/ng0297-175; PMID: 9020843
- Papermaster DS, Schneider BG, Besharse JC. Vesicular transport of newly synthesized opsin from the Golgi apparatus toward the rod outer segment. Ultrastructural immunocytochemical and autoradiographic evidence in Xenopus retinas. Invest Ophthalmol Vis Sci 1985; 26:1386 - 404; PMID: 2931395
- Deretic D, Traverso V, Parkins N, Jackson F, Rodriguez de Turco EB, Ransom N. Phosphoinositides, ezrin/moesin, and rac1 regulate fusion of rhodopsin transport carriers in retinal photoreceptors. Mol Biol Cell 2004; 15:359 - 70; http://dx.doi.org/10.1091/mbc.E03-04-0203; PMID: 13679519
- Mazelova J, Astuto-Gribble L, Inoue H, Tam BM, Schonteich E, Prekeris R, et al. Ciliary targeting motif VxPx directs assembly of a trafficking module through Arf4. EMBO J 2009; 28:183 - 92; http://dx.doi.org/10.1038/emboj.2008.267; PMID: 19153612
- Deretic D, Williams AH, Ransom N, Morel V, Hargrave PA, Arendt A. Rhodopsin C terminus, the site of mutations causing retinal disease, regulates trafficking by binding to ADP-ribosylation factor 4 (ARF4). Proc Natl Acad Sci U S A 2005; 102:3301 - 6; http://dx.doi.org/10.1073/pnas.0500095102; PMID: 15728366
- Sung CH, Chuang JZ. The cell biology of vision. J Cell Biol 2010; 190:953 - 63; http://dx.doi.org/10.1083/jcb.201006020; PMID: 20855501
- Calvert PD, Strissel KJ, Schiesser WE, Pugh EN Jr., Arshavsky VY. Light-driven translocation of signaling proteins in vertebrate photoreceptors. Trends Cell Biol 2006; 16:560 - 8; http://dx.doi.org/10.1016/j.tcb.2006.09.001; PMID: 16996267
- Arshavsky VY, Burns ME. Photoreceptor signaling: supporting vision across a wide range of light intensities. J Biol Chem 2012; 287:1620 - 6; http://dx.doi.org/10.1074/jbc.R111.305243; PMID: 22074925
- Zhang H, Constantine R, Vorobiev S, Chen Y, Seetharaman J, Huang YJ, et al. UNC119 is required for G protein trafficking in sensory neurons. Nat Neurosci 2011; 14:874 - 80; http://dx.doi.org/10.1038/nn.2835; PMID: 21642972
- Baehr W, Devlin MJ, Applebury ML. Isolation and characterization of cGMP phosphodiesterase from bovine rod outer segments. J Biol Chem 1979; 254:11669 - 77; PMID: 227876
- Anant JS, Ong OC, Xie HY, Clarke S, O’Brien PJ, Fung BK. In vivo differential prenylation of retinal cyclic GMP phosphodiesterase catalytic subunits. J Biol Chem 1992; 267:687 - 90; PMID: 1309771
- Glomset JA, Gelb MH, Farnsworth CC. Prenyl proteins in eukaryotic cells: a new type of membrane anchor. Trends Biochem Sci 1990; 15:139 - 42; http://dx.doi.org/10.1016/0968-0004(90)90213-U; PMID: 2187294
- Zhang FL, Casey PJ. Protein prenylation: molecular mechanisms and functional consequences. Annu Rev Biochem 1996; 65:241 - 69; http://dx.doi.org/10.1146/annurev.bi.65.070196.001325; PMID: 8811180
- Wright LP, Philips MR. Thematic review series: lipid posttranslational modifications. CAAX modification and membrane targeting of Ras. J Lipid Res 2006; 47:883 - 91; http://dx.doi.org/10.1194/jlr.R600004-JLR200; PMID: 16543601
- Hrycyna CA, Clarke S. Modification of eukaryotic signaling proteins by C-terminal methylation reactions. Pharmacol Ther 1993; 59:281 - 300; http://dx.doi.org/10.1016/0163-7258(93)90071-K; PMID: 8309992
- Ong OC, Ota IM, Clarke S, Fung BK. The membrane binding domain of rod cGMP phosphodiesterase is posttranslationally modified by methyl esterification at a C-terminal cysteine. Proc Natl Acad Sci U S A 1989; 86:9238 - 42; http://dx.doi.org/10.1073/pnas.86.23.9238; PMID: 2556707
- Christiansen JR, Kolandaivelu S, Bergo MO, Ramamurthy V. RAS-converting enzyme 1-mediated endoproteolysis is required for trafficking of rod phosphodiesterase 6 to photoreceptor outer segments. Proc Natl Acad Sci U S A 2011; 108:8862 - 6; http://dx.doi.org/10.1073/pnas.1103627108; PMID: 21555557
- Veske A, Orth U, Ruether K, Zrenner E, Rosenberg T, Baehr W, et al. Mutations in the gene for the β-subunit of photoreceptor-specific cGMP phosphodiesterase (PDEβ) in patients with retinal dystrophies and dysfunctions. In: Anderson, Hollyfield, LaVail, eds. Degenerative Diseases of the Retina. New York: Plenum Press, 1995:313-22.
- Trueblood CE, Boyartchuk VL, Picologlou EA, Rozema D, Poulter CD, Rine J. The CaaX proteases, Afc1p and Rce1p, have overlapping but distinct substrate specificities. Mol Cell Biol 2000; 20:4381 - 92; http://dx.doi.org/10.1128/MCB.20.12.4381-4392.2000; PMID: 10825201
- Sohocki MM, Bowne SJ, Sullivan LS, Blackshaw S, Cepko CL, Payne AM, et al. Mutations in a new photoreceptor-pineal gene on 17p cause Leber congenital amaurosis. Nat Genet 2000; 24:79 - 83; http://dx.doi.org/10.1038/71732; PMID: 10615133
- Ramamurthy V, Roberts M, van den Akker F, Niemi G, Reh TA, Hurley JB. AIPL1, a protein implicated in Leber’s congenital amaurosis, interacts with and aids in processing of farnesylated proteins. Proc Natl Acad Sci U S A 2003; 100:12630 - 5; http://dx.doi.org/10.1073/pnas.2134194100; PMID: 14555765
- Liu X, Bulgakov OV, Wen XH, Woodruff ML, Pawlyk B, Yang J, et al. AIPL1, the protein that is defective in Leber congenital amaurosis, is essential for the biosynthesis of retinal rod cGMP phosphodiesterase. Proc Natl Acad Sci U S A 2004; 101:13903 - 8; http://dx.doi.org/10.1073/pnas.0405160101; PMID: 15365173
- Kolandaivelu S, Huang J, Hurley JB, Ramamurthy V. AIPL1, a protein associated with childhood blindness, interacts with alpha-subunit of rod phosphodiesterase (PDE6) and is essential for its proper assembly. J Biol Chem 2009; 284:30853 - 61; http://dx.doi.org/10.1074/jbc.M109.036780; PMID: 19758987
- Gillespie PG, Prusti RK, Apel ED, Beavo JA. A soluble form of bovine rod photoreceptor phosphodiesterase has a novel 15-kDa subunit. J Biol Chem 1989; 264:12187 - 93; PMID: 2545702
- Cook TA, Ghomashchi F, Gelb MH, Florio SK, Beavo JA. Binding of the delta subunit to rod phosphodiesterase catalytic subunits requires methylated, prenylated C-termini of the catalytic subunits. Biochemistry 2000; 39:13516 - 23; http://dx.doi.org/10.1021/bi001070l; PMID: 11063588
- Zhang H, Hosier S, Terew JM, Zhang K, Cote RH, Baehr W. Assay and functional properties of PrBP(PDEdelta), a prenyl-binding protein interacting with multiple partners. Methods Enzymol 2005; 403:42 - 56; http://dx.doi.org/10.1016/S0076-6879(05)03005-3; PMID: 16473576
- Zhang H, Li S, Doan T, Rieke F, Detwiler PB, Frederick JM, et al. Deletion of PrBP/delta impedes transport of GRK1 and PDE6 catalytic subunits to photoreceptor outer segments. Proc Natl Acad Sci U S A 2007; 104:8857 - 62; http://dx.doi.org/10.1073/pnas.0701681104; PMID: 17496142
- Silvius JR, l’Heureux F. Fluorimetric evaluation of the affinities of isoprenylated peptides for lipid bilayers. Biochemistry 1994; 33:3014 - 22; http://dx.doi.org/10.1021/bi00176a034; PMID: 8130214
- Hessel E, Heck M, Müller P, Herrmann A, Hofmann KP. Signal transduction in the visual cascade involves specific lipid-protein interactions. J Biol Chem 2003; 278:22853 - 60; http://dx.doi.org/10.1074/jbc.M302747200; PMID: 12676942
- Heilmeyer LM Jr., Serwe M, Weber C, Metzger J, Hoffmann-Posorske E, Meyer HE. Farnesylcysteine, a constituent of the alpha and beta subunits of rabbit skeletal muscle phosphorylase kinase: localization by conversion to S-ethylcysteine and by tandem mass spectrometry. Proc Natl Acad Sci U S A 1992; 89:9554 - 8; http://dx.doi.org/10.1073/pnas.89.20.9554; PMID: 1409665
- Li TS, Volpp K, Applebury ML. Bovine cone photoreceptor cGMP phosphodiesterase structure deduced from a cDNA clone. Proc Natl Acad Sci U S A 1990; 87:293 - 7; http://dx.doi.org/10.1073/pnas.87.1.293; PMID: 2153291
- Fukada Y, Takao T, Ohguro H, Yoshizawa T, Akino T, Shimonishi Y. Farnesylated gamma-subunit of photoreceptor G protein indispensable for GTP-binding. Nature 1990; 346:658 - 60; http://dx.doi.org/10.1038/346658a0; PMID: 2385292
- Inglese J, Glickman JF, Lorenz W, Caron MG, Lefkowitz RJ. Isoprenylation of a protein kinase. Requirement of farnesylation/alpha-carboxyl methylation for full enzymatic activity of rhodopsin kinase. J Biol Chem 1992; 267:1422 - 5; PMID: 1730692
- Karan S, Zhang H, Li S, Frederick JM, Baehr W. A model for transport of membrane-associated phototransduction polypeptides in rod and cone photoreceptor inner segments. Vision Res 2008; 48:442 - 52; http://dx.doi.org/10.1016/j.visres.2007.08.020; PMID: 17949773
- Azadi S, Molday LL, Molday RS. RD3, the protein associated with Leber congenital amaurosis type 12, is required for guanylate cyclase trafficking in photoreceptor cells. Proc Natl Acad Sci U S A 2010; 107:21158 - 63; http://dx.doi.org/10.1073/pnas.1010460107; PMID: 21078983