Abstract
Epidermis forms a barrier between the dry external environment and the interior of the body, preventing water and ions from exiting, and toxins, antigens and bacteria from entering. Residing in the extracellular lipid membranes of the stratum corneum, this vital barrier requires an acidic environment in order to remain functional and competent against various insults. The acid pH not only limits the growth of pathogenic skin flora, but also is required for the enzymatic lipid processing that results in an effective permeability barrier. The mechanisms behind this "acid mantle" have long been debated. Originally, exogenous sources of acid were thought to cause this drop in SC pH. However, more recent evidence has emerged that identifies endogenous sources as the primary mode of SC acidification. A sodium/proton pump, NHE1, has been implicated in acidifying the border between stratum granulosum and SC. Another significant mechanism contributing to the acid mantle is free fatty acid conversion from phospholipid by a secretory phospholipase A2 (sPLA2). Lastly, urocanic acid generation via histidase is postulated to be another endogenous source of acid, although this has been disputed. Studies of the neonatal acidification processes in murine epidermis demonstrate the central role sPLA2 plays in forming the acid mantle at the beginning of life. Maintenance of an acidic SC in adults, however, appears to require additional mechanisms. This (secretory phospholipase A2 family) enzyme plays a significant and varied role in many mammalian cells, and the individual localization and function of the various sPLA2 members identified in skin (sPLA2-IB, -IIA, -IIC, -IID, -IIE, -IIF, -III, -V, -X, and -XII) still must be defined. More importantly, given the role of sPLA2s in inflammatory dermatoses such as atopic dermatitis and psoriasis, further discoveries could lead to novel, life-changing therapies.
Introduction
Epidermis forms a barrier between the dry external environment and the interior of the body, preventing water and ions from exiting and toxins, antigens and bacteria from entering.Citation1 Residing in the extracellular lipid membranes of the stratum corneum (SC), this vital barrier requires an acidic environment in order to remain functional and competent against various insults.Citation2 The acid pH not only limits the growth of pathogenic skin flora,Citation3 but also is required for the enzymatic lipid processing that results in an effective permeability barrier.Citation2
The mechanisms behind this “acid mantle” have long been debated. Originally, exogenous sources of acid were thought to cause this drop in SC pH.Citation3,Citation4 However, more recent evidence has emerged that identifies endogenous sources as the primary mode of SC acidification. A sodium/proton pump, NHE1, has been implicated in acidifying the border between stratum granulosum and SC.Citation5 Another significant mechanism contributing to the acid mantle is free fatty acid conversion from phospholipid by a secretory phospholipase A2 (sPLA2).Citation6 Lastly, urocanic acid generation via histidase is postulated to be another endogenous source of acid,Citation7 although this has been disputed.Citation1
Studies of the neonatal acidification processes in murine epidermis demonstrate the central role sPLA2 plays in forming the acid mantle at the beginning of life.Citation8 Maintenance of an acidic SC in adults, however, appears to require additional mechanisms.Citation9
This (secretory phospholipase A2 family) enzyme plays a significant and varied role in many mammalian cells and the individual localization and function of the various sPLA2 members identified in skin (sPLA2-IB, -IIA, -IIC, -IID, -IIE, -IIF, -III, -V, -X and -XII) still must be defined.Citation10 More importantly, given the role of sPLA2s in inflammatory dermatoses such as atopic dermatitis and psoriasis,Citation11,Citation12 further discoveries could lead to novel, life-changing therapies.
Function of Acidification
The identification of the SC “acid mantle” was first recognized by Heuss in 1892.Citation13 Subsequently, there have been numerous studies confirming this observation using progressively more sophisticated methods.Citation14–Citation21 Using tape stripping to remove sequential layers of the SC, Ohman and Valhquist demonstrated that SC pH was inhomogenous, distributed with a progressively neutral pH from the apex to the base of the SC.Citation20 Later studies using fluorescent lifetime imaging revealed discrete microdomains of acidity within larger areas of neutral pH at the base of the SC.Citation5 It is here that the process of SC acidification begins in the neonatal period, as well as the period after barrier abrogation.Citation22 The increasing acidity seen in the upper SC layers derives from an increasing number of uniformly acidic microdomains rather that the increasing acidity of a fixed number of individual microdomains.Citation5,Citation22
It has long been recognized that SC acidification functions as a defense against microbial invasion, as it was shown that an acidic pH inhibits the colonization of pathogens such as Staphylococcus aureus and Streptococcus pyogenes while encouraging the growth of normal skin flora.Citation3,Citation23 In addition, the loss of acidic pH coupled with the initiation of the inflammatory cycle in epidermal barrier disruptions can create a vicious cycle of inflamed and colonized skin. An alkaline environment, as in the urea-soaked skin of diaper dermatitis, is an important initiating factor of bacterial and yeast infections,Citation24 due to the increased growth of pathogens and epidermal barrier abnormality.
In recent years, SC acidity has been shown to be required for formation of a competent permeability barrier.Citation25 It has been shown to be important in the formation of a permeability barrier to protect the moist interior of the body from the dry external environment. This barrier consists of the external lipid membranes between the corneocytes of the SC.Citation26 This lipid is excreted from lamellar bodies (LB) in SG keratinocytes but does not form lipid bilayers and function as an effective permeability barrier until it is processed into various lipid species, including ceramide. Ca2+ and K+ control lipid secretion;Citation27 however H+ controls post-secretory lipid processing.Citation25 Several studies have shown that an acidic SC is required in order for the formation of a functionally competent permeability barrier, as without an acidic environment, the extracellular processing of lipids that is required for the formation of an effective permeability barrier cannot occur. A significant delay in barrier recovery is seen when acutely disrupted skin sites are immersed in neutral pH buffers.Citation25 Specifically, two key enzymes, β-glucocerebrosidase and acid sphingomyelinase, which metabolize spingomyelin and glucosylceramide to ceramide, require on an acidic pH for optimal enzymatic activity.Citation28,Citation29 The activity of β-glucocerebrosidase is reduced in skin with a neutral pH and activity is restored after re-acidification of the epidermis.Citation2 Abnormalities of SC integrity and cohesion also occur in a neutral pH environment.Citation2 Accelerated corneodesmosome (CD) degradation accompanies this loss of SC integrity, which is attributed to increased serine protease activity at a neutral pH.Citation2 Inhibiting serine proteases maintains CD density, preserving the integrity of the SC. Together, these two pathways can explain why the loss of SC acidification leads to loss of SC integrity and cohesion, as well as the abnormality of epidermal lipid barrier function.
Mechanisms of Acidification
There are many mechanisms that have been postulated to contribute to the acid mantle of the skin (see ). Originally, exogenous mechanisms have long been thought to be the main contributors to acidification. For example, eccrine gland-derived products, such as lactic acidCitation4 is one of many exogenous sources thought to further decrease SC pH. Free fatty acids of pilosebaceous origins also were thought to be an exogenous contributing mechanism.Citation3,Citation30 The significance of this mechanism is questionable, as a normal SC acid mantle is seen in asebia mice, which show a profound hypoplasia of sebaceous glands.Citation8 Microbial metabolites also were postulated to contribute to an acidic pH.Citation30 However, the microbial colonization of neonatal skin is not seen to increase concurrently with early acidification. In addition, acidification has been shown to begin from the deeper levels of the SC, not the surface, which would be expected if these exogenous pathways were indeed the source of the acid mantle.Citation8 This evidence seems to minimize, if not exclude, these exogenous mechanisms from contributing to SC acidification.
More recently, studies have identified that endogenous mechanisms are essential in the formation of an acidic SC. Endogenous mechanisms such as the Sodium-Hydrogen antiporter (NHE1) and activation of the sPLA2 family of enzymes are the best-studied of these mechanisms.
When either the sPLA2 or NHE-1 pathways are compromised, the bulk SC pH rises, indicating that other acidifying mechanisms cannot completely compensate for them. NHE-1, one of the essential contributors to the acid mantle, is from a family of ion transporters that is important in intracellular regulation and maintenance.Citation31 NHE-1 has been shown to be expressed in keratinocytes and melanocytes.Citation32 NHE-1 acidifies the extracellular microdomains present at the SG-SC interface, where initial processing of lipids by β-glucocerebrosidase and acid sphingomyelinase occur.Citation5 Impairment of lipid processing and alteration of barrier function occurs within 2 hours of pharmacological inhibition of NHE1, moreover NHE1 knockout mice lack these acidic domains in the lower SC and suffer impaired barrier function.Citation5 Additionally, this important component of the acidification process is directly regulated by changes in external pH, as alkalinization is shown to be a major stimulus for NHE1 expression.Citation33 Defects of acidification in the lower domains of aged epidermis have recently been attributed to decreased NHE1 expression.Citation9 This pathway plays a significant role in contributing to the SC pH, possibly being the mechanism providing the initial acidification required for lipid processing and permeability barrier formation.
Phospholipid to free fatty acid conversion by sPLA2 has emerged as another significant mechanism of lowering SC pH. PL disappear during cornificationCitation34 and the processing of PL results in a family of nonessential fatty acids within the SC interstices, which are required for normal barrier homeostasis.Citation6 The specific phospholipases responsible for this phenomenon have not been fully elucidated, but the application of inhibitors of the 14 kDa family of secretory phospholipases result in a blockade of PL processing, altering epidermal barrier homeostasis.Citation6,Citation35 A more recent study has shown that the inhibition of sPLA2 specifically alters barrier function via disrupting SC acidification, providing direct evidence in its role in contributing to the acid mantle.Citation36 The consequences of the disruption of acidification, such as the loss of SC integrity, cohesion and delayed barrier recovery, are shown to occur with the blockade of the sPLA2 pathway. Furthermore, these abnormalities can be prevented during sPLA2 inhibition with the co-application of the end products of PL hydrolysis, non-essential FFA such as PA (C16:0) or stearic acid (18:0).Citation36 An acidic pH alone is not sufficient to reverse the barrier abnormalities, showing that the PL-derived generation of FFA is not only significant as an acidification mechanism, but also important in the formation of the barrier (see ).
Finally, in vitro studies have shown acidification via urocanic acid generation from histidine by the deiminating enzyme histidase.Citation7 It is questionable whether this pathway is truly essential for acidification of SC. Although levels of histidase would be expected to rise in conjunction with neonatal SC acidification, a recent study has shown that this is not the case.Citation1 Additionally, this study showed histidase deficient mice do not exhibit a higher pH compared to control mice and flaky tail mice (ft/ft), deficient in the processing of filaggrin, actually show a lower SC pH. Two other endogenous mechanisms of acidification, free fatty acid generation by isoforms of secretory phospholipase A2 (sPLA2) and sodium/proton pump antiporter-1 (NHE-1), are upregulated in ft/ft mice.Citation1 This compensatory response is sufficient to appropriately acidify the SC, demonstrating that the filaggrin-histidine-urocanic acid cascade is not essential in the formation of the acid mantle.
The Importance of sPLA2: Neonatal Studies
Among the variety of pathways that contribute to epidermal acidification, it can be shown that sPLA2 conversion of PL to FFA is the most significant in neonatal SC acidification. Skin surface pH is neutral at birth in both humans and animals.Citation37–Citation39 Although a fully developed cornified envelope and abundant extracellular lamellar bilayers are present,Citation40,Citation41 the lack of acidification in neonatal skin is associated with, impaired barrier homeostasis has been shown in the neutral SC of neonates, even though basal barrier function has been shown to be normal.Citation42
Examining the mechanisms involved in the development of acidification in the first week of birth in neonatal rats elucidated that sPLA2 is largest contributor to the drop in SC pH. sPLA2 activity increases 66.4% between day 0 and 4 after birth and progressively extends from the deep layers of the SC to all SC layers by day 5.Citation8 Application of a sPLA2 inhibitor delayed the development of the acid mantle, accounting for a two-third to one pH unit of bulk acidification.Citation36 Evidence points to sPLA2 as the major pathway of acidification, whereas other mechanisms contribute and prevent the complete blockade of acidification. Interestingly, concurrent inhibition of NHE1 and sPLA2 did not increase neonatal SC pH above levels achieved with either inhibitor alone, suggesting the existence of yet unidentified mechanisms of acidification.Citation8
Recently, several studies have shown that activation of LXR, a nuclear hormone receptor, can accelerate SC acidification in neonatal rodents, in part because of an increase in sPLA2 activity.Citation43 This accelerated drop in epidermal pH was accompanied by an improvement of SC integrity and barrier homeostasis. This study provides some insight to sPLA2 regulation, although it is possible LXR can also be modulating other pathways of acidification.
Peroxisome proliferators-activated receptors (PPARs) are members of the same subfamily of nuclear hormone receptors as LXR and isotypes PPARα, PPARβ/δ and PPARγ have all be shown to be expressed in keratinocytes.Citation44 Topical treatment with PPAR activators not only stimulates keratinocyte differentiation,Citation45,Citation46 it improves permeability barrier homeostasis after an acute disruption.Citation47 Topical PPARα application is shown to accelerate neonatal acidification, as well as accelerate the kinetics of barrier recovery following acute disruption by tape stripping. Activation of only the PPARα isoform was shown to increase sPLA2 activity.Citation48 Concurrent inhibition of sPLA2 with the application of a PPARα activator blocked the ability of the PPARα activator to acidify the neonatal SC. However, the inhibitor of sPLA2 also regulates Ca2+ influx;Citation49 therefore, another mechanism could be preventing the PPARα activator from restoring acidifying processes. Although it seems like sPLA2 plays a prominent role in acidification, there is still much to be uncovered about the specific regulation of this enzyme.
Declining SC Acidification in Aging
SC acidification is defective in both moderately aged humans and mice, although the contributing mechanisms are not fully elucidated. Studies have shown that in humans over 50 years old, the surface pH of SC increases progressively over time.Citation9 This was also found in a mouse model (aged 12–15 months), where researchers were able to assess that the difference begins at the SG-SC interface and diverged further throughout the epidermis.Citation9 Delayed barrier recovery rates are seen in aged mice in comparison to young mice and recovery rates in aged skin became comparable to young skin with external acidification treatment. Similar abnormalities seen in neonatal skin lacking an acidic SC are also seen in aged skin, including abnormal SC integrity and delayed formation of mature lamellar membranes. In contrast to studies on NHE1 in aging skin, loss of sPLA2 activity and/or expression has not been adequately examined in aged epidermis.
However, NHE1 levels show a developmental decrease with aging and seem to contribute to the loss of acidification in aged epidermis.Citation9 The actions of NHE1 are localized to the lower SC, which is contiguous with the acidification defect seen in aged epidermis.Citation5,Citation9 In addition, the protein levels of NHE1 are seen to decrease after birth, in contrast to increasing levels of sPLA2 function postnatally.Citation8 The evidence is highly suggestive of the role NHE1 plays in contributing to the loss of acidification in aging. However, the extent to which loss of NHE1 contributes to a higher pH still needs to be quantitated and in uncovering that, the specific role of sPLA2 in the aging epidermis is not yet defined.
The Secretory Phospholipase Family
Secretory phospholipase A2 is a subtype of the larger phospholipase A2 family. These enzymes catalyze the hydrolysis of the sn-2 ester bond of phospholipid substrates, producing free fatty acids and lysophospholipids.Citation10 These products have a wide variety of functions across many mammalian tissues, including the formation of eicosanoids, which are important mediators of vascular tone, tissue homeostasis and inflammation. There are 12 groups of phospholipases A2 (I–XII) and are subdivided into different categories based on substrate specificities, cellular location and calcium sensitivity: group IV cytosolic phospholipases A2, group VI calcium-independent phospholipases A2, groups VII and VIII platelet-activating factor acetyl hydrolases and low molecular weight secreted phospholipases A2 (sPLA2-IB, -IIA, -IIC, -IID, -IIE, -IIF, -III, -V, -X and -XII).Citation10
sPLA2s play a part in normal skin function, maintaining barrier homeostasis through contributing to the acid mantle. However, it is still not clear exactly which of the many sPLA2 subtypes is responsible for FFA release and subsequent acidification. There have been many studies attempting to shed some light on the many different roles of sPLA2, but unfortunately, there are many contradictory reports and very little unrefuted findings in this area (see ). One hurdle to establishing definitive data is the difference of sPLA2 localization and function between humans and mice. For example, murine sPLA2-IIA is expressed almost exclusively in the small intestine, whereas human sPLA2-IIA is found in numerous tissues.Citation50 This suggests that the enzyme in the human and mouse models are not functional orthologs of each other, therefore conclusions drawn in a murine model cannot necessarily apply to the human model.
In a study analyzing different subtypes expressed in the different layers of epidermis during various stages of development, murine epidermis expressed groups I, II, V, X and XII sPLA2s,Citation51 although expression between the basal and suprabasal layers was dependent on calcium conditions. sPLA2-IIF, -V and -XII were expressed suprabasally, according to in vivo immunohistochemical studies. Their presence in the upper SC suggested that they also might have a role in SC acidification, in addition to sPLA2-IB that has previously been thought to function in that regard.Citation6,Citation52 However, the sPLA2-IB inhibitor used in that study, p-bromophenacyl bromide, also alkylates the active site histidine that is conserved in all sPLA2s and most likely inhibits multiple sPLA2s.Citation53 A more recent study has shown that in filaggrin-deficient ft/ft mice, sPLA2-IIA is upregulated along with NHE1 expression, while sPLA2 forms -IIF and -X do not,Citation1 contrasting with a previous study that demonstrated that sPLA2-IIA does not contribute to acidification and barrier function and sPLA2s-X is the main isoform to be secreted from keratinocytes.Citation54 To assign more definitive roles to these isoforms, more enzyme-specific sPLA2s antibodies and inhibitors are needed, which in part will enable the identification of specific sPLA2s present within LB. Cytochemical studies show that sPLA2 activity is codelivered with ceramide precursors and phospholipids (PL) in lamellar bodies at the SG-SC interface,Citation55 but more specific EM localization and antibody studies are lacking.
In addition to SC acidification, members of the sPLA2 family have been postulated to mediate inflammation, hyperproliferation and even stimulate melanocyte dendricity. The basal compartment exhibits a different expression profile than the suprabasal layers, showing the presence of sPLA2-IIA, -IID, -X. sPLA2-IIC is found throughout the epidermis.Citation51 This result, as well as other studies in references Citation56 and Citation57, suggest that sPLA2-IIA functions in the hyperproliferation of keratinocytes. sPLA2-X has been shown to release arachidonic acid from adherent cells,Citation58 suggesting this enzyme may play a role in eicosanoid formation. sPLA2-X also functions in the release of lysophophatidylcholine (LPC), stimulating melanocyte dendricity.Citation59 sPLA2-IIA and -IID may also have the ability to release arachidonic acid,Citation58 therefore it is hypothesized that these basally located sPLA2s may play a role in inflammatory hyperproliferation.
A recent study has shown a possible inflammatory role for sPLA2-III in skin, as transgenic mice overexpressing sPLA2-III exhibit severe dermatitis.Citation60 It also showed upregulation of sPLA2-IID and -V in these transgenic mice, suggesting that these two isoforms are involved in inflammatory processes. Surprisingly, there was a marked absence of sPLA2-X in the skin of transgenic and normal mice, as previous studies suggested sPLA2-X is the major isoform expressed in mouse skin. This same study provided evidence of sPLA2-III in normal epidermis for the first time,Citation51,Citation60 in addition to its role in inflammation.
There is much to be discovered about the sPLA2 family of enzymes. Hopefully, with the development of more specific inhibitors and more sensitive technology, their function and localization can be definitively pinpointed. With that knowledge, they can be used as therapeutic targets in many inflammatory diseases of the epidermis.
sPLA2 in Psoriasis and Atopic Dermatitis
The important role of sPLA2s in the epidermis is demonstrated by its involvement in atopic dermatitis and psoriasis. Psoriasis is a disorder characterized by epidermal hyperproliferation, altered keratinocyte maturation and inflammation. An early study showed that overexpression of sPLA2IIA in the skin of transgenic mice result in hyperkeratosis and epidermal hyperplasia, resembling the classic psoriasis phenotype.Citation61 More recently, studies have shown a dramatic change in the location of sPLA2-X in psoriatic skin. It disappears from its usual location in the basal compartment of the SC into the papillae and lower portions of the dermis.Citation11 Its release from HaCat keratinocytes is also stimulated by TNFα and IFNγ, which are prominent cytokines in psoriatic skin.Citation11 sPLA2-IID is seen to be massively upregulated throughout the psoriatic epidermis and dermis,Citation11 suggesting its role in inflammatory processes. Additionally, increased expression of sPLA2-IIA in the basal epidermal layer and the dermis was seen, which supports previous claims that the IIA isoform is involved in hyperproliferation.Citation56,Citation57 Increased sPLA2-IIA levels could possibly contribute to the sustained activation of mitogen-activated protein (MAP) kinase, resulting in delayed terminal differentiation and hyperproliferation of keratinocytes.Citation62 sPLA2-V was found to be completely downregulated in psoriatic skin, indicating a lack of involvement in this particular skin disease, although it could play a role in other inflammatory disorders. These findings are just the beginning of elucidating what effect these enzymes have on the psoriatic disease process and if they could be effective targets for therapy.
Atopic dermatitis (AD) is another common skin disorder that is marked by inflammatory dysregulation. Its chronic cycles of pruritus, dermatitis and lichenification are characterized by an elevated pH and impaired barrier homeostasis, leading to a host of negative downstream consequences, including lipid depletion.Citation63 It is not clear what the role of sPLA2 plays in the pathogenesis of this disease, but it was found that sPLA2s-IIF and -IID are upregulated in the skin of mice with induced AD.Citation12 Another study showed the upregulation of sPLA2-IIE mRNA in epidermis of mice with AD, however, there are no protein studies yet available on this finding.Citation64,Citation65 These results suggest the role of these sPLA2s in the inflammatory process in AD. However, it is yet to be seen whether their pathogenic mechanism contributes to lipid dysfunction, cytokine dysfunction via arachidonic acid release or perhaps even antimicrobial dysfunction. More recent studies suggest that sPLA2-IIA, -IID or -V play a role in platelet-activating factor arachidonic acid release and could be potential targets for anti-inflammatory interventions in AD and other dermatoses.Citation66
Determining the in vivo biological functions of sPLA2s is a daunting challenge. However, determining those functions can lead to novel and targeted treatments for atopic dermatitis and psoriasis, as well as other common inflammatory skin diseases.
Figures and Tables
Figure 1 Endogenous and exogenous pathways of acidification and functional consequences in the stratum corneum. Acidification in the SC is the result of several mechanisms. Specific functions of the SC are dependent on this process to maintain the epidermal permeability barrier.
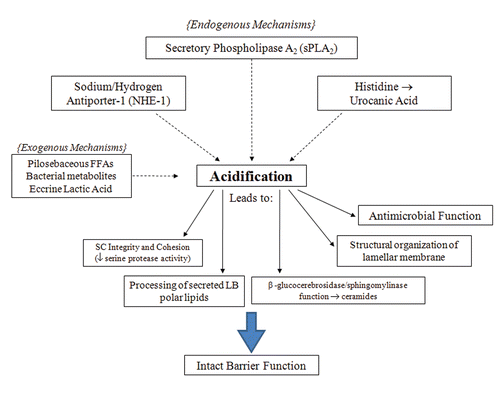
Figure 2 The downstream effects of the phopholipid to free fatty acid pathway in the stratum corneum interstices. Lamellar bodies deliver both phospholipid and sPLA2 to the SC. This pathway, mediated by sPLA2, plays a functional, as well as structural role, in the maintenance of the barrier.
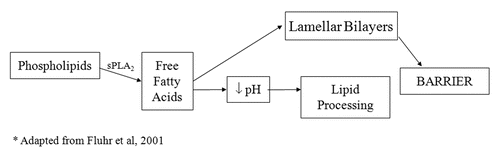
Table 1 Localization and function of sPLA2 subtypes in the epidermis.
Acknowledgements
This work was supported by NIH grant 1R01AG028492 (T.M.). Thanks to Jerelyn Magnusson for expert secretarial assistance.
References
- Fluhr JW, Elias PM, Man MQ, Hupe M, Selden C, Sundberg JP, et al. Is the filaggrin-histidine-urocanic acid pathway essential for stratum corneum acidification?. J Invest Dermatol 2010; 130:2141 - 2144
- Hachem JP, Crumrine D, Fluhr J, Brown BE, Feingold KR, Elias PM. pH directly regulates epidermal permeability barrier homeostasis and stratum corneum integrity/cohesion. J Invest Dermatol 2003; 121:345 - 353
- Puhvel SM, Reisner RM, Sakamoto M. Analysis of lipid composition of isolated human sebaceous gland homogenates after incubation with cutaneous bacteria. Thin-layer chromatography. J Invest Dermatol 1975; 64:406 - 411
- Thueson DO, Chan EK, Oechsli LM, Hahn GS. The roles of pH and concentration in lactic acid-induced stimulation of epidermal turnover. Dermatol Surg 1998; 24:641 - 645
- Behne MJ, Meyer JW, Hanson KM, Barry NP, Murata S, Crumrine D, et al. NHE1 regulates the stratum corneum permeability barrier homeostasis. Microenvironment acidification assessed with fluorescence lifetime imaging. J Biol Chem 2002; 277:47399 - 47406
- Mao-Qiang M, Feingold KR, Jain M, Elias PM. Extracellular processing of phospholipids is required for permeability barrier homeostasis. J Lipid Res 1995; 36:1925 - 1935
- Krien PM, Kermici M. Evidence for the existence of a self-regulated enzymatic process within the human stratum corneum—an unexpected role for urocanic acid. J Invest Dermatol 2000; 115:414 - 420
- Fluhr J, Behne M, Brown BE, Moskowitz DG, Selden C, Mao-Qiang M, et al. Stratum corneum acidification in neonatal skin: Secretory phospholipase A2 and the sodium/hydrogen antiporter-1 acidify neonatal rat stratum corneum. J Invest Dermatol 2004; 122:320 - 329
- Choi EH, Man MQ, Xu P, Xin S, Liu Z, Crumrine DA, et al. Stratum corneum acidification is impaired in moderately aged human and murine skin. J Invest Dermatol 2007; 127:2847 - 2856
- Valentin E, Lambeau G. Increasing molecular diversity of secreted phospholipases A(2) and their receptors and binding proteins. Biochim Biophys Acta 2000; 1488:59 - 70
- Haas U, Podda M, Behne M, Gurrieri S, Alonso A, Furstenberger G, et al. Characterization and differentiation-dependent regulation of secreted phospholipases A in human keratinocytes and in healthy and psoriatic human skin. J Invest Dermatol 2005; 124:204 - 211
- Murakami M, Yoshihara K, Shimbara S, Sawada M, Inagaki N, Nagai H, et al. Group IID heparin-binding secretory phospholipase A(2) is expressed in human colon carcinoma cells and human mast cells and upregulated in mouse inflammatory tissues. Eur J Biochem 2002; 269:2698 - 2707
- Heuss E. Die Reaktion des Schweisses beim gesunden Menschen. Monatsschr Prakt Dermatol 1892; 14:343 - 501
- Schade H, Marchionini A. Zur physikalischen chemie der hautoberflache. Arch f Derm u Syph 1928; 290 - 293
- Blank IH. Measurement of pH of the skin surface. I Technique. J Invest Dermatol 1939; 67 - 74
- Draize JH. The determination of the pH of th skin of man and common laboratory animals. J Invest Dermatol 1942; 5:77
- Schirren CG. Does the glass electrode determine the same pH-values on the skin surface as the quinhydrone electrode?. J Invest Dermatol 1955; 24:485 - 488
- Beare JM, Cheeseman EA, Gailey AA, Neill DW. The pH of the skin surface of children with seborrhoeic dermatitis compared with unaffected children. Br J Dermatol 1958; 70:233 - 241
- Dikstein S, Zlotogorski A. Measurement of skin pH. Acta Derm Venereol Suppl (Stockh) 1994; 185:18 - 20
- Ohman H, Vahlquist A. In vivo studies concerning a pH gradient in human stratum corneum and upper epidermis. Acta Derm Venereol 1994; 74:375 - 379
- Ohman H, Vahlquist A. The pH gradient over the stratum corneum differs in X-linked recessive and autosomal dominant ichthyosis: a clue to the molecular origin of the “acid skin mantle”?. J Invest Dermatol 1998; 111:674 - 677
- Behne MJ, Barry NP, Hanson KM, Aronchik I, Clegg RW, Gratton E, et al. Neonatal development of the stratum corneum pH gradient: localization and mechanisms leading to emergence of optimal barrier function. J Invest Dermatol 2003; 120:998 - 1006
- Korting HC, Lukacs A, Vogt N, Urban J, Ehret W, Ruckdeschel G. Influence of the pH-value on the growth of Staphylococcus epidermidis, Staphylococcus aureus and Propionibacterium acnes in continuous culture. Zentralbl Hyg Umweltmed 1992; 193:78 - 90
- Brook I. Microbiology of secondarily infected diaper dermatitis. Int J Dermatol 1992; 31:700 - 702
- Mauro T, Holleran WM, Grayson S, Gao WN, Man MQ, Kriehuber E, et al. Barrier recovery is impeded at neutral pH, independent of ionic effects: implications for extracellular lipid processing. Arch Dermatol Res 1998; 290:215 - 222
- Elias PM, Menon GK. Structural and lipid biochemical correlates of the epidermal permeability barrier. Adv Lipid Res 1991; 24:1 - 26
- Lee SH, Elias PM, Feingold KR, Mauro T. A role for ions in barrier recovery after acute perturbation. J Invest Dermatol 1994; 102:976 - 979
- Holleran WM, Takagi Y, Menon GK, Legler G, Feingold KR, Elias PM. Processing of epidermal glucosylceramides is required for optimal mammalian cutaneous permeability barrier function. J Clin Invest 1993; 91:1656 - 1664
- Feingold KR. The importance of lipids in cutaneous function. J Lipid Res 2007; 48:2529 - 2530
- Di Marzio L, Cinque B, De Simone C, Cifone MG. Effect of the lactic acid bacterium Streptococcus thermophilus on ceramide levels in human keratinocytes in vitro and stratum corneum in vivo. J Invest Dermatol 1999; 113:98 - 106
- Noel J, Pouyssegur J. Hormonal regulation, pharmacology and membrane sorting of vertebrate Na+/H+ exchanger isoforms. Am J Physiol 1995; 268:283 - 296
- Sarangarajan R, Shumaker H, Soleimani M, Le Poole C, Boissy RE. Molecular and functional characterization of sodium-hydrogen exchanger in skin as well as cultured keratinocytes and melanocytes. Biochim Biophys Acta 2001; 1511:181 - 192
- Hachem JP, Man MQ, Crumrine D, Uchida Y, Brown BE, Rogiers V, et al. Sustained serine proteases activity by prolonged increase in pH leads to degradation of lipid processing enzymes and profound alterations of barrier function and stratum corneum integrity. J Invest Dermatol 2005; 125:510 - 520
- Elias PM, Menon GK, Grayson S, Brown BE. Membrane structural alterations in murine stratum corneum: relationship to the localization of polar lipids and phospholipases. J Invest Dermatol 1988; 91:3 - 10
- Mao-Qiang M, Jain M, Feingold KR, Elias PM. Secretory phospholipase A2 activity is required for permeability barrier homeostasis. J Invest Dermatol 1996; 106:57 - 63
- Fluhr JW, Kao J, Jain M, Ahn SK, Feingold KR, Elias PM. Generation of free fatty acids from phospholipids regulates stratum corneum acidification and integrity. J Invest Dermatol 2001; 117:44 - 51
- Behrhrendt H, Green M. Pattern of skin pH from birth through adolescence 1971; Springfield Thomas
- Visscher MO, Chatterjee R, Munson KA, Pickens WL, Hoath SB. Changes in diapered and nondiapered infant skin over the first month of life. Pediatr Dermatol 2000; 17:45 - 51
- Yosipovitch G, Maayan-Metzger A, Merlob P, Sirota L. Skin barrier properties in different body areas in neonates. Pediatrics 2000; 106:105 - 108
- Akiyama M, Smith LT, Shimizu H. Expression of transglutaminase activity in developing human epidermis. Br J Dermatol 2000; 142:223 - 225
- Hanley K, Jiang Y, Holleran WM, Elias PM, Williams ML, Feingold KR. Glucosylceramide metabolism is regulated during normal and hormonally stimulated epidermal barrier development in the rat. J Lipid Res 1997; 38:576 - 584
- Fluhr J, Fowler AJ, Hachem JP, Crumrine D, Elias PM, Feingold KR. Stratum corneum acidification in neonates: Impact on permeability barrier homeostasis and integrity/cohesion. J Invest Dermatol 2002; 119:249
- Fluhr JW, Crumrine D, Mao-Qiang M, Moskowitz DG, Elias PM, Feingold KR. Topical liver x receptor activators accelerate postnatal acidification of stratum corneum and improve function in the neonate. J Invest Dermatol 2005; 125:1206 - 1214
- Kuenzli S, Saurat JH. Peroxisome proliferator-activated receptors in cutaneous biology. Br J Dermatol 2003; 149:229 - 236
- Komuves LG, Hanley K, Lefebvre AM, Man MQ, Ng DC, Bikle DD, et al. Stimulation of PPARalpha promotes epidermal keratinocyte differentiation in vivo. J Invest Dermatol 2000; 115:353 - 360
- Mao-Qiang M, Fowler AJ, Schmuth M, Lau P, Chang S, Brown BE, et al. Peroxisome-proliferator-activated receptor (PPAR)gamma activation stimulates keratinocyte differentiation. J Invest Dermatol 2004; 123:305 - 312
- Man MQ, Choi EH, Schmuth M, Crumrine D, Uchida Y, Elias PM, et al. Basis for improved permeability barrier homeostasis induced by PPAR and LXR activators: liposensors stimulate lipid synthesis, lamellar body secretion and post-secretory lipid processing. J Invest Dermatol 2006; 126:386 - 392
- Fluhr JW, Man MQ, Hachem JP, Crumrine D, Mauro TM, Elias PM, et al. Topical peroxisome proliferator activated receptor activators accelerate postnatal stratum corneum acidification. J Invest Dermatol 2009; 129:365 - 374
- Ogata Y, Sakurai T, Nakao S, Kuboyama N, Moriwaki K, Furuyama S, et al. 4-Bromophenacyl bromide induces Ca2+ influx in human gingival fibroblasts. Comp Biochem Physiol C Toxicol Pharmacol 2002; 131:315 - 322
- Cupillard L, Mulherkar R, Gomez N, Kadam S, Valentin E, Lazdunski M, et al. Both group IB and group IIA secreted phospholipases A2 are natural ligands of the mouse 180 kDa M-type receptor. J Biol Chem 1999; 274:7043 - 7051
- Gurrieri S, Furstenberger G, Schadow A, Haas U, Singer AG, Ghomashchi F, et al. Differentiation-dependent regulation of secreted phospholipases A2 in murine epidermis. J Invest Dermatol 2003; 121:156 - 164
- Mazereeuw-Hautier J, Redoules D, Tarroux R, Charveron M, Salles JP, Simon MF, et al. Identification of pancreatic type I secreted phospholipase A2 in human epidermis and its determination by tape stripping. Br J Dermatol 2000; 142:424 - 431
- Singer AG, Ghomashchi F, Le Calvez C, Bollinger J, Bezzine S, Rouault M, et al. Interfacial kinetic and binding properties of the complete set of human and mouse groups I, II V, X and XII secreted phospholipases A2. J Biol Chem 2002; 277:48535 - 48549
- Schadow A, Scholz-Pedretti K, Lambeau G, Gelb MH, Furstenberger G, Pfeilschifter J, et al. Characterization of group X phospholipase A(2) as the major enzyme secreted by human keratinocytes and its regulation by the phorbol ester TPA. J Invest Dermatol 2001; 116:31 - 39
- Freinkel RK, Traczyk TN. Lipid composition and acid hydrolase content of lamellar granules of fetal rat epidermis. J Invest Dermatol 1985; 85:295 - 298
- Mulherkar R, Rao R, Rao L, Patki V, Chauhan VS, Deo MG. Enhancing factor protein from mouse small intestines belongs to the phospholipase A2 family. FEBS Lett 1993; 317:263 - 266
- Desai SJ, Mulherkar R, Wagle AS, Deo MG. Ontogeny of enhancing factor in mouse intestines and skin. Histochemistry 1991; 96:371 - 374
- Murakami M, Kambe T, Shimbara S, Higashino K, Hanasaki K, Arita H, et al. Different functional aspects of the group II subfamily (Types IIA and V) and type X secretory phospholipase A(2)s in regulating arachidonic acid release and prostaglandin generation. Implications of cyclooxygenase-2 induction and phospholipid scramblase-mediated cellular membrane perturbation. J Biol Chem 1999; 274:31435 - 31444
- Scott GA, Arioka M, Jacobs SE. Lysophosphatidylcholine mediates melanocyte dendricity through PKCzeta activation. J Invest Dermatol 2007; 127:668 - 675
- Sato H, Taketomi Y, Isogai Y, Masuda S, Kobayashi T, Yamamoto K, et al. Group III secreted phospholipase A2 transgenic mice spontaneously develop inflammation. Biochem J 2009; 421:17 - 27
- Grass DS, Felkner RH, Chiang MY, Wallace RE, Nevalainen TJ, Bennett CF, et al. Expression of human group II PLA2 in transgenic mice results in epidermal hyperplasia in the absence of inflammatory infiltrate. J Clin Invest 1996; 97:2233 - 2241
- Haase I, Hobbs RM, Romero MR, Broad S, Watt FM. A role for mitogen-activated protein kinase activation by integrins in the pathogenesis of psoriasis. J Clin Invest 2001; 108:527 - 536
- Elias PM. Therapeutic implications of a barrier-based pathogenesis of atopic dermatitis. Ann Dermatol 2010; 22:245 - 254
- Murakami M, Yoshihara K, Shimbara S, Lambeau G, Gelb MH, Singer AG, et al. Cellular arachidonate-releasing function and inflammation-associated expression of group IIF secretory phospholipase A2. J Biol Chem 2002; 277:19145 - 19155
- Murakami M, Yoshihara K, Shimbara S, Lambeau G, Singer A, Gelb MH, et al. Arachidonate release and eicosanoid generation by group IIE phospholipase A(2). Biochem Biophys Res Commun 2002; 292:689 - 696
- Jorgensen KM, Felberg HS, Berge RK, Laegreid A, Johansen B. Platelet activating factor stimulates arachidonic acid release in differentiated keratinocytes via arachidonyl non-selective phospholipase A2. Arch Dermatol Res 2010; 302:221 - 227