Abstract
In plants, a particular class of short interfering (si)RNAs can serve as a signal to induce cytosine methylation at homologous genomic regions. If the targeted DNA has promoter function, this RNA-directed DNA methylation (RdDM) can result in transcriptional gene silencing (TGS). RNA-directed transcriptional gene silencing (RdTGS) of transgenes provides a versatile system for the study of epigenetic gene regulation. We used transcription of a nopaline synthase promoter (ProNOS)-inverted repeat (IR) to provide a RNA signal that triggers de novo cytosine methylation and TGS of a homologous ProNOS copy in trans. Utilizing a ProNOS-NPTII reporter gene showing high sensitivity to silencing in this two component system, a forward genetic screen for EMS-induced no rna-directed transcriptional silencing (nrd) mutations was performed in Arabidopsis thaliana. Three nrd mutant lines were found to contain one novel loss-of-function allele of idn2/rdm12 and two of nrpd2a/nrpe2a. IDN2/RDM12 encodes a XH/XS domain protein that is able to bind double-stranded RNA with 5′ overhangs, while NRPD2a/NRPE2a encodes the common second-largest subunit of the plant specific DNA-dependent RNA polymerases IV and V involved in silencing processes. Both idn2/rdm12 and nrpd2a/nrpe2a release target transgene expression and reduce CHH context methylation at transgenic as well as endogenous RdDM target regions to similar extents. Nevertheless, accumulation of IR-derived siRNA is not affected, allowing us to present a refined model for the pathway of RdDM and RdTGS that positions function of IDN2 downstream of siRNA formation and points to an important role for its XH domain.
Introduction
Methylation of cytosines at position 5 is a common modification of plant genomic DNA that is associated with epigenetic phenomena such as transgene silencing, transposon suppression, maternal/paternal imprinting and paramutation.Citation1,Citation2 In the genome of the model plant Arabidopsis thaliana, approximately 7% of cytosines are methylated.Citation3 As in other plants, and in contrast to mammalian somatic cells, where 5-methyl-cytosine occurs in CG context only, DNA methylation in A. thaliana occurs in CG as well as CHG and CHH context (with H standing for C, A or T). Shotgun bisulfite sequencing studies revealed that around 24% of cytosines in CG context, but only 6.7% in CHG and 1.7% in CHH context are methylated.Citation4 Only unmethylated cytosines are incorporated during DNA replication. Thus, DNA methylated on both strands before semi-conservative replication will bear methylated cytosines just on one strand, the template strand, afterwards. This leads to the necessity to methylate cytosines on the newly synthesized strand in order to re-establish the initial DNA methylation pattern. The maintenance of methylation in CG context in A. thaliana is performed by the Dnmt1-class enzyme METHYLTRANSFERASE 1 (MET1) using the hemimethylated DNA as substrate,Citation5 whereas maintenance of CHG methylation is achieved by the activity of the plant specific CHROMOMETHYLASE 3 (CMT3) thought to be guided by histone H3 lysine 9 dimethylation.Citation6,Citation7 A particular case is DNA methylation in CHH context, which is established de novo by DOMAIN REARRANGED METHYLTRANSFERASE 2 (DRM2) after each cell cycle in reaction to a sequence specific signal provided by a class of small RNAs.Citation8-Citation10
Small RNA-based pathways are important for genome stability over generations,Citation11 as well as for regulation of gene expression, the latter being performed either at the level of transcription, referred to as transcriptional gene silencing (TGS), or at the level of mRNA stability or translation, referred to as post-transcriptional gene silencing (PTGS).Citation12 The pathways involving small RNAs in plants share common biochemical features. A double stranded (ds)RNA is formed that is subsequently processed to 21–24 nt long dsRNA fragments exhibiting staggered ends with 3′ overhangs. One strand of such a dsRNA fragment is then incorporated into an effector complex and guides this complex to partially or fully complementary RNA or DNA to conduct regulatory function. Genetic analysis has established a core pathway leading to siRNA-mediated DNA methylation and related TGS of endogenous as well as transgenic targets in A. thaliana (for a review see refs. Citation13-Citation16). It is initiated by the production of single stranded RNA from target sequences by the plant specific DNA-dependent RNA polymerase IV (RNAP IV).Citation17,Citation18 The resulting RNAP IV-dependent RNAs (p4-RNAs)Citation19 then serve as substrate of RNA-DEPENDENT RNA POLYMERASE 2 (RDR2), which synthesizes a complementary strand to generate long dsRNA.Citation20,Citation21 This is then processed by DICER-LIKE 3 (DCL3) into small dsRNAs of predominantly 24 nt length.Citation20,Citation21 The 3′ termini of resulting dsRNA fragments are subsequently methylated by HUA ENHANCER1 (HEN1).Citation22,Citation23 Single short interfering (si)RNA strands from the fragments are loaded onto ARGONAUTE4 (AGO4)Citation24,Citation25 and to a lesser extent onto related AGO6 and AGO9.Citation26-Citation28 A second plant specific DNA-dependent RNA polymerase V (RNAP V) is thought to produce different transcripts (p5-RNAs)Citation19 of target loci that serve as scaffold to attract the siRNA-AGO complexes,Citation17,Citation29-Citation32 which in turn guide DRM2 to the genomic loci to be methylated de novo.Citation10,Citation33 DNA methylation at target sequences might then serve as a signal for further transcription by RNAP IV, allowing self-perpetuation of RdDM via a positive feedback loop.
In order to extend the knowledge of factors involved in RNA-directed transcriptional gene silencing (RdTGS), we performed a forward genetic screen in A. thaliana using a transgene system showing particularly efficient silencing of a single T-DNA-copy transgene locus via RdTGS.Citation34,Citation35 Thus, we identified new mutant alleles of INVOLVED IN DE NOVO 2/RNA-DIRECTED DNA METHYLATION 12 (IDN2/RDM12), a recently identified component of the RdDM pathway,Citation36,Citation37 with the ability to bind dsRNA with 5′ overhangs,Citation38-Citation40 and of NUCLEAR RNA POLYMERASE D 2a/NUCLEAR RNA POLYMERASE E 2a (NRPD2a/NRPE2a), the common second-largest subunit of RNAP IV and RNAP V.Citation30 Based on the effects of idn2/rdm12 in comparison to nrpd2a/nrpe2a on target gene expression, RdDM of transgenic as well as endogenous target regions and siRNA accumulation, we present a refined model for RdDM and RdTGS that positions function of IDN2/RDM12 downstream of siRNA formation, with a potential role in siRNA-p5-RNA interaction.
Results
Forward-genetic screen for mutations abrogating RNA-directed transcriptional gene silencing
To extend the knowledge of factors involved in RdDM and related RdTGS, we performed a screen for ethyl methanesulfonate (EMS)-induced nrd mutants that reactivate expression of a silenced reporter gene, according to the strategy outlined by Page and Grossniklaus.Citation41 The A. thaliana line (K/K;H/H) submitted to mutagenesis comprised a SILENCER (H) transgene residing on chromosome 4 containing an inverted repeat (IR) of the NOPALIN SYNTHASE promoter (ProNOS) sequence under control of the cauliflower mosaic virus 35S promoter (Pro35S)Citation34 and a TARGET (Kchr1–10) transgene on chromosome 1 containing a NEOMYCIN PHOSPHOTRANSFERASE II (NPTII) reporter gene controlled by a ProNOS conferring resistance to kanamycin (), which was previously found to show efficient DNA methylation and silencing in the presence of the SILENCER.Citation35,Citation42 Transcription of the ProNOS-IR in the SILENCER leads to formation of double-stranded RNA, which is processed to short interfering (si) RNAs with ProNOS-homology. These siRNAs can induce DNA methylation of homologous DNA sequences in trans and thus transcriptionally silence ProNOS-controlled genes.Citation34 As TARGET Kchr1–10 is highly sensitive to RdTGS, plants homozygous for TARGET and SILENCER (K/K;H/H) are sensitive to kanamycin (). Seeds were incubated with EMS, sown and grown to M1 plants, which were allowed to self-pollinate. The resulting M2 seeds, the first generation in which a mutation can be homozygous and thus, if recessive, show its impact on the phenotype, were germinated on medium containing 200 mg/l kanamycin to screen for individuals that had reverted to kanamycin resistance. Presence and integrity of TARGET and SILENCER in resulting M2 nrd candidates were verified via PCR using primer combinations specific for different parts of the transgenes. Accordingly, the symbol “nrd” is used to refer to mutant plants homozygous for TARGET and SILENCER transgene unless specified otherwise. M2 plants were allowed to self-pollinate and kanamycin resistance was verified for the resulting M3 generation (). M3 seedlings of independent mutant lines nrd1 as well as nrd2–1 and nrd2–2 (for which intercrosses had revealed that they affect the same gene; see Fig. S1A) showed consistent resistance when grown on medium containing kanamycin, but did not have the same vigor as wild-type seedlings homozygous for the TARGET in the absence of the SILENCER (K/K).
Figure 1. Mutations nrd1, nrd2–1 and nrd2–2 release RdTGS and RdDM of a ProNOS-NPTII reporter gene. (A) Transgene system: The SILENCER (H) transgene contains an inverted repeat (IR) of the NOPALINE SYNTHASE promoter (ProNOS) sequence under control of the strong constitutive cauliflower mosaic virus 35S promoter (Pro35S). Transcripts of the ProNOS-spacer-SONorP structure can fold to form double-stranded RNA with ProNOS-homology, which is then processed to short interfering (si)RNAs. These siRNAs serve as a signal for in trans DNA methylation and transcriptional silencing of a ProNOS copy that controls transcription of a NEOMYCIN PHOSPHOTRANSFERASE II (NPTII) (conferring kanamycin resistance if expressed) in the unlinked TARGET (K) transgene. In addition, the SILENCER contains a HYGROMYCIN PHOSPHOTRANSFERASE (HPT) gene conferring hygromycin resistance and the TARGET a GUS reporter gene (not shown). (B) Test for kanamycin resistance on medium containing 200 mg/l kanamycin. (C) Quantification of NPTII protein by ELISA: NPTII protein amouts in relation to total soluble protein were measured in extracts from leaves of 8-week-old plants. Results are displayed relative to the mean value for un-silenced expression in (K/K;-/-) plants (set to 1). Per genotype, five individual plants were tested. Column hights represent mean values; error bars represent standard deviations. (D) TARGET ProNOS cytosine methylation was determined by quantitative PCR after cleavage of genomic DNA from 8-week-old plants with methylation-sensitive restriction enzymes (C in recognition sequence underlined: methylation of cytosine blocks cleavage according to REBASE http://rebase.neb.com/rebase/rebase.html) Psp1406I (olive, symmetric CG context: AACGTT), NheI (blue, CHG and CHH context: GCTAGC), Alw26I (orange, asymmetric CHH context: GTCTC, GAGAC) and NcoI (yellow, asymmetric CHH context, control outside of the methylated region: CCATGG). Per genotype, five individual plants were tested. Results are displayed relative to the mean value for input DNA (set to 1). Column highs represent mean values; error bars represent standard deviations.
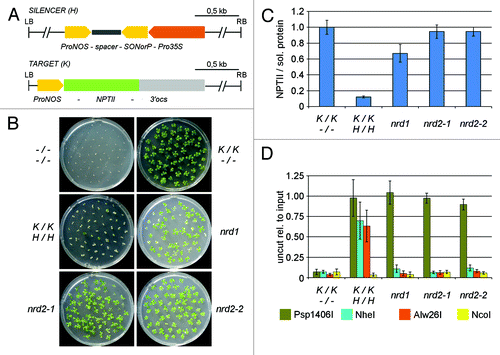
As kanamycin resistance can arise in A. thaliana mutants by loss of chloroplast-localized transporter proteins required for kanamycin uptake,Citation43,Citation44 rather than by reactivated NPTII expression, the amounts of NPTII protein in mutant plants were tested by ELISA (). M3 nrd1, nrd2–1 and nrd2–2 plants showed clearly more NPTII than (K/K;H/H) plants, indicating that their kanamycin resistance was due to a reactivation of NPTII expression. However, in particular in nrd1, NPTII levels stayed below that of (K/K;−/−) control plants containing the native TARGET transgene. Consistent with the allelic status of nrd2–1 and nrd2–2, high NPTII levels were found in their intercrosses (Fig. S1B). To address whether nrd1, nrd2–1 and nrd2–2 mutations release RdDM of the TARGET transgene, we analyzed ProNOS DNA methylation in the ProNOS-NPTII reporter gene by methylation sensitive restriction enzyme cleavage-qPCR (). The results showed a reduction of cytosine methylation in non-CG context (NheI, Alw26I), but not CG context (Psp1406I), which is a hallmark of mutations affecting RdDM.Citation21,Citation29,Citation45,Citation46 Cleavage with NcoI, whose recognition site lies outside the region targeted by RdDM and thus is not methylated, served as a control for the accessibility of genomic DNA.Citation35
Map-based cloning identifies nrd1 as a new idn2 allele and nrd2–1 and nrd2–2 as new nrpd2a alleles
M3 plants of nrd1, nrd2–1 and nrd2–2 were crossed with A. thaliana accession Ler to establish mapping populations (Fig. S2A). F2 generation seedlings obtained from these crosses by self-pollination were screened for individuals resistant to hygromycin (HygR, SILENCER present) and kanamycin (KanR, TARGET present, homozygous for mutation releasing RdTGS). HygR KanR frequencies were approximately 11% for nrd1, nrd2–1 and nrd2–2 (Fig. S2B), consistent with the 14% expected for single recessive mutations. To rule out possible false positives, F3 progeny was obtained from F2 by self-pollination and germinated in presence of hygromycin and kanamycin. Only F2 that met the expected minimal 56% HygRKanR in their F3 progeny (data not shown) were included in mapping populations.
The nrd1 mutation was mapped to a region on the lower arm of chromosome 3 (Fig. S3A) spanning ~555 kb physical distance as defined by the recombination events positioned between markers P613960 and P616207 as well as P617590 and IDMS3, respectively (). Sequencing of gene loci known to be involved in RdDM within this region revealed a G→A mutation at position 1883 in exon 5 of IDN2 (At3g48670, ). To verify that the mutation in IDN2 is causative for the release of RdDM and RdTGS in nrd1, we complemented nrd1 by introducing the wild-type IDN2 gene via Agrobacterium-mediated transformation (Fig. S4A). Batches of T2 generation seedlings obtained from five independent T1 transformants by self-pollination were tested on kanamycin-containing medium ( and data not shown). Approximately 75% of T2 progeny showed sensitivity to kanamycin, a proof of successful complementation of nrd1 by a single IDN2 transgene locus. T2 plants of two of these lines that were further analyzed showed decreased NPTII levels (Fig. S4B) as well as re-established DNA methylation at the endogenous RdDM target AtSN1 (Fig. S4C). Thus, nrd1 is a new idn2 allele and was renamed idn2–8 according to idn2 mutant alleles described previously.Citation36-Citation40 The G→A transition in idn2–8 leads to the exchange of a glycine (G) residue for an arginine (R) at protein level (). Mutant idn2–8 transcripts accumulate to similar levels as IDN2 transcripts (Fig. S5A), pointing to an effect of the idn2–8 mutation at the protein rather than the mRNA level. Sequence alignment of XH-domain containing proteins of A. thaliana and Oryza sativa revealed that the affected G residue is highly conserved (Fig. S5B). Hence, the replacement of the glycine might compromise XH domain function.
Figure 2. Map-based cloning of nrd1. (A) Physical map indicating markers and recombination events (numbers in parentheses, of 234 chromosomes in total) used to delineate the position of nrd1 on the lower arm of chromosome 3. (B) Positions of the nucleotide (top) and related amino acid change (bottom) in nrd1 in the IDN2 gene model (according to TAIR 10). (C) Complementation of nrd1 by transgenic IDN2. Seeds were germinated on medium containing 200 mg/l kanamycin. Approx. 75% of T2 progeny obtained by self-pollination of (single locus) nrd1 + IDN2 T1 transformants inherit a transgenic functional IDN2 and thus are sensitive to kanamycin due to re-establishment of RdTGS of the ProNOS-NPTII gene.
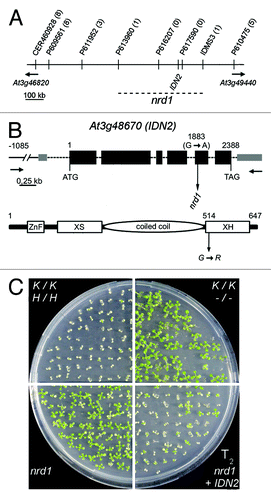
Mutations nrd2–1 and nrd2–2 were mapped to a region at the upper arm of chromosome 3 (Fig. S3B and C, respectively) spanning ~829 kb physical distance as defined by recombination events between markers C3AB015474 and C3P0484614 as well as MN38693286 and CER456071, respectively (). Sequencing of gene loci known to be involved in RdDM within this region revealed G→A mutations in exon 2 at position 1590 (nrd2–2) and exon 7 at position 5977 (nrd2–1) of NRPD2a/NRPE2a (At3g23780, ) encoding the common, second-largest subunit of DNA-dependent RNA polymerase IV and V.Citation30 As nrd2–1 and nrd2–2 were shown to be allelic (Fig. S1) and NRPD2a/NRPE2a is well established to be required for transgene RdTGS,Citation29 it is very likely that these nucleotide changes are causative for the release of RdTGS in nrd2–1 and nrd2–2, respectively. Thus, nrd2–1 was renamed nrpd2a-54 and nrd2–2 was renamed nrpd2a-55 following the nrpd2a allele counting by Lopez et al.Citation47 The G→A transition in nrpd2a-54 leads to an exchange of a glutamate for a lysine at position 1079 of the protein, while the G→A transition in nrpd2a-55 leads to a substitution of a glycine for an aspartate at position 174. Both affected amino acids are highly conserved among the second largest subunits of DNA-dependent RNA polymerases.Citation48-Citation50
Figure 3. Map-based cloning of nrd2–1 and nrd2–2. (A) Physical map indicating markers and recombination events (numbers in parentheses separated by semicolon, of 172 chromosomes in total for nrd2–1 and 134 for nrd2–2, respectively) used to delineate the position of nrd2 on the upper arm of chromosome 3. (B) Positions of the nucleotide (top) and related amino acid change (bottom) in nrd2–1 and nrd2–2 in the NRPD2a/NRPE2a gene model (according to TAIR10).
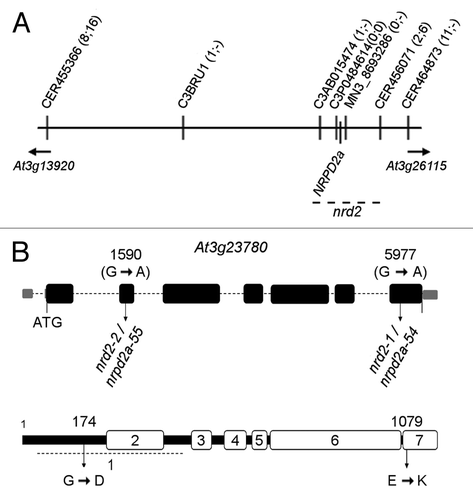
CHH and CHG-context DNA methylation at RdDM targets is similarly reduced in idn2 and nrpd2a mutants
As methylation sensitive restriction cleavage-qPCR () can test methylation only at the few restriction sites available, the overall methylation of the ProNOS in the TARGET ProNOS-NPTII reporter gene in wild-type and in M3 generation of idn2–8, nrpd2a-54 and nrpd2a-55 plants was determined by bisulfite sequencing (; Fig. S6). The results showed that cumulative cytosine methylation in the ProNOS region undergoing RdDM that is close to 70% in wild-type (K/K;H/H) plants is reduced to 25% and below in all three mutants (). This reduction is primarily due to an extensive loss of methylation in CHH context, with a somewhat less pronounced effect in idn2–8 than in nrpd2a-54 and nrpd2a-55. Methylation in CG context is hardly altered in idn2–8 and only slightly reduced in nrpd2a-54 and nrpd2a-55 plants compared with wild-type. The analysis of the spatial distribution of the cytosine methylation along the ProNOS shows an almost uniform reduction at CHH context sites, while the partial loss of methylation in CG and CHG context seems more prominent toward the transcription start site (TSS) at the 3′ end of the promoter ().
Figure 4. Detailed TARGET ProNOS DNA methylation analysis in idn2–8, nrpd2a-54 and nrpd2a-55. DNA methylation patterns in the ProNOS of the ProNOS-NPTII reporter gene were analyzed in detail by bisulfite sequencing. (A) Cumulative methylation levels at all cytosines in the analyzed region (gray columns), cytosines in CG context (black columns), CHG context (blue columns; H stands for A, C or T) and CHH context (red columns). (B) Methylation levels at individual cytosines in CG context (black columns), CHG context (blue columns) and CHH context (red columns). A black arrowhead marks the ProNOS transcription start site. Numbers of clones sequenced per target and genotype were: 15 (K/K −/−), 19 (K/K;H/H), 20 (idn2–8), 15 (nrpd2a-54), 21 (nrpd2a-55)
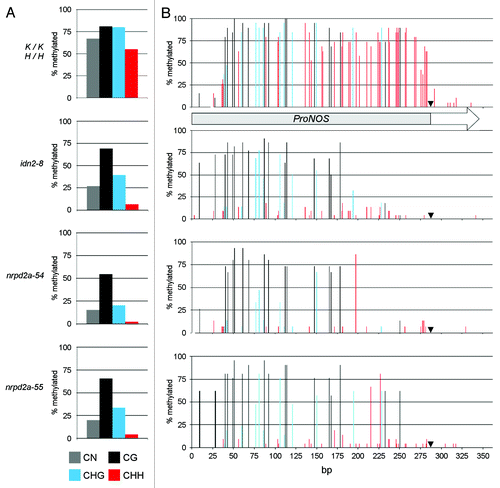
To ensure that methylation loss was not limited to the ProNOS of the TARGET, DNA methylation at the endogenous RdDM targets AtSN1,Citation24 MEA-ISRCitation9 and AtMU1,Citation51 and at AtCOPIA4 as a RdDM independent control,Citation52 was examined by bisulfite sequencing (; Figs. S7–10). In M3 idn2–8, nrpd2a-54 and nrpd2a-55 plants, DNA methylation is obviously reduced at AtSN1 and MEA-ISR in CHH and CHG context and at AtMU1 in CHH context only. Methylation in CG context resembles wild-type levels in all three mutants. Methylation at AtCOPIA4 is unaltered in all contexts in idn2–8, nrpd2a-54 and nrpd2a-55. Thus these mutations affect methylation patterns by inhibition of de novo methylation in a similar way at transgenic and endogenous RdDM targets.
Figure 5. Detailed DNA methylation analysis at endogenous AtSN1, MEA-ISR, AtMu1 and AtCOPIA4 sequences in idn2–8, nrpd2a-54 and nrpd2a-55. DNA methylation patterns of endogenous sequences (A) AtSN1, (B) AtMU1, (C) MEA-ISR and (D) AtCOPIA4 were analyzed in detail by bisulfite sequencing in non-mutagenized control plants (black columns), idn2–8 (dark gray columns), nrpd2a-54 (light gray columns) and nrpd2a-55 (white columns). A minimum of 12 clones was sequenced per target and genotype. Exact numbers are indicated in Figures S8–10.
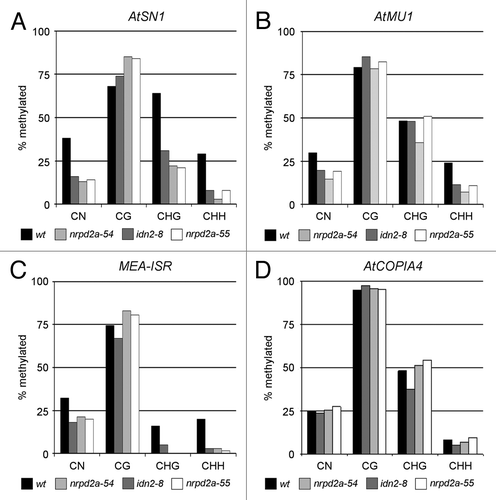
ProNOS-IR derived siRNAs are not affected in idn2 and nrpd2a mutants
As there was extensive loss of CHH context ProNOS methylation, we analyzed the amount of ProNOS-IR derived 24nt siRNAs in wild-type, idn2–8 and nrpd2a-55 plants (). Northern blots showed no differences in SILENCER transgene-derived 24nt, 22nt and 21nt siRNAs between wild-type and mutant plants. This indicates the requirement of IDN2/RDM12 and NRPD2a/NRPE2a for ProNOS-NPTII silencing in the used transgene system in steps downstream of siRNA formation.
Figure 6.ProNOS-derived and endogenous siRNAs in idn2–8 and nrpd2a-55. Northern blot for siRNA derived from transcription of ProNOS IR in the SILENCER. (A) Blots were hybridized with a RNA probe specific for sense ProNOS siRNAs. (B) Equal loading was confirmed by re-hybridization with miR167-specific probe after stripping.
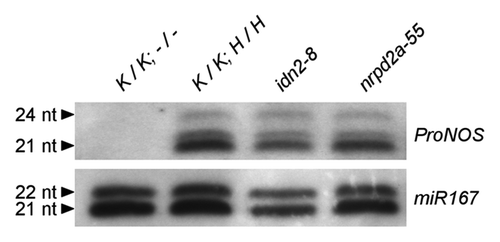
Discussion
The nearly complete loss of CHH context methylation at AtSN1 and CHH and CHG context methylation at MEA-ISR in idn2–8 is similar to results presented by Ausin et al.Citation38,Citation53 for deletion allele idn2–1 and by Zhang et al.Citation40 for T-DNA insertion allele idn2–5/rdm12–2, respectively. This suggests that idn2–8 is a loss-of-function allele. The observation that a single amino acid exchange in the XH domain severely compromises IDN2/RDM12 function points to an important role of this domain. And as the extend of reduction of CHH context methylation at ProNOS, AtSN1, MEA-ISR and AtMU1 in nrpd2a-54 and nrpd2a-55 is similar to that in idn2–8, nrpd2a-54 and nrpd2a-55 can be considered loss-of-function alleles as well.
A core pathway for RdDM in A. thaliana has emerged from genetic analysis (). Both, NRPD2a/NRPE2a and IDN2/RDM12 have been previously reported to be required for RdDM at endogenousCitation17,Citation18,Citation38,Citation53 and transgenic target sequences.Citation29,Citation36,Citation37 For NRPD2a/NRPE2a as common second-largest component of RNAP IV and RNAP V,Citation30 the placement in the circular RdDM pathway is well established.Citation15,Citation54 Less clear is where in the pathway IDN2/RDM12 is localized. Similar to SUPPRESSOR OF GENE SILENCING 3 (SGS3), its counterpart from PTGS,Citation55 IDN2/RDM12 has the potential to bind dsRNA with blunt ends and 5′ overhangs via its XS domain in vitro.Citation38-Citation40 However, as dsRNA occurs more than once in the RdDM pathway,Citation15 alternative positions of IDN2/RDM12 action have been suggested. In analogy to the cooperation of SGS3 and RDR6 in generating dsRNA in PTGS,Citation55 IDN2/RDM12 has been proposed to team up with RDR2 in the production of dsRNA from p4-transcripts.Citation36 Alternatively, IDN2/RDM12 could act in stabilizing siRNAs-p5-transcript complexes in the process of guiding DRM2-mediated DNA methylation.Citation38 In our transgene system ProNOS dsRNA is generated by RNAP II-dependent transcription of the promoter-IR in the SILENCER transgene. Thus, RdDM in this experimental system works according to a linear pathway in which dsRNA and subsequent primary siRNA formation are independent from RNAP IV and V and are not affected by nrpd2a-55. Similar observations have been made for nrpd2aCitation29 and rdr2 mutants,Citation21 in a comparable transgene setup. ProNOS siRNAs in idn2–8 plants were also not reduced. As ProNOS CHH context DNA methylation was markedly reduced at the same time, IDN2/RDM12 needs to have a role downstream of siRNA formation in RdDM. Consistently, siRNAs derived from endogenous RdDM-target AtSN1 are only partially reduced in idn2/rdm12 mutants, while a strong impact of idn2/rdm12 on CHH and CHG context methylation as seen by us and others for AtSN1, MEA-ISR and AtMU1.Citation36,Citation38,Citation40
Figure 7. Genetic model of RdDM. The core pathway leading to RdDM is initiated by the production of single stranded RNA from target sequences by multi-subunit RNAP IV. The resulting p4-RNA then serves as substrate of RDR2, which synthesizes a complementary strand to generate dsRNA. This dsRNA is then processed by DCL3 into 24 nt dsRNA fragments and single strands of 24 nt short interfering (si)RNA are incorporated mainly into AGO4. Multi-subunit RNAP-V is thought to transcribe RdDM target loci, with the resulting p5-RNA serving as scaffold to attract the siRNA-AGO4 complexes, which in turn guide DRM2 to the genomic loci to be methylated de novo. Transcription of an inverted repeat (IR) by multi-subunit RNAP II provides a shortcut in the pathway, as dsRNA as a substrate for DCL3 action is produced independently of RNAP IV and RDR2 (solid arrows). NRPD2a/NRPE2a (golden) is a subunit common to RNAP IV and RNAP V, but is not required for RNAP II function. IDN2 (red) has a role downstream of siRNA formation, possibly by stabilizing a siRNA-p5-RNA complex.
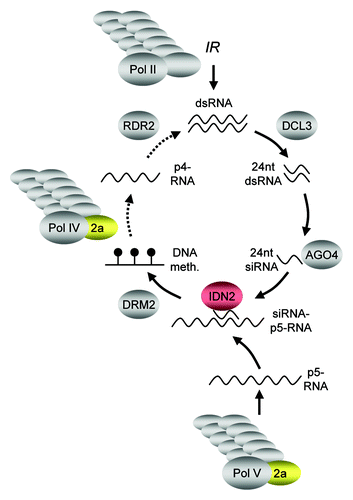
IDN2/RDM12 is a member of a large gene family in plants characterized by the presence of zinc finger, XS, coiled-coil and XH domains.Citation61 Somewhat contradicting results have been reported on the possible involvement of further members of this gene family in RdDM. Two independent studies by Zhang et al.Citation40 and Ausin et al.Citation53 combining genetic and biochemical approaches did not find functional redundancy between IDN2/RDM12 and gene family members FDM1/IDP1/IDNL1 and FDM2/IDP2/IDNL2. Rather, FDM1/IDP1/IDNL1 and FDM2/IDP2/IDNL2 were reported to be functionally redundant and to form a complex with IDN2/RDM12 dimers via the XH domains. In contrast, Xie et al.Citation39 claimed based on analysis of double mutants that up to five gene family members, FDM1/IDP1/IDNL1, FDM2/IDP2/IDNL2, FDM3, FDM4 and FDM5 act partially redundant to IDN2/RDM12 in RdDM. However, their observation that double mutants showed stronger loss of DNA methylation than the respective single mutants might be due to the used alleles that carried T-DNA insertions in the 5′ UTR or in introns and thus could still have conferred some gene function. Our data showing extensive loss of CHH context DNA methylation in idn2–8 similar to nrpd2a-54 and nrpd2a-55 at the ProNOS and endogenous RdDM targets. This rather argues against the interpretation that IDN2/RDM12 function in RdDM can be replaced by other gene family members.
Thus, albeit a core pathway of RdDM in A. thaliana as a model plant is known, genetic analysis in a transgene-based experimental system shortcutting part of the pathway has provided important information. We expect that ongoing characterizing and mapping of mutations releasing transgene RdTGS will continue to produce valuable insight into gene silencing pathways.
Materials and Methods
Plant material and cultivation
The transgenic A. thaliana line double homozygous for TARGET and SILENCER transgenes (Kchr1–10/Kchr1–10;H/H) has been described in Fischer et al.Citation35 A. thaliana was cultivated on soil at 21°C under a 16 h light/8 h dark (long day) regime for propagation and seed production; and at 21°C under a 8 h light/16 h dark (short day) regime for generation of rosette leaf material for molecular analysis. For kanamycin resistance tests, seeds were surface-sterilized (10 min, 8% NaClO) and germinated under long day regime on agar-plates with germination medium (½ strength MS salts, 10 g/l sucrose) containing 200 mg/l kanamycin. Resistance was evaluated according to root growth and primary leaf development after 3 weeks.
EMS mutant screen
EMS (ethyl-methanesulfonate) mutagenesis of seeds homozygous for TARGET and SILENCER transgenes (Kchr1–10/Kchr1–10;H/H) in the accession Col-0 was performed by Lehle Seeds. From the obtained 32 batches of M2 seeds, (each batch representing the progeny from approximately 1500 M1 plants), 20,000 seeds per batch were germinated on medium containing kanamycin (200 mg/l). Resistant M2 plants were transferred to soil and allowed to set seeds by selfing. The suppression of TGS was confirmed by germinating resulting M3 seeds on kanamycin containing selective medium (200mg/l). Lines showing more than 90% resistant M3 plants as judged by good root growth and development of primary leaves were considered true candidate lines for no rna-directed transcriptional silencing (nrd) mutations.
Protein analysis
Amount of NPTII protein was determined using Agdia PathoScreen Kit for NPTII (Agdia cat. no: PSP73000/0288). Rosette leafs of 8-week-old short-day-grown plants were flash frozen in liquid nitrogen, grinded using a swingmill (Retsch, MM301) and resuspended in protein extraction buffer. All further procedures were performed according to manufacturers’ recommendations. Per genotype, leaves from five individual plants were assayed in technical duplicates. Total protein in the same extracts was determined using a Pierce BCA Protein Assay kit (Pierce, cat. no. 23225). Twenty-five microliters of the NPTII ELISA raw extract were added to 500 µl of BCA working solution and incubated for 30 min at 37°C in a water bath. After incubation, 500 µl of bi-distilled water were added to every sample and absorbance at 592 nm was determined using an Ultrospec 3100pro UV/Vis spectrophotometer (Amersham Bioscience, cat. no. 80–2112–38) and converted to protein concentration using a BSA serial dilution in concentration range between 0.125 and 2 µg/ml as standard.
DNA methylation analysis using Bisulfite sequencing
Approximately 0.15 µg of DNA extracted from leaves of 8 week old plants grown under short day regime were bisulfite-treated using Qiagen Epitect Bisulfite Kit (Qiagen cat. no. 59104) following the manufacturer’s instructions. One microliter of treated DNA solution was used to amplify ProNOS, AtSN1, AtMU1, AtCOPIA4 and MEA-ISR in a 50 µL reaction using GoTaq Flexi DNA polymerase (Promega, cat. no. M8308). Primers used for amplification were bitop2f, bitop3r (ProNOS);Citation35 JP1821, JP1822 (AtSN1);Citation24 JP3100, JP3101 (AtCOPIA);Citation52 JP1026, JP1027 (MEA-ISR);Citation38 JP1387, JP1388 (AtMU1).Citation51 Amplified fragments were cloned into vector pSC-A using a Strataclone PCR cloning kit (Agilent Technologies cat.no. 240207). Plasmids were isolated using QuiaPrep Spin Miniprep Kit (Qiagen, cat. no. 27104) and checked for insert size via restriction cleavage using EcoRI. Positive clones were sequenced using M13 forward and M13 reverse primer. Sequences from at least 12 individual clones per locus and genotype were obtained and DNA methylation patterns were analyzed using CyMATE software.Citation56
DNA methylation analysis using methylation sensitive restriction enzymes
Approximately 50 ng of DNA extracted from adult leaves of 8 week old plants grown under short day regime dissolved in 400 µl of distilled water were added to 50 µl of 10-times Tango buffer (Fermentas) and 50 µl distilled water to reach a final volume of 500 µl. Aliquots of 100 µl were taken and combined with 10 U of restriction enzymes Psp1406I, NheI, Alw26I and NcoI (Fermentas), respectively. One control was kept without restriction enzyme. Aliquots were incubated at 37°C for 16 h and subsequently for 5 min at 85°C. After inactivation, 399 µl of bi-distilled water were added (final volume: 500 µL). Quantitative PCR was performed in 25 µl volume in an iCycler IQTM PCR device (Biorad cat. no 170–8740). 12.5 µL SYBR green Supermix (Biorad cat. no 170–8882) and 1.25 µL of ProNOS-top-F and ProNOS-top-rev primers (final concentration 0.25 µM each) were added to 10 µL of cleaved and control templates, respectively. The following temperature regime was used for the PCR: 5 min 95°C, 40x (15 sec 95°C, 30 sec 62°C, 30 sec 72°C). PCR was calibrated using a logarithmic dilution series from 10−2 to 10−5 of genomic DNA. Data analysis was performed using ΔΔCt method according to Pfaffl.Citation57 Results are presented relative to the mean signal obtained for the control samples without restriction enzyme.
Mapping of mutations
M3 nrd1, nrd2–1 and nrd2–2 (all K/K;H/H, respectively) mutant plants derived from accession Col-0 were crossed with wild-type mapping partner Landsberg erecta (Ler) by manual pollination of emasculated Ler flowers. Success of crosses was confirmed by GUS staining of leaf discs of the resulting F1 progeny. GUS positive plants were allowed to self-pollinate. The resulting F2 progeny was germinated on ½ strengh MS medium supplied with 10 g/l sucrose containing hygromycin (20 mg/l) and kanamycine (200 mg/l). The resistance phenotype was evaluated after 3 weeks and should only appear if the plant is homozygous for the respective mutation. Plants resistant to hygromycin and kanamycin (HygR KanR) were transferred to soil and allowed to reproduce by self-pollination. Individual DNA preparations were derived from leaf material. Potential “false positive” F2 plants erroneously scored HygR KanR were ruled out by checking segregation of resistance in their F3 progeny. A control population was established by crossing non-mutagenized wild-type (K/K;H/H) with Ler. The resulting F1 generation was checked by GUS assay and allowed to self-pollinate. Resulting F2 seeds were germinated on germination medium containing hygromycin (20 mg/l). HygR plants were transferred to soil and presence of the TARGET was confirmed by GUS assay. HygRGUS+ plants were checked for presence of the SILENCER transgene via specific PCR.
Construction of complementation vector and transformation procedure
To generate a minimal pCAMBIA without functional genes between left (LB) and right (RB) border, pCAMBIA1300 was cut with PdmI/XmnI (GAANN NNTTC; blunt end; three sites) and PvuII (CAG CTG; blunt end; two sites) and self-ligated. The resulting minimal pCAMBIA contained a single EcoRI restrictions site between LB and RB. A ProMAS-BAR-35Ster resistance cassette flanked by EcoRI-sites was amplified from genomic DNA of a SAIL line containing a T-DNA derived from pDAP101 into the EcoRI site of the minimal pCAMBIA to generate pCAMBIA-proMAS-BAR-35St (pCMBAR). LacZ and MCS of plasmid pGEM7f(+) were amplified using primers pGEM7Z-MCS-for (5′–AACCTGCAGGGCGCGTCCATTCGCCATTC-3′) and pGEM7Z-MCS-rev (5′-ATTCTGCAGCGGAAGAGCGCCCAATACGC-3′) and introduced into pCMBAR at a unique PstI restriction site. The resulting vector named pCMBL contains unique AatII, ZraI, PspXI, SciI, XhoI, XmaI, SmaI, BstBI, HindIII, BspEI and BstXI restriction sites for the insertion of DNA fragments. The wild-type IDN2 ORF (including 3′UTR) and a fragment of around 1300 bp upstream of the transcriptional start site was amplified from A. thaliana accession Col-0 genomic DNA using primers IDN2-clone-for (5′-CTTGACTCGAGACTTGCCTTGTGTCAGCG-3′) and IDN2-clone-rev (5′-ACGCTCGAGGGGTCAATATCAAATTTGAC-3′) to introduce a XhoI restriction sites and cloned into pSC-A vector using a Strataclone PCR-cloning kit (Agilent Technologies, cat.no. 240205). After propagating the vector, the functional IDN2 gene was excised by XhoI digestion and cloned into the XhoI restriction site of pCMBL2 yielding the binary vector pCMBL2+IDN2. pCMBL2+IDN2 was propagated in E. coli DH5α cells, purified using a Qiagen Plasmid Midi Kit (Qiagen, cat.no. 12143) and introduced into Agrobacterium tumefaciens strain pGV2260 by electroporation.
Small RNA analysis
For analysis of ProNOS-derived siRNAs, a RNA preparation enriched in small RNAs was extracted from leaves of 8 week old plants grown under short day regime. Leaves were harvested, frozen in liquid nitrogen and stored at -80°C. Approximately 500 mg of frozen plant material were ground to powder and resuspended in 15 ml of TRIzol reagent by vigorous vortexing for 1 min at room temperature. The suspension was transferred to a 30 ml Corex-tube and 3 ml of chloroform were added. After vortexing for 1 min, centrifugation for 30 min at 4°C in a Sorvall RC5B Plus centrifuge using rotor Sorvall HB-6 was performed. The upper phase was transferred into a 50 ml disposable vessel, 1 volume of 80% ethanol was added and the total volume was applied to an RNeasy Maxi (Qiagen, cat. no. 75162) column. After centrifugation for 5 min at 3166xg at room temperature, the flow-through was transferred to a new vessel and 1.4 volumes of 100% ethanol were added. Four ml of this solution were applied to a RNeasy Midi column (Qiagen, cat.no. 75142) followed by centrifugation for 5 min at 3166xg. This step was repeated until the whole volume had passed the column. The RNeasy Midi column was washed twice with RPE buffer (Qiagen) and subsequently the small RNA fraction was eluted by adding 250 µl of RNase-free water to the dried membrane followed by a final centrifugation step for 5 min at 3166 xg. After elution, the concentration of RNA was determined using an Ultrospec 3100pro UV/Vis spectrophotometer (Amersham Bioscience, cat. no. 80–2112–38). The eluted small RNAs were precipitated over night at -20°C by adding 3 M sodium acetate solution (pH 5.2) to a final concentration of 0.3 M and 2.5 volumes of 100% ethanol. After centrifugation at 18640xg for 20 min the sediment was washed with 70% ethanol and dried under vacuum for 30 min. The pellet was resuspended in 25 µl of RNase-free water and 25 µl of gel loading buffer II (Ambion, cat.no. AM8546G) were added. The mixture was incubated at 95°C for 5 min, separated using a 15% polyacrylamid – 7 M urea gel, blotted and probed for detection of ProNOS-sense siRNAs according to established protocols.Citation58,Citation59 For re-hybridization with a miR167 probe, the membrane was stripped at 95°C in 0.1x SSC containing 0.5% SDS. The miR167 probe (Table S1) was labeled with 32P using T4 POLYNUCLEOTIDE KINASE (Fermentas, cat.no. EK0031) according to manufacturer’s protocol. The stripped membrane was pre-hybridized with hybridization buffer according to Church and Gilbert for 16 h at 42°C.Citation60 Subsequently the labeled probe was added and allowed to hybridize for 24 h at 42°C. The blot was washed in 2xSSC containing 0.2% SDS and exposed to an X-ray film with intensifier screen for 3 d at -80°C.
Abbreviations: | ||
AGO | = | ARGONAUTE |
AtCOPIA4 | = | A. thaliana COPIA 4 |
AtMU1 | = | A. thaliana MUTATOR 1 |
AtSN1 | = | A. thaliana SINE 1 |
CMT3 | = | CHROMOMETHYLASE 3 |
Col-0 | = | Columbia-0 |
DCL3 | = | DICER-LIKE 3 |
DRM2 | = | DOMAINS-REARRANGED METHYLATRANSFERASE 2 |
dsRNA | = | double-stranded RNA |
EMS | = | ethyl methanesulfonate |
HEN1 | = | HUA ENHANCER1 |
IDN2 | = | INVOLVED IN DE NOVO 2 |
IR | = | inverted repeat |
Ler | = | Landsberg erecta |
MEA-ISR | = | MEDEA-INTERGENIC SUBTELOMERIC REPEATS |
MET1 | = | METHYLTRANSFERASE 1 |
NPTII | = | NEOMYCIN PHOSPHOTRANSFERASE II |
nrd | = | no rna-directed transcriptional silencing |
NRPD2a/NRPE2a | = | NUCLEAR RNA POLYMERASE D 2a / NUCLEAR RNA POLYMERASE E 2a |
p4-RNAs | = | RNAP IV-dependent RNAs |
p5-RNAs | = | RNAP V-dependent RNAs |
RNAP IV | = | RNA polymerase IV |
RNAP V | = | RNA polymerase V |
ProNOS | = | NOPALINE SYNTHASE promoter |
PTGS | = | post-transcriptional gene silencing |
RdDM | = | RNA-directed DNA methylation |
RDM12 | = | RNA-DIRECTED DNA METHYLATION 12 |
RdTGS | = | RNA-directed transcriptional gene silencing |
RDR2 | = | RNA-DEPENDENT RNA POLYMERASE 2 |
siRNA | = | short interfering RNA |
SGS3 | = | SUPPRESSOR OF GENE SILENCING 3 |
TGS | = | transcriptional gene silencing |
Additional material
Download Zip (8.7 MB)Acknowledgments
We thank Christa Fricke, Inge Glaser and Beate Kamm for excellent technical assistance and Renate Schmidt and Ingo Schubert for critical comments on the manuscript. This work received support from IPK Gatersleben (A.F.) and the German Research Foundation (DFG) collaborative research center (SFB) 648 “Molecular Mechanisms of Information Processing in Plants” (M.K.).
Disclosure of Potential Conflicts of Interest
No potential conflicts of interest were disclosed.
References
- Stam M, Mittelsten Scheid O. Paramutation: an encounter leaving a lasting impression. Trends Plant Sci 2005; 10:283 - 90; http://dx.doi.org/10.1016/j.tplants.2005.04.009; PMID: 15949762
- Slotkin RK, Martienssen R. Transposable elements and the epigenetic regulation of the genome. Nat Rev Genet 2007; 8:272 - 85; http://dx.doi.org/10.1038/nrg2072; PMID: 17363976
- Rozhon W, Baubec T, Mayerhofer J, Mittelsten Scheid O, Jonak C. Rapid quantification of global DNA methylation by isocratic cation exchange high-performance liquid chromatography. Anal Biochem 2008; 375:354 - 60; http://dx.doi.org/10.1016/j.ab.2008.01.001; PMID: 18249178
- Cokus SJ, Feng S, Zhang X, Chen Z, Merriman B, Haudenschild CD, et al. Shotgun bisulphite sequencing of the Arabidopsis genome reveals DNA methylation patterning. Nature 2008; 452:215 - 9; http://dx.doi.org/10.1038/nature06745; PMID: 18278030
- Kishimoto N, Sakai H, Jackson J, Jacobsen SE, Meyerowitz EM, Dennis ES, et al. Site specificity of the Arabidopsis METI DNA methyltransferase demonstrated through hypermethylation of the superman locus. Plant Mol Biol 2001; 46:171 - 83; http://dx.doi.org/10.1023/A:1010636222327; PMID: 11442057
- Henikoff S, Comai L. A DNA methyltransferase homolog with a chromodomain exists in multiple polymorphic forms in Arabidopsis.. Genetics 1998; 149:307 - 18; PMID: 9584105
- Lindroth AM, Cao X, Jackson JP, Zilberman D, McCallum CM, Henikoff S, et al. Requirement of CHROMOMETHYLASE3 for maintenance of CpXpG methylation. Science 2001; 292:2077 - 80; http://dx.doi.org/10.1126/science.1059745; PMID: 11349138
- Cao X, Jacobsen SE. Locus-specific control of asymmetric and CpNpG methylation by the DRM and CMT3 methyltransferase genes. Proc Natl Acad Sci U S A 2002; 99:Suppl 4 16491 - 8; http://dx.doi.org/10.1073/pnas.162371599; PMID: 12151602
- Cao X, Jacobsen SE. Role of the arabidopsis DRM methyltransferases in de novo DNA methylation and gene silencing. Curr Biol 2002; 12:1138 - 44; http://dx.doi.org/10.1016/S0960-9822(02)00925-9; PMID: 12121623
- Cao X, Aufsatz W, Zilberman D, Mette MF, Huang MS, Matzke M, et al. Role of the DRM and CMT3 methyltransferases in RNA-directed DNA methylation. Curr Biol 2003; 13:2212 - 7; http://dx.doi.org/10.1016/j.cub.2003.11.052; PMID: 14680640
- Wei W, Ba Z, Gao M, Wu Y, Ma Y, Amiard S, et al. A role for small RNAs in DNA double-strand break repair. Cell 2012; 149:101 - 12; http://dx.doi.org/10.1016/j.cell.2012.03.002; PMID: 22445173
- Hohn T, Vazquez F. RNA silencing pathways of plants: silencing and its suppression by plant DNA viruses. Biochim Biophys Acta 2011; 1809:588 - 600; PMID: 21683815
- Chinnusamy V, Zhu JK. RNA-directed DNA methylation and demethylation in plants. Sci China C Life Sci 2009; 52:331 - 43; http://dx.doi.org/10.1007/s11427-009-0052-1; PMID: 19381459
- Matzke M, Kanno T, Daxinger L, Huettel B, Matzke AJ. RNA-mediated chromatin-based silencing in plants. Curr Opin Cell Biol 2009; 21:367 - 76; http://dx.doi.org/10.1016/j.ceb.2009.01.025; PMID: 19243928
- Law JA, Jacobsen SE. Establishing, maintaining and modifying DNA methylation patterns in plants and animals. Nat Rev Genet 2010; 11:204 - 20; http://dx.doi.org/10.1038/nrg2719; PMID: 20142834
- Haag JR, Pikaard CS. Multisubunit RNA polymerases IV and V: purveyors of non-coding RNA for plant gene silencing. Nat Rev Mol Cell Biol 2011; 12:483 - 92; http://dx.doi.org/10.1038/nrm3152; PMID: 21779025
- Herr AJ, Jensen MB, Dalmay T, Baulcombe DC. RNA polymerase IV directs silencing of endogenous DNA. Science 2005; 308:118 - 20; http://dx.doi.org/10.1126/science.1106910; PMID: 15692015
- Onodera Y, Haag JR, Ream T, Costa Nunes P, Pontes O, Pikaard CS. Plant nuclear RNA polymerase IV mediates siRNA and DNA methylation-dependent heterochromatin formation. Cell 2005; 120:613 - 22; http://dx.doi.org/10.1016/j.cell.2005.02.007; PMID: 15766525
- Mosher RA, Tan EH, Shin J, Fischer RL, Pikaard CS, Baulcombe DC. An atypical epigenetic mechanism affects uniparental expression of Pol IV-dependent siRNAs. PLoS One 2011; 6:e25756; http://dx.doi.org/10.1371/journal.pone.0025756; PMID: 22003406
- Xie Z, Johansen LK, Gustafson AM, Kasschau KD, Lellis AD, Zilberman D, et al. Genetic and functional diversification of small RNA pathways in plants. PLoS Biol 2004; 2:E104; http://dx.doi.org/10.1371/journal.pbio.0020104; PMID: 15024409
- Daxinger L, Kanno T, Bucher E, van der Winden J, Naumann U, Matzke AJ, et al. A stepwise pathway for biogenesis of 24-nt secondary siRNAs and spreading of DNA methylation. EMBO J 2009; 28:48 - 57; http://dx.doi.org/10.1038/emboj.2008.260; PMID: 19078964
- Yang Z, Ebright YW, Yu B, Chen X. HEN1 recognizes 21-24 nt small RNA duplexes and deposits a methyl group onto the 2′ OH of the 3′ terminal nucleotide. Nucleic Acids Res 2006; 34:667 - 75; http://dx.doi.org/10.1093/nar/gkj474; PMID: 16449203
- Yu B, Bi L, Zhai J, Agarwal M, Li S, Wu Q, et al. siRNAs compete with miRNAs for methylation by HEN1 in Arabidopsis.. Nucleic Acids Res 2010; 38:5844 - 50; http://dx.doi.org/10.1093/nar/gkq348; PMID: 20448024
- Zilberman D, Cao X, Jacobsen SE. ARGONAUTE4 control of locus-specific siRNA accumulation and DNA and histone methylation. Science 2003; 299:716 - 9; http://dx.doi.org/10.1126/science.1079695; PMID: 12522258
- Li CF, Pontes O, El-Shami M, Henderson IR, Bernatavichute YV, Chan SW, et al. An ARGONAUTE4-containing nuclear processing center colocalized with Cajal bodies in Arabidopsis thaliana.. Cell 2006; 126:93 - 106; http://dx.doi.org/10.1016/j.cell.2006.05.032; PMID: 16839879
- Zheng X, Zhu J, Kapoor A, Zhu JK. Role of Arabidopsis AGO6 in siRNA accumulation, DNA methylation and transcriptional gene silencing. EMBO J 2007; 26:1691 - 701; http://dx.doi.org/10.1038/sj.emboj.7601603; PMID: 17332757
- Havecker ER, Wallbridge LM, Hardcastle TJ, Bush MS, Kelly KA, Dunn RM, et al. The Arabidopsis RNA-directed DNA methylation argonautes functionally diverge based on their expression and interaction with target loci. Plant Cell 2010; 22:321 - 34; http://dx.doi.org/10.1105/tpc.109.072199; PMID: 20173091
- Eun C, Lorkovic ZJ, Naumann U, Long Q, Havecker ER, Simon SA, et al. AGO6 functions in RNA-mediated transcriptional gene silencing in shoot and root meristems in Arabidopsis thaliana.. PLoS One 2011; 6:e25730; http://dx.doi.org/10.1371/journal.pone.0025730; PMID: 21998686
- Kanno T, Huettel B, Mette MF, Aufsatz W, Jaligot E, Daxinger L, et al. Atypical RNA polymerase subunits required for RNA-directed DNA methylation. Nat Genet 2005; 37:761 - 5; http://dx.doi.org/10.1038/ng1580; PMID: 15924141
- Ream TS, Haag JR, Wierzbicki AT, Nicora CD, Norbeck AD, Zhu JK, et al. Subunit compositions of the RNA-silencing enzymes Pol IV and Pol V reveal their origins as specialized forms of RNA polymerase II. Mol Cell 2009; 33:192 - 203; http://dx.doi.org/10.1016/j.molcel.2008.12.015; PMID: 19110459
- Wierzbicki AT, Haag JR, Pikaard CS. Noncoding transcription by RNA polymerase Pol IVb/Pol V mediates transcriptional silencing of overlapping and adjacent genes. Cell 2008; 135:635 - 48; http://dx.doi.org/10.1016/j.cell.2008.09.035; PMID: 19013275
- Lahmy S, Pontier D, Cavel E, Vega D, El-Shami M, Kanno T, et al. PolV(PolIVb) function in RNA-directed DNA methylation requires the conserved active site and an additional plant-specific subunit. Proc Natl Acad Sci U S A 2009; 106:941 - 6; http://dx.doi.org/10.1073/pnas.0810310106; PMID: 19141635
- Naumann U, Daxinger L, Kanno T, Eun C, Long Q, Lorkovic ZJ, et al. Genetic evidence that DNA methyltransferase DRM2 has a direct catalytic role in RNA-directed DNA methylation in Arabidopsis thaliana.. Genetics 2011; 187:977 - 9; http://dx.doi.org/10.1534/genetics.110.125401; PMID: 21212233
- Aufsatz W, Mette MF, van der Winden J, Matzke AJ, Matzke M. RNA-directed DNA methylation in Arabidopsis.. Proc Natl Acad Sci U S A 2002; 99:Suppl 4 16499 - 506; http://dx.doi.org/10.1073/pnas.162371499; PMID: 12169664
- Fischer U, Kuhlmann M, Pecinka A, Schmidt R, Mette MF. Local DNA features affect RNA-directed transcriptional gene silencing and DNA methylation. Plant J 2008; 53:1 - 10; http://dx.doi.org/10.1111/j.1365-313X.2007.03311.x; PMID: 17971044
- Zheng Z, Xing Y, He XJ, Li W, Hu Y, Yadav SK, et al. An SGS3-like protein functions in RNA-directed DNA methylation and transcriptional gene silencing in Arabidopsis.. Plant J 2010; 62:92 - 9; http://dx.doi.org/10.1111/j.1365-313X.2010.04130.x; PMID: 20059743
- Lorković ZJ, Naumann U, Matzke AJ, Matzke M. Involvement of a GHKL ATPase in RNA-Directed DNA Methylation in Arabidopsis thaliana.. Curr Biol 2012; 22:933 - 8; http://dx.doi.org/10.1016/j.cub.2012.03.061; PMID: 22560611
- Ausin I, Mockler TC, Chory J, Jacobsen SE. IDN1 and IDN2 are required for de novo DNA methylation in Arabidopsis thaliana.. Nat Struct Mol Biol 2009; 16:1328 - 30; http://dx.doi.org/10.1038/nsmb.1690; PMID: 19935683
- Xie M, Ren G, Costa-Nunes P, Pontes O, Yu B. A subgroup of SGS3-like proteins act redundantly in RNA-directed DNA methylation. Nucleic Acids Res 2012; 40:4422 - 31; http://dx.doi.org/10.1093/nar/gks034; PMID: 22302148
- Zhang CJ, Ning YQ, Zhang SW, Chen Q, Shao CR, Guo YW, et al. IDN2 and its paralogs form a complex required for RNA-directed DNA methylation. PLoS Genet 2012; 8:e1002693; http://dx.doi.org/10.1371/journal.pgen.1002693; PMID: 22570638
- Page DR, Grossniklaus U. The art and design of genetic screens: Arabidopsis thaliana.. Nat Rev Genet 2002; 3:124 - 36; http://dx.doi.org/10.1038/nrg730; PMID: 11836506
- Schubert D, Lechtenberg B, Forsbach A, Gils M, Bahadur S, Schmidt R. Silencing in Arabidopsis T-DNA transformants: the predominant role of a gene-specific RNA sensing mechanism versus position effects. Plant Cell 2004; 16:2561 - 72; http://dx.doi.org/10.1105/tpc.104.024547; PMID: 15367719
- Aufsatz W, Nehlin L, Voronin V, Schmidt A, Matzke AJ, Matzke M. A novel strategy for obtaining kanamycin resistance in Arabidopsis thaliana by silencing an endogenous gene encoding a putative chloroplast transporter. Biotechnol J 2009; 4:224 - 9; http://dx.doi.org/10.1002/biot.200800156; PMID: 19226556
- Conte S, Stevenson D, Furner I, Lloyd A. Multiple antibiotic resistance in Arabidopsis is conferred by mutations in a chloroplast-localized transport protein. Plant Physiol 2009; 151:559 - 73; http://dx.doi.org/10.1104/pp.109.143487; PMID: 19675150
- Kanno T, Mette MF, Kreil DP, Aufsatz W, Matzke M, Matzke AJ. Involvement of putative SNF2 chromatin remodeling protein DRD1 in RNA-directed DNA methylation. Curr Biol 2004; 14:801 - 5; http://dx.doi.org/10.1016/j.cub.2004.04.037; PMID: 15120073
- Kanno T, Bucher E, Daxinger L, Huettel B, Böhmdorfer G, Gregor W, et al. A structural-maintenance-of-chromosomes hinge domain-containing protein is required for RNA-directed DNA methylation. Nat Genet 2008; 40:670 - 5; http://dx.doi.org/10.1038/ng.119; PMID: 18425128
- López A, Ramírez V, García-Andrade J, Flors V, Vera P. The RNA silencing enzyme RNA polymerase v is required for plant immunity. PLoS Genet 2011; 7:e1002434; http://dx.doi.org/10.1371/journal.pgen.1002434; PMID: 22242006
- Cromie KD, Ahmad K, Malik T, Buyukuslu N, Glass RE. Trans-dominant mutations in the 3′-terminal region of the rpoB gene define highly conserved, essential residues in the beta subunit of RNA polymerase: the GEME motif. Genes Cells 1999; 4:145 - 59; http://dx.doi.org/10.1046/j.1365-2443.1999.00248.x; PMID: 10320480
- Malik T, Ahmad K, Buyukuslu N, Cromie K, Glass RE. Intragenic suppression of trans-dominant lethal substitutions in the conserved GEME motif of the beta subunit of RNA polymerase: evidence for functional cooperativity within the C-terminus. Genes Cells 1999; 4:501 - 15; http://dx.doi.org/10.1046/j.1365-2443.1999.00276.x; PMID: 10526237
- Sidorenko L, Dorweiler JE, Cigan AM, Arteaga-Vazquez M, Vyas M, Kermicle J, et al. A dominant mutation in mediator of paramutation2, one of three second-largest subunits of a plant-specific RNA polymerase, disrupts multiple siRNA silencing processes. PLoS Genet 2009; 5:e1000725; http://dx.doi.org/10.1371/journal.pgen.1000725; PMID: 19936058
- Bäurle I, Smith L, Baulcombe DC, Dean C. Widespread role for the flowering-time regulators FCA and FPA in RNA-mediated chromatin silencing. Science 2007; 318:109 - 12; http://dx.doi.org/10.1126/science.1146565; PMID: 17916737
- Johnson LM, Bostick M, Zhang X, Kraft E, Henderson I, Callis J, et al. The SRA methyl-cytosine-binding domain links DNA and histone methylation. Curr Biol 2007; 17:379 - 84; http://dx.doi.org/10.1016/j.cub.2007.01.009; PMID: 17239600
- Ausin I, Greenberg MV, Simanshu DK, Hale CJ, Vashisht AA, Simon SA, et al. INVOLVED IN DE NOVO 2-containing complex involved in RNA-directed DNA methylation in Arabidopsis.. Proc Natl Acad Sci U S A 2012; 109:8374 - 81; http://dx.doi.org/10.1073/pnas.1206638109; PMID: 22592791
- Pontier D, Yahubyan G, Vega D, Bulski A, Saez-Vasquez J, Hakimi MA, et al. Reinforcement of silencing at transposons and highly repeated sequences requires the concerted action of two distinct RNA polymerases IV in Arabidopsis.. Genes Dev 2005; 19:2030 - 40; http://dx.doi.org/10.1101/gad.348405; PMID: 16140984
- Fukunaga R, Doudna JA. dsRNA with 5′ overhangs contributes to endogenous and antiviral RNA silencing pathways in plants. EMBO J 2009; 28:545 - 55; http://dx.doi.org/10.1038/emboj.2009.2; PMID: 19165150
- Hetzl J, Foerster AM, Raidl G, Mittelsten Scheid O. CyMATE: a new tool for methylation analysis of plant genomic DNA after bisulphite sequencing. Plant J 2007; 51:526 - 36; http://dx.doi.org/10.1111/j.1365-313X.2007.03152.x; PMID: 17559516
- Pfaffl MW. A new mathematical model for relative quantification in real-time RT-PCR. Nucleic Acids Res 2001; 29:e45; http://dx.doi.org/10.1093/nar/29.9.e45; PMID: 11328886
- Mette MF, Aufsatz W, van der Winden J, Matzke MA, Matzke AJ. Transcriptional silencing and promoter methylation triggered by double-stranded RNA. EMBO J 2000; 19:5194 - 201; http://dx.doi.org/10.1093/emboj/19.19.5194; PMID: 11013221
- Mette MF, Aufsatz W, Kanno T, Daxinger L, Rovina P, Matzke M, et al. Analysis of double-stranded RNA and small RNAs involved in RNA-mediated transcriptional gene silencing. Methods Mol Biol 2005; 309:61 - 82; PMID: 15990398
- Church GM, Gilbert W. Genomic sequencing. Proc Natl Acad Sci U S A 1984; 81:1991 - 5; http://dx.doi.org/10.1073/pnas.81.7.1991; PMID: 6326095
- Bateman A. The SGS3 protein involved in PTGS finds a family. BMC Bioinformatics 2002; 3:21; PMID: 12162795