Abstract
Expression level of lens epithelial derived growth factor (LEDGF) is vital for LEDGF-mediated cell survival and cytoprotection against proapoptotic stimuli. We previously demonstrated that LEDGF is transcriptionally regulated by Sp1-responsive elements within a CpG island in the LEDGF promoter. Herein, we report on the existence of epigenetic signaling involved in the repression of LEDGF transcription in lens epithelial cells (LECs) facing UVB. UVB exposure led to histone H3 dimethylation and deacetylation at its CpG island, where a histone deacetylase/histone methylase (HDAC1/SUV39H1) complex was recruited. Exposure of LECs to UVB stress altered LEDGF protein and mRNA expression as well as promoter activity, while failing to methylate the CpG island. These events were correlated with increased reactive oxygen species (ROS) and increased cell death. LEDGF promoter activity and expression remained unaltered after 5-Aza treatment, but were relieved with tricostatin A, an inhibitor of HDACs. Expression analysis disclosed that UVB radiation altered the global expression levels of acetylated histone proteins, diminished total histone acetyltransferase (HAT) activity and increased HDAC activity and HDAC1 expression. In silico analysis of LEDGF proximal promoter and ChIP analyses disclosed HDAC1/SUV39H1 complex anchored to the -170/-10 nt promoter regions at Sp1-responsive elements and also attenuated Sp1 binding, resulting in HDAC1- and SUV39H1-dependent deacetylation and dimethylation of H3 at K9. Acetylation of H3K9 was essential for LEDGF active transcription, while enrichment of H3K9me2 at Sp1-responsive elements within CpGs (-170/-10) by UVB radiation repressed LEDGF transcription. Our study may contribute to understanding diseases associated with LEDGF aberrant expression due to specific epigenetic modifications, including blinding disorders.
Introduction
A multidomain nuclear protein, LEDGF has a variety of functions in cytoprotection and the generation of cellular abnormalities.Citation1,Citation2 LEDGF is expressed in many types of cells, including the eye lens epithelial cells (LECs). Its expression level is tightly regulated and mediates cellular survival signaling. LEDGF upregulates stress-associated gene transcription, such as the small heat shock proteins (Hsps) 27 and αB-crystallin and, thereby, maintains cellular homeostasis during various cellular and environmental stresses.Citation3,Citation4 Stress-inducible LEDGF interacts with DNA and protein to cooperatively perform its cellular functions.Citation5 Aberrant expression of LEDGF has been found in cancer cells, and its increased expression has been proven to affect tumor progression by abnormally stimulating survival signaling.Citation6 Conversely, cells lacking or expressing reduced levels of LEDGF show weaker resistance to oxidative or cellular stress.Citation2,Citation3,Citation7 Importantly, LEDGF expression is enhanced in cells which have lower prevalence of reactive oxygen species (ROS) evoked by mild UVB or H2O2 exposure, while cells exposed to these oxidants for longer periods or at higher doses repress the LEDGF transcript.Citation8,Citation9 These studies indicate that level of LEDGF expression is vital and can be governed at the transcriptional level.
LEDGF acts as a transcriptional co-activator as well as a transactivator.Citation10,Citation11 It performs various functions by interacting with protein, DNA and chromatin.Citation10,Citation12-Citation15 LEDGF is derived from a single gene and shares the first 325 amino acids with p52, an alternative splice variant mapped to chromosome 9p22.3 genetic locus.Citation3,Citation12 PWWP and A/T hook domains at the N-terminal end of LEDGF are involved in tethering of the lentiviral pre-integration complex and chromatin.Citation1,Citation15,Citation16 Moreover, during internal and external cellular stress, its helix-turn-helix (HTH)-like motifs (amino acids 421–442 and amino acids 471–492) bind to the heat shock element (HSE, nGAAn) and activate transcription of small Hsps.Citation10,Citation17,Citation18 N-terminal end of LEDGF has been found to interact with stress-related response elements (STRE, nA/TGGGGA/Tn), thereby tuning transcription in favor of cellular survival.Citation7,Citation10,Citation19 Most importantly, LEDGF binds not only to HSE or STRE, but also to markers of active chromatin as well as RNA polymerase II, and correlates with transcriptional activity of the transcriptional unit.Citation20 Recently, LEDGF was found to selectively bind to supercoiled DNA and to recruit its binding partners to active transcription units.Citation21 In addition, LEDGF also exerts biological activity by interacting with many proteins such as Myc-interacting protein JPO2Citation22 and mixed-lineage leukemia (MLL)/menin complex,Citation23 a domesticated transposase PogZ (pogo transposable element derived protein with zinc finger),Citation24 Cdc7-activator of S-phase kinase (ASK),Citation25 methyl CpG Binding Protein MeCP2Citation26 and its integrase binding domain (IBD; residues 347 to 429) to integrase.Citation1 More recently, sumoylation has been identified in LEDGF protein. This posttranslational modification negatively regulates LEDGF half-life and transcriptional activity.Citation27,Citation28 Taken together, these reports indicate a wide-spectrum activity of LEDGF and underscore its biological importance.
Oxidative stress driven by ROS accumulation induced by UVB radiation or cellular stress has been suggested as a major player in the progression of various age-associated degenerative disorders, including eye lens cataractogenesis.Citation29-Citation34 However, skin and eye are maximally exposed to the sun and, therefore, are major targets for UVB-induced insults.Citation35-Citation37 Recent evidence shows that ROS generated by internal or external environmental stresses modulate gene transcription via epigenetic reprogramming.Citation38,Citation39 Although the features and biological activity of LEDGF have been studied, little is known about how dynamic transcriptional changes of LEDGF are controlled in normal or redox cellular environment.
Recently, characterization of LEDGF gene promoter revealed the regulatory mechanisms for LEDGF gene transcription.Citation9,Citation40 LEDGF promoter region is evolutionarily conserved in different species and is devoid of TATA box and enriched with G/C bases containing three active Sp1-response elements, each of which differentially regulates LEDGF transcription.Citation9 Furthermore, we have found that all three Sp1-responsive elements were located within a CpG island present in the LEDGF promoter (current study). Based on recent studies suggesting that genes with CpG island can be under the control of epigenetic reprogramming,Citation41 we posit that the LEDGF promoter containing CpG island may be a target for chromatin remodeling during stress (current study). In recent years, significant progress has been achieved in understanding the functional importance of histone methylation in regulating genome organization.Citation42,Citation43 In general, methylation of H3K4, H3K36, and H3K79 correlates primarily with transcriptionally active chromatin, whereas methylation of H3K9 and H3K27, as well as H4K20, is associated with transcription silencing.Citation42,Citation44-Citation48 Furthermore, there is functional evidenced that H4K20 plays an important role in DNA repair and thereby maintains the genome integrity.Citation48,Citation49 Moreover, acetylation of histones, which is processed by histone acetyltransferase enzymes (HATs) at lysine residues, is generally associated with active gene transcription. This process can be reversed by histone deacetylases (HDACs).Citation50 Previously, we have shown that Sp1 specifically and differentially regulates human LEDGF gene transcription by directly binding to Sp1 sites present within the CpG island of LEDGF to regulate its transcription.Citation9 Additionally, we reported that Sp1 overexpression increases LEDGF mRNA and protein expression in cells, and these cells gain resistance against UVB,Citation9 a known inducer of etiopathogenesis-induced injury, including cataractogenesis.
Given the regulatory role of environmental factors in epigenetic reprogramming, we sought to elucidate the UVB-induced epigenetic mechanisms involved in the modulation of LEDGF transcription. Using LECs facing UVB stress as a model system (since eye are maximally exposed to sunlight), we showed that LEDGF gene transcription is controlled through chromatin modification via epigenetic alterations. LEDGF mRNA levels, along with regional and global levels of H3K9me2, H3K9ac and cell viability, are altered during UV stress, but the CpG island in LEDGF is not methylated. We provide evidence that UVB-induced silencing of LEDGF expression is caused by a coordinated effect of histone modifications, H3K9me2 and histone deacetylation at Sp1 binding sites within the CpG island of the LEDGF promoter. This process also diminished the interaction of Sp1 to Sp1-responsive elements. Uncovering the link between UVB stress and epigenetic mechanisms involved in silencing gene transcription will allow the formulation of efficient strategies to prevent UVB-induced etiopathology.
Results
LECs exposed to UVB radiation showed decreased viability and increased ROS expression; LEDGF expression was critical for cell survival
Given the injurious effect of UVB stress on cells/tissuesCitation7 and the protective effect of LEDGF,Citation19 we first determined the effect of UVB exposure on the viability of LECs over- or under-expressing LEDGF. In cells exposed to UVB (40, 80 and 100 J/m2), as shown in , MTS assay revealed a marked reduction in cell survival (), which was dependent on the dose of UVB radiation. Quantitation of ROS by H2DCF fluorescent dye demonstrated increases in ROS levels (), which were correlated with cell viability.
Figure 1. LECs require LEDGF expression for resistance against UVB-induced damage. (A) UVB radiation caused reduced hLEC viability. Cells were exposed to UVB at different doses (40, 80 and 100 J/m2). After 24h, cell viability was determined by MTS assay. (B) Intracellular ROS were measured at 24h following UVB exposure by replacing the medium with Hank's medium containing 10 μM H2-DCF-DA at Ex485/Em530 nm. (C) LEDGF expression affected cell viability after UVB exposure. MTS assay was performed to examine viability of cells under- or overexpressing LEDGF after UVB exposure (40, 80 and 100 J/m2). When LEDGF was depleted in hLECs by Si-LEDGF, the cells became more vulnerable to UVB-induced damage, while LECs overexpressing LEDGF had resistance against UVB stress. Transfection efficiency was normalized with GFP OD values measured at ex485/em530. Values are mean ± SEM of three independent experiments. Asterisks indicate statistically significant difference (p < 0.001 vs. control). (D) Representative Western analysis for LEDGF protein to show effective silencing of LEDGF in hLECs with specific siRNA strategy. (E) Diagrammatic representation of UVB stress schedule conducted in the study.
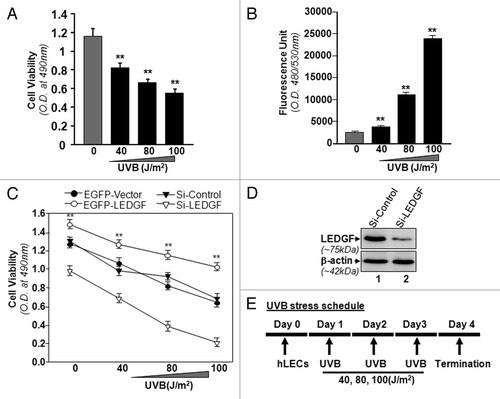
Our previous studies had shown that LEDGF is a stress-responsive gene and its overexpression is cytoprotective against internal and external cellular stresses.Citation2,Citation7,Citation19 In the present study, we tested whether cells expressing reduced levels of LEDGF were more susceptible to UVB radiation. We knocked down LEDGF by using siRNA specific LEDGF (Si-LEDGF, ). Results revealed that depletion of LEDGF caused a significant decrease in cell viability compared with scrambled siRNA (Si-Control) transfected cells () facing UVB-induced oxidative stress. In another set of experiments, we overexpressed LEDGF (pEGFP-LEDGF) in cells and then exposed them to UVB radiation (40, 80 and 100 J/m2). The survival rate was significantly higher in these cells compared with empty vector (pEGFP-Vector) transfected cells (). As a whole, the data demonstrated that LEDGF expression is crucial for the resistance against UVB-induced cellular injury.
UVB radiation modulated LEDGF expression in LECs in dose- and exposure-dependent fashion
To test the hypothesis that UVB-induced repression of LEDGF expression in LECs is a possible cause of reduced cell viability, we exposed LECs to variable doses of UVB radiation for single or multiple time periods as described in the “Materials and Methods” section and shown in . We found that, in hLECs exposed only once, the expression level of LEDGF mRNA was significantly increased at J/m2. (), but the level was significantly reduced in LECs exposed to 100 J/m2.
Figure 2. Effect of single or multiple doses of UVB exposure on the expression of LEDGF mRNA. (A) Single exposures to variable doses of UVB differentially enhanced LEDGF expression in LECs. Cultured cells were exposed one time to different doses of UVB radiation as shown. Real-time PCR analysis was performed with mRNA isolated from hLECs, and expression of LEDGF mRNA was normalized with β-actin. Values are mean ± SD of three independent experiments. Asterisks indicate statistically significant difference (p < 0.001 vs. control). (B) Multiple high doses (80 and 100 J/m2) of UVB exposure suppressed the LEDGF mRNA expression. Real-time PCR analysis was performed with mRNA isolated from hLECs after multiple exposures to UVB (three times at 24h intervals) . Values are mean ± SD of three independent experiments. Asterisks indicate statistically significant difference (p < 0.001 vs. control). (C) LECs exposed to multiple high doses (80 and 100 J/m2) of UVB radiation displayed reduced LEDGF protein expression. Cells were either unexposed or exposed to UVB radiation three times at variable doses at 24h intervals. After 96h, nuclear extract was isolated, resolved onto SDS-GEL and immunoblotted using antibody specific to LEDGF. The membrane was striped or restriped and reprobed with β-actin antibody for internal/loading assessment.
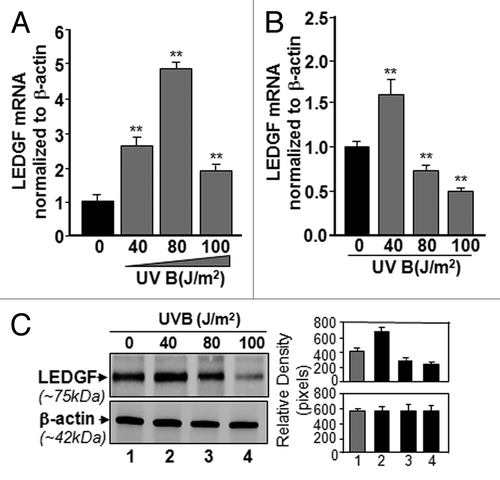
Next, we examined the effect of multiple exposures to UVB radiation on LEDGF expression in LECS. Cultured cells were exposed to different doses of UVB radiation three times at intervals of 24h. Following incubation for 24h of last UVB exposure, total RNA was isolated and subjected to real-time PCR using probes specific to LEDGF. The multiple exposures to UVB (doses of 80 and 100 J/m2) significantly decreased LEDGF expression (). In a parallel experiment, cellular extracts were prepared and immunoblotted using antibody specific to LEDGF. Expression of LEDGF protein was reduced, and lower levels of LEDGF protein were associated with reduction in its transcript (). Conversely, expression in internal control β-actin was not altered, suggesting that modulation in LEDGF expression in LECs exposed to UVB was selective and specific. Expression analysis indicated that UVB exposure modulated LEDGF expression in dose- and exposure-dependent fashion.
Derepression of LEDGF promoter by TSA, an HDAC inhibitor, suggested that LEDGF promoter was epigenetically regulated during UVB exposure
We previously showed that human LEDGF expression is differentially regulated by GC-box elements,Citation9 that direct binding of Sp1 is essential to these sites, and that Sp1 is essential for LEDGF transcription. Environmental stresses, including UVB or UVB-induced ROS-driven stress, causes epigenetic reprogramming.Citation51 Given the location of all Sp1-responive elements within the CpG island in the proximal promoter, and given the fact that CpG islands are known to be frequent targets for epigenetic modification and transcription silencing,Citation52-Citation55 we posit that aberrant epigenetic programming/reprogramming may be involved in the regulation/repression of LEDGF transcription in LECs or LECs facing UVB exposure. First, we examined whether the HDAC inhibitor TSA or the DNA demethylating agent 5-AzaC modulated transcriptional activity of the LEDGF promoter. Cells were transfected with LEDGF promoter ranging from -170/+35 nt containing all Sp1 sites within the CpG island. Transfectants were either not treated or treated with TSA or 5-AzaC, and subjected three times to different doses of UVB exposure. demonstrates that activity of LEDGF promoter containing the Sp1 site within the CpG region can be derepressed in LECs or LECs facing UVB radiation by TSA treatment, but not by 5-AzaC, suggesting involvement of histone modification in LEDGF regulation (, middle panel). In a parallel experiment, cells treated with 5-AzaC failed to release promoter activity, ruling out the role of DNA methylation, at least with promoter spanning from -170/+35 containing the CpG island. Since the promoter activity assay is highly sensitive, cells given multiple exposures to UVB were exposed only two times at 24h intervals and, after 48h, CAT activity was determined. Taken together, the data indicated a decrease in promoter activity in cells exposed to 80 and 100 J/m2 radiation (), while promoter activity was relieved in cells treated with TSA, suggesting the involvement of epigenetic control and, specifically, histone modification at the LEDGF promoter in LECs or LECs exposed to UVB radiation.
Figure 3. TSA, an HDAC inhibitor, was able to relieve repressed LEDGF promoter-driven CAT activity in LECs or LECs facing multiple UVB exposures, but 5-AzaC was not. Promoter activity assay was performed by co-transfecting LECs with LEDGF-CAT reporter plasmid (-170/+35bp) or empty CAT vector along with pSEAP vector. Transfectants were exposed to variable doses of UVB radiation as shown. Cells were pretreated as follows: (A) untreated control; (B) TSA treated; (C) 5-AzaC treated, followed by UVB. After 48h cell lysate was prepared to measure CAT activity. Transfection efficiencies were normalized with protein concentration and SEAP values (OD; ex/em, 360/449). Values are mean ± SD of three independent experiments. Asterisks indicate statistically significant difference (p < 0.001 vs. control).
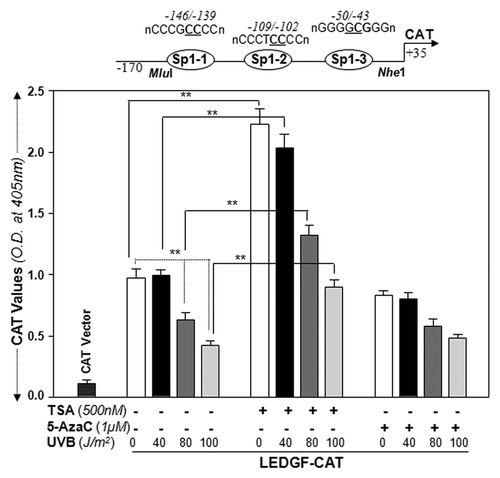
Multiple UVB exposures elevated the expression of DNA methyltransferase DNMT3b but did not affect the unmethylated status of the CpG island in the LEDGF promoter
Transcription and expression of LEDGF are decreased by UVB stress, indicating that the oxidative stress generated may affect gene promoter level. UV-B is known to induce ROS, and high oxidative stress because of ROS accumulation can epigenetically modify the core promoter regions of genes.Citation38,Citation56 We hypothesized that UVB may alter the critical promoter region through epigenetic reprogramming. Data shown in , albeit, argued that the LEDGF promoter was not methylated during UVB-induced stress, as 5-Aza did not alter the LEDGF promoter activity. However, we wanted to confirm that, indeed, LEDGF promoter was not methylated at CpG containing Sp1 sites. In mammals, there are three major DNA methyltransferase (DNMT) isoforms: DNMT1, DNMT3a and DNMT3b, and all have been found to be responsible for DNA methylation.Citation57 We performed Western Blot analysis to assess the levels of DNMT expression in cells exposed to UVB radiation. Nuclear extract isolated from cells exposed to UVB three times at 24h intervals was immunoblotted. The expression levels of DNMT1 and DNMT3a were unchanged. Strikingly, DNMT3b expression was found to be upregulated (), but that did not appear to affect LEDGF promoter activity, as evidenced by the 5-AzaC treatment ().
Figure 4. LECs exposed to UVB displayed enhanced expression of DNA methyl transferase DNMT3b, but could not methylate LEDGF promoter. (A) Nuclear extract from UVB exposed hLECs were resolved on 4–20% SDS gel and immunoblotted using anti-DNA methyl transferases as shown. The same membranes were used to re-probe the antibodies following restriping. Protein band appearing by β-actin antibody served as internal/loading control. Right panel shows relative densitometry of protein bands. (B) MSP analysis disclosed that status of LEDGF promoter (-175/+27) containing Sp1 sites within the CpG island was not altered. Top panel shows the diagrammatic representation of the LEDGF promoter, predicted CpG island and regions where the PCR primers bind. Lower panel shows the representative gel image from MSP analysis of genomic DNA isolated from LECs exposed to variable doses of UVB-exposed or unexposed to UVB.
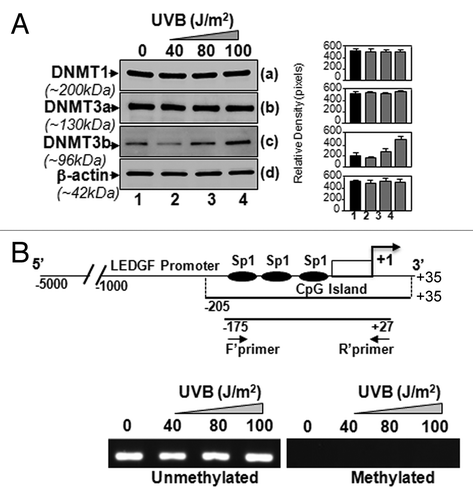
Next, we analyzed whether the increased expression of DNMT3b and decreased transcription of LEDGF were associated with its promoter methylation. In a previous study, we showed that the 5′-flanking region sequence of the human LEDGF gene is a TATA-less promoter, and minimal promoter (-170/+10) is enriched with GC content containing three active Sp1-responsive elements.Citation9 In the current study, through in silico analysis, we spotted a major CpG island in the LEDGF promoter (-205/+35), including all three active Sp1 binding sites, specifically within -170/+10-bp. We hypothesized that changes in these Sp1 cis-elements within the CpG island can alter the expression of the LEDGF gene. We explored the DNA methylation of GC-rich Sp1 cis-elements of the LEDGF promoter after multiple UVB exposures, and performed methylation-specific PCR. We designed the MSP primers for specific regions (, upper panel) to amplify the region of interest after modifying genomic DNA with sodium bisulfite. As shown in (lower panel), we did not detect any methylation at the CpG island of Sp1 binding sites of the LEDGF promoter after UVB exposure in hLECs. Collectively, the results revealed that UVB radiation did not alter the expression level of LEDGF through DNA methylation.
UVB radiation-modulated histone acetylation status and reduced acetylation were due to diminished activity of HAT and increased activity of HDACs
Since our DNA-methylation results revealed that the LEDGF promoter-containing CpG island was not methylated during UV exposure, we postulated that histone modifications may be involved in the regulation of LEDGF transcription, as indicated by data obtained from . We examined histone acetylation status, as it is known that histone deacetylation can repress gene expression, affecting cellular homeostasis.Citation58-Citation60 Total chromatin was prepared and immunoblotted as described in “Materials and Methods.” Expression assay detection of core histone proteins in cells with multiple UVB exposures showed modulation in the expression of posttranslational modifications (). Additionally, the data disclosed an increase in the expression level of HDAC1 in these cells (). This was accompanied by a substantial decrease in the levels of acetylated histone H3 (acetyl-H3) () and H2B (). No detectable change in the expression levels of histones H3 () and H2B () protein was noticed between the UVB-treated and untreated control.
Figure 5. UVB-induced differential expression of posttranslationally modified acetyl-H3 and H2B was associated with decreased total HAT activity. (A) UVB-exposed and unexposed LECs were harvested and processed for nuclear protein extraction. Extract was resolved through 4–20% SDS-PAGE and immunoblotted with anti-acetyl histone or anti-histone antibodies as shown. (B) Total HAT activity was measured after multiple UVB exposures following the company’s protocol (Epigentek), and data were compared with results from untreated samples. The data represent mean ± SEM of three independent experiments. Asterisks indicate statistically significant difference (p < 0.001 vs. corresponding control).
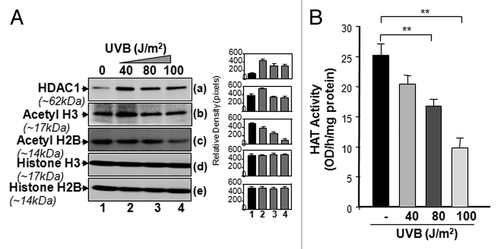
Next, we assessed the effect of UVB exposure on the activity of histone acetylation/deacetylation enzymes. Acetylation of histone status is regulated by the opposing actions of HAT and HDAC enzymes. Considering the UVB-induced altered expression of acetyl-H3, H2B and HDAC1, we evaluated the total HAT and HDAC1 activity in UVB-treated samples. Nuclear extracts were isolated from cultured LECs exposed to multiple doses of UVB radiation and were processed to assess HAT or HDAC1 activity. UVB-treated cells showed a marked decrease in total HAT activity (), but activity of HDAC1 was significantly increased (data not shown) compared with control vehicle. The changes in HAT or HDAC1 activity were dependent upon the dose of UVB radiation applied.
In vivo DNA-protein binding assay demonstrated diminished Sp1 binding to LEDGF promoter during UVB stress
To delineate the cause of repression of LEDGF expression during UVB radiation, we performed ChIP assays with Sp1 antibody as described earlier.Citation9 Chromatin samples were prepared from LECs exposed to UVB multiple times. Protein-DNA complex was pulled down with a ChIP grade antibody or with control IgG. Immunoprecipitated complex was processed for PCR using the primers that encompassed LEDGF promoter consisting of Sp1-responsive elements (, top panel). Data revealed a significant decrease in the interaction of Sp1 with its target elements at LEDGF promoter in UVB treated samples (; left panel, B). This experiment demonstrated that reduced expression of LEDGF transcript during high oxidative stress induced by multiple doses of UVB radiation may be associated with decreased interaction of Sp1 with its target elements.
UVB stress-induced deacetylation of histone H3 and enrichment of HDAC1at the Sp1-responsive element-containing CpG island of LEDGF promoter
The above data demonstrated that Sp1-DNA interactions were reduced and that cells displayed reduced levels of LEDGF expression during UVB radiation (). CpG island are mostly located in the regulatory region of gene promoters.Citation61 Based on current findings and literature showing that acetylation and deacetylation of histones are the hallmark of relaxed and compressed chromatin for active and inactive transcription, respectively, we envisaged the probability of alterations in histone code at Sp1 sites within the CpG island, which led to blocking of Sp1 access to DNA during UVB stress. To explore the relationship between the Sp1 site within the CpG island and H3 acetylation status, we investigated the level of histone acetylation by ChIP assay using anti-acetyl-H3, anti-acetyl-H2B and HDAC1 antibodies. We selected these antibodies based on protein expression analysis. In addition, our laboratory and others have tested the specificity of antibodies used for chromatin immunoprecipitation. Sp1 binding sites at the LEDGF promoter were amplified using region-specific primers as shown in . ChIP experiments disclosed that, in LECs exposed to UVB radiation, prevalence of both acetylated histones tested, acetyl-H3 and acetyl-H2B was significantly reduced compared with control samples not exposed to UVB (). To examine whether HDAC1 is also recruited to the site, we further performed ChIP assay with antibody specific to HDAC1. (left panel) shows that HDAC1 was recruited at the Sp1 site in the CpG island . Collectively, these data indicate that Sp1 binding sites or regions are under dynamical epigenetic reprogramming (deacetylation of histones) in cells facing UVB stress for longer periods.
Figure 6. ChIP analyses revealing reduced binding of Sp1 and the status of histone acetylation associated with LEDGF promoter. Left panel shows Sp1 binding to its responsive cis-elements (B), association and recruitment of site-specifically modified H3 (C), H2B (D), and HDAC1(E) to the Sp1 binding sites within CpG island of LEDGF gene promoter in LECs exposed or unexposed to UVB radiation. Right panel represents control; DNA selected beyond Sp1 binding sites is shown. ChIP assay was performed. Also, amplification of chromatin extract before precipitation is shown as control (input, A)
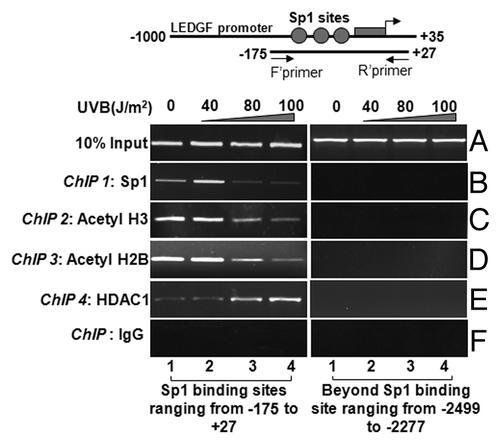
UVB exposure enhanced expression of SUV39H1 and methylated histones, and induced recruitment of the histone methyltransferase SUV39H1 and H3K9Me2 at and around Sp1-responsive elements in the CpG island of LEDGF promoter
To clarify the contribution of histone methylation, if any, on the repression of LEDGF expression during UVB exposure, we first examined global expression levels of histone methylase SUV39H1, the best characterized histone H3 methyltransferase, which specifically methylates lysine 9 of histone H3. We found that, indeed, SUV39H1 was increased in LECs exposed to UVB radiation compared with control, and the increase was dependent on the dose of UVB (). Next, we probed and re-probed membrane with antibodies specific to H3K9me2 or H3K9me3, following stripping and re-stripping of the membrane. We observed that the global expression levels of H3K9me2 () and H3K9me3 () considerably increased in UVB-treated cells compared with untreated control. Taken together, the data revealed that deacetylation in H3 and H2B and di- or tri-methylation of H3K9 were the most significantly altered epigenetic mark after UVB treatment, at least in LECs.
Next, we pursued ChIP analyses to elucidate if histone H3K9me2, H3K9me3 or SUV39H1 were enriched/recruited to Sp1 site-containing CpG island . We posited that noticeable upregulation of SUV39H1 (), a histone methylase enzyme that di- or tri-methylates histones (), may be synergistically involved with deacetylation reprogramming in LEDGF repression. We performed ChIP analyses using SUV39H1 antibody. As expected, SUV39H1 was recruited to the Sp1 binding regions of the LEDGF promoter in cells exposed to UVB radiation (); the level of SUV39H1 was dependent upon the dose of UVB radiation. SUV39H1 specifically methylated H3K9.
Di- or tri-methylation of H3K9 has been shown to be associated with transcription repression.Citation62-Citation64 To explore whether H3K9 was di- or tri-methylated and limited to Sp1 site-containing CpG region, we performed ChIP assay with antibodies specific to methylated histone H3. Histone H3 was found to be dimethylated at K9 during UVB radiation () and located within the CpG island containing Sp1 sites. We did not detect any bands after immunoprecipitation using anti-H3K9me3 antibody () or in control IgG (), indicating that UVB specifically induced H3K9me2.
Based upon our data demonstrating the interruption in Sp1 interaction with its responsive elements and the deacetylation of histones coupled with H3K9me2 at or around Sp1 sites within the CpG island, resulting in the transcription repression during UVB radiation, it seems likely that aberrant epigenetic reprogramming plays a role in LEDGF repression.
Discussion
LEDGF expression is essential for maintenance of cellular integrity. Unusually, high expression of LEDGF promotes cell survival, and may initiate cellular abnormalities or promote tumor formationCitation6,Citation65,Citation66. Low expression leads to negative regulation of survival signaling. Recent studies have investigated various features of LEDGF protein, regulation of its gene and its role in cancer and HIV. These studies found that the expression level of LEDGF and its interaction with DNA/chromatin and/or proteins are vital for its biological activities.Citation9 We recently showed that LEDGF gene is regulated by Sp1-response elements in LEDGF promoter, and that the gene is further controlled by sumoylation of Sp1.Citation9 This indicates that LEDGF expression is tightly controlled, and that the cellular nucleus coordinates expression in favor of cell survival. However, no studies have been performed to explore the possible role of epigenetics in regulating LEDGF expression. Currently, during bioinformatics analysis of LEDGF promoter, we observed that the proximal promoter of LEDGF contained a CpG island, located -205 from transcription start site (TSS), that, strikingly, encompassed the three Sp1 sites (current study and Singh et al., 2012).Citation9 It is now widely recognized that CpG sites function as tethering sites for chromatin-modifying proteins and the enrichment of histone modification, which ultimately regulate gene expression.Citation67-Citation69 We posited that the CpG island present in the LEDGF promoter may provide a platform for LEDGF epigenetic regulation, which may selectively minimize transcription of LEDGF by restricting access of transcription factor Sp1 to its binding site.
In the present work, we sought to elucidate the role of epigenetic modifications specifically around the CpG island site in human LEDGF promoter. We investigated the effect of histone modification on the modulation of LEDGF expression in cells under normal physiological conditions and cells exposed to UVB. As eyes are frequently exposed to sunlight, and UVB is known to initiate and accelerate cataractogenesis,Citation34,Citation35 UVB and LECs served as a model system for exploring how LEDGF expression might be epigenetically regulated. Because LEDGF is a survival factor, we first studied its ability to protect cells against UVB radiation. Experimentation revealed that loss of cellular LEDGF led to cell death from UVB stress, and such cells expressed higher levels of ROS (). Environmental stress is known to influence chromatin structure,Citation70 including DNA methylation and histone modifications, acetylation/deacetylation, and mono-, di-, or tri-methylation. These dynamic modifications repress or de-repress gene transcription.Citation44,Citation71,Citation72 We found that, in cells facing UVB exposure, LEDGF expression was under the control of the transcriptional machinery. It was intriguing to observe that the level of LEDGF transcript was increased in cells exposed only once to UVB at lower doses, while cells with multiple exposures (beyond 40J/m2) showed significantly lower LEDGF mRNA level and protein expression (). ROS generation induced by environmental stressors above threshold levels is known to lead to aberrant epigenetic reprogramming.Citation38,Citation56 We think that the increased expression of LEDGF at mild doses of UVB may be a feedback survival mechanism to prevent cell death, while greater exposure to UVB radiation may promote epigenetic reprogramming that leads to suppression of LEDGF expression. Notably, LEDGF mRNA declined in LECs facing higher doses or more exposures to UVB radiation (), indicating that LEDGF expression is transcriptionally altered. Based on the literature, we envisage that this may be controlled by epigenetic reprogramming, and transcription is most likely turned off or on as a result of UVB stress that alters DNA methylation status at CpG or though histone acetylation. In the current work, we found regulation of LEDGF promoter by histone modification; DNA methylation at CpG sites did not occur during UVB exposure ( and ). We base this conclusion on the observation that treatment with TSA, an HDAC inhibitor, released the repression of LEDGF promoter activity, while treatment with 5-AzaC, a DNA demethylating agent, did not affect promoter activity. Histone modification at the CpG island containing Sp1 sites appears to be independent of DNA methylation, and histone reprogramming at the CpG region does not appear to be influenced by methylation or demethylation of DNA. Furthermore, UVB radiation did not affect the status of the CpG island, suggesting that a “histone code” formed at or within the CpG region is responsible for the magnitude of LEDGF expression.
We also confirmed the methylation status of the CpG region containing all Sp1 sites by MSP analysis(). This clarified that, indeed, the CpG island containing Sp1-responsive elements (ranging from -175/+27) was not methylated, even in LECs exposed to UVB (). Surprisingly, our expression assay revealed that the expression level of DNA methyltransferase, DNMT3b was upregulated () but failed to alter the status of the CpG island in the LEDGF promoter. DNMT3b, unlike DNMT1, is known to act in de novo methylation, targeting unmethylated CpG sites.Citation73
However, based upon our data showing that the CpG island is not methylated, and because CpGs serve as a platform for histone modification, we surmised that deacetylation and/or methylation of histones at the LEDGF CpG site ultimately controls LEDGF expression in the cellular background. To explore this possibility, we assessed the expression levels of HDAC1 along with acetyl-H3 and acetyl-H2B and found that expression levels of HDAC1 were significantly increased, as were the levels of acetylated histone H3 and H2B. This was directly related to decreased activity of HAT () and increased activity of HDAC1 (data not shown). HDAC1 was recruited at Sp1 sites within the CpG island and appears to promote the deacetylation of histones, as evidenced by our ChIP experiment in cells exposed to multiple higher doses of UVB radiation (). Interestingly, the in vivo DNA binding assay on -170 bp LEDGF promoter showed that Sp1 binding to its responsive elements was significantly reduced (), suggesting that Sp1 access was hindered by deacetylation of histones at Sp1 sites within the CpG island. We recognize that Sp1 may interact with other cofactors in inhibiting Sp1 binding to its sites, and we assume the existence or co-occurrence of other chromatin repressive mechanisms, possibilities that require further investigation. However, we found that Sp1 binding was reduced, as evidenced by ChIP analyses. Previously, we conclusively demonstrated that LEDGF is regulated by Sp1, which directly and functionally binds to the response elements present in the LEDGF promoter. We believe that one cause for suppression of LEDGF expression during chronic exposure to UVB is interference with Sp1 access to chromatin due to histone deacetylation. Such epigenetic reprogramming may be one action related to UVB-induced aberrant signaling that plays a role in repressing LEDGF transcription via interrupting Sp1 interaction with the LEDGF promoter. A major regulatory signals affecting chromatin structure and, hence, gene activity is dictated by posttranslational modifications, such as methylation, acetylation, phosphorylation, sumoylation of histones. The states of chromatin modifications may be changed due to stimuli within the cellular microenvironment, and that may lead to the different transcriptional states that result from different combinations of histone modifications, which may be stable and heritable. We think that this epigenetic reprogramming may be in favor of cellular survival or vice versa, depending upon intensity or the nature of the regulatory signaling evoked. Furthermore, we observed that multiple exposure of UVB decreased total HAT activity and facilitated depletion of acetylated histones H3 and H2B, which should be due to the interaction of HDAC1 to the Sp1 binding sites (active spots of Sp1 at LEDGF promoter). Due to these UVB-induced changes at the CpG region containing Sp1 sites, we have examined the reduced binding of Sp1 to its target sites, which may involve heterochromatization of the active region of the LEDGF promoter by deacetylation, resulting in repression of LEDGF transcription.
Furthermore, histones have long been known to be substrates for methylation.Citation74 Early studies using metabolic labeling followed by sequencing of bulk histones showed that several lysine residues, including lysines 4, 9, 27 and 36 of H3 and lysine 20 of H4 are preferred sites of methylation. In addition, members of the protein arginine methyltransferase family can methylate histones in vitro.Citation75 However, direct evidence linking histone methylation to gene activity was not available until recently. One major obstacle in studying the function of histone methylation is the lack of information regarding the responsible enzymes. Recent demonstration that the human homolog of the Drosophila heterochromatic protein Su(var)3–9 is an H3-specific lysine methyltransferase provided substantial evidence for the involvement of histone methylation on transcriptional regulation. In the present study, SUV39H1 was also recruited at the Sp1 binding sites present within the CpG island of the LEDGF promoter in UVB-exposed cells, as disclosed by ChIP analyses (). Our work demonstrates that histone H3 at this region are modified through dimethylation of the K9 residue, possibly initiated by the interaction of SUV39H1 with the LEDGF promoter. H3K9 methylation has been found to occur within or around the CpG island. In the current study, H3K9me2 appears to have occurred within the CpG island around the Sp1-responsive elements, causing LEDGF silencing during UVB exposure. Our data could not reveal precisely the precise location or whether other cofactor(s) are recruited to the site, which may be involved in controlling LEDGF expression. Further work is necessary to determine whether protein-protein interactions are involved in the process. However, we found that deacetylation of histones H3 and H2B over Sp1-responsive elements within the CpG island, where histone deacetylase HDAC1 was recruited, controlled the magnitude of LEDGF transcription in cells. In addition, repression of LEDGF transcription during UVB radiation could be the result of histone deacetylation and dimethylation, which interfered with the access of Sp1 to chromatin. Further studies are needed to uncover other epigenetic-associated molecules involved in regulating LEDGF.
Figure 7. Sp1 binding sites within CpG island in LEDGF promoter were enriched with SUV39H1 and dimethylated histones in LECs. (A) Western analysis of SUV39H1, H3K9me2, and H3K9me3 in the extract isolated from LECs exposed to UVB radiation. Right panel shows the relative densitometry of protein bands. (B) ChIP analyses revealed recruitment of histone methyltransferase SUV39H1 and enrichment of H3K9me2 at CpG island containing Sp1 sites. Chromatin immunoprecipitation analysis was performed using chromatin extract from LECs exposed to multiple doses of UVB radiation as shown in the scheme () with specific antibodies, as indicated. Top panel shows LEDGF promoter and location of PCR primers designed for the study. DNA fragments present in the immunoprecipitates were amplified with primers that specifically recognize a fragment of the LEDGF promoter containing Sp1 binding sites (-175 to +27). As a negative control, primers designed beyond the Sp1 sites (-2499 to -2277) or a mock ChIP with control IgG was used. PCR products were separated on 2.5% agarose gels and stained with ethidium bromide.
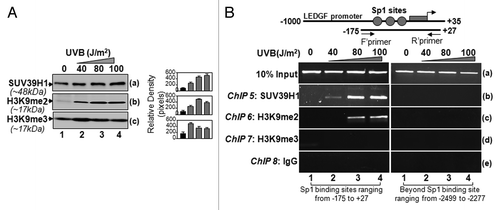
In conclusion, our current work demonstrates conclusively that LEDGF transcription is epigenetically regulated and that chromatin remodeling occurs in LECs exposed to UVB radiation, leading to LEDGF repression. H3K9me2 and histone deacetylation are key events involved in controlling LEDGF expression, regardless of DNA methylation status at the CpG island of the LEDGF promoter. Identification of the LEDGF promoter and a plausible mechanism of promoter regulation may be the foundation for further studies on how various environmental and cellular factors affect LEDGF expression in normal and disease states.
Materials and Methods
Cell Culture
Human lens epithelial cells (hLECs) (a gift of Dr V. N. Reddy, Eye Research Institute, Oakland University, Rochester, MI) were maintained routinely in our laboratory. Briefly, cells were cultured in a 75-mm tissue culture flask in Dulbecco’s Modified Eagle’s Medium (DMEM) supplemented with 15% heat-inactivated fetal bovine serum (FBS), 100μg/ml streptomycin, and 100μg/ml penicillin in a 5% CO2 environment at 37°C following standard methods. Cells were harvested and cultured in 96, 24, 48 or 6 well plates and 100 mm Petri dishes and exposed to UVB radiation according to the requirements of the experiment.
Assay for intracellular redox state
Intracellular redox state levels were measured using the fluorescent dye, H2-DCF-DA as described earlier.Citation76,Citation77 Cells (1 × 104) were cultured in 96-well plates and were subjected to single or multiple exposure of variable doses of UVB (40, 80 and 100 J/m2). Cells were washed once with HBSS and incubated in the same buffer containing 10μM of H2-DCF-DA. This is a nonpolar compound that is converted into a polar derivative (dichlorofluorescein) by cellular esterase following incorporation into cells. Following 30min of incubation at room temperature, intracellular fluorescence was detected with excitation at 485nm and emission at 530nm (Ex485/Em530) using Spectra Max Gemini EM (Molecular Devices).
Cell survival assay (MTS assay)
A colorimetric MTS assay (Promega, Cat. no. G3582) was performed as described earlier. This assay of cellular proliferation uses 3-(4, 5-dimethylthiazol-2-yl)-5-(3-carboxymethoxyphenyl)-2 to 4-sulfophenyl)-2H-tetrazolium salt. Upon being added to medium containing viable cells, MTS is reduced to a water-soluble formazan salt. The O.D. 490 nm values were measured after 4 h with an ELISA reader.
Real-time PCR
Cells were exposed or unexposed to single or multiple times (three time every 24h of interval) of different doses UVB radiation. These cells were further incubated for 24h following UVB. Total RNA was isolated by using Trizol reagent (Invitrogen, Cat. no. 15596–026) and was converted to cDNA using Superscript II RNAase H-Reverse Transcriptase. Quantitative real-time PCR was performed with SYBR Green Master Mix (Roche Diagnostic Corporation, Cat. no. 04707516001) in a Roche® LC480 Sequence detector system (Roche Diagnostic Corporation). The comparative Cp method was used to calculate relative fold expression levels using Lightcycler® 480 software, release 1.5.0 SP3. The Cps of target genes was normalized to β-actin as an endogenous control in each group. PCR conditions consisted of 10 min hot start at 95°C, followed by 45 cycles of 10s at 95°C, 30s at 60°C, and 10s at 72°C. Primer sequence as follows: LEDGF: Forward primer: 5′-CAGCAACAGCATCTGTTAATCTAAA-3′ and Reverse primer: 5′-GGGCTGTTTTACCATTTTGG-3′.
Small Interfering RNA (siRNA) Assay
The LEDGF-specific small interfering (si)RNA expression plasmid was designed according to the method described earlier.Citation78 The sequence was selected from location 1340–1360 (5′-AAAGACAGCATGAGGAAGCGA-3′). The sense and antisense oligonucleotides with the internal loop were synthesized by Invitrogen. These were annealed and ligated into the BamHI and HindIII sites of pSilencer 4.1-CMV hygro (Ambion, Cat. no. AM5777) pSilencer 4.1- pCMVhygro expressing a scrambled siRNA (Ambion, Cat. no. AM5777) was used as a control. siRNA constructs were transfected into hLECs with Neon transfection systems (Invitrogen). 24h of post transfection, stable transfected cells were selected using 400μg/ml of hygromycin (Invitrogen, Cat. no. 10687-010) over a period of 9 d. Silencing was confirmed by LEDGF protein expression.
Preparation of LEDGF promoter-CAT construct
The genomic human phagemid P1 clone (Genomic System) was used to construct 5′ flanking region of human LEDGF gene as reported previously.Citation18 The genomic P1 clone comprising the LEDGF gene was subjected to Genomic PCR with primers containing Mlu I and Nhe I, and a fragment encompassing -170 to +35 bp was ligated to basic pCAT vector (Promega, Cat. no. E1871) with the appropriate restriction enzymes as reported earlier.Citation18 The plasmid was amplified by transforming into bacteria (Top 10, Invitrogen, Cat. no. C4040-06) using the standard method, sequenced, and was used for CAT assay.
Transfection and chloramphenicol acetyltransferase assay (CAT Assay)
CAT assay was performed using a CAT-ELISA (Roche Diagnostics) kit as described earlier.Citation5,Citation9 Briefly, hLECs were cultured at a density of 5 × 105 cells in 5 ml of DMEM containing 15% FBS per 60-mm Petri dish in a 37°C incubator containing 5% CO2. Cells were washed with the same medium and transfected/cotransfected with Neon transfection system (Invitrogen) with promoter/CAT reporter constructs along with 1μg of pSEAP vector.Citation5 After 24 h of incubation, cells were subjected to multiple UVB radiation and extract was prepared and protein concentration was normalized. CAT-ELISA was performed to monitor CAT activity. The absorbance was measured at 405 nm using a micro titer plate ELISA reader. The concentration of plasmid DNA was equal in each transfection to maintain the similar DNA burden on cells and to avoid any nonspecific effect(s). Transactivation activities were adjusted for transfection efficiencies using SEAP values (OD; ex/em, 360/449).
Preparation of lens epithelial cell nuclear extract
Human LEC nuclear extract was prepared as described earlier.Citation18,Citation79 Briefly, human LECs (1 × 106) were cultured in 100-mm plates and subjected to single or multiple exposure of variable doses of UVB radiation. Twenty-four h after the last exposure of UVB radiation, the cells were washed gently with chilled phosphate-buffered saline (pH 7.2). Cells were collected by centrifugation using a microcentrifuge and resuspended in 5 pellet volumes of cytoplasmic extract buffer (10 mM HEPES, 60 mM KCl, 1 mM EDTA, 0.075% (v/v) Nonidet P-40, 1 mM phenylmethylsulfonyl fluoride, adjusted to pH 7.6). After a short incubation on ice, the cytoplasmic extract was removed from the pellet. Following washing with cytoplasmic extract without detergent (Nonidet P-40), the fragile nuclei were resuspended in nuclear extract buffer [20 mM TRIS-HCl, 420 mM NaCl, 1.5 mM MgCl2, 0.2 mm EDTA, 1 mM phenylmethylsulfonyl fluoride, and 25% (v/v) glycerol, adjusted to pH 8.0]. Salt concentration was adjusted to 400 mM using 5M NaCl, and the extract was incubated on ice for 10 min with occasional vortexing. Finally, the extract was spun at 14,000 rpm for 30 min to pellet the nuclei. Protein was estimated according to the Bradford method.
Western blot and antibodies
Nuclear extract was prepared as described above. Equal amounts of protein samples were resolved onto a 4–20% SDS gel, blotted onto PVDF membrane (Perkin Elmer), and immune stained with primary antibodies at the appropriate dilutions of LEDGF monoclonal antibody (BD Biosciences, Cat. no. 611715). DNMT1 (Cat.no. 5032), DNMT3a (Cat. no. 2160), DNMT3b (Cat. no. 2161), Acetyl H3 (Cat. no. 9671), Acetyl H2B (Cat. no. 5410), Histone H3 (Cat.no. 4499), Histone H2B (Cat. no. 5546), H3K9me2 (Cat. no. 9726) antibodies were obtained from Cell Signaling Technologies. H3K9me3 (Cat. no. ab8898), SUV39H1 (Cat. no. ab 12405) and HDAC1 (Cat. no. ab46985) antibodies were purchased from abcam. Membranes were incubated with horseradish peroxidase-conjugated secondary antibodies (1:1500). Specific protein bands were visualized by incubating the membrane with luminol reagent (Santa Cruz Biotechnology, Cat. no. sc-2048) and recorded with FUJIFILM-LAS-4000 luminescent image analyzer (FUJIFILM Medical System Inc.). To ascertain comparative expression and equal loading of the protein samples, the membrane stained earlier was stripped and re-probed with β-actin antibody (Abcam, Cat. no. ab25894).
Histone acetyl transferase (HAT) activity assay
Total histone acetyl transferase activity was measured using EpiQuik HAT Activity/Inhibition Assay Kit (Epigentek, Cat. no. P-4003-48). Breifly, nuclear extracts were incubated with substrate and assay buffer for 60 min. High affinity anti-acetylated histone antibody was added to this followed by added detection antibody. Developing solution was added until the color developed. Absorbance was measured using an ELISA plate reader. Absorbance was normalized to the amount of protein in each sample.
Chromatin immunoprecipitation (ChIP) analysis
ChIP analysis was conducted by the ChIP-IT express kit (Active Motif, Cat. no. 53008) as described earlier.Citation9 Cells were processed following the company’s protocol. The fixation reactions were stopped by adding Glycine Fix-Stop solution. After washing with ice-cold PBS, cells were collected in solution containing PMSF, and centrifuged at 4°C. Cell pellet was disrupted with a Dounce homogenizer (10 strokes of 10 sec each to aid in nuclei release) in 1 ml ice-cold lysis buffer containing protease inhibitor and PMSF. After centrifugation, nuclei were resuspended in shearing buffer and incubated on ice for 10 min. Chromatin was then sheared to 200–300 bp using a closed system ultrasonic cell disruptor (Microson). Samples were centrifuged at 15,000 rpm for 10 min at 4°C, and the supernatant was stored at -80°C. An aliquot of this material was retained as “input” DNA. The remaining chromatin sample was divided; one-half was immunoprecipitated with the test antibodies and the second half was used for a mock immunoprecipitation with a control IgG. ChIP assay bands were compared with assay bands obtained with the input DNA. Mock immunoprecipitation reactions were performed using control IgG. Regions of the human LEDGF promoter that contained Sp1 binding sites were amplified using specific primers. For comparison, a 222-bp sequence from the human LEDGF promoter beyond 2 kb Sp1 binding site was also amplified from the IP and mock IP samples. ChIP assays were conducted via standard PCR amplification (Go-Taq, Promega, Cat. no. M8291) followed by agarose gel electrophoresis. Amplified DNA bands were resolved on 2.5% agarose gels, and images were obtained using FUJIFILM-LAS-4000 luminescent image analyzer (FUJIFILM Medical system Inc.). PCR band sizes were verified using a low molecular mass DNA ladder (Fermentas, Pittsburgh, PA, USA). Primers used in ChIP analyses: Unrelated to Sp1 site primers: Forward, 5′-CAACGTGGCAAAACCCTATC-3′ and reverse, 5′-CCTGACCTCAAGTGGACCAT-3′; Sp1–1 site: Forward, 5′-GCCACTTTCTCCCTAACACG-3′ and reverse, 5′-AACCCTACGTCCCCAAGTTC-3′. The thermocycler programs were as follows: 94оC for 3 min; 35 cycles of 94оC for 20 sec, 55 оC for 30sec, 72 оC for 30sec; and extended at 72 оC for 3 min at the end. The reaction products were analyzed onto a 2.5% low molecular weight agarose gel.
Methylation-Specific PCR (MSP) and methylation detection
Genomic DNA was extracted from cells or cells exposed to single or multiple time with variable doses of UVB radiation as mentioned elsewhere by using commercially available kit (genomicPrep Mini Spin kit, GE Healthcare, Cat. no. 28-9042-75), and DNA was then treated with bisulfate as described in MethylDetectorTM, Instruction Manual (Active Motif, Cat. no. 55001) as well as by Zhu et al.Citation80 Briefly, conversion reaction was performed in PCR tube containing 1μg denatured genomic and conversion reaction buffer with hydroquinone and 3M sodium bisulfite in total volume of 260 μl. Tubes were placed in thermocycler set at 94оC for 3 min, then at 50оC conversion for 5h, followed by a hold (4оC). Reaction mixture containing the bisulfite-modified DNA was purified with DNA purification column (Active Motif, Cat. no. 55001). The eluted DNA was analyzed by MSP using regions specific primers chemically synthesized using designer program at The MethPrimer website; www.urogene.org/methprimer. Primers and PCR conditions for LEDGF promoter were as follows: unmethylated forward primer, 5′-GAGGTTTGGATATTTGTTTTTAAAATT-3′; reverse primer, 5′CCACCCCTACTAACTTCTCTACATA-3′, and methylated forward primer, 5-GTTCGGATATTCGTTTTTAAAATC-3′; reverse primer,5′-GCCCTACTAACTTCTCTACGTA-3′. The thermocycler programs were as follows: 94оC for 3 min; 30 cycle of 94оC for 30 sec, 50 оC for 30sec, 72 оC for 30sec; and extended at 72 оC for 10 min at the end. The reaction products were analyzed onto a 2.5% low molecular weight agarose gel.
Statistical analysis
All the statistical analyses were done in Graphpad Prism software. Comparison between two groups were done with Student’s t-test. Multiple comparisons were done by ANOVA. A p value < 0.001 was defined as indicating a statistically significant difference.
Acknowledgments
Grants provided by the National Eye Institute (NIH) (EY013394) (to DPS) and Research for Preventing Blindness (RPB) are gratefully acknowledged.
Disclosure of Potential Conflicts of Interest
No potential conflicts of interest were disclosed.
Author Contributions
D.P.S. and B.B. designed the study; B.B., B.C., N.F. and E.K. acquired the data; B.B and D.P.S. analyzed and interpreted the data; B.B. and D.P.S. wrote the manuscript; D.P.S. supervised the study.
References
- Poeschla EM. Integrase, LEDGF and HIV replication. Cell Mol Life Sci 2008; 65:1403 - 24; http://dx.doi.org/10.1007/s00018-008-7540-5; PMID: 18264802
- Shinohara T, Singh DP, Fatma N. LEDGF, a survival factor, activates stress-related genes. Prog Retin Eye Res 2002; 21:341 - 58; http://dx.doi.org/10.1016/S1350-9462(02)00007-1; PMID: 12052388
- Singh DP, Ohguro N, Chylack LT Jr., Shinohara T. Lens epithelium-derived growth factor: increased resistance to thermal and oxidative stresses. Invest Ophthalmol Vis Sci 1999; 40:1444 - 51; PMID: 10359326
- Nakamura M, Singh DP, Kubo E, Chylack LT Jr., Shinohara T. LEDGF: survival of embryonic chick retinal photoreceptor cells. Invest Ophthalmol Vis Sci 2000; 41:1168 - 75; PMID: 10752956
- Singh DP, Kubo E, Takamura Y, Shinohara T, Kumar A, Chylack LT Jr., et al. DNA binding domains and nuclear localization signal of LEDGF: contribution of two helix-turn-helix (HTH)-like domains and a stretch of 58 amino acids of the N-terminal to the trans-activation potential of LEDGF. J Mol Biol 2006; 355:379 - 94; http://dx.doi.org/10.1016/j.jmb.2005.10.054; PMID: 16318853
- Bhargavan B, Fatma N, Chhunchha B, Singh V, Kubo E, Singh DP. LEDGF gene silencing impairs the tumorigenicity of prostate cancer DU145 cells by abating the expression of Hsp27 and activation of the Akt/ERK signaling pathway. Cell Death Dis 2012; 3:e316; http://dx.doi.org/10.1038/cddis.2012.57; PMID: 22647853
- Matsui H, Lin L-R, Singh DP, Shinohara T, Reddy VN. Lens epithelium-derived growth factor: increased survival and decreased DNA breakage of human RPE cells induced by oxidative stress. Invest Ophthalmol Vis Sci 2001; 42:2935 - 41; PMID: 11687539
- Sharma P, Shingh D, Fatma N, Chattopadhyay N, Chylack L, Shinohara T. Attenuation of LEDGF-DNA Binding Affinity and Down Regulation of LEDGF Promoter Activity in Human Lens Epithelial Cells Treated with TGFb1. Invest Ophthalmol Vis Sci 2002; 43:E2344
- Singh DP, Bhargavan B, Chhunchha B, Kubo E, Kumar A, Fatma N. Transcriptional protein Sp1 regulates LEDGF transcription by directly interacting with its cis-elements in GC-rich region of TATA-less gene promoter. PLoS One 2012; 7:e37012; http://dx.doi.org/10.1371/journal.pone.0037012; PMID: 22615874
- Singh DP, Fatma N, Kimura A, Chylack LT Jr., Shinohara T. LEDGF binds to heat shock and stress-related element to activate the expression of stress-related genes. Biochem Biophys Res Commun 2001; 283:943 - 55; http://dx.doi.org/10.1006/bbrc.2001.4887; PMID: 11350077
- Ge H, Si Y, Roeder RG. Isolation of cDNAs encoding novel transcription coactivators p52 and p75 reveals an alternate regulatory mechanism of transcriptional activation. EMBO J 1998; 17:6723 - 9; http://dx.doi.org/10.1093/emboj/17.22.6723; PMID: 9822615
- Singh DP, Kimura A, Chylack LT Jr., Shinohara T. Lens epithelium-derived growth factor (LEDGF) and p52 are derived from a single gene by alternative splicing. Gene 2000; 242:265 - 73; http://dx.doi.org/10.1016/S0378-1119(99)00506-5; PMID: 10721720
- Sutherland HG, Newton K, Brownstein DG, Holmes MC, Kress C, Semple CA, et al. Disruption of Ledgf/Psip1 results in perinatal mortality and homeotic skeletal transformations. Mol Cell Biol 2006; 26:7201 - 10; http://dx.doi.org/10.1128/MCB.00459-06; PMID: 16980622
- Maurer-Stroh S, Dickens NJ, Hughes-Davies L, Kouzarides T, Eisenhaber F, Ponting CP. The Tudor domain ‘Royal Family’: Tudor, plant Agenet, Chromo, PWWP and MBT domains. Trends Biochem Sci 2003; 28:69 - 74; http://dx.doi.org/10.1016/S0968-0004(03)00004-5; PMID: 12575993
- Stec I, Nagl SB, van Ommen GJ, den Dunnen JT. The PWWP domain: a potential protein-protein interaction domain in nuclear proteins influencing differentiation?. FEBS Lett 2000; 473:1 - 5; http://dx.doi.org/10.1016/S0014-5793(00)01449-6; PMID: 10802047
- Engelman A, Cherepanov P. The lentiviral integrase binding protein LEDGF and HIV-1 replication. PLoS Pathog 2008; 4:e1000046; http://dx.doi.org/10.1371/journal.ppat.1000046; PMID: 18369482
- Fatma N, Singh DP, Shinohara T, Chylack LT Jr.. Transcriptional regulation of the antioxidant protein 2 gene, a thiol-specific antioxidant, by lens epithelium-derived growth factor to protect cells from oxidative stress. J Biol Chem 2001; 276:48899 - 907; http://dx.doi.org/10.1074/jbc.M100733200; PMID: 11677226
- Sharma P, Fatma N, Kubo E, Shinohara T, Chylack LT Jr., Singh DP. Lens epithelium-derived growth factor relieves transforming growth factor-beta1-induced transcription repression of heat shock proteins in human lens epithelial cells. J Biol Chem 2003; 278:20037 - 46; http://dx.doi.org/10.1074/jbc.M212016200; PMID: 12649267
- Machida S, Chaudhry P, Shinohara T, Singh DP, Reddy VN, Chylack LT Jr., et al. Lens epithelium-derived growth factor promotes photoreceptor survival in light-damaged and RCS rats. Invest Ophthalmol Vis Sci 2001; 42:1087 - 95; PMID: 11274090
- De Rijck J, Bartholomeeusen K, Ceulemans H, Debyser Z, Gijsbers R. High-resolution profiling of the LEDGF chromatin interaction in the ENCODE region. Nucleic Acids Res 2010; 38:6135 - 47; http://dx.doi.org/10.1093/nar/gkq410; PMID: 20484370
- Tsutsui KM, Sano K, Hosoya O, Miyamoto T, Tsutsui K. Nuclear protein LEDGF recognizes supercoiled DNA by a novel DNA-binding domain. Nucleic Acids Res 2011; 39:5067 - 81; http://dx.doi.org/10.1093/nar/gkr088; PMID: 21345933
- Maertens GN, Cherepanov P, Engelman A. Transcriptional co-activator p75 binds and tethers the Myc-interacting protein JPO2 to chromatin. J Cell Sci 2006; 119:2563 - 71; http://dx.doi.org/10.1242/jcs.02995; PMID: 16735438
- Yokoyama A, Cleary ML. Menin critically links MLL proteins with LEDGF on cancer-associated target genes. Cancer Cell 2008; 14:36 - 46; http://dx.doi.org/10.1016/j.ccr.2008.05.003; PMID: 18598942
- Bartholomeeusen K, Christ F, Hendrix J, Rain JC, Emiliani S, Benarous R, et al. Lens epithelium-derived growth factor/p75 interacts with the transposase-derived DDE domain of PogZ. J Biol Chem 2009; 284:11467 - 77; http://dx.doi.org/10.1074/jbc.M807781200; PMID: 19244240
- Hughes S, Jenkins V, Dar MJ, Engelman A, Cherepanov P. Transcriptional co-activator LEDGF interacts with Cdc7-activator of S-phase kinase (ASK) and stimulates its enzymatic activity. J Biol Chem 2010; 285:541 - 54; http://dx.doi.org/10.1074/jbc.M109.036491; PMID: 19864417
- Leoh LS, van Heertum B, De Rijck J, Filippova M, Rios-Colon L, Basu A, et al. The stress oncoprotein LEDGF interacts with the methyl CpG binding protein MeCP2 and influences its transcriptional activity. Mol Cancer Res 2012; 10:378 - 91; http://dx.doi.org/10.1158/1541-7786.MCR-11-0314; PMID: 22275515
- Bueno MTD, Garcia-Rivera JA, Kugelman JR, Morales E, Rosas-Acosta G, Llano M. SUMOylation of the lens epithelium-derived growth factor/p75 attenuates its transcriptional activity on the heat shock protein 27 promoter. J Mol Biol 2010; 399:221 - 39; http://dx.doi.org/10.1016/j.jmb.2010.03.063; PMID: 20382164
- Ishihara K, Fatma N, Bhargavan B, Chhunchha B, Kubo E, Dey S, et al. Lens epithelium-derived growth factor deSumoylation by Sumo-specific protease-1 regulates its transcriptional activation of small heat shock protein and the cellular response. FEBS J 2012; 279:3048 - 70; http://dx.doi.org/10.1111/j.1742-4658.2012.08686.x; PMID: 22748127
- Ames BN, Shigenaga MK, Hagen TM. Oxidants, antioxidants, and the degenerative diseases of aging. Proc Natl Acad Sci U S A 1993; 90:7915 - 22; http://dx.doi.org/10.1073/pnas.90.17.7915; PMID: 8367443
- Kubo ERI, Miyoshi N, Fukuda M, Akagi Y. Cataract formation through the polyol pathway is associated with free radical production. Exp Eye Res 1999; 68:457 - 64; http://dx.doi.org/10.1006/exer.1998.0624; PMID: 10192803
- Marsili S, Salganik RI, Albright CD, Freel CD, Johnsen S, Peiffer RL, et al. Cataract formation in a strain of rats selected for high oxidative stress. Exp Eye Res 2004; 79:595 - 612; http://dx.doi.org/10.1016/j.exer.2004.06.008; PMID: 15500819
- Salganik RI. The benefits and hazards of antioxidants: controlling apoptosis and other protective mechanisms in cancer patients and the human population. J Am Coll Nutr 2001; 20:Suppl 464S - 72S, discussion 473S-5S; PMID: 11603657
- Salganik RI, Solovyova NA, Dikalov SI, Grishaeva ON, Semenova LA, Popovsky AV. Inherited enhancement of hydroxyl radical generation and lipid peroxidation in the S strain rats results in DNA rearrangements, degenerative diseases, and premature aging. Biochem Biophys Res Commun 1994; 199:726 - 33; http://dx.doi.org/10.1006/bbrc.1994.1289; PMID: 8135816
- Spector A. Oxidative stress-induced cataract: mechanism of action. FASEB J 1995; 9:1173 - 82; PMID: 7672510
- Spector A. Review: Oxidative stress and disease. J Ocul Pharmacol Ther 2000; 16:193 - 201; http://dx.doi.org/10.1089/jop.2000.16.193; PMID: 10803430
- Spector A, Kuszak JR, Ma W, Wang R-R, Ho Ys, Yang Y. The effect of photochemical stress upon the lenses of normal and glutathione peroxidase-1 knockout mice. Exp Eye Res 1998; 67:457 - 71; http://dx.doi.org/10.1006/exer.1998.0548; PMID: 9820794
- Fartasch M, Diepgen TL, Schmitt J, Drexler H. The relationship between occupational sun exposure and non-melanoma skin cancer: clinical basics, epidemiology, occupational disease evaluation, and prevention. Dtsch Arztebl Int 2012; 109:715 - 20; PMID: 23181135
- Zawia NH, Lahiri DK, Cardozo-Pelaez F. Epigenetics, oxidative stress, and Alzheimer disease. Free Radic Biol Med 2009; 46:1241 - 9; http://dx.doi.org/10.1016/j.freeradbiomed.2009.02.006; PMID: 19245828
- Holliday R. Epigenetics: a historical overview. Epigenetics 2006; 1:76 - 80; http://dx.doi.org/10.4161/epi.1.2.2762; PMID: 17998809
- Desfarges S, Abderrahmani A, Hernàndez-Novoa B, Munoz M, Ciuffi A. LEDGF TATA-less promoter is driven by the transcription factor Sp1. J Mol Biol 2011; 414:177 - 93; http://dx.doi.org/10.1016/j.jmb.2011.10.010; PMID: 22019592
- Yuen RK, Neumann SM, Fok AK, Peñaherrera MS, McFadden DE, Robinson WP, et al. Extensive epigenetic reprogramming in human somatic tissues between fetus and adult. Epigenetics Chromatin 2011; 4:7; http://dx.doi.org/10.1186/1756-8935-4-7; PMID: 21545704
- Peters AHFM, Kubicek S, Mechtler K, O’Sullivan RJ, Derijck AA, Perez-Burgos L, et al. Partitioning and plasticity of repressive histone methylation states in mammalian chromatin. Mol Cell 2003; 12:1577 - 89; http://dx.doi.org/10.1016/S1097-2765(03)00477-5; PMID: 14690609
- Zhang Y, Reinberg D. Transcription regulation by histone methylation: interplay between different covalent modifications of the core histone tails. Genes Dev 2001; 15:2343 - 60; http://dx.doi.org/10.1101/gad.927301; PMID: 11562345
- Fischle W, Wang Y, Allis CD. Histone and chromatin cross-talk. Curr Opin Cell Biol 2003; 15:172 - 83; http://dx.doi.org/10.1016/S0955-0674(03)00013-9; PMID: 12648673
- Bannister AJ, Zegerman P, Partridge JF, Miska EA, Thomas JO, Allshire RC, et al. Selective recognition of methylated lysine 9 on histone H3 by the HP1 chromo domain. Nature 2001; 410:120 - 4; http://dx.doi.org/10.1038/35065138; PMID: 11242054
- Czermin B, Melfi R, McCabe D, Seitz V, Imhof A, Pirrotta V. Drosophila enhancer of Zeste/ESC complexes have a histone H3 methyltransferase activity that marks chromosomal Polycomb sites. Cell 2002; 111:185 - 96; http://dx.doi.org/10.1016/S0092-8674(02)00975-3; PMID: 12408863
- Schotta G, Lachner M, Sarma K, Ebert A, Sengupta R, Reuter G, et al. A silencing pathway to induce H3-K9 and H4-K20 trimethylation at constitutive heterochromatin. Genes Dev 2004; 18:1251 - 62; http://dx.doi.org/10.1101/gad.300704; PMID: 15145825
- Jørgensen S, Schotta G, Sørensen CS. Histone H4 Lysine 20 methylation: key player in epigenetic regulation of genomic integrity. Nucleic Acids Res 2013; In press http://dx.doi.org/10.1093/nar/gkt012; PMID: 23345616
- Martin C, Zhang Y. The diverse functions of histone lysine methylation. Nat Rev Mol Cell Biol 2005; 6:838 - 49; http://dx.doi.org/10.1038/nrm1761; PMID: 16261189
- Garcia SN, Pereira-Smith O. MRGing chromatin dynamics and cellular senescence. Cell Biochem Biophys 2008; 50:133 - 41; http://dx.doi.org/10.1007/s12013-008-9006-7; PMID: 18231726
- Lang-Mladek C, Popova O, Kiok K, Berlinger M, Rakic B, Aufsatz W, et al. Transgenerational inheritance and resetting of stress-induced loss of epigenetic gene silencing in Arabidopsis. Mol Plant 2010; 3:594 - 602; http://dx.doi.org/10.1093/mp/ssq014; PMID: 20410255
- Low M, Read EL, Borek E. Methylation of DNA in HeLa cells after ultraviolet irradiation. Int J Radiat Oncol Biol Phys 1976; 1:289 - 94; http://dx.doi.org/10.1016/0360-3016(76)90053-5; PMID: 972089
- Jones PA, Baylin SB. The epigenomics of cancer. Cell 2007; 128:683 - 92; http://dx.doi.org/10.1016/j.cell.2007.01.029; PMID: 17320506
- Fahrner JA, Eguchi S, Herman JG, Baylin SB. Dependence of histone modifications and gene expression on DNA hypermethylation in cancer. Cancer Res 2002; 62:7213 - 8; PMID: 12499261
- Katiyar SK, Singh T, Prasad R, Sun Q, Vaid M. Epigenetic alterations in ultraviolet radiation-induced skin carcinogenesis: interaction of bioactive dietary components on epigenetic targets. Photochem Photobiol 2012; 88:1066 - 74; http://dx.doi.org/10.1111/j.1751-1097.2011.01020.x; PMID: 22017262
- Donkena KV, Young CY, Tindall DJ. Oxidative stress and DNA methylation in prostate cancer. Obstet Gynecol Int 2010; 2010:302051; http://dx.doi.org/10.1155/2010/302051; PMID: 20671914
- Okano M, Xie S, Li E. Cloning and characterization of a family of novel mammalian DNA (cytosine-5) methyltransferases. Nat Genet 1998; 19:219 - 20; http://dx.doi.org/10.1038/890; PMID: 9662389
- Moresi V, Carrer M, Grueter CE, Rifki OF, Shelton JM, Richardson JA, et al. Histone deacetylases 1 and 2 regulate autophagy flux and skeletal muscle homeostasis in mice. Proc Natl Acad Sci U S A 2012; 109:1649 - 54; http://dx.doi.org/10.1073/pnas.1121159109; PMID: 22307625
- Mihaylova MM, Vasquez DS, Ravnskjaer K, Denechaud PD, Yu RT, Alvarez JG, et al. Class IIa histone deacetylases are hormone-activated regulators of FOXO and mammalian glucose homeostasis. Cell 2011; 145:607 - 21; http://dx.doi.org/10.1016/j.cell.2011.03.043; PMID: 21565617
- Zhong L, D’Urso A, Toiber D, Sebastian C, Henry RE, Vadysirisack DD, et al. The histone deacetylase Sirt6 regulates glucose homeostasis via Hif1alpha. Cell 2010; 140:280 - 93; http://dx.doi.org/10.1016/j.cell.2009.12.041; PMID: 20141841
- Deaton AM, Bird A. CpG islands and the regulation of transcription. Genes Dev 2011; 25:1010 - 22; http://dx.doi.org/10.1101/gad.2037511; PMID: 21576262
- Stewart MD, Li J, Wong J. Relationship between histone H3 lysine 9 methylation, transcription repression, and heterochromatin protein 1 recruitment. Mol Cell Biol 2005; 25:2525 - 38; http://dx.doi.org/10.1128/MCB.25.7.2525-2538.2005; PMID: 15767660
- Li B, Jackson J, Simon MD, Fleharty B, Gogol M, Seidel C, et al. Histone H3 lysine 36 dimethylation (H3K36me2) is sufficient to recruit the Rpd3s histone deacetylase complex and to repress spurious transcription. J Biol Chem 2009; 284:7970 - 6; http://dx.doi.org/10.1074/jbc.M808220200; PMID: 19155214
- Papp B, Müller J. Histone trimethylation and the maintenance of transcriptional ON and OFF states by trxG and PcG proteins. Genes Dev 2006; 20:2041 - 54; http://dx.doi.org/10.1101/gad.388706; PMID: 16882982
- Ganapathy V, Daniels T, Casiano CA. LEDGF: a novel nuclear autoantigen at the crossroads of cell survival and apoptosis. Autoimmun Rev 2003; 2:290 - 7; http://dx.doi.org/10.1016/S1568-9972(03)00063-6; PMID: 12965181
- Mediavilla-Varela M, Pacheco FJ, Almaguel F, Perez J, Sahakian E, Daniels TR, et al. Docetaxel-induced prostate cancer cell death involves concomitant activation of caspase and lysosomal pathways and is attenuated by LEDGF. Mol Cancer 2009; 8:68; http://dx.doi.org/10.1186/1476-4598-8-68; PMID: 19715609
- Blackledge NP, Zhou JC, Tolstorukov MY, Farcas AM, Park PJ, Klose RJ. CpG islands recruit a histone H3 lysine 36 demethylase. Mol Cell 2010; 38:179 - 90; http://dx.doi.org/10.1016/j.molcel.2010.04.009; PMID: 20417597
- Bernstein BE, Mikkelsen TS, Xie X, Kamal M, Huebert DJ, Cuff J, et al. A bivalent chromatin structure marks key developmental genes in embryonic stem cells. Cell 2006; 125:315 - 26; http://dx.doi.org/10.1016/j.cell.2006.02.041; PMID: 16630819
- Guenther MG, Levine SS, Boyer LA, Jaenisch R, Young RA. A chromatin landmark and transcription initiation at most promoters in human cells. Cell 2007; 130:77 - 88; http://dx.doi.org/10.1016/j.cell.2007.05.042; PMID: 17632057
- Tyson F, Heindel J. Environmental Influences on Epigenetic Regulation. Environ Health Perspect 2005; 113:A839
- Jenuwein T, Allis CD. Translating the histone code. Science 2001; 293:1074 - 80; http://dx.doi.org/10.1126/science.1063127; PMID: 11498575
- Margueron R, Trojer P, Reinberg D. The key to development: interpreting the histone code?. Curr Opin Genet Dev 2005; 15:163 - 76; http://dx.doi.org/10.1016/j.gde.2005.01.005; PMID: 15797199
- Okano M, Bell DW, Haber DA, Li E. DNA methyltransferases Dnmt3a and Dnmt3b are essential for de novo methylation and mammalian development. Cell 1999; 99:247 - 57; http://dx.doi.org/10.1016/S0092-8674(00)81656-6; PMID: 10555141
- Murray K. The Occurrence of Epsilon-N-Methyl Lysine in Histones. Biochemistry 1964; 3:10 - 5; http://dx.doi.org/10.1021/bi00889a003; PMID: 14114491
- Gary JD, Clarke S. RNA and protein interactions modulated by protein arginine methylation. Prog Nucleic Acid Res Mol Biol 1998; 61:65 - 131; http://dx.doi.org/10.1016/S0079-6603(08)60825-9; PMID: 9752719
- Kubo E, Fatma N, Akagi Y, Beier DR, Singh SP, Singh DP. TAT-mediated PRDX6 protein transduction protects against eye lens epithelial cell death and delays lens opacity. Am J Physiol Cell Physiol 2008; 294:C842 - 55; http://dx.doi.org/10.1152/ajpcell.00540.2007; PMID: 18184874
- Fatma N, Kubo E, Sharma P, Beier DR, Singh DP. Impaired homeostasis and phenotypic abnormalities in Prdx6-/-mice lens epithelial cells by reactive oxygen species: increased expression and activation of TGFbeta. [beta] Cell Death Differ 2005; 12:734 - 50; http://dx.doi.org/10.1038/sj.cdd.4401597; PMID: 15818411
- Takamura Y, Fatma N, Kubo E, Singh DP. Regulation of heavy subunit chain of gamma-glutamylcysteine synthetase by tumor necrosis factor-alpha in lens epithelial cells: role of LEDGF. Am J Physiol Cell Physiol 2006; 290:C554 - 66; http://dx.doi.org/10.1152/ajpcell.00398.2005; PMID: 16403949
- Fatma N, Singh DP, Shinohara T, Chylack LT Jr.. Transcriptional regulation of the antioxidant protein 2 gene, a thiol-specific antioxidant, by lens epithelium-derived growth factor to protect cells from oxidative stress. J Biol Chem 2001; 276:48899 - 907; http://dx.doi.org/10.1074/jbc.M100733200; PMID: 11677226
- Zhu WG, Srinivasan K, Dai Z, Duan W, Druhan LJ, Ding H, et al. Methylation of adjacent CpG sites affects Sp1/Sp3 binding and activity in the p21(Cip1) promoter. Mol Cell Biol 2003; 23:4056 - 65; http://dx.doi.org/10.1128/MCB.23.12.4056-4065.2003; PMID: 12773551