Abstract
Normal development depends on the precise sequence of changes in the configuration of chromatin; these are primarily related to specific biochemical modifications such as acetylation or methylation of histones and DNA methylation. While the role of DNA methylation during preimplantation development has been studied extensively, little is known about histone modifications related to early embryonic development. Here, we investigated gene-specific histone modifications in in vitro produced bovine blastocysts. Selected genes thought to be critical for bovine preimplantation development were examined and included POU5F1 (OCT4), NANOG, INFT, GAPDH, SLC2A3 and IGF1. We used chromatin immunoprecipitation from pools of bovine blastocysts to unravel several modifications of histone H3 in relation to mRNA expression profiles. We focused on the two cell compartments of the blastocyst, the inner cell mass (ICM) and the trophectoderm (TE). We show that gene expression patterns in the ICM and TE of the bovine blastocyst are consistent with histone modification patterns on the promoter of the corresponding genes. The data show a complex epigenetic pattern of promoter occupancy by transcriptionally permissive and repressive H3 modifications. These results pave the way to in-depth epigenetic studies of preimplantation embryos that are crucial to gain a better understanding of the epigenetic changes frequently observed after use of assisted reproductive technologies.
Introduction
The nucleosome forms the basic unit of chromatin, and consists of DNA wrapped around an octamer of core histones H2A, H2B, H3 and H4. Chromatin can be altered by a variety of enzymes that covalently modify histones. These post-translational modifications of histone tails are critical for the ability of the genome to store, release and inherit biological information.Citation1 These biochemical modifications, including histone acetylation, phosphorylation, carboxylation and methylation, are associated with regulatory changes of the genome. Histone methyltransferases (HMTs) catalyze site-specific methylation of histone proteins, which, depending on the site, can be involved in either repression or activation of genes. Extensively studied histone methylation sites include H3K4, H3K9, H3K27, H3K36, H3K79 and H4K20,Citation2 which are involved in various biological processes, including transcriptional activation, DNA repair and cell cycle regulation, and thus play a crucial role in growth and development.Citation3,Citation4
Normal development depends on a precise sequence of changes in the configuration of chromatin that are primarily related to the acetylation and methylation status of histones and the methylation of the genomic DNA. These epigenetic modifications control tissue-specific gene expression. During early mammalian development, reprogramming of genomic DNA modifications occurs concomitantly with the formation of the zygote. The paternal DNA is actively and rapidly demethylated after fertilization, while the maternal DNA undergoes passive demethylation, as shown in bovine, murine, porcine, rat and human zygotes.Citation5-Citation10 Initially, the embryonic DNA is further demethylated; the beginning of remethylation correlates with the species-specific onset of transcription from the embryonic genome, leading to higher methylation levels in the ICM rather than in TE in blastocyst stages.Citation7,Citation11 These mechanisms ensure that critical steps during early development, such as timing of first cell division, compaction and blastocyst formation with the emergence of two distinct cell layers, the inner cell mass (ICM) and trophectoderm (TE), followed by expansion and hatching of the blastocyst, are regulated by well-orchestrated gene expression. While the biological role of DNA methylation has been studied extensively, little is known on histone modifications related to early embryonic development.
The development of effective chromatin immunoprecipitation (ChIP) protocols has enabled studies of protein-DNA interactions and mapping of histone modifications on DNA.Citation12,Citation13 ChIP assays have recently been refined to allow analysis of small cell samples, including mouse embryos.Citation14 H3K27me3 asymmetry was observed on developmentally regulated promoters in the ICM and TE compartments of mouse blastocysts.Citation14 Here, we investigated gene-specific histone modifications in bovine blastocysts. We validated the ChIP method with whole blastocysts produced in vitro and subsequently applied the method to isolated bovine ICMs and TEs. Genes selected for this study are thought to be critical for bovine preimplantation development and include POU5F1 (formerly called OCT4), NANOG, INFT (interferon tau), SLC2A3 (formerly called GLUT3), IGF1 (insulin-like growth factor I) and the housekeeping gene GAPDH (glyceraldehyde 3-phosphate dehydrogenase). The promoters of these genes were assessed for enrichment in H3K4me3, a transcriptionally permissive modification associated with transcription start sites (TSSs), acetylated H3K9 (H3K9ac), a mark of active promoters, and H3K27me3 and H3K9me3, two transcriptionally repressive modifications. We set out, for the first time to our knowledge, to identify differences in histone modifications between the ICM of bovine blastocysts and TE in relation to gene expression.
Results
In this study, we examined H3K4me3, a transcriptionally permissive modification associated with TSSs; H3K9ac, a mark of transcriptionally active promoters; and H3K27me3 and H3K9me3, two repressive modifications when localized on promoters. Enrichment patterns of these modified histones on the promoter of two expressed genes (GAPDH and POU5F1) and one repressed gene (IGF1) were determined in intact bovine blastocysts and, subsequently, on a greater panel of genes in ICMs and TEs isolated from in vitro produced bovine blastocysts. Histone modifications were analyzed in relation to the mRNA expression data of the selected genes by RT-qPCR ()
Figure 1. mRNA expression profile of the genes selected for ChIP analysis and concomitant mRNA analysis. Results are presented as fold change relative to ICM-expression (set to 1) (M ± SD) (based on 8 replicates)
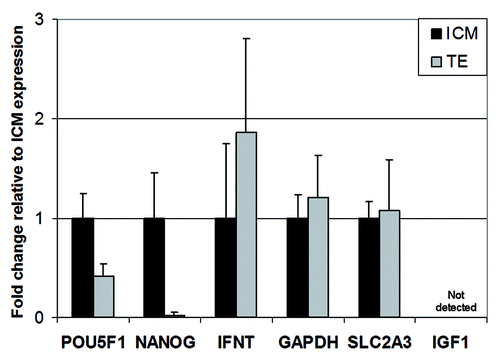
Analysis of intact blastocysts
First, we performed a pilot ChIP study in which we validated the ChIP method with chromatin from intact bovine blastocysts (). As expected, H3K4me3 was enriched on the promoters of the selected genes relative to the control (no antibody), albeit at much higher levels on GAPDH and POU5F1. These findings were consistent with their expression profile. H3K4me3 levels detected on IGF1 were barely above control levels (). Levels for H3K9ac were apparently low compared with H3K4me3, which may have been due to the relative low level of H3K9 acetylation in these embryos, and/or to the efficiency of the ChIP antibody (data not shown).Citation14 In contrast to H3K4me3, H3K27me3 and H3K9me3 were strongly detected on IGF1, which is in line with its transcriptionally inactive state. Lastly, slight enrichment of GAPDH and POU5F1 in H3K9me3 was detected, suggesting variable levels of expression of these genes in the whole embryo, in particular POU5F1. For the GAPDH housekeeping gene, slight anti-H3K9me3 precipitation of the promoter could be due to presence of this modification in the 3′ direction of the TSS, which is located near to the qPCR analyzed region of the promoter. This is likely because primers amplifying the GAPDH promoter are positioned -299 to -398 bp 5′ of TSS and chromatin shearing resulted in fragment length of on average 450 bp, which means that some fragments were up to 700 bp. Some highly expressed genes have been reported to harbor H3K9me3 within their transcribed regions although the promoter is devoid of this histone mark.Citation15
Figure 2. Analysis of histone modifications in intact bovine blastocysts. Modified histones examined are indicated on the x-axis, for a promoter region of the GAPDH, IGF1 and POU5F1. H3K4me3_1 and _2, and H3K27me3_1 and _2 each correspond to a round of duplicate ChIPs. H3K9me3 ChIPs were done in one replicate only. Each ChIP was analyzed by duplicate qPCR for each gene.
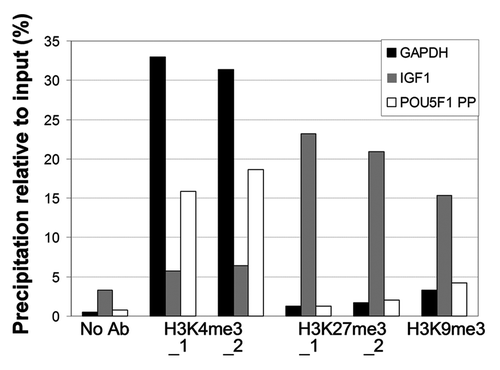
Collectively, these data show that ChIP can be reliably used for bovine blastocysts. Our results show that enrichment profiles of the histone modifications examined here were as expected and revealed either permissive or repressive features consistent with the mRNA expression patterns of the corresponding genes.
The ICM and TE compartments display histone modifications consistent with gene expression patterns
Next, we analyzed the enrichment patterns of the modified histones in the ICM and TE of in vitro produced blastocysts separately to reveal potential spatial differences in histone modifications and gene expression. To determine whether the specificity of ICM vs. TE gene expression patterns was reflected at the epigenetic level, ICMs and TEs were separated from day 8 in vitro produced blastocysts with an average number of ~130 cells and a total of 102 ICMs and 105 TEs were used for these experiments. All ICMs and TEs were pooled to prepare enough cross-linked chromatin from each compartment. ICM and TE chromatin fractions were aliquoted for ChIP as described previouslyCitation14to allow analysis of several different histone modifications from the same chromatin batch (each modification was ChIPed in duplicate). A summary of the results is shown in .
Figure 3. Analysis of histone modifications in purified ICM and TE compartments of bovine blastocysts. Modified histones examined are indicated on the x-axis, for a promoter region of the following genes: (A) GAPDH, (B) SLCA2A3, (C) IGF1, (D) POU5F1 proximal promoter (PP), proximal enhancer (PE) and distal enhancer (DE), (E) NANOG and (F) INFT. Data are from duplicate ChIPs for each modification, each analyzed by duplicate qPCR.
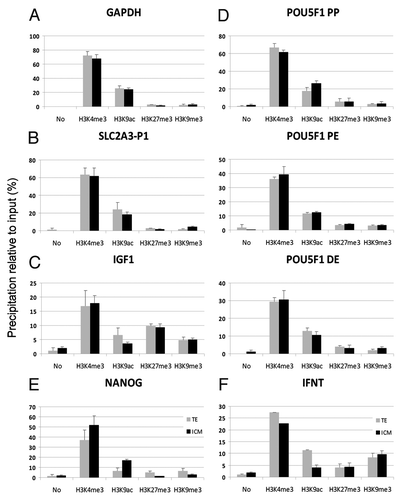
We found a similar enrichment pattern of the proximal promoter (referred to as POU5F1-PP), the proximal enhancer (POU5F1-PE) and the distal enhancer (POU5F1-DE) by each of the histone modifications examined, in both the ICM and TE (). ChIP revealed H3K4me3 and H3K9ac promoter occupancy, in line with the active state of the promoter at least in some blastomeres, as well as low level occupancy (relative to background) by H3K27me3 and H3K9me3, consistent with repression of the gene in other sets of blastomeres. The higher acetylation observed in the average of all ICM cells indicates a more widespread activation in this compartment.
In contrast to POU5F1, NANOG was expressed at a higher level in the ICM relative to the TE (). In line with this expression pattern, the NANOG promoter showed greater occupancy by H3K4me3 and H3K9ac in the ICM rather than in the TE, and, conversely, slightly more H3K27me3 and H3K9me3 in the TE ().
The INFT gene was expressed in both the ICM and TE, though at a higher level in the TE. Accordingly, we observed greater occupancy in H3K4me3 and H3K9ac on the INFT promoter in the TE than in the ICM (). Moreover, detection of H3K9ac was consistent with expression of INFT in the ICM compartment. However, as described for other genes in this study, we also found that INFT displayed H3K27me3 and H3K9me3 at similar levels in the ICM and TE. Previously, it was shown that high H3K9ac can override H3K27me3 and activate gene expression.Citation16,Citation17 With regard to H3K9ac and H3K9me3, this could be the result of an allele-specific pattern and/or due to variation between adjacent or nearby nucleosomes.
As anticipated from the ubiquitous expression profile, the GAPDH promoter, active in the ICM and TE (), was enriched in H3K4me3 and H3K9ac, while H3K9me3 and H3K27me3 were not detected above background level (). SLC2A3 was expressed at similar levels in the ICM and TE and displayed similar promoter enrichment in H3K4me3 and H3K9ac (). In contrast, the IGF1 promoter, silenced in both blastocyst compartments, carried a complex pattern of histone modifications, including H3K4me3, H3K9ac, H3K9me3 and H3K27me3 (). This pattern may be accounted for by a transcriptionally “primed” state, characterized by the presence of H3K4me3 and H3K9ac marks, together with H3K27me3. It may also reveal distinct combinations of histone marks in different cells in the embryo at this stage.
Table 1. RT-qPCR Primers used for mRNA analysis in this study
To take potential differences in precipitation efficiency between different antibodies into account, we also presented the data on a per antibody basis. Results were consistent with the gene-based analysis and did not reveal deviations from the above analysis (Fig. S1). When correlating the levels of histone modification for all genes in ICM and TE, we found a close correlation for H3K4me3 and H3K9ac (r = 0.83). All other histone modification levels did not correlate although they displayed a clear trend according to their biological function (permissive or repressive) ( Fig. S2).
Collectively, our ChIP analyses of bovine ICMs and TEs show consistent histone occupancy levels corresponding to the respective mRNA expression profiles. The data also reveal a complex pattern of occupancy by transcriptionally permissive and repressive histone modifications. In both situations, apparent complexity of the associated epigenetic profiles was consistent with an intermediate gene expression pattern identified in embryos.Citation18,Citation19
Discussion
Bovine preimplantation development is important not only because Bos taurus is an agriculturally important species but also because bovine early development is increasingly seen as a model for human development.Citation20,Citation21 Both are uniparous, both have a similar rate of preimplantation development, which is slower than for murine embryos, and both have a gestation period of nine months, compared with 21 d for the mouse. In vitro production methods for bovine embryos are well advanced due to the availability of large numbers of ovaries from local slaughterhouses. Bovine blastocysts developing in vivo are readily obtained non-surgically by uterine flushing and one result of this is a longstanding international trade in bovine embryos (www.iets.org).
SCNT and ARTs including in vitro fertilization, ICSI, cryopreservation, and in vitro embryo culture are associated with changes in DNA methylation and with fetal and placental abnormalities and an increased frequency of rare epigenetic diseases, such as Beckwith-Wiedemann syndrome in humans.Citation7,Citation22-Citation27While numerous studies into the DNA methylation status of bovine embryos produced by ARTs have been reported (see ref. Citation28), little is known about the histone status of preimplantation embryos. Using immunofluorescence and specific antibodies, histone H4 lysine was found to be differentially acetylated in bovine embryos produced in vitro by SCNT or by parthenogenetic activation.Citation29 Here, we employed for the first time immunoprecipitation of specific chromatin marks in the two compartments of the bovine blastocyst. The results show general consistency between mRNA expression and histone modification and revealed a rather high variation of the epigenetic marks in some of the genes analyzed here.
Low level H3K9ac occupancy has been shown to be associated with silencing of potentially active genes in ES cells.Citation30 However, one cannot fully exclude the possibility that H3K4me3 and H3K27me3 were enriched in different cells within the ICM and TE, which would be compatible with the apparent co-detection of both marks on the same genomic site. In contrast, H3K9me3 enrichment is consistent with the repressed state of the specific genes.
Pou5f1/Oct4 is a member of the POU transcription factor family with a germ line-specific expression profile. It has been widely used to identify pluripotent cells in many different species.Citation31-Citation33Oct4 protein is found in mouse, porcine and bovine blastocysts, both in the ICM and trophectoderm.Citation31 In contrast, the POU5F1 transcript is ubiquitously present in all cells of embryos at the morula stage; however, in Day 7 bovine blastocysts, POU5F1 was not visible in the TE by in situ hybridization, indicating that transcriptional downregulation of Oct4 upon differentiation is similar to that observed in mouse and other mammals.Citation34 The lack of Oct4 expression is associated with failure to form the inner cell mass in murine blastocystsCitation35 and with apoptosis of murine primordial germ cells.Citation36 We found a similar enrichment pattern of the three POU5F1 promoter and enhancer regions (POU5F1-PP; POU5F1-PE; POU5F1-DE) analyzed in this study by each of the histone modifications in ICM and TE. The mosaic occupancy pattern demonstrates subtle regulation of POU5F1 expression, which is consistent with previous findings in which it was shown that the pluripotent status of a cell is subject to well-defined POU5F1 expression levels.Citation37 Oct4 is an essential transcription factor for reprogramming of somatic cells into induced pluripotent stem cells.Citation38
Nanog is a transcription factor that is critically involved in self-renewal of pluripotent stem cells. Expression of Nanog is essential for the maintenance of pluripotency, the absence of Nanog is associated with the loss of pluripotency and differentiation of stem cells into derivatives of various germ layers.Citation39,Citation40 Our results revealed a higher enrichment of permissive histone modifications on the NANOG promoter in ICM cells relative to the TE cells, which is in line with the critical role of Nanog in maintaining pluripotency. Low level promoter occupancy by the repressive marks in the TE may suggest transcriptional repression of NANOG by other epigenetic mechanisms such as DNA methylation in the TE.Citation41
Interferon tau (INFT) is thought to be primarily expressed from the trophectodermal part of the hatched blastocyst and is critical for maternal recognition of pregnancy in this species.Citation42 It is also considered a sensitive marker of developmental stage and quality of bovine embryos. Early and high expression of INFT is indicative for poor quality of bovine embryos.Citation43 In general, results presented here are in line with this expression profile, but also show the complexity of the regulation of expression of this gene.
GAPDH is a housekeeping gene that is consistently expressed in many tissues and cells, including embryos. Our mRNA data revealed similar expression levels in both ICM and TE, which is in line with the crucial biochemical function of the gene. Histone analysis confirmed this expression profile and revealed similar histone promoter occupancy in both cell compartments of the bovine blastocyst.
Glucose transporters, including SLC2A3, were expressed in all stages of in vitro produced bovine embryos.Citation44 The isoforms have specialized functions at different developmental stages in bovine embryos. SLC2A3 serves as a high affinity transporter to ensure glucose supply from the external environment. It plays a crucial role in the uptake of maternal glucose by the blastocyst.Citation45 SLC2A3 transcription was upregulated in in vitro derived as compared with in vivo produced blastocysts indicating that this isoform is a sensitive marker for suboptimal embryo environment.Citation45 The histone enrichment profile found in this study was in line with the ubiquitous mRNA expression pattern of this gene.
IGF-I is a growth factor belonging to Insulin-like-Growth Factor family; it has anti-apoptotic activities and exerts strong mitogenic effects in several mammalian cell types, including blastomeres from preimplantation embryos. It plays an essential role in mammalian reproduction, as shown by the impaired ovarian activity and embryo development in gene knockout mouse models (for review see ref. Citation46). The lack of detectable IGF1 transcripts in bovine embryos up to the blastocyst stage indicates a predominantly paracrine mode of action as it is found in large amounts in the oviductal and uterine secretions. However, bovine embryos are capable of producing IGF-II, IGF-IIR and IGF-IR in large amounts, particularly after hatching, which may be important for the formation of the filamentous conceptus.Citation47 The histone modification profile for IGF1 is characterized by a complex occupancy of specific marks which maintain the repressive status of this gene during preimplantation development. Apparent dual occupancy of the IGF1 promoter by H3K9me3 and H3K27me3 suggests dual repression by both of these marks. Alternatively, this may reflect epigenetic mosaicism of the IGF1 promoter in the ICM and TE, whereby the gene is kept repressed by H3K9me3 in a set of blastomeres, regardless of the cell compartment, and by H3K27me3 (potentially with or without H3K4me3) in another set of cells. This alternative would be consistent with the mosaic expression pattern of genes in the ICM and/or TE in bovine blastocysts.Citation48
The above observation raises the question of whether a promoter can truly be co-enriched simultaneously in permissive and repressive marks on a given allele, supporting a view of “bivalency”Citation17 or “multivalency” (in cases in which there are more than two marks detected),Citation49 or whether the marks occur on different alleles in different cells. Demonstrating bi- or multi-valency, e.g., by sequential ChIP,Citation17,Citation50 would be challenging for mammalian embryos, given the low amount of chromatin available for ChIP. Even with embryos readily available in large numbers (e.g., zebrafish), sequential ChIP lacks robustness and provides inconsistent results.Citation19,Citation49 Significant improvements in ChIP technology may ultimately enable the distinction between co-occupancy of two or more modified histones at a particular site on the same allele, and enrichment in different cells.
In conclusion, the present results demonstrate for the first time the feasibility of histone modification analysis on individual gene promoters in bovine blastocysts. This extends the insight into the epigenetic make-up of early embryos in a significant manner. Previously, this was restricted to analysis of DNA methylation status and imprint marks. Future studies will be directed toward greater sensitivity of ChIP assay to allow analysis from fewer embryos. Here, we used in vitro produced blastocysts to collect enough chromatin. It is well known that in vitro culture conditions may have profound effects on mRNA expression profile primarily mediated via epigenetic changes. The use of in vivo produced embryos in a ChIP system with greater sensitivity could reveal the physiological standard in histone marks during preimplantation development.
Material and Methods
Bovine blastocyst production in vitro and derivation of ICMs and TEs
Oocyte collection and in vitro maturation of bovine oocytes
Oocytes for in vitro maturation were collected as described.Citation43,Citation51 Briefly, ovaries from Holstein Friesian cattle were transported from a local abattoir and washed three times with 0.9% NaCl containing penicillin and streptomycin. Viable cumulus oocyte complexes (COCs) were isolated by slicing the ovaries in Dulbecco’s PBS medium (Sigma Aldrich), supplemented with 0.33 mM Na-pyruvate, 5.56 mM Glucose, 0.9 mM calcium chloride dihydrate, 50 µg/ml streptomycin, 6 µg/ml penicillin G, 4 IU/L heparin and 1 mg/ml BSA (fraction V, Sigma Aldrich). Subsequently, COCs with a homogeneous granulated cytoplasm and surrounding cumulus cells (category I and II) were selected under a stereomicroscope and collected in TCM-air pH 7.2 (TCM199, Sigma Aldrich) containing 50 µg/ml gentamycin sulfate (Sigma Aldrich), 0.2 mM Na-pyruvate (Sigma Aldrich), 4.2 mM NaHCO3 (Roth) and 1 mg/ml BSA (FAF, Sigma Aldrich). COCs were in vitro matured in TCM199 at pH 7.4 supplemented with 0.2 mM Na-pyruvate (Sigma Aldrich), 25 mM NaHCO3 (Roth), 1 mg/ml BSA (FAF, Sigma Aldrich) supplemented with 10 IU/ml of eCG and 5 IU/ml of hCG (Suigonan®, Intervet). COCs were matured in groups of 15 in separate 100 µl drops under silicone oil (Serva) at 39°C in a humidified atmosphere composed of 5% CO2 in air for 22–24 h.
In vitro production of bovine blastocysts
In vitro fertilization (IVF) was performed with matured COCs using 1 × 106 sperm/ml from frozen/thawed semen from one bull with proven performance in IVF.Citation42,Citation50 Frozen semen was thawed at 30°C for 1 min and layered on top of 1 ml of 90% BoviPure™ (Labotect, Goettingen, Germany) and centrifuged at 300 g for 10 min. After centrifugation, the supernatant was removed and the pellet was resuspended in 750 µl fertilization medium (Fert-TALP) containing 6 mg/ml BSA (fraction V) and centrifuged at 400 × g for 3 min. In a second centrifugation step, the pellet was resuspended in Fert-TALP supplemented with HHE [(10 µM hypotaurine (Sigma Aldrich), 0.1 IU/ml heparin (Serva) and 1 µM epinephrine (Sigma Aldrich)] and 6 mg/ml BSA (Fraction V). Matured COCs were washed twice in drops of 100 µl Fert-TALP and transferred for fertilization to 100 µl drops of Fert-TALP containing HHE in groups of 15. COCs and sperm were co-incubated at 5% CO2 in air at 39°C for 19 h.
Prior to in vitro culture of presumptive zygotes, cumulus cells were removed by vortexing for 5 min in TCM-air and the zygotes were washed twice in culture medium (SOFaa) containing 4 mg/ml BSA (FAF). Groups of 5 zygotes were placed in 30 µl drops under silicone oil and incubated at 5% O2, 90% N2 and 5% CO2 (Air Products) in humidified atmosphere at 39°C in SOFaa. Blastocyst formation was evaluated at day 8 (IVF = day 0) of in vitro culture.
Isolation of ICM and TE
Expanded blastocysts with a clearly visible ICM were selected for isolation of ICM and TE cells. Embryos were washed in drops of PBS plus PVA (1 mg/ml; Sigma Aldrich) prior to manual bisection under a stereomicroscope. Scratches in the Petri dish were made to avoid slipping of embryos during bisection by lowering down a microblade (Storz) while the ICM was oriented in the apical part. This resulted in demi-blastocysts, i.e., ICM cells with few adhering TE cells and a pure TE cells fraction. Immediately after bisection, the TE cells were washed twice in PBS/PVA before they were snap frozen in 0.6 ml tubes in groups of 4 for RT-qPCR analysis and groups of 13–24 where collected in 500 µl PBS with 20 mM sodium butyrate and prepared for ChIP analysis as described below.
Adhering TE cells were removed from the ICM cells by immunosurgery in three steps. First, ICMs were incubated in TNBS (Sigma) at 4°C for 8–10 min. After washing twice in PBS/PVA, ICMs were incubated for 20 min at room temperature in anti-DNP-BSA prior to culture for 30 min in guinea pig complement serum (Sigma) at 39°C under oil in the incubator. For removal of the lysed TE cells, ICMs were washed twice in PBS/PVA with a fire polished pipette (diameter 3 μm) and then snap-frozen for RT-qPCR analysis in groups of 4 and groups of 14–20 where collected in 500 µl PBS with 20mM sodium butyrate. A total of 79 intact blastocysts were used for the ChIP validation study. A total of 102 ICMs and 105 TEs, respectively, were pooled for subsequent analysis. This provided chromatin for 8 ChIPs and corresponding input and no-antibody control from ICMs, and 8 ChIPs and the corresponding input and no-antibody control from TEs, respectively.
Gene expression analysis
RNA preparation
Poly(A)+ RNA was isolated using a Dynabeads mRNA Direct Kit (Invitrogen, C) according to the manufacturer’s instruction with some modifications. Briefly, samples consisting of 8 pools of 4 ICM or TE cells each were transferred in 40 µl of lysis-binding buffer [100 mM Tris–HCl. pH 8.0, 500 mM LiCl, 10 mM EDTA, 1% LiDS, 5 mM DTT] and incubated at room temperature for 10 min. A total of 2 pg rabbit globin mRNA per pool was always added as external standard. Prewashed Dynabeads Oligo d(T)25 (5 µl) were pipetted into the lysate. After incubation for 15 min at room temperature on a shaker to allow binding the poly(A)+RNA to the beads, beads and mRNA were separated using a Dynal MPC-E-1 magnetic separator. The beads with the poly(A)+ RNA were briefly washed once in washing buffer A (10 mM Tris-HCL. pH 8.0, 0.15 mM LiCl, 1 mM EDTA, 0.1% LiDS) and twice with washing buffer B (10 mM TRIS-HCl. pH 8.0, 0.15 mM LiCl, 1 mM EDTA) by resuspension followed by magnetic separation. Poly(A)+ RNA was eluted from the beads by incubation in 11 µl of sterile water at 68°C for 3 min and immediately used for cDNA synthesis.
Reverse transcription (RT)
RT was performed in a reaction mixture consisting of 2 µL of 10× RT buffer (Invitrogen), 2 µl of 50 mM MgCl2 (Invitrogen), 2 µl of 10 mM dNTP solution (Bioline), 1 µL (20 Units) of RNAsin (Applied Biosystems), 1 µl (50 Units) of murine leukemia virus (MuLV) reverse transcriptase (Applied Biosystems), 1 µl of hexamer primers (50 mM) (Applied Biosystems), the entire 11 µl mRNA sample, and sufficient water to bring the volume to 20 µl. The samples were incubated at 25°C for 10 min for primer annealing and then incubated at 42°C for 1 h. Finally, the samples were heated to 95°C for 5 min.
Real-Time PCR
Real-Time PCR was performed in 96-well Optical Reaction Plates (Applied Biosystems). The 20 µl PCR reaction mixture in each well included 10 µl of 2xPower SYBR Green PCR Master Mix (Applied Biosystems), 0.4 µl each of the forward and reverse primers (5 µM), 2 µl of cDNA, and 7.2 µl dH2O to bring the volume to 20 µl. PCR reactions were performed in an ABI 7500 Fast Real-Time System (Applied Biosystems) using the following program: 10 min at 95°C for nucleic acid denaturation and activation of the Taq Polymerase, followed by 40 cycles of 95°C for 15 sec and 60°C for 1 min and, finally, a slow heating cycle to obtain a dissociation curve for the products. The specificity of the PCR product was confirmed by dissociation curve analysis and size detection by agarose gel electrophoresis.
In each run, a cDNA dilution prepared from pooled blastocysts and from rabbit globin mRNA was included to give a standard curve for each gene. These standard curves were used for calculation of the relative concentration of each target gene normalized to the signal from the globin mRNA that had been included in the mRNA samples as a control. Quantification was performed with the Sequence Detection Software 1.4 by fitting the threshold cycle (Ct) values to the standard curves. The relative concentration was calculated on a per cell basis because the average cell number differed between the ICM and the TE cell pools. Primers used in this study are shown in . We cannot completely rule out the possibility that the immunosurgical treatment affected mRNA levels of the genes expressed from the ICM. However, alternative methodological approaches such as laser microdissection would be similar in that respect.
Immunoprecipitation of specific histones from bovine ICMs and TEs
Antibodies
Antibodies to H3K4me3 (cat# pAb-003-050, lot# A.3152-001P), H3K9ac (cat# pAb-ACHAHS-044, lot# DA-0010) and H3K9me3 (cat# pAb-056-050, lot# A93-0012) were from Diagenode (Liège, Belgium; www.diagenode.com) and H3K27me3 (cat# 07-449, lot#DAM1612188) was from Millipore (Millipore Inc.; www.millipore.com). All other reagents were from Sigma-Aldrich unless otherwise indicated.
Chromatin Immunoprecipitation
The µChIP was performed as described,Citation13,Citation14 with minor modifications, from intact blastocysts and isolated ICMs and TEs, respectively. Two µg of antibody was bound to 5 µl Dynabeads Protein A (Invitrogen, Oslo, Norway; www.invitrogen.com) in RIPA buffer (10 mM TRIS-HCl pH 7.5, 140 mM NaCl, 1 mM EDTA, 1% Triton X-100, 0.1% SDS, 0.1% Na-deoxycholate). Intact blastocysts, ICMs and TEs were cross-linked for 8 min in 1% formaldehyde solved in PBS/20 mM Na butyrate and quenched with 125 mM glycine. Samples were washed twice in PBS/20 mM Na butyrate, snap frozen in liquid nitrogen and stored at -80°C. A volume of 160 µl lysis buffer (50 mM TRIS-HCl, pH 8, 10 mM EDTA, 1% SDS, protease inhibitor cocktail, 1 mM PMSF, 20 mM sodium butyrate) was split in two parts and used to successively washing through 6 tubes for each sample type to collect all cellular material in the last tube of the series. Each sample tube was removed from -80°C and placed on ice for 5–10 sec before being washed with lysis buffer. Washing and pooling was done on ice using siliconized pipette tips, keeping the same tip throughout the procedure. Sonication of cells lysates was done for 3 × 30 sec on ice with 30 sec pauses, plus another 3 × 30 sec sonication of the pellet after removal of the soluble fraction (see below for details), to produce ~400–500 bp chromatin fragments (Labsonic-M, 3-mm probe; cycle 0.5, 30% power; Sartorius AG, Goettingen, Germany, http://www.sartorius.com). Fragment size of ChIP DNA was assessed by quantitative (q) PCR.Citation15 RIPA buffer (275 µl, with protease inhibitors, 1 mM PMSF, 20 mM butyrate) was added and samples centrifuged at 12,000 g in a swing-out rotor. The supernatant (470 µl) was transferred into a 1.5 ml tube. An additional 500 µl RIPA buffer was added to the pellet (not visible), mixed and centrifuged, and 530 µl supernatant was pooled with the first supernatant. Another 150 µl RIPA buffer was added to the pellet, mixed, sonicated again for 3 × 30 sec on ice and added a volume of 390 µl RIPA buffer. Following centrifugation the third supernatant was pooled with the previous two ones, and 150 µl aliquots were transferred into tubes containing antibodies bound to beads. Tubes with antibody-coated beads and chromatin were rotated at 40 rpm for 2 h at 4°C. Magnetic beads containing ChIP material were washed three times in 100 µl RIPA and once in 100 µl TE buffer. Tube shift was performed to transfer ChIP material into a clean tube while in TE. Elution buffer (150 µl) and Proteinase K (1 µl at 20 µg/µl) was added and DNA elution, cross-link reversal and protein digestion were performed for 2 h at 68°C on a thermomixer. After DNA recovery beads were washed with another 150 µl for 5 min and both supernatants were pooled. ChIP samples were added to elution buffer without SDS to a total volume of 490 µl, and DNA was purified by phenol-chloroform isoamylalcohol extraction. Lastly, DNA was ethanol-precipitated with 10 µl acrylamide carrier and dissolved in 50 µl TE buffer.
Quantitative PCR analysis of ChIP DNA
Immunoprecipitated DNA from 2 independent ChIPs, control and input samples was analyzed by duplicate qPCR starting from 5 μl of template DNA on a MyiQ Real-Time PCR Detection System using iQ SYBR Green (Bio-Rad, www.biorad.com). PCR conditions were 95°C for 3 min and 40 cycles of 95°C for 30 sec, 60°C for 30 sec, and 72°C for 30 sec. ChIP primers used are listed in and positions of the amplicons are shown in . Data are expressed as quantification of the amount of precipitated DNA relative to the input sample by using a standard curve.
Table 2. ChIP primers used in this study
Abbreviations: | ||
Anti-DNP | = | anti-dinitrophenyl |
ART | = | assisted reproductive technology |
BSA | = | bovine serum albumin |
ChIP | = | chromatin immunoprecipitation |
COC | = | cumulus oocyte complexes |
DNA | = | deoxyribonucleic acid |
DTT | = | dithiothreitol |
eCG | = | equine chorionic gonadotropin |
EDTA | = | ethylenediaminetetraacetic acid |
ES cells | = | embryonic stem cells |
FAF | = | fatty acid free |
GAPDH | = | glyceraldehyde 3-phosphate dehydrogenase |
H3 | = | histone 3 |
H2A | = | histone 2A |
H2B | = | histone 2B |
H4 | = | histone 4 |
H3K4me3 | = | trimethylated H3 lysine 4 |
H3K9ac | = | acetylated H3K9 |
H3K27me3 | = | trimethylated H3K27 |
H3K9me3 | = | trimethylated H3K9 |
HAT | = | histone acetyltransferase |
hCG | = | human chorionic gonadotropin |
HMT | = | histone methyltransferase |
ICM | = | inner cell mass |
ICSI | = | intracytoplasmic sperm injection |
IGF1 | = | insulin like growth factor 1 |
INFT | = | interferon tau |
IVF | = | in vitro fertilization |
LIDS | = | lithium dodecyl sulphate |
PBS | = | phosphate buffered saline |
PMSF | = | phenylmethanesulfonylfluoride |
POU5F1, POU class 5 homeobox 1 gene (alias Oct4 | = | POU domain transcription factor OCT4) |
PVA | = | polyvinyl alcohol |
RNA | = | ribonucleic acid |
RT | = | reverse transcription |
RT-qPCR | = | reverse transcriptase-quantitative polymerase chain reaction |
SLC2A3 | = | solute carrier family 2 (facilitated glucose transporter, member 3) |
SCNT | = | somatic cell nuclear transfer |
SDS | = | sodium dodecyl sulfate |
TCM | = | tissue culture medium |
TE | = | trophectoderm |
TNBS | = | trinitrobenzenesulfonic acid |
TSS | = | transcription start sites |
Additional material
Download Zip (884.1 KB)Acknowledgment
This study was funded by DFG (Ni 256/30-2), and Rebirth to H.N. and the Research Council of Norway and University of Oslo to P.C.
Disclosure of Potential Conflicts of Interest
No potential conflicts of interest were disclosed.
Supplemental Materials
Supplemental materials may be found here: www.landesbioscience.com/journals/epigenetics/article/23899
References
- Fischle W, Wang Y, Allis CD. Binary switches and modification cassettes in histone biology and beyond. Nature 2003; 425:475 - 9; http://dx.doi.org/10.1038/nature02017; PMID: 14523437
- Greer EL, Shi Y. Histone methylation: a dynamic mark in health, disease and inheritance. Nat Rev Genet 2012; 13:343 - 57; http://dx.doi.org/10.1038/nrg3173; PMID: 22473383
- Downs JA, Nussenzweig MC, Nussenzweig A. Chromatin dynamics and the preservation of genetic information. Nature 2007; 447:951 - 8; http://dx.doi.org/10.1038/nature05980; PMID: 17581578
- Carrozza MJ, Utley RT, Workman JL, Côté J. The diverse functions of histone acetyltransferase complexes. Trends Genet 2003; 19:321 - 9; http://dx.doi.org/10.1016/S0168-9525(03)00115-X; PMID: 12801725
- Mayer W, Niveleau A, Walter J, Fundele R, Haaf T. Demethylation of the zygotic paternal genome. Nature 2000; 403:501 - 2; http://dx.doi.org/10.1038/35000656; PMID: 10676950
- Oswald J, Engemann S, Lane N, Mayer W, Olek A, Fundele R, et al. Active demethylation of the paternal genome in the mouse zygote. Curr Biol 2000; 10:475 - 8; http://dx.doi.org/10.1016/S0960-9822(00)00448-6; PMID: 10801417
- Dean W, Santos F, Stojkovic M, Zakhartchenko V, Walter J, Wolf E, et al. Conservation of methylation reprogramming in mammalian development: aberrant reprogramming in cloned embryos. Proc Natl Acad Sci U S A 2001; 98:13734 - 8; http://dx.doi.org/10.1073/pnas.241522698; PMID: 11717434
- Santos F, Hendrich B, Reik W, Dean W. Dynamic reprogramming of DNA methylation in the early mouse embryo. Dev Biol 2002; 241:172 - 82; http://dx.doi.org/10.1006/dbio.2001.0501; PMID: 11784103
- Beaujean N, Taylor J, Gardner J, Wilmut I, Meehan R, Young L. Effect of limited DNA methylation reprogramming in the normal sheep embryo on somatic cell nuclear transfer. Biol Reprod 2004; 71:185 - 93; http://dx.doi.org/10.1095/biolreprod.103.026559; PMID: 14998909
- Xu Y, Zhang JJ, Grifo JA, Krey LC. DNA methylation patterns in human tripronucleate zygotes. Mol Hum Reprod 2005; 11:167 - 71; http://dx.doi.org/10.1093/molehr/gah145; PMID: 15695773
- Reik W, Dean W, Walter J. Epigenetic reprogramming in mammalian development. Science 2001; 293:1089 - 93; http://dx.doi.org/10.1126/science.1063443; PMID: 11498579
- Dahl JA, Collas P. Q2ChIP, a quick and quantitative chromatin immunoprecipitation assay, unravels epigenetic dynamics of developmentally regulated genes in human carcinoma cells. Stem Cells 2007; 25:1037 - 46; http://dx.doi.org/10.1634/stemcells.2006-0430; PMID: 17272500
- Dahl JA, Collas P. MicroChIP--a rapid micro chromatin immunoprecipitation assay for small cell samples and biopsies. Nucleic Acids Res 2008; 36:e15; http://dx.doi.org/10.1093/nar/gkm1158; PMID: 18202078
- Dahl JA, Reiner AH, Klungland A, Wakayama T, Collas P. Histone H3 lysine 27 methylation asymmetry on developmentally-regulated promoters distinguish the first two lineages in mouse preimplantation embryos. PLoS One 2010; 5:e9150; http://dx.doi.org/10.1371/journal.pone.0009150; PMID: 20161773
- Dahl JA, Reiner AH, Collas P. Fast genomic muChIP-chip from 1,000 cells. Genome Biol 2009; 10:R13; http://dx.doi.org/10.1186/gb-2009-10-2-r13; PMID: 19208222
- Azuara V, Perry P, Sauer S, Spivakov M, Jørgensen HF, John RM, et al. Chromatin signatures of pluripotent cell lines. Nat Cell Biol 2006; 8:532 - 8; http://dx.doi.org/10.1038/ncb1403; PMID: 16570078
- Bernstein BE, Mikkelsen TS, Xie X, Kamal M, Huebert DJ, Cuff J, et al. A bivalent chromatin structure marks key developmental genes in embryonic stem cells. Cell 2006; 125:315 - 26; http://dx.doi.org/10.1016/j.cell.2006.02.041; PMID: 16630819
- Chazaud C, Yamanaka Y, Pawson T, Rossant J. Early lineage segregation between epiblast and primitive endoderm in mouse blastocysts through the Grb2-MAPK pathway. Dev Cell 2006; 10:615 - 24; http://dx.doi.org/10.1016/j.devcel.2006.02.020; PMID: 16678776
- Lindeman LC, Andersen IS, Reiner AH, Li N, Aanes H, Østrup O, et al. Prepatterning of developmental gene expression by modified histones before zygotic genome activation. Dev Cell 2011; 21:993 - 1004; http://dx.doi.org/10.1016/j.devcel.2011.10.008; PMID: 22137762
- Niemann H, Wrenzycki C. Alterations of expression of developmentally important genes in preimplantation bovine embryos by in vitro culture conditions: implications for subsequent development. Theriogenology 2000; 53:21 - 34; http://dx.doi.org/10.1016/S0093-691X(99)00237-X; PMID: 10735059
- Wrenzycki C, Herrmann D, Lucas-Hahn A, Korsawe K, Lemme E, Niemann H. Messenger RNA expression patterns in bovine embryos derived from in vitro procedures and their implications for development. Reprod Fertil Dev 2005; 17:23 - 35; http://dx.doi.org/10.1071/RD04109; PMID: 15745629
- De Rycke M, Liebaers I, Van Steirteghem A. Epigenetic risks related to assisted reproductive technologies: risk analysis and epigenetic inheritance. Hum Reprod 2002; 17:2487 - 94; http://dx.doi.org/10.1093/humrep/17.10.2487; PMID: 12351517
- Farin PW, Piedrahita JA, Farin CE. Errors in development of fetuses and placentas from in vitro-produced bovine embryos. Theriogenology 2006; 65:178 - 91; http://dx.doi.org/10.1016/j.theriogenology.2005.09.022; PMID: 16266745
- Kang YK, Koo DB, Park JS, Choi YH, Chung AS, Lee KK, et al. Aberrant methylation of donor genome in cloned bovine embryos. Nat Genet 2001; a 28:173 - 7; http://dx.doi.org/10.1038/88903; PMID: 11381267
- Kang YK, Koo DB, Park JS, Choi YH, Kim HN, Chang WK, et al. Typical demethylation events in cloned pig embryos. Clues on species-specific differences in epigenetic reprogramming of a cloned donor genome. J Biol Chem 2001; b 276:39980 - 4; http://dx.doi.org/10.1074/jbc.M106516200; PMID: 11524426
- Niemann H, Wrenzycki C, Lucas-Hahn A, Brambrink T, Kues WA, Carnwath JW. Gene expression patterns in bovine in vitro-produced and nuclear transfer-derived embryos and their implications for early development. Cloning Stem Cells 2002; 4:29 - 38; http://dx.doi.org/10.1089/153623002753632020; PMID: 12006154
- Powell K. Fertility treatments: Seeds of doubt. Nature 2003; 422:656 - 8; http://dx.doi.org/10.1038/422656a; PMID: 12700731
- Niemann H, Kues WA, Lucas-Hahn A, Carnwath JW. Somatic Cloning and Epigenetic Reprogramming in Mammals. In: Atala A, Lanza R, Thomson JA, and Nerem R (eds.). Principles of Regenerative Medicine, Elsevier, ISBN: 978-0123814227, 2011:129-158.
- Maalouf W E, Alberio R, Campbell KHS.. Differential acetylation of histone H4 lysine during development of in vitro fertilized, cloned and parthenogenetically activated bovine embryos. Epigenetics 2008; 3:199 - 209
- Kouzarides T, Berger SL. Chromatin modifications and their mechanisms of action. In: Allis CD, JenuweinT, Reinberg D (eds) Epigenetics CSHL, Press: New York, 2007:191-209.
- Kirchhof N, Carnwath JW, Lemme E, Anastassiadis K, Schöler H, Niemann H. Expression pattern of Oct-4 in preimplantation embryos of different species. Biol Reprod 2000; 63:1698 - 705; http://dx.doi.org/10.1095/biolreprod63.6.1698; PMID: 11090438
- Yeom YI, Fuhrmann G, Ovitt CE, Brehm A, Ohbo K, Gross M, et al. Germline regulatory element of Oct-4 specific for the totipotent cycle of embryonal cells. Development 1996; 122:881 - 94; PMID: 8631266
- Yoshimizu T, Sugiyama N, De Felice M, Yeom YI, Ohbo K, Masuko K, et al. Germline-specific expression of the Oct-4/green fluorescent protein (GFP) transgene in mice. Dev Growth Differ 1999; 41:675 - 84; http://dx.doi.org/10.1046/j.1440-169x.1999.00474.x; PMID: 10646797
- Kurosaka S, Eckardt S, McLaughlin KJ. Pluripotent lineage definition in bovine embryos by Oct4 transcript localization. Biol Reprod 2004; 71:1578 - 82; http://dx.doi.org/10.1095/biolreprod.104.029322; PMID: 15229144
- Nichols J, Zevnik B, Anastassiadis K, Niwa H, Klewe-Nebenius D, Chambers I, et al. Formation of pluripotent stem cells in the mammalian embryo depends on the POU transcription factor Oct4. Cell 1998; 95:379 - 91; http://dx.doi.org/10.1016/S0092-8674(00)81769-9; PMID: 9814708
- Kehler J, Tolkunova E, Koschorz B, Pesce M, Gentile L, Boiani M, et al. Oct4 is required for primordial germ cell survival. EMBO Rep 2004; 5:1078 - 83; http://dx.doi.org/10.1038/sj.embor.7400279; PMID: 15486564
- Niwa H, Miyazaki J, Smith AG. Quantitative expression of Oct-3/4 defines differentiation, dedifferentiation or self-renewal of ES cells. Nat Genet 2000; 24:372 - 6; http://dx.doi.org/10.1038/74199; PMID: 10742100
- Takahashi K, Yamanaka S. Induction of pluripotent stem cells from mouse embryonic and adult fibroblast cultures by defined factors. Cell 2006; 126:663 - 76; http://dx.doi.org/10.1016/j.cell.2006.07.024; PMID: 16904174
- Chambers I, Colby D, Robertson M, Nichols J, Lee S, Tweedie S, et al. Functional expression cloning of Nanog, a pluripotency sustaining factor in embryonic stem cells. Cell 2003; 113:643 - 55; http://dx.doi.org/10.1016/S0092-8674(03)00392-1; PMID: 12787505
- Zaehres H, Lensch MW, Daheron L, Stewart SA, Itskovitz-Eldor J, Daley GQ. High-efficiency RNA interference in human embryonic stem cells. Stem Cells 2005; 23:299 - 305; http://dx.doi.org/10.1634/stemcells.2004-0252; PMID: 15749924
- Barrand SK, Andersen IS, Collas P. Promoter-exon relationship of H3 lysine 9, 27, 36 and 79 methylation on pluripotency-associated genes. Biochem Biophys Res Commun 2010; 401:611 - 7; http://dx.doi.org/10.1016/j.bbrc.2010.09.116; PMID: 20920475
- Bazer FW. Mediators of maternal recognition of pregnancy in mammals. Proc Soc Exp Biol Med 1992; 199:373 - 84; PMID: 1549616
- Wrenzycki C, Herrmann D, Keskintepe L, Martins A Jr., Sirisathien S, Brackett B, et al. Effects of culture system and protein supplementation on mRNA expression in pre-implantation bovine embryos. Hum Reprod 2001; 16:893 - 901; http://dx.doi.org/10.1093/humrep/16.5.893; PMID: 11331635
- Augustin R, Pocar P, Navarrete-Santos A, Wrenzycki C, Gandolfi F, Niemann H, et al. Glucose transporter expression is developmentally regulated in in vitro derived bovine preimplantation embryos. Mol Reprod Dev 2001; 60:370 - 6; http://dx.doi.org/10.1002/mrd.1099; PMID: 11599048
- Lazzari G, Wrenzycki C, Herrmann D, Duchi R, Kruip T, Niemann H, et al. Cellular and molecular deviations in bovine in vitro-produced embryos are related to the large offspring syndrome. Biol Reprod 2002; 67:767 - 75; http://dx.doi.org/10.1095/biolreprod.102.004481; PMID: 12193383
- Velazquez MA, Zaraza J, Oropeza A, Webb R, Niemann H. The role of IGF1 in the in vivo production of bovine embryos from superovulated donors. Reproduction 2009; 137:161 - 80; http://dx.doi.org/10.1530/REP-08-0362; PMID: 19029343
- Yaseen MA, Wrenzycki C, Herrmann D, Carnwath JW, Niemann H. Changes in the relative abundance of mRNA transcripts for insulin-like growth factor (IGF-I and IGF-II) ligands and their receptors (IGF-IR/IGF-IIR) in preimplantation bovine embryos derived from different in vitro systems. Reproduction 2001; 122:601 - 10; http://dx.doi.org/10.1530/rep.0.1220601; PMID: 11570968
- Kues WA, Sudheer S, Herrmann D, Carnwath JW, Havlicek V, Besenfelder U, et al. Genome-wide expression profiling reveals distinct clusters of transcriptional regulation during bovine preimplantation development in vivo. Proc Natl Acad Sci U S A 2008; 105:19768 - 73; http://dx.doi.org/10.1073/pnas.0805616105; PMID: 19064908
- Andersen IS, Østrup O, Lindeman LC, Aanes H, Reiner AH, Mathavan S, et al. Epigenetic complexity during the zebrafish mid-blastula transition. Biochem Biophys Res Commun 2012; 417:1139 - 44; http://dx.doi.org/10.1016/j.bbrc.2011.12.077; PMID: 22209792
- Vastenhouw NL, Zhang Y, Woods IG, Imam F, Regev A, Liu XS, et al. Chromatin signature of embryonic pluripotency is established during genome activation. Nature 2010; 464:922 - 6; http://dx.doi.org/10.1038/nature08866; PMID: 20336069
- Eckert J, Niemann H. In vitro maturation, fertilization and culture to blastocysts of bovine oocytes in protein-free media. Theriogenology 1995; 43:1211 - 25; http://dx.doi.org/10.1016/0093-691X(95)00093-N; PMID: 16727707