Abstract
The histone lysine demethylase KDM5B plays key roles in gene repression by demethylating trimethylated lysine 4 of histone H3 (H3K4me3), a modification commonly found at the promoter region of actively transcribed genes. KDM5B is known to regulate the expression of genes involved in cell cycle progression; however, little is known about the post-translational modifications that regulate KDM5B. Herein, we report that KDM5B is SUMOylated at lysine residues 242 and 278 and that the ectopic expression of the hPC2 SUMO E3 ligase enhances this SUMOylation. Interestingly, the levels of KDM5B and its SUMOylated forms are regulated during the cell cycle. KDM5B is modulated by RNF4, an E3 ubiquitin ligase that targets SUMO-modified proteins to proteasomal degradation. Digital gene expression analyses showed that cells expressing the SUMOylation-deficient KDM5B harbor repressed mRNA expression profiles of cell cycle and DNA repair genes. Chromatin immunoprecipitations confirmed some of these genes as KDM5B targets, as they displayed reduced H3K4me3 levels in cells ectopically expressing KDM5B. We propose that SUMOylation by hPC2 regulates the activity of KDM5B.
Introduction
KDM5B, also known as JARID1B or PLU-1, belongs to the KDM5/JARID1 family of histone lysine demethylases.Citation1,Citation2 KDM5B is overexpressed in several cancer types such as breastCitation3 and prostate cancer.Citation4 It catalyzes the demethylation of the activation mark H3K4me3, commonly found at the promoter region of genes.Citation1,Citation2,Citation4,Citation5 Genome-wide analyses of KDM5B occupancy have revealed its association with transcription start sites and intragenic regions of genes involved in cell cycle, mitosis, chromatin and nucleotide metabolism, development and embryonic stem cell self-renewal.Citation6,Citation7
KDM5B has been shown to regulate cancer cell proliferation by epigenetically repressing the transcription of certain tumor suppressor and cell cycle genes such as BRCA1,Citation8 microRNA let-7e,Citation9 and CDKN1A.Citation10 Furthermore, elevated expression of KDM5B in a slow cycling subpopulation of melanoma cells was demonstrated to be required for maintenance of tumor growth.Citation11 KDM5B-dependent demethylation of the promoter regions of genes that control cell lineage choice such as Egr1 and BMI1 was associated with blockage of terminal differentiation of mouse embryonic stem cells (ESCs).Citation12 In addition, other studies have reported the involvement of KDM5B in regulating ESCs self-renewalCitation6 and promoting differentiation along the neural lineage.Citation7 KDM5B was also shown to play a role in cellular senescence via retinoblastoma-mediated recruitment of KDM5B to E2F-target genes in mouse and primary human cells.Citation13,Citation14
Histone lysine demethylases are known to be regulated by post-translational modifications. For example, ubiquitination of the lysine demethylases KDM4A and Jhd2 results in their proteasome-mediated degradation.Citation15-Citation17 Moreover, the activity of the lysine demethylase complex PHF2-ARID5B is regulated by phosphorylation by the protein kinase A.Citation18 However, it is currently unknown whether lysine demethylases are regulated by SUMOylation, which is a common post-translational modification catalyzed on lysines by a cascade of specific enzymes.Citation19,Citation20 SUMO-conjugated proteins undergo a maturation step promoted by SUMO-specific proteases (SENPs) exposing a Gly-Gly motif at their C-terminus. Following maturation, SUMOs are activated by the SUMO-activating enzyme E1 (AOS1/UBA2) and subsequently transferred to UBC9, the SUMO-conjugating enzyme. Several SUMO E3 ligases often function with UBC9 to enhance SUMO conjugation.Citation19,Citation20
SUMOylation is known to regulate the activity of several transcription factors. Early studies focused on the conjugation of SUMO-1 to c-Jun,Citation21 c-Myb,Citation22 NF-κB,Citation23 and p53Citation24,Citation25 reported that mutation of the SUMOylation sites of these factors resulted in increased transactivation capacity. Interestingly, SUMOylation of Sp3 was shown to modulate the transcriptional activity of this protein by altering its subnuclear distribution.Citation26 In contrast, SUMOylation of the heat shock factors HSF1 and HSF2 was found to stimulate their DNA-binding activity.Citation27,Citation28 More recently, SUMOylation of p53 was shown to promote activation or repression of target genes in a specific context.Citation29 These findings demonstrate the diverse effects of SUMOylation on factors that regulate gene expression.
SUMOylation has been shown to regulate a variety of protein properties and functions. SUMO-conjugation has the potential to modify protein surfaces and positively or negatively modulate protein-protein interactions. For instance, SUMOylation of the carboxyl-terminus binding protein (CtBP) causes loss of interaction with the PDZ domain of nNOS,Citation30 while SUMOylation of p300 promotes association with HDAC6.Citation31 SUMOylation of the poly (A) polymerase has been shown to control its stability, subcellular localization, and polyadenylation activity.Citation32
KDM5B was reported to interact with the SUMO E3 ligase human polycomb protein 2 (hPC2).Citation33 hPC2, also commonly referred to as chromobox homolog 4 (CBX4), has been shown to enhance SUMOylation of numerous proteins including CCTC-binding factor (CTCF),Citation34 carboxyl-terminus binding protein (CtBP),Citation35 Smad-interacting protein 1 (SIP1),Citation36 DNA methyltransferase 3a (DNMT3A),Citation37 homeodomain interacting protein kinase 2 (HIPK2),Citation38 Zinc finger protein 131 (ZNF131),Citation39 BMI1 polycomb ring finger oncogeneCitation40 and the heterogeneous nuclear ribonucleoprotein K (hnRNP K).Citation41
Herein we report that conjugation of SUMO-3 to the histone lysine demethylase KDM5B negatively regulates its occupancy at target genes and, consequently, affects the expression of certain genes. KDM5B associates with hPC2, a SUMO E3 ligase that specifically enhances SUMOylation of KDM5B. We observe that the levels of SUMOylated KDM5B are cell cycle regulated and that the SUMO-dependent ubiquitin ligase RNF4 is able to promote degradation of KDM5B. These data suggest that SUMOylation is a post-translational modification that regulates histone lysine demethylases.
Results
KDM5B is covalently modified by SUMO
Histone lysine demethylases are key modulators of gene expression; however, little is known about the mechanisms regulating their activity. We examined whether certain KDM5 family members were post-translationally modified by SUMOylation. Expression vectors encoding FLAG-KDM5B, MYC-KDM5C, and FLAG-KDM5D were transfected into HEK293T cells along with bicistronic vectors encoding for UBC9, the SUMO conjugating enzyme, and SUMO-1 or a conjugation-defective SUMO-1 mutant (ΔGGSUMO-1). Slower migrating forms of FLAG-KDM5B and MYC-KDM5C were observed by immunoblotting only in cells expressing SUMO-1, and not ΔGGSUMO-1 (, lanes 1–4, top panels). These findings suggest that KDM5B and KDM5C are SUMOylated in vivo. The SUMOylation of KDM5B and KDM5C was specific, as slower migrating forms of KDM5D were not observed (, lanes 5, 6, top panels). Immunoblotting with anti-Tubulin and anti-SUMO-1 antibodies confirmed equivalent protein expression (, middle panels) and the presence of other SUMO-1 modified species, absent in ΔGGSUMO-1-transfected cells (, lower panels).
Figure 1. KDM5B and KDM5C are covalently modified by SUMO. (A) SUMOylation of KDM5B and KDM5C, but not KDM5D. Whole cell lysates from HEK293T cells transfected with FLAG-KDM5B, MYC-KDM5C or FLAG-KDM5D in combination with HA-UBC9/HIS-SUMO-1 or HA-UBC9/HIS-ΔGGSUMO-1 were immunoblotted with the indicated antibodies. The molecular mass markers are shown on the left in kDa and the migration of the unmodified and modified KDM5 family members is indicated on the right. Asterisks (*) indicate high molecular weight SUMOylated proteins in the lower panels. (B) KDM5B is SUMOylated by SUMO-1 and SUMO-3. Whole cell lysates from HEK293T cells transfected with FLAG-KDM5B alone or in combination with HIS-SUMO-1 or HIS-SUMO-3 in the presence or absence of HA-UBC9 were immunoblotted with the indicated antibodies. The molecular mass markers are shown on the left in kDa and the migration of the unmodified and modified KDM5B is indicated on the right. Asterisk indicates high molecular weight proteins conjugated by HIS-tagged SUMO-1 and SUMO-3. (C) SUMO-3 is covalently conjugated to KDM5B. HIS-SUMO-3 conjugated proteins were purified under denaturing conditions from total cell lysates of HEK293T cells expressing FLAG-KDM5B alone or in combination with HIS-SUMO-3. SUMOylated proteins bound to Ni-NTA agarose beads were eluted and immunoblotted with anti-FLAG antibodies. The migration of SUMO-3-FLAG-KDM5B is shown on the right. (D) Endogenous KDM5B is SUMOylated. Total cell lysates from MCF-7 cells stably expressing HIS-SUMO-3 were incubated with Ni-NTA agarose beads under denaturing conditions. SUMOylated proteins bound to beads were then immunoblotted with a polyclonal antibody against KDM5B. An equal number of MCF7 cells lacking expression of HIS-SUMO-3 were used as a control. The migration of endogenous SUMO-3-KDM5B is shown on the right and the arrowhead indicates a non-specific band.
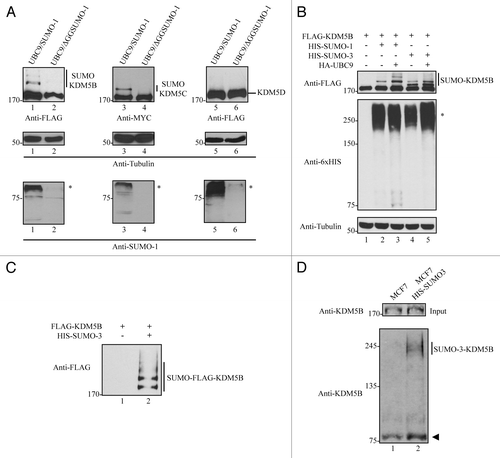
Since several studies have evaluated the genomic occupancy of KDM5B,Citation6,Citation7,Citation42,Citation43 we focused our analysis on this member of the KDM5 family. To evaluate whether KDM5B could also be conjugated by SUMO-3 (SUMO-2 is essentially identical to SUMO-3), HEK293T cells were transfected with KDM5B alone or in combination with SUMO-1 or SUMO-3 in the presence or absence of UBC9 (). The expression of SUMO-1 or SUMO-3 resulted in the appearance of slower migrating forms of KDM5B (, top panel, lanes 2 and 4). As expected, co-expression of UBC9 enhanced KDM5B SUMOylation in the presence of SUMO-1 or SUMO-3 (, top panel, lanes 3 and 5). Similar levels of exogenously expressed SUMO-1 and SUMO-3 were detected by immunoblotting with anti-6xHIS (, middle panel).
To confirm that these slower migrating forms of KDM5B were in fact SUMOylated-KDM5B, we ectopically expressed FLAG-KDM5B with or without HIS-SUMO-3 in HEK293 cells. Cell lysates were prepared and proteins conjugated to HIS-SUMO-3 were enriched using nickel beads (NI-NTA) that recognize HIS-tagged proteins. The bound proteins were separated by SDS-PAGE and immunoblotted with anti-FLAG antibodies. Indeed, SUMO-3-conjugated KDM5B species were identified in NI-NTA affinity pull-downs (). To verify that endogenous KDM5B was SUMOylated, we purified SUMO-3 conjugated proteins from MCF7 cells stably expressing HIS-SUMO3 using Ni-NTA beads under denaturing conditions. Endogenous KDM5B conjugated to HIS-SUMO-3 was indeed detected by immunoblotting with a polyclonal antibody against KDM5B ().
We performed the reciprocal immunoprecipitations, where KDM5B SUMOylation was detected by performing immunoprecipitations with anti-FLAG antibodies using lysates from cells transfected with SUMO-1 or SUMO-3, and FLAG-KDM5B. The proteins were separated by SDS-PAGE and then immunoblotted with anti-SUMO-1 and anti-SUMO-2/3 antibodies. Indeed, slower migrating forms of KDM5B were observed with SUMO-1 or SUMO-3 (Fig. S1A and B). Taken together, these observations demonstrate that KDM5B is SUMOylated in vivo by either SUMO-1 or SUMO-3. We arbitrarily chose to perform our studies with SUMO-3.
SUMOylation of KDM5B is enhanced by hPC2
KDM5B has been shown to interact with hPC2,Citation33 a member of the Polycomb group of proteins and a known SUMO E3 ligase.Citation35 To examine whether hPC2 might enhance SUMOylation of KDM5B, we first evaluated the localization of these proteins in the human osteosarcoma cell line, U2OS. Immunofluorescence analysis of cells expressing KDM5B and hPC2 revealed that these two proteins co-localized within discrete nuclear foci (). We next reasoned that the expression of hPC2 should potentiate the SUMOylation of KDM5B. Immunoblotting analysis of whole cell lysates from HEK293T cells expressing KDM5B and SUMO-3 alone or in combination with hPC2 or PIASy, another SUMO E3 ligase, revealed that co-expression of hPC2 strongly increased the levels of SUMOylated KDM5B (, lane 2). Importantly, this enhancement in KDM5B SUMOylation was specifically promoted by hPC2 given that expression of PIASy did not result in further increase in the levels of SUMO-3 conjugated KDM5B. These findings show that hPC2 specifically enhances the conjugation of SUMO to KDM5B.
Figure 2. hPC2 enhances the SUMOylation of KDM5B. (A) KDM5B co-localizes with hPC2 in U2OS cells. DAPI-stained nuclei (blue) and ectopically expressed FLAG-KDM5B (red), and YFP-hPC2 (green) were detected by fluorescence microscopy. The scale bar denotes 5 µm and images were merged in the indicated panel. Arrowheads indicate transfected cells. (B) SUMOylation of KDM5B is enhanced by hPC2 and not PIASy. Whole cell extracts from HEK293T cells expressing FLAG-KDM5B and HIS-SUMO-3 alone or in combination with YFP-hPC2 or Myc-tagged PIASy were subjected to immunoblotting with the indicated antibodies on the left. The migration of SUMO-3-FLAG-KDM5B is shown on the right. The migration of protein molecular weight markers is shown on the left in kDa.
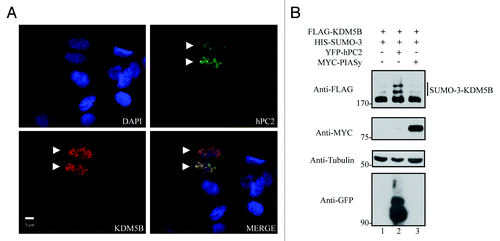
Lysines 242 and 278 are the major SUMOylation sites of KDM5B
A SUMOylation site prediction performed with SUMOspCitation44 suggested the existence of 4 putative SUMO-acceptor sites within the 1544 amino acid residues of human KDM5B. Among these predicted sites, lysines 242 and 278 received the highest scores. Interestingly, these residues are located within a ψKxE (ψ for an aliphatic amino acid) consensus SUMOylation motif and are conserved across different species of vertebrates (). Furthermore, K242 and K278 are contained within a region between the AT-rich interaction domain (ARID) and the first plant homeobox (PHD) domain of KDM5B (). Single mutations of K242 and K278 to arginine were introduced into KDM5B and the SUMOylation status of these mutants evaluated by immunoblotting with anti-FLAG antibodies using whole cell lysates from HEK293T cells co-expressing SUMO-3 and hPC2 (). hPC2 was expressed to increase the amount of SUMOylated KDM5B and enhance the sensitivity of the SUMOylation assays. Mutation of K242 led to the loss of the heaviest form of SUMOylated KDM5B modified by SUMO-3 (, top panel, lane 2). Analysis of the K278R mutant also resulted in the disappearance of the heaviest SUMOylated form of KDM5B (, top panel, lane 3). To assess the contribution of both K242 and K287 residues to the levels of SUMOylated KDM5B, concomitant mutations of these residues to arginine (2KR) were evaluated. Strikingly, these mutations resulted in the loss of all SUMOylated forms of KDM5B (, top panel, lane 4).
Figure 3. Mapping of the SUMO-acceptor sites of KDM5B. (A) Diagram depicting the distribution of the protein domains found in human KDM5B and the localization of two putative SUMOylation sites, K242, and K278. Clustal W amino acid sequence alignment of KDM5B from human (Homo sapiens; NP_006609), mouse (Mus musculus; NP_690855), chicken (Gallus gallus; NP_001026200), frog (Xenopus tropicalis; XP_002939684), and fish (Danio rerio; Q6IQX0) is depicted. The evolutionarily conserved putative SUMO-acceptor sites K242 and K278 are represented in bold. The abbreviations are JmjN (Jumonji N) domain; ARID (AT-rich interaction domain); PHD (plant homeobox domain), JmjC (Jumonji C) domain; and C5HC2 (Zinc finger domain). (B) KDM5B is SUMOylated by SUMO-3 at residues K242 and K278. Whole cell lysates obtained from HEK293T cells expressing HIS-SUMO-3, YFP-hPC2 and FLAG-KDM5BWT (wild type) or mutants K242R, K278R, and 2KR (K242R and K278R) were immunoblotted with the indicated antibodies. Asterisks (*) and (**) indicate high molecular weight SUMOylated proteins and non-conjugated SUMO-3, respectively.
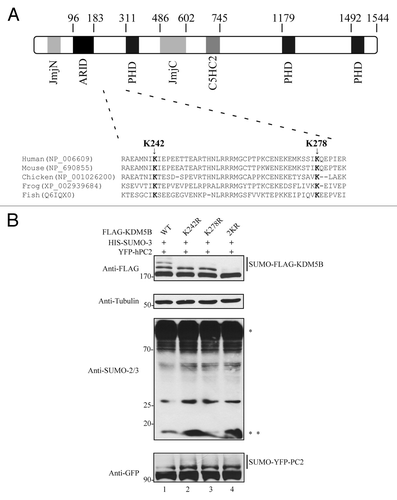
In addition, the SUMOylation-deficient mutant KDM5B (KDM5B2KR) was co-expressed with SUMO-3 in the presence or absence of hPC2. Immunoblotting analysis of total cell lysates revealed that KDM5B2KR could no longer be SUMOylated in both conditions. Only one band corresponding to unSUMOylated KDM5B was observed following immunoblotting with anti-FLAG (Fig. S2). These results show that lysine residues 242 and 278 are the sites of conjugation by SUMO-3 and correspond to the major predicted SUMO-acceptor sites of KDM5B. We assessed whether the lack of SUMOylation of KDM5B2KR impaired localization and interaction with hPC2. We observed that KDM5B2KR maintained its ability to co-localize and physically interact with hPC2 in the nucleus as observed with wild type KDM5B (Fig. S3).
Levels of SUMOylated KDM5B are dynamically regulated
Since KDM5B is known to regulate cell cycle progression,Citation8,Citation9 we examined whether KDM5B protein levels were altered during the phases of the cell cycle. Mouse embryonic fibroblasts (MEFs) and U2OS cells were left asynchronous or arrested in mitosis using nocodazole and whole cell lysates were immunoblotted for KDM5B. Endogenous KDM5B was upregulated in mitosis in both cell lines, suggesting that its protein levels are indeed cell cycle regulated (). Next, we assessed whether levels of ectopically expressed KDM5B were also cell cycle regulated. To this end, HEK293T cells transfected with FLAG-KDM5B were left asynchronous or arrested in mitosis with nocodazole and in S phase with hydroxyurea. Interestingly, the amount of exogenous FLAG-KDM5B was significantly increased in cells arrested with either nocodazole or hydroxyurea (). We next examined whether the levels of SUMOylated KDM5B following cell cycle arrest were upregulated. Cells transfected with KDM5B and expression vectors used to induce SUMOylation were arrested with nocodazole or hydroxyurea. Total cell extracts from these cells, immunoblotted with anti-FLAG antibodies, revealed that the levels of SUMO-3-modified KDM5B were increased in cells arrested at G2/M (nocodazole) or G1/S (hydroxyurea, ). To quantify the increase in SUMOylation vs. the increase in KDM5B per se, cells expressing KDM5B, SUMO-3, and hPC2 were arrested in S phase with hydroxyurea and then released to allow their progression. Cell lysates were prepared for up to 8 h after the release and we quantified the ratio of SUMO/unSUMO-KDM5B to ensure that the increase was not due to an increase in KDM5B protein levels per se. Indeed, we observed an increase, albeit, modest in SUMOylated KDM5B, as the cells progressed from the S phase (). As a control for appropriate cell cycle progression toward the G2/M phase, we performed immunoblots with anti-cyclin B1 antibodies and tubulin for equivalent loading.
Figure 4. SUMOylated and unSUMOylated KDM5B protein levels are regulated along the cell cycle. (A) KDM5B protein levels are upregulated in mitotic cells. MEF and U2OS cells were arrested at the G2/M phase with nocodazole for 22 h or not (asynchronous) and total cell lysates subjected to immunoblotting with anti-KDM5B and anti-Tubulin (loading control) antibodies. Relative KDM5B protein amounts (bottom of KDM5B immunoblots) were calculated based on band intensities quantified using ImageJCitation45 and normalized to Tubulin levels. (B) HEK293T cells ectopically expressing FLAG-KDM5B were arrested or not (asynchronous) at the G2/M and G1/S phase following 22 h of treatment with nocodazole or hydroxyurea, respectively. Whole cell lysates were analyzed by immunoblotting with anti-FLAG and anti-Tubulin (loading control) antibodies. Relative KDM5B protein amounts (bottom of FLAG immunoblot) were calculated based on band intensities quantified using ImageJCitation45 and normalized with Tubulin. (C) Levels of SUMOylated KDM5B are increased in cells arrested at the G2/M and G1/S phases. HEK293T cells were transfected with expression vectors encoding FLAG-KDM5B, HIS-SUMO-3, and YFP-hPC2. Cells were arrested or not (asynchronous) at the G2/M (nocodazole) and G1/S (hydroxyurea) phase. Immunoblots were performed using total cell lysates and antibodies against FLAG and Tubulin (loading control). (D) SUMOylation of KDM5B is regulated during the cell cycle. HEK293T cells expressing KDM5B, SUMO-3, and hPC2 were arrested at the G1/S phase by treatment with hydroxyurea for 22 h. Subsequently, cells were released in fresh culture medium to allow transition to G2/M. Whole cell lysates were then collected every 2 h for a period of 8 h. Levels of SUMOylated and unSUMOylated KDM5B were detected by immunoblotting with anti-FLAG antibodies. Cell cycle progression was monitored by immunoblotting with anti-Cyclin B1 antibodies. The ratio between the amounts of SUMOylated vs. unSUMOylated KDM5B (bottom of FLAG immunoblot) was calculated based on band intensities quantified using ImageJCitation45 and normalized with Tubulin. (E) RNF4 promotes degradation of KDM5B. HEK293T cells were transfected with FLAG-KDM5B and HIS-SUMO-3 plasmids in the absence or presence of increasing amounts of an expression vector encoding FLAG-RNF4. Total cell lysates were immunoblotted with anti-FLAG antibodies to detect KDM5B or RNF4, as indicated. The migration of FLAG-KDM5B and FLAG-RNF4 is shown on the right. The antibodies used are indicated on the left with the migration of the molecular mass markers in kDa. (F) Degradation of KDM5B by RNF-4 is mediated by the proteasomal pathway. HEK293T cells expressing FLAG-KDM5B in the presence or absence of FLAG-RNF4 were treated or not with MG132 for 6 h. Total cell lysates were immunoblotted with the antibodies indicated on the left. The migration of FLAG-KDM5B and FLAG-RNF4 is shown on the right. The antibodies used are indicated on the left with the migration of the molecular mass markers in kDa.
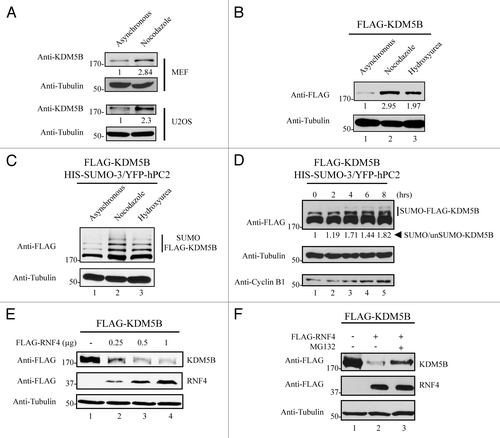
The SUMO binding protein, RNF4, has been shown to mediate ubiquitination and proteasome-dependent degradation of several proteins upon their SUMOylation.Citation46-Citation50 To evaluate whether KDM5B protein levels could be regulated by RNF4, a SUMO-targeted E3 ubiquitin ligase, HEK293T cells were transfected with vectors encoding KDM5B and SUMO-3 in the absence or presence of increasing RNF4. Indeed, immunoblotting analysis of total cell extracts from these cells revealed that enhanced expression of RNF4 was able to promote degradation of KDM5B in a dose-responsive manner (, upper panel). The reduced KDM5B levels observed in the presence of RNF4 was mediated by the proteasome degradation pathway, since treatment with the proteasome inhibitor, MG132, significantly elevated the levels of KDM5B (, lanes 2 and 3). Taken together, these findings indicate that KDM5B protein levels are cell cycle modulated and regulated by RNF4.
SUMOylation modulates the occupancy of KDM5B at target genes, but not its histone demethylase activity
To evaluate whether the histone lysine demethylase activity of KDM5B is regulated by SUMOylation, we compared the demethylase activity of wild type KDM5B and KDM5B2KR proteins. U2OS cells transfected with KDM5B or KDM5B2KR were immunostained with anti-H3K4me3 antibodies. Cells expressing FLAG-KDM5B or FLAG-KDM5B2KR (red) presented a reduction in the levels of H3K4me3 (green), indicating that SUMOylation of KDM5B does not affect its global histone demethylase activity (Fig. S4).
Furthermore, we evaluated the ability of KDM5B or KDM5B2KR to occupy certain of its known target genes including STARD4, miR-1792, RB1CC1, and BMI-1.Citation42,Citation51,Citation52 HEK293T were transfected with eGFP fusion versions of KDM5B and KDM5B2KR along with HIS-SUMO-3 and FLAG-hPC2 to ensure high levels of wild type KDM5B SUMOylation. As expected, SUMOylation of KDM5B-eGFP was readily observed in these samples and was severely impaired with the KDM5B2KR-eGFP (). The KDM5B2KR-eGFP protein maintains some residual SUMOylation in comparison to FLAG-KDM5B2KR, due to some SUMOylation on the eGFP moiety (Fig. S6), nevertheless KDM5B2KR-eGFP permits us to specifically assess the SUMOylation at K242 and K278 and this protein is more efficiently recognized in chromatin immunoprecipitations (ChIP) assays than the FLAG-tagged KDM5B (data not shown).
Figure 5. SUMOylation modulates the occupancy of KDM5B at target genes. (A) ChIP assays were performed using HEK293T transfected with vectors encoding HIS-SUMO-3, YFP-hPC2, and KDM5BWT-eGFP or KDM5B2KR-eGFP. Equivalent expression of KDM5B-eGFP proteins was examined by immunoblotting total cell lysates with the indicated antibodies. Slower migrating forms representing SUMOylated KDM5B were more abundant in the WT vs. the 2KR mutant. (B) HEK293T cells expressing SUMO-3, hPC2, and KDM5BWT-eGFP or KDM5B2KR-eGFP were subjected to chromatin immunoprecipitations with antibodies against GFP or control IgG. The occupancy of KDM5BWT-eGFP or KDM5B2KR-eGFP at the promoter regions of the genes STARD4, miR-1792, RB1CC1, and BMI-1 was evaluated by real-time qPCR. Error bars represent ± 1 SD of triplicate qPCR reactions. The asterisk (*) indicates p < 0.05 (Student’s t-test). (C) ChIP assays were performed with antibodies against histone 3 (H3), H3K4me3, and immunoglobulin G using HEK293T cells expressing SUMO-3, hPC2, and KDM5BWT-eGFP or KDM5B2KR-eGFP. The ChIP assays were normalized to H3 levels. Relative levels of trimethylated H3K4 present at the promoter regions of the genes STARD4, miR-1792, RB1CC1, and BMI-1 were evaluated by real-time qPCR. Error bars represent ± 1 SD of triplicate qPCR reactions. The asterisk (*) indicates p < 0.05 (Student’s t-test). (D) HEK293T cells were co-transfected with vectors encoding HIS-SUMO-3 and FLAG-hPC2 (Control) or in combination with plasmids expressing KDM5BWT-eGFP (WT) or KDM5B2KR-eGFP (2KR). Total RNA was isolated, reverse transcribed and mRNA expression levels of STARD4, RB1CC1 and BMI-1 were evaluated by qPCR. Results were normalized according to the expression levels of the house keeping genes GAPDH and CLTC. Error bars represent ± 1 SD of triplicate qPCR reactions. The asterisk (*) indicates p < 0.05 (Student’s t-test).
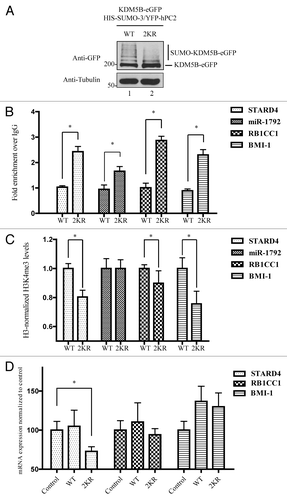
We performed ChIP assays under conditions of hyper-SUMOylation in the presence of SUMO-3 and hPC2. Under these conditions, wild type KDM5B-eGFP had little to no binding at the promoter region of the STARD4, miR-1792, RB1CC1, and BMI-1 genes, however KDM5B2KR was significantly enriched (). These findings suggested that SUMOylation at K242 and K278 prevents promoter occupancy and the lack of SUMOylation de-represses this effect. In addition, ChIP analysis using antibodies against histone 3 (H3) and H3K4me3 demonstrated significantly lower levels of H3K4me3 at three (STARD4, RB1CC1, BMI-1) out of the four promoter regions analyzed (), consistent with the presence of active KDM5B2KR. Subsequently, we examined whether this change in H3K4me3 levels resulted in reduced gene expression of these target genes. Indeed, we observed that cells expressing KDM5B2KR had significantly lower levels of STARD4 mRNA () when compared with cells expressing only HIS-SUMO-3 and FLAG-hPC2 (control). We detected similar mRNA levels of RB1CC1 and BMI-1 in cells expressing KDM5BWT (WT) or KDM5B2KR (2KR) suggesting that expression of these genes is likely to be controlled by additional factors. These data suggest that SUMOylation of residues K242 and K278 is a mechanism that controls expression of specific genes by negatively modulating KDM5B gene occupancy.
The SUMOylation-deficient KDM5B regulates the expression of a unique set of genes
To identify additional genes differentially regulated by the SUMOylation-deficient KDM5B protein, we transfected HEK293T cells with plasmids expressing KDM5B-eGFP or KDM5B2KR-eGFP along with HIS-SUMO-3 and FLAG-hPC2 to ensure maximal KDM5B SUMOylation. Equivalent expression of KDM5BWT- and KDM5B2KR-eGFP across all three biological replicates was assessed by immunoblotting total cell lysates with anti-GFP antibodies (). Total RNA isolated from these cells expressing wild type or KDM5B2KR with HIS-SUMO-3 and FLAG-hPC2 and their RNA profiles were compared with control cells expressing HIS-SUMO-3 and FLAG-hPC2 alone. The expression of 230 cancer-related human genes was assessed using an nCounter Human Cancer Reference Kit by NanoString Technologies. The quantitative transcriptional profile identified a significant (p < 0.05, the Student t test, unequal variance) alteration in the levels of 52 genes that were repressed and 6 genes that were upregulated equally in KDM5WT and KDM5B2KR cells (Table S1). Interestingly, 13 genes were significantly downregulated in cells expressing KDM5B2KR, but not in KDM5B (; Fig. S5). Of these 13 genes, the decreased expression of PPARG, MCL1, CDKN2B, TIMP1, and TIMP2 was shown previously by others to be regulated by KDM5B.Citation6,Citation43 To further support our data, we measured the levels of the active mark H3K4me3 at the promoter region of a subset of these genes. Indeed, we confirmed that TYRO3, TIMP2, CDKN2B, ERCC4, and XRCC5 are indeed KDM5B targets as their gene promoter regions had reduced H3K4me3 levels in cells ectopically expressing KDM5B ().
Figure 6. SUMOylation-deficient KDM5B regulates the expression of a unique set of genes. (A) HEK293T cells were co-transfected with vectors encoding HIS-SUMO-3 and FLAG-hPC2 in combination with plasmids expressing KDM5BWT-eGFP or KDM5B2KR-eGFP. Immunoblotting of total cell lysates with anti-GFP and anti-Tubulin antibodies revealed similar levels of KDM5BWT-eGFP and KDM5B2KR-eGFP across the triplicate transfections used for the digital gene expression analysis. (B) Total RNA isolated from biological triplicates of HEK293T cells transfected with vectors encoding for HIS-SUMO-3 and FLAG-hPC2 (Control) or in combination with plasmids expressing KDM5BWT-eGFP (WT) or KDM5B2KR-eGFP (2KR) was subjected to digital gene expression analysis (NanoString). The bar graph depicts the mRNA expression levels of 5 out of 12 genes found to be significantly downregulated only in cells expressing KDM5B2KR-eGFP. The asterisk (*) indicates p < 0.05 (Student’s t-test). (C) Levels of H3K4me3 at the promoter region of the genes illustrated in panel B were assessed in mock (Control) or KDM5B-eGFP-transfected HEK293T cells. ChIP assays were performed with antibodies against histone 3 (H3), H3K4me3, and immunoglobulin G. The asterisk (*) indicates p < 0.05 (the Student t-test).
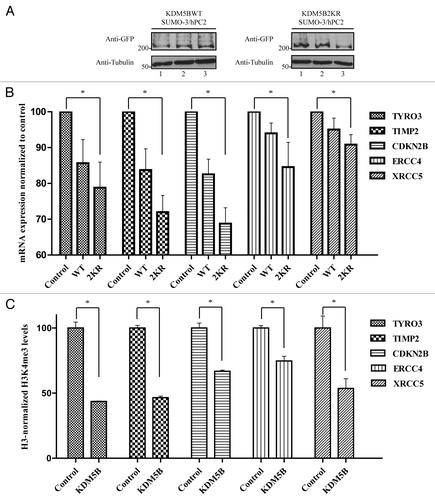
We noted that a set of genes were repressed by both KDM5B and KDM5B2KR and these include RAF1, TFE3, DAP3, CDK6, and FGF2 identified by Li et al.;Citation43 IGFBP2, TOP2A, and CDC2 identified by Scibetta et al.;Citation53 CDH1 identified by Schmitz et al.;Citation7 GADD45A identified by Hao et al.;Citation54 FGFR3, FGFR4, YY1, JUN, CCND1, and CCND2 identified by Xie et al. Noteworthy, the quantitative transcriptional profile did not display genes repressed only by KDM5B (Table S1). These findings suggest that SUMOylation of KDM5B may provide a higher order of gene regulation by modulating its occupancy at target genes.
Discussion
In the present manuscript, we report that SUMO conjugation of the histone lysine demethylase KDM5B negatively regulates its occupancy at target genes and, consequently, affects the expression of certain of its target genes. KDM5B associates with the hPC2, a SUMO E3 ligase, and specifically enhances SUMOylation of KDM5B. We observe that the levels of SUMO-KDM5B are cell cycle regulated and that the SUMO-dependent ubiquitin ligase RNF4 is able to promote degradation of KDM5B. These data suggest that the SUMOylation may regulate gene expression by modulating the KDM5B protein levels and/or its occupancy at target genes.
Of the genes downregulated by KDM5B, we identified known cell cycle regulators such as CDK6, CDC2, CCND1, and CCND2 (Table S1). This is consistent with the role of KDM5B in regulating cell cycle progression.Citation8,Citation9 Interestingly, we observed the regulation of KDM5B protein levels and its SUMOylated form during the cell cycle with peak levels in the G2/M phase. Notably, other SUMOylated proteins such as BubR1Citation55 and the transcription factor Sp1Citation56 have also been shown to display increased SUMOylation during cell division. As we demonstrate that SUMOylation negatively regulates KDM5B gene occupancy and protein levels, the oscillating levels of SUMOylated KDM5B throughout the cell cycle, suggest that conjugation of SUMO to KDM5B might influence the expression of genes involved in cell cycle progression.
SUMOylation-deficient KDM5B was also capable of downregulating the expression of a unique set of genes, as shown using the Human Cancer Reference kit of 230 genes (NanoString Technologies). We observed a decreased expression of KDM5B-regulated genes previously identified by others such as STARD4, PPARG, MCL1, CDKN2B, TIMP1, and TIMP2.Citation6,Citation43 We confirmed using ChIP assays that a corresponding decrease in the H3K4me3 activation mark was observed for STARD4 in cells expressing SUMOylation-deficient KDM5B. We also observed a decrease in the H3K4me3 activation mark at the miR-1792, RB1CC1, and BMI-1, but a corresponding decrease in mRNA expression was not observed.
We suspect that not all KDM5B-mediated lowering of H3K4me3 levels translate into reduced transcription and this is consistent with previous studies that observed a discrepancy between KDM5B occupancy and expression of its target genes.Citation7,Citation49 In fact, only 3.4% of KDM5B target genes were found to be differentially expressed following its depletion in embryonic stem cells.Citation7 In our analysis, we also identified a new set of KDM5B targets that are SUMO-regulated. These include TYRO3, ERCC4, XRCC5, XPC, MET, BRAF, ABL1, and BCL2. We also confirmed the decreased H3K4me3 on the gene promoters of TYRO3, ERCC4, and XRCC5. Most of these KDM5B targets are related to DNA damage signaling, suggesting that KDM5B may regulate genes required for DNA repair.
KDM5B has been shown to interact with hPC2,Citation33 a member of the Polycomb group of proteins and a known SUMO E3 ligase.Citation35 The expression of hPC2 enhanced the SUMOylation of KDM5B at evolutionarily conserved lysine residues 242 and 278. Moreover, we observe that hPC2 co-localizes in discrete nuclear foci and associates by co-immunoprecipitation assays with KDM5B. The SUMO-deficient protein, KDM5B2KR, retained its association with hPC2 showing that SUMOylation does not interfere with hPC2 interaction. Moreover, KDM5B2KR also retained full histone demethylase activity. These findings suggest that hPC2 is the SUMO E3 ligase that mediates KDM5B SUMOylation and that SUMOylation does not interfere with known KDM5B protein-protein interactions or its demethylase activity.
SUMOylation has been previously shown to negatively modulate the gene occupancy of other transcription factors such as PPARγCitation57 and SCM.Citation58 We observed that the SUMO-deficient KDM5B occupied target gene promoters leading to the demethylation of H3K4me3 resulting in gene silencing. SUMOylation may affect the KDM5B protein levels and/or its occupancy on chromatin. KDM5B was regulated by RNF4 via proteasome-dependent degradation. RNF4 regulates the levels of several proteins upon their SUMOylation.Citation46-Citation50 Our findings suggest the existence of an RNF4-dependent mechanism responsible for modulating the levels of KDM5B at target genes. Interestingly, RNF4 has been shown to stimulate gene transcription by promoting DNA demethylation.Citation59 This activity was dependent on the presence of functional RING finger and SUMO interacting motifs within RNF4 suggesting that SUMOylation of an unknown substrate may play a role in this mechanism. RNF4 may enhance the expression of KDM5B target genes by also stimulating concomitant DNA demethylation.
The ARID domain (residues 96 to 183) of KDM5B is known to mediate specific-DNA interactionsCitation53 and the first PHD domain of KDM5C was shown to interact with H3K9me3.Citation1 Interestingly, the PHD domain of KDM5C is ~78% similar to the first PHD domain of KDM5B (residues 311 to 356). Since the sites of SUMOylation are located between the ARID and PHD domains, SUMOylation of KDM5B may negatively affect its association with certain target genes by preventing its interaction with chromatin.
Along with our study showing that SUMOylation can negatively regulate the function of KDM5B, a few other groups have demonstrated mechanisms that also contribute to the regulation of KDM5B. For instance, the forkhead box P3 (FOXP3) protein was shown to competitively displace KDM5B from specific loci.Citation42 Moreover, PARylation of KDM5B mediated by PARP-1 was shown to inhibit the enzymatic activity of KDM5B and prevent its association with promoters of genes positively regulated by PARP-1.Citation60
In conclusion, our findings establish a link between SUMOylation and histone lysine demethylases by demonstrating that SUMO-conjugation of KDM5B regulates its occupancy at target genes. We have also observed that KDM5C is SUMOylated in vivo, suggesting that SUMOylation may regulate other histone lysine demethylases.
Materials and Methods
Cell culture and transfections
HEK293T, U2OS, MCF-7 cells, and mouse embryo fibroblasts (MEFs) were maintained in high glucose Dulbecco’s modified Eagle’s medium supplemented with 10% fetal bovine serum (FBS), 100 U/mL penicillin, 100 µg/mL streptomycin, and 1 mM sodium pyruvate. HEK293T and U2OS cells were transfected by calcium phosphate precipitation method and protein expression evaluated 48 h after transfection. Alternatively, HEK293T cells used for chromatin immunoprecipitation (ChIP) were transfected with Lipofectamine 2000 (Invitrogen) following the manufacturer’s instructions. To arrest cells at the G2/M or G1/S phases of the cell cycle, HEK293T, U2OS, and MEFs were treated with nocodazole (Sigma; 100 ng/mL) or hydroxyurea (Sigma; 1 mM) for 22 h.
Mammalian expression vectors
KDM5B/JARID1B tagged with an N-terminal FLAG tag was expressed from the vector pEV-FLAG-KDM5BWT and KDM5C tagged with MYC was expressed from pCS3+-SMCX-6MYC.Citation61 Both plasmids were kindly provided by Ralf Janknecht (University of Oklahoma Health Sciences Center). FLAG-KDM5D was expressed from the vector pFLAG-CMV2JARID1DCitation62 and was kindly obtained from Ramin Shiekhattar (The Wistar Institute). Mutations of lysines 242 and 278 to arginines were introduced in the KDM5B/JARID1B cDNA by performing site directed mutagenesis using primers K242R forward 5′-GAGCAGAGGC CATGAATATT AGAATAGAAC CCGAGGA-3′, K242R-reverse 5′-TCCTCGGGTT CTATTCTAAT ATTCATGGCC TCTGCTC-3′ and K278R-forward 5′-GAAAGAAATG AAGAGTAGCA TCAGGCAAGA ACCTATTGAG AG-3′, K278R-reverse 5′-CTCTCAATAG GTTCTTGCCT GATGCTACTC TTCATTTCTT TC-3′, creating the vectors pEV-FLAG-KDM5BK242R and pEV-FLAG-KDM5BK278R. KDM5B2KR was generated by replacing lysine 278 to arginine with the above primers and pEV-FLAG-KDM5BK242R as template. HIS-tagged SUMOs were expressed from plasmids pcDNA3-His6SUMO1WT and pcDNA3-HIS 6SUMO3WTCitation63 a kind gift from Pierre Thibault (Institut de Recherche en Immunologie et Cancérologie, Université de Montréal). The bicistronic plasmid Dual S1/I/UCitation64 was kindly provided by German Rosas-Acosta (University of Texas at El Paso, El Paso, TX, USA) and was used to drive the simultaneous expression of HIS-SUMO-1 and HA-UBC9. FLAG-hPC2 and YFP-hPC2 were expressed from the plasmids pCMV5-FLAG-Pc2 and pCS2-YFP-hPC2,Citation35 kindly provided by David Wotton (University of Virginia). Human RNF4 was expressed from the pFLAGRNF4 vector kindly provided by Muriel Aubry (Université de Montréal). Plasmids pKDM5BWT-eGFP and pKDM5B2KR-eGFP were created by PCR amplification of the KDM5B cDNA from pEV-FLAG-KDM5BWT and pEV-FLAG-KDM5B2KR using primers FW 5′-TATAGGATCC ACCATGGGGT CCGAGGCGGC CACCACACTG CA-3′ and REV- 5′-TATAGTCGAC GATGACTTTC GGCTTGGTGC GT-3′ and cloned in-frame into pEGFP-N1 within the BglII and SalI sites.
Immunoblotting, immunoprecipitations and antibodies
Immunoblotting, immunofluorescence, and ChIP assays were performed using the following antibodies: mouse monoclonal JARID1B (Abnova, M02A), rabbit polyclonal JARID1B (Abcam, ab50958), mouse monoclonal FLAG (Sigma), mouse monoclonal SUMO1 (Invitrogen, 33-2400), rabbit polyclonal Cyclin B1 (Santa Cruz, Sc-752), rabbit polyclonal His-probe (Santa Cruz, Sc-803), rabbit polyclonal SUMO2/3 (Calbiochem, ST1135), mouse monoclonal Tubulin (Sigma, T5168), rabbit polyclonal GFP (Abcam, Ab290), rabbit monoclonal H3 (Abcam, Ab1791), and rabbit monoclonal H3K4me3 (Millipore, 17-614). For immunoprecipitations, HEK293T cells were lysed in lysis buffer (1% Triton-X100, 150 mM NaCl, 20 mM Tris-HCl pH 8.0) or RIPA buffer (50 mM Tris-HCl pH 8.0, 150 mM NaCl, 0.5% sodium deoxycholate, 0.1% SDS, 1% NP-40) containing protease inhibitors (Protease inhibitor cocktail, Roche) 48 h after transfection. Lysates were initially incubated with specific antibodies for 2 h on ice and rotated at 4 °C with a mixture of protein A and G Sepharose beads for 30 min. The beads were then washed 3 times with lysis or RIPA buffer and 2 times with phosphate-buffered saline solution (PBS). Bound proteins were eluted in 2× Laemmli buffer and further analyzed by immunoblotting.
Immunofluorescence
U2OS cells were plated on glass coverslips placed in 6-well plates, transfected by calcium phosphate precipitation and fixed 48 h later in 4% paraformaldehyde for 10 min. Cells were then washed twice with PBS and permeabilized for 15 min in 0.5% Triton-X100 in PBS. Coverslips were washed 3 times with PBS, incubated in blocking solution (10% FBS in PBS), and further incubated with primary antibodies diluted in antibody dilution buffer (5% FBS in PBS) for 1 h. Following incubation, coverslips were washed 3 times with PBS and incubated with secondary antibodies in antibody dilution buffer for 45 min. Cells were washed 3 times with PBS and mounted on glass slides with mounting solution containing 4'-6-Diamidino-2-phenylindole (DAPI) at 1µg/mL. Images were obtained using a Zeiss M1 fluorescence microscope.
ChIP and qPCR
HEK293T cells transfected with plasmids pCMV5-FLAG-Pc2 and pcDNA3-HIS6SUMO3WT in combination with expression vectors pKDM5BWT-eGFP or pKDM5B2KR-eGFP were trypsinized and crosslinked with 1% paraformaldehyde for 10 min at room temperature. Fixed samples were quenched for 5 min with 125 mM glycine. Cell pellets were washed twice with PBS, resuspended in swelling buffer (100 mM Tris pH 7.6, 10 mM KAc, 15 mM MgAc, 1% IGEPAL, supplemented with protease inhibitors), and incubated on ice for 10 min. Nuclei were released by passing resuspended cells 10 times through a 1 ml insulin syringe. Released nuclei were then centrifuged for 5 min at 4 °C and resuspended in nuclei lysis buffer (50 mM Tris-Cl pH 8.0, 10 mM EDTA, 1% SDS, supplemented with protease inhibitors). Following 10 min of incubation on ice, samples were sonicated 4 times for 10 s at 50% duty. Samples were centrifuged for 20 min at 4 °C 16 000 × g and chromatin precleared with blocked protein A Sepharose by rotating samples for 15 min at 4 °C. Pre-cleared samples were then diluted in dilution buffer (16.7 mM Tris-Cl pH 8.0, 0.01% SDS, 1.1% Triton X-100, 1.2 mM EDTA, 167 mM NaCl, 1 mM PMSF). Ten percent aliquots were saved as inputs and the remaining sample was equally distributed into 1.5 ml tubes. Specific antibodies against histone H3, trimethylated H3K4, GFP or control IgG were added to individual samples and incubated overnight under rotation at 4°C. Next, previously blocked protein A Sepharose beads were added to immunocomplexes and rotated for 15 min at room temperature. Beads were then washed twice in dialysis buffer (50 mM Tris-Cl pH 8.0, 2 mM EDTA, 0.2% Sarkosyl) and 4 times in wash buffer (100 mM Tris-Cl pH 9.0, 500 mM LiCl, 1% IGEPAL, 1% sodium deoxycholate, 1 mM PMSF). Elution was then performed by resuspending samples in elution buffer (50 mM NaHCO3, 1% SDS) followed by rigorous shaking for 15 min. After two rounds of elution, NaCl was added to a final concentration of 250 mM and samples were boiled for 15 min. Contaminant RNA was digested with RNase A for 30 min at 37þC and the DNA was subsequently purified using PCR purification spin columns (Qiagen). Samples were used for quantitative real-time PCR (qRT-PCR) reactions using SsoFAST Evagreen supermix (Bio-Rad) and a 7500 Fast Real-Time PCR system (Applied Biosystems). The primers used to analyze the occupancy of KDM5B at the promoter regions of BMI-1,Citation51 mir-1792,Citation52 STARD4,Citation42 and RB1CC1Citation42 have been previously described.
Quantitative RT-PCR and digital gene expression analysis
HEK293T cells plated on 6-well plates were transfected with vectors pCMV5-FLAG-Pc2 and pcDNA3-HIS6SUMO3WT or in combination with pKDM5BWT-eGFP or pKDM5B2KR-eGFP in triplicates. Cells were harvested 48 h after transfection and separated in two aliquots. One aliquot was lysed in 2× Laemmli buffer and further analyzed by immunoblotting with mouse anti-GFP (Roche, 11 814 460 001) to access expression of KDM5B-eGFP fusion proteins. The remaining aliquot was used for extraction of total RNA with Trizol (Invitrogen) according to the manufacturer’s protocol. For quantitative RT-PCR, total RNA was reverse transcribed using Super Script II (Invitrogen) and then used for quantitative PCR analysis with specific primers for BMI-1(forward 5′-CGCTTGGCTC GCATTCATTT-3′ and reverse 5′-AAATCCCGGA AAGAGCAGCC-3′), STARD4 (forward 5′-GCGAGTTGCT AAGAAAACGA AAG-3′ and reverse 5′-CAATCCAAAC GACAAGGCCC-3′) and RB1CC1 (forward 5′-TGGATCTCAA ACCAGGTGAG G-3′ and reverse 5′-ATCTGTTTTG TGCCTTTTTG GC-3′). The mRNA levels of the house keeping genes GAPDH (forward 5′-GACAGTCAGC CGCATCTTCT-3′ and reverse 5′-GCGCCCAATA CGACCAAATC-3′) and CLTC (forward 5′-ACGTTTGGCA TCTACCCTGG-3′ and reverse 5′-GCGAAGCAGA CCTCTTTCCA-3′) were used to normalize the expression of BMI-1, STARD4, and RB1CC1 across the different samples analyzed. All qRT-PCR reactions were performed with SsoFAST Evagreen supermix (Bio-Rad) and a 7500 Fast Real-Time PCR system (Applied Biosystems). Digital gene expression analysis was performed using the same total RNA samples used for the qRT-PCR reactions. The number of mRNA transcripts of 230 genes was evaluated using the nCounter analysis system (NanoString Technologies) and an nCounter Human Cancer Reference kit (NanoString Technologies). Transcript levels were normalized to the expression of the house keeping genes GAPDH and CLTC.
Purification of SUMO conjugates under denaturing condition
For the detection of endogenous SUMOylated KDM5B, 1.2 × 107 MCF-7 cells stably expressing or not HIS-SUMO-3 were lysed in Gu-HCl lysis buffer (6 M guanidine-HCl, 0.1 M Na2HPO4/NaH2PO4, 10 mM Tris-HCl, 10 mM imidazole, 1 mM β-Mercaptoethanol, pH 8.0). Lysates were sonicated 3 times and cleared by centrifugation (10 min, 16 000 × g). The supernatant was mixed with equilibrated Ni-NTA agarose (Qiagen) and incubated overnight at 4 °C under rotation. Subsequently, Ni-NTA agarose beads were washed once in Gu-HCl buffer and Urea wash buffer 1 (8 M Urea, 0.1 M Na2HPO4/NaH2PO4, 10 mM Tris-HCl, 10 mM imidazole, 1 mM β-Mercaptoethanol, pH 8.0) and twice in Urea wash buffer 2 (8 M Urea, 0.1 M Na2HPO4/NaH2PO4, 10 mM Tris-HCl, 10 mM imidazole, 1 mM β-Mercaptoethanol, 0.1% Triton X-100, pH 6.3). Bound proteins were then eluted by boiling beads in 2X SDS sample buffer (200 mM imidazole) for 5 min. Next, eluted proteins were further analyzed by immunoblotting. Analysis of SUMOylation of KDM5B in HEK293T cells ectopically expressing or not HIS-SUMO-3 and FLAG-KDM5B was evaluated by purifying SUMO conjugates following the same procedure.
Abbreviations: | ||
KDM5B | = | lysine-specific demethylase 5B |
H3K4me3 | = | histone H3 tri-methylated on lysine 4 |
hPC2 | = | human polycomb group protein 2 |
SUMO | = | small ubiquitin-like modifier |
RNF4 | = | ring finger protein 4 |
ChIP | = | chromatin immunoprecipitation |
Additional material
Download Zip (9.1 MB)Disclosure of Potential Conflicts of Interest
No potential conflicts of interest were disclosed.
Acknowledgments
We thank Pierre Thibault for helpful discussions and reagents. We also thank Gillian Vogel and Zhenbao Yu for proof-reading the manuscript. This work was supported by the Canadian Institute of Health Research MOP-67070 and MOP-93811. MB is funded by post-doctoral fellowships from McGill Integrated Cancer Research Training Program and Fonds de Recherche en Santé du Québec.
Supplemental Materials
Supplemental materials may be found here: www.landesbioscience.com/journals/epigenetics/article/26112
References
- Iwase S, Lan F, Bayliss P, de la Torre-Ubieta L, Huarte M, Qi HH, Whetstine JR, Bonni A, Roberts TM, Shi Y. The X-linked mental retardation gene SMCX/JARID1C defines a family of histone H3 lysine 4 demethylases. Cell 2007; 128:1077 - 88; http://dx.doi.org/10.1016/j.cell.2007.02.017; PMID: 17320160
- Christensen J, Agger K, Cloos PA, Pasini D, Rose S, Sennels L, Rappsilber J, Hansen KH, Salcini AE, Helin K. RBP2 belongs to a family of demethylases, specific for tri-and dimethylated lysine 4 on histone 3. Cell 2007; 128:1063 - 76; http://dx.doi.org/10.1016/j.cell.2007.02.003; PMID: 17320161
- Lu PJ, Sundquist K, Baeckstrom D, Poulsom R, Hanby A, Meier-Ewert S, Jones T, Mitchell M, Pitha-Rowe P, Freemont P, et al. A novel gene (PLU-1) containing highly conserved putative DNA/chromatin binding motifs is specifically up-regulated in breast cancer. J Biol Chem 1999; 274:15633 - 45; http://dx.doi.org/10.1074/jbc.274.22.15633; PMID: 10336460
- Xiang Y, Zhu Z, Han G, Ye X, Xu B, Peng Z, Ma Y, Yu Y, Lin H, Chen AP, et al. JARID1B is a histone H3 lysine 4 demethylase up-regulated in prostate cancer. Proc Natl Acad Sci U S A 2007; 104:19226 - 31; http://dx.doi.org/10.1073/pnas.0700735104; PMID: 18048344
- Seward DJ, Cubberley G, Kim S, Schonewald M, Zhang L, Tripet B, Bentley DL. Demethylation of trimethylated histone H3 Lys4 in vivo by JARID1 JmjC proteins. Nat Struct Mol Biol 2007; 14:240 - 2; http://dx.doi.org/10.1038/nsmb1200; PMID: 17310255
- Xie L, Pelz C, Wang W, Bashar A, Varlamova O, Shadle S, Impey S. KDM5B regulates embryonic stem cell self-renewal and represses cryptic intragenic transcription. EMBO J 2011; 30:1473 - 84; http://dx.doi.org/10.1038/emboj.2011.91; PMID: 21448134
- Schmitz SU, Albert M, Malatesta M, Morey L, Johansen JV, Bak M, Tommerup N, Abarrategui I, Helin K. Jarid1b targets genes regulating development and is involved in neural differentiation. EMBO J 2011; 30:4586 - 600; http://dx.doi.org/10.1038/emboj.2011.383; PMID: 22020125
- Yamane K, Tateishi K, Klose RJ, Fang J, Fabrizio LA, Erdjument-Bromage H, Taylor-Papadimitriou J, Tempst P, Zhang Y. PLU-1 is an H3K4 demethylase involved in transcriptional repression and breast cancer cell proliferation. Mol Cell 2007; 25:801 - 12; http://dx.doi.org/10.1016/j.molcel.2007.03.001; PMID: 17363312
- Mitra D, Das PM, Huynh FC, Jones FE. Jumonji/ARID1 B (JARID1B) protein promotes breast tumor cell cycle progression through epigenetic repression of microRNA let-7e. J Biol Chem 2011; 286:40531 - 5; http://dx.doi.org/10.1074/jbc.M111.304865; PMID: 21969366
- Wong PP, Miranda F, Chan KV, Berlato C, Hurst HC, Scibetta AG. Histone demethylase KDM5B collaborates with TFAP2C and Myc to repress the cell cycle inhibitor p21(cip) (CDKN1A). Mol Cell Biol 2012; 32:1633 - 44; http://dx.doi.org/10.1128/MCB.06373-11; PMID: 22371483
- Roesch A, Fukunaga-Kalabis M, Schmidt EC, Zabierowski SE, Brafford PA, Vultur A, Basu D, Gimotty P, Vogt T, Herlyn M. A temporarily distinct subpopulation of slow-cycling melanoma cells is required for continuous tumor growth. Cell 2010; 141:583 - 94; http://dx.doi.org/10.1016/j.cell.2010.04.020; PMID: 20478252
- Dey BK, Stalker L, Schnerch A, Bhatia M, Taylor-Papidimitriou J, Wynder C. The histone demethylase KDM5b/JARID1b plays a role in cell fate decisions by blocking terminal differentiation. Mol Cell Biol 2008; 28:5312 - 27; http://dx.doi.org/10.1128/MCB.00128-08; PMID: 18591252
- Chicas A, Kapoor A, Wang X, Aksoy O, Evertts AG, Zhang MQ, Garcia BA, Bernstein E, Lowe SW. H3K4 demethylation by Jarid1a and Jarid1b contributes to retinoblastoma-mediated gene silencing during cellular senescence. Proc Natl Acad Sci U S A 2012; 109:8971 - 6; http://dx.doi.org/10.1073/pnas.1119836109; PMID: 22615382
- Nijwening JH, Geutjes EJ, Bernards R, Beijersbergen RL. The histone demethylase Jarid1b (Kdm5b) is a novel component of the Rb pathway and associates with E2f-target genes in MEFs during senescence. PLoS One 2011; 6:e25235; http://dx.doi.org/10.1371/journal.pone.0025235; PMID: 21980403
- Van Rechem C, Black JC, Abbas T, Allen A, Rinehart CA, Yuan GC, Dutta A, Whetstine JR. The SKP1-Cul1-F-box and leucine-rich repeat protein 4 (SCF-FbxL4) ubiquitin ligase regulates lysine demethylase 4A (KDM4A)/Jumonji domain-containing 2A (JMJD2A) protein. J Biol Chem 2011; 286:30462 - 70; http://dx.doi.org/10.1074/jbc.M111.273508; PMID: 21757720
- Mallette FA, Mattiroli F, Cui G, Young LC, Hendzel MJ, Mer G, Sixma TK, Richard S. RNF8- and RNF168-dependent degradation of KDM4A/JMJD2A triggers 53BP1 recruitment to DNA damage sites. The EMBO journal 2012; 31:1865 - 78; http://dx.doi.org/10.4161/cc.20124; PMID: 22487685
- Mersman DP, Du HN, Fingerman IM, South PF, Briggs SD. Polyubiquitination of the demethylase Jhd2 controls histone methylation and gene expression. Genes Dev 2009; 23:951 - 62; http://dx.doi.org/10.1101/gad.1769209; PMID: 19346402
- Baba A, Ohtake F, Okuno Y, Yokota K, Okada M, Imai Y, Ni M, Meyer CA, Igarashi K, Kanno J, et al. PKA-dependent regulation of the histone lysine demethylase complex PHF2-ARID5B. Nat Cell Biol 2011; 13:668 - 75; http://dx.doi.org/10.1038/ncb2228; PMID: 21532585
- Geiss-Friedlander R, Melchior F. Concepts in sumoylation: a decade on. Nat Rev Mol Cell Biol 2007; 8:947 - 56; http://dx.doi.org/10.1038/nrm2293; PMID: 18000527
- Yeh ET. SUMOylation and De-SUMOylation: wrestling with life’s processes. J Biol Chem 2009; 284:8223 - 7; http://dx.doi.org/10.1074/jbc.R800050200; PMID: 19008217
- Muller S, Berger M, Lehembre F, Seeler JS, Haupt Y, Dejean A. c-Jun and p53 activity is modulated by SUMO-1 modification. J Biol Chem 2000; 275:13321 - 9; http://dx.doi.org/10.1074/jbc.275.18.13321; PMID: 10788439
- Bies J, Markus J, Wolff L. Covalent attachment of the SUMO-1 protein to the negative regulatory domain of the c-Myb transcription factor modifies its stability and transactivation capacity. J Biol Chem 2002; 277:8999 - 9009; http://dx.doi.org/10.1074/jbc.M110453200; PMID: 11779867
- Desterro JM, Rodriguez MS, Hay RT. SUMO-1 modification of IkappaBalpha inhibits NF-kappaB activation. Mol Cell 1998; 2:233 - 9; http://dx.doi.org/10.1016/S1097-2765(00)80133-1; PMID: 9734360
- Rodriguez MS, Desterro JM, Lain S, Midgley CA, Lane DP, Hay RT. SUMO-1 modification activates the transcriptional response of p53. EMBO J 1999; 18:6455 - 61; http://dx.doi.org/10.1093/emboj/18.22.6455; PMID: 10562557
- Gostissa M, Hengstermann A, Fogal V, Sandy P, Schwarz SE, Scheffner M, Del Sal G. Activation of p53 by conjugation to the ubiquitin-like protein SUMO-1. EMBO J 1999; 18:6462 - 71; http://dx.doi.org/10.1093/emboj/18.22.6462; PMID: 10562558
- Ross S, Best JL, Zon LI, Gill G. SUMO-1 modification represses Sp3 transcriptional activation and modulates its subnuclear localization. Mol Cell 2002; 10:831 - 42; http://dx.doi.org/10.1016/S1097-2765(02)00682-2; PMID: 12419227
- Goodson ML, Hong Y, Rogers R, Matunis MJ, Park-Sarge OK, Sarge KD. Sumo-1 modification regulates the DNA binding activity of heat shock transcription factor 2, a promyelocytic leukemia nuclear body associated transcription factor. J Biol Chem 2001; 276:18513 - 8; http://dx.doi.org/10.1074/jbc.M008066200; PMID: 11278381
- Hong Y, Rogers R, Matunis MJ, Mayhew CN, Goodson ML, Park-Sarge OK, Sarge KD. Regulation of heat shock transcription factor 1 by stress-induced SUMO-1 modification. J Biol Chem 2001; 276:40263 - 7; PMID: 11514557
- Stindt MH, Carter S, Vigneron AM, Ryan KM, Vousden KH. MDM2 promotes SUMO-2/3 modification of p53 to modulate transcriptional activity. Cell Cycle 2011; 10:3176 - 88; http://dx.doi.org/10.4161/cc.10.18.17436; PMID: 21900752
- Lin X, Sun B, Liang M, Liang YY, Gast A, Hildebrand J, Brunicardi FC, Melchior F, Feng XH. Opposed regulation of corepressor CtBP by SUMOylation and PDZ binding. Mol Cell 2003; 11:1389 - 96; http://dx.doi.org/10.1016/S1097-2765(03)00175-8; PMID: 12769861
- Girdwood D, Bumpass D, Vaughan OA, Thain A, Anderson LA, Snowden AW, Garcia-Wilson E, Perkins ND, Hay RT. P300 transcriptional repression is mediated by SUMO modification. Mol Cell 2003; 11:1043 - 54; http://dx.doi.org/10.1016/S1097-2765(03)00141-2; PMID: 12718889
- Vethantham V, Rao N, Manley JL. Sumoylation regulates multiple aspects of mammalian poly(A) polymerase function. Genes Dev 2008; 22:499 - 511; http://dx.doi.org/10.1101/gad.1628208; PMID: 18281463
- Zhou W, Chen H, Zhang L. The PcG protein hPc2 interacts with the N-terminus of histone demethylase JARID1B and acts as a transcriptional co-repressor. BMB Rep 2009; 42:154 - 9; http://dx.doi.org/10.5483/BMBRep.2009.42.3.154; PMID: 19336002
- MacPherson MJ, Beatty LG, Zhou W, Du M, Sadowski PD. The CTCF insulator protein is posttranslationally modified by SUMO. Mol Cell Biol 2009; 29:714 - 25; http://dx.doi.org/10.1128/MCB.00825-08; PMID: 19029252
- Kagey MH, Melhuish TA, Wotton D. The polycomb protein Pc2 is a SUMO E3. Cell 2003; 113:127 - 37; http://dx.doi.org/10.1016/S0092-8674(03)00159-4; PMID: 12679040
- Long J, Zuo D, Park M. Pc2-mediated sumoylation of Smad-interacting protein 1 attenuates transcriptional repression of E-cadherin. J Biol Chem 2005; 280:35477 - 89; http://dx.doi.org/10.1074/jbc.M504477200; PMID: 16061479
- Li B, Zhou J, Liu P, Hu J, Jin H, Shimono Y, Takahashi M, Xu G. Polycomb protein Cbx4 promotes SUMO modification of de novo DNA methyltransferase Dnmt3a. Biochem J 2007; 405:369 - 78; http://dx.doi.org/10.1042/BJ20061873; PMID: 17439403
- Roscic A, Möller A, Calzado MA, Renner F, Wimmer VC, Gresko E, Lüdi KS, Schmitz ML. Phosphorylation-dependent control of Pc2 SUMO E3 ligase activity by its substrate protein HIPK2. Mol Cell 2006; 24:77 - 89; http://dx.doi.org/10.1016/j.molcel.2006.08.004; PMID: 17018294
- Oh Y, Chung KC. Small ubiquitin-like modifier (SUMO) modification of zinc finger protein 131 potentiates its negative effect on estrogen signaling. J Biol Chem 2012; 287:17517 - 29; http://dx.doi.org/10.1074/jbc.M111.336354; PMID: 22467880
- Ismail IH, Gagné JP, Caron MC, McDonald D, Xu Z, Masson JY, Poirier GG, Hendzel MJ. CBX4-mediated SUMO modification regulates BMI1 recruitment at sites of DNA damage. Nucleic Acids Res 2012; 40:5497 - 510; http://dx.doi.org/10.1093/nar/gks222; PMID: 22402492
- Pelisch F, Pozzi B, Risso G, Muñoz MJ, Srebrow A. DNA damage-induced heterogeneous nuclear ribonucleoprotein K sumoylation regulates p53 transcriptional activation. J Biol Chem 2012; 287:30789 - 99; http://dx.doi.org/10.1074/jbc.M112.390120; PMID: 22825850
- Katoh H, Qin ZS, Liu R, Wang L, Li W, Li X, Wu L, Du Z, Lyons R, Liu CG, et al. FOXP3 orchestrates H4K16 acetylation and H3K4 trimethylation for activation of multiple genes by recruiting MOF and causing displacement of PLU-1. Mol Cell 2011; 44:770 - 84; http://dx.doi.org/10.1016/j.molcel.2011.10.012; PMID: 22152480
- Li Q, Shi L, Gui B, Yu W, Wang J, Zhang D, Han X, Yao Z, Shang Y. Binding of the JmjC demethylase JARID1B to LSD1/NuRD suppresses angiogenesis and metastasis in breast cancer cells by repressing chemokine CCL14. Cancer Res 2011; 71:6899 - 908; http://dx.doi.org/10.1158/0008-5472.CAN-11-1523; PMID: 21937684
- Ren J, Gao X, Jin C, Zhu M, Wang X, Shaw A, Wen L, Yao X, Xue Y. Systematic study of protein sumoylation: Development of a site-specific predictor of SUMOsp 2.0. Proteomics 2009; 9:3409 - 12; http://dx.doi.org/10.1002/pmic.200800646; PMID: 19504496
- Schneider CA, Rasband WS, Eliceiri KW. NIH Image to ImageJ: 25 years of image analysis. Nat Methods 2012; 9:671 - 5; http://dx.doi.org/10.1038/nmeth.2089; PMID: 22930834
- Lallemand-Breitenbach V, Jeanne M, Benhenda S, Nasr R, Lei M, Peres L, Zhou J, Zhu J, Raught B, de Thé H. Arsenic degrades PML or PML-RARalpha through a SUMO-triggered RNF4/ubiquitin-mediated pathway. Nat Cell Biol 2008; 10:547 - 55; http://dx.doi.org/10.1038/ncb1717; PMID: 18408733
- Tatham MH, Geoffroy MC, Shen L, Plechanovova A, Hattersley N, Jaffray EG, Palvimo JJ, Hay RT. RNF4 is a poly-SUMO-specific E3 ubiquitin ligase required for arsenic-induced PML degradation. Nat Cell Biol 2008; 10:538 - 46; http://dx.doi.org/10.1038/ncb1716; PMID: 18408734
- Weisshaar SR, Keusekotten K, Krause A, Horst C, Springer HM, Göttsche K, Dohmen RJ, Praefcke GJ. Arsenic trioxide stimulates SUMO-2/3 modification leading to RNF4-dependent proteolytic targeting of PML. FEBS Lett 2008; 582:3174 - 8; http://dx.doi.org/10.1016/j.febslet.2008.08.008; PMID: 18708055
- Martin N, Schwamborn K, Schreiber V, Werner A, Guillier C, Zhang XD, Bischof O, Seeler JS, Dejean A. PARP-1 transcriptional activity is regulated by sumoylation upon heat shock. EMBO J 2009; 28:3534 - 48; http://dx.doi.org/10.1038/emboj.2009.279; PMID: 19779455
- van Hagen M, Overmeer RM, Abolvardi SS, Vertegaal AC. RNF4 and VHL regulate the proteasomal degradation of SUMO-conjugated Hypoxia-Inducible Factor-2alpha. Nucleic Acids Res 2010; 38:1922 - 31; http://dx.doi.org/10.1093/nar/gkp1157; PMID: 20026589
- Luis NM, Morey L, Mejetta S, Pascual G, Janich P, Kuebler B, Cozutto L, Roma G, Nascimento E, Frye M, et al. Regulation of human epidermal stem cell proliferation and senescence requires polycomb- dependent and -independent functions of Cbx4. Cell Stem Cell 2011; 9:233 - 46; http://dx.doi.org/10.1016/j.stem.2011.07.013; PMID: 21885019
- Pospisil V, Vargova K, Kokavec J, Rybarova J, Savvulidi F, Jonasova A, Necas E, Zavadil J, Laslo P, Stopka T. Epigenetic silencing of the oncogenic miR-17-92 cluster during PU.1-directed macrophage differentiation. EMBO J 2011; 30:4450 - 64; http://dx.doi.org/10.1038/emboj.2011.317; PMID: 21897363
- Scibetta AG, Santangelo S, Coleman J, Hall D, Chaplin T, Copier J, Catchpole S, Burchell J, Taylor-Papadimitriou J. Functional analysis of the transcription repressor PLU-1/JARID1B. Mol Cell Biol 2007; 27:7220 - 35; http://dx.doi.org/10.1128/MCB.00274-07; PMID: 17709396
- Hao H, Kim DS, Klocke B, Johnson KR, Cui K, Gotoh N, Zang C, Gregorski J, Gieser L, Peng W, et al. Transcriptional regulation of rod photoreceptor homeostasis revealed by in vivo NRL targetome analysis. PLoS Genet 2012; 8:e1002649; http://dx.doi.org/10.1371/journal.pgen.1002649; PMID: 22511886
- Yang F, Hu L, Chen C, Yu J, O’Connell CB, Khodjakov A, Pagano M, Dai W. BubR1 is modified by sumoylation during mitotic progression. J Biol Chem 2012; 287:4875 - 82; http://dx.doi.org/10.1074/jbc.M111.318261; PMID: 22167194
- Wang YT, Yang WB, Chang WC, Hung JJ. Interplay of posttranslational modifications in Sp1 mediates Sp1 stability during cell cycle progression. J Mol Biol 2011; 414:1 - 14; http://dx.doi.org/10.1016/j.jmb.2011.09.027; PMID: 21983342
- Chung SS, Ahn BY, Kim M, Kho JH, Jung HS, Park KS. SUMO modification selectively regulates transcriptional activity of peroxisome-proliferator-activated receptor γ in C2C12 myotubes. Biochem J 2011; 433:155 - 61; http://dx.doi.org/10.1042/BJ20100749; PMID: 20950277
- Smith M, Mallin DR, Simon JA, Courey AJ. Small ubiquitin-like modifier (SUMO) conjugation impedes transcriptional silencing by the polycomb group repressor Sex Comb on Midleg. J Biol Chem 2011; 286:11391 - 400; http://dx.doi.org/10.1074/jbc.M110.214569; PMID: 21278366
- Hu XV, Rodrigues TM, Tao H, Baker RK, Miraglia L, Orth AP, Lyons GE, Schultz PG, Wu X. Identification of RING finger protein 4 (RNF4) as a modulator of DNA demethylation through a functional genomics screen. Proc Natl Acad Sci U S A 2010; 107:15087 - 92; http://dx.doi.org/10.1073/pnas.1009025107; PMID: 20696907
- Krishnakumar R, Kraus WL. PARP-1 regulates chromatin structure and transcription through a KDM5B-dependent pathway. Mol Cell 2010; 39:736 - 49; http://dx.doi.org/10.1016/j.molcel.2010.08.014; PMID: 20832725
- Kim J, Shin S, Subramaniam M, Bruinsma E, Kim TD, Hawse JR, Spelsberg TC, Janknecht R. Histone demethylase JARID1B/KDM5B is a corepressor of TIEG1/KLF10. Biochem Biophys Res Commun 2010; 401:412 - 6; http://dx.doi.org/10.1016/j.bbrc.2010.09.068; PMID: 20863814
- Lee MG, Norman J, Shilatifard A, Shiekhattar R. Physical and functional association of a trimethyl H3K4 demethylase and Ring6a/MBLR, a polycomb-like protein. Cell 2007; 128:877 - 87; http://dx.doi.org/10.1016/j.cell.2007.02.004; PMID: 17320162
- Galisson F, Mahrouche L, Courcelles M, Bonneil E, Meloche S, Chelbi-Alix MK, Thibault P. A novel proteomics approach to identify SUMOylated proteins and their modification sites in human cells. Mol Cell Proteomics 2011; 10:004796; http://dx.doi.org/10.1074/mcp.M110.004796; PMID: 21098080
- Pal S, Rosas JM, Rosas-Acosta G. Identification of the non-structural influenza A viral protein NS1A as a bona fide target of the Small Ubiquitin-like MOdifier by the use of dicistronic expression constructs. J Virol Methods 2010; 163:498 - 504; http://dx.doi.org/10.1016/j.jviromet.2009.11.010; PMID: 19917317