Abstract
In utero environmental perturbations have been associated with epigenetic changes in the offspring and a lifelong susceptibility to cardiovascular diseases (CVD). DNA methylation at the ATP-binding cassette transporter A1 (ABCA1) gene was previously associated with CVD, but whether these epigenetic marks respond to changes in the maternal environment is unknown. This study was undertaken to assess the associations between the maternal metabolic profile and ABCA1 DNA methylation levels in placenta and cord blood. Placenta and cord blood samples were obtained at delivery from 100 women including 26 with impaired glucose tolerance (IGT) diagnosed following a 75 g-oral glucose tolerance test (OGTT) between week 24 and 28 of gestation. ABCA1 DNA methylation and mRNA levels were measured using bisulfite pyrosequencing and quantitative real-time PCR, respectively. We report that ABCA1 DNA methylation levels on the maternal side of the placenta are correlated with maternal high density lipoprotein cholesterol (HDL-C) levels (r < –0.21; P < 0.04) and glucose levels 2 h post-OGTT (r = 0.25; P = 0.02). On the fetal side of the placenta, ABCA1 DNA methylation levels are associated with cord blood triglyceride levels (r = –0.28; P = 0.01). ABCA1 DNA methylation variability on both sides of the placenta are also associated with ABCA1 mRNA levels (r < –0.35; P = 0.05). As opposed to placenta, cord blood DNA methylation levels are negatively correlated with maternal glucose 2 h post-OGTT (r = –0.26; P = 0.02). In conclusion, the epivariations observed in placenta and cord blood likely contribute to an optimal materno–fetal cholesterol transfer. These in utero epigenetics adaptations may also potentially trigger the long-term susceptibility of the newborn to dyslipidemia and CVD.
Introduction
Pregnancy is associated with a number of metabolic adaptations that support a normal fetal growth. These adaptations include maternal weight gain, changes in blood lipid profile, and insulin resistance.Citation1 If the pancreatic β-cells cannot face the increased demand of superimposed gestational insulin resistance, pregnant women develop gestational diabetes mellitus (GDM). GDM, defined as impaired glucose tolerance with first recognition during pregnancy, is the most frequent metabolic perturbation observed in pregnant women with a prevalence ranging from 2 to 20% according to the population studied and diagnostic criteria used.Citation2 Fetal exposure to GDM is associated with a long-term increased risk for obesity, type 2 diabetes (T2D) and cardiovascular disease (CVD).Citation3-Citation5 This concept of fetal programming of adult metabolic diseases (originating from Barker’s hypothesisCitation6) is well supported by several studies and thus now widely accepted. However, the molecular mechanisms involved have not been clearly defined so far. Recent studies have suggested that epigenetic modifications might explain, to some extent, the link between suboptimal fetal conditions and long-term risks for cardio-metabolic disorders in the newborn.Citation7
Epigenetics refers to DNA modifications independent of the primary sequence that are involved in gene transcription regulation.Citation8 DNA methylation is the most stable and studied epigenetic modification and is generally associated with a repression of gene expression.Citation9 The addition of a methyl group is catalyzed by the DNA methyltransferases and occurs at position 5' of the pyrimidine ring of the cytosine upstream of a guanine (CpG dinucleotide).Citation10 Cytosine methylation in a CpG context has been shown to be associated to environmental stimuli including the hyperglycemic intrauterine environment.Citation11-Citation13 This is now supported by an in vitro study showing that a chronic exposure to hyperglycemia induces persistent DNA methylation and gene expression profile alterations of key metabolic genes.Citation14
Epigenetic adaptations of the placenta and newborn appear to be important for the regulation of placental functions, fetal growth, and development. They have been associated with risk factors (birth weight, child body mass index [BMI] and adiposity) known to trigger an increased long-term susceptibility to metabolic disorders.Citation15-Citation19 The newborn epigenetic signature acquired in the womb also appears to be persistent throughout adult life in animal models and in human epidemiological studies.Citation20-Citation22
Previously, a study carried on by Tobi et al.Citation22 has reported that DNA methylation levels at the ATP-binding cassette transporter A1 (ABCA1) gene locus are higher in leukocytes of adults exposed prenatally to the ending World War II Dutch famine, suggesting that the ABCA1 DNA methylation profile might be sensitive to in utero adverse environmental exposure. ABCA1 transfers cholesterol from cells to apolipoproteins A-1 (apo-A1), thus contributing to the formation of nascent high-density lipoproteins (HDL).Citation23 In human placenta, ABCA1 is highly expressed in endothelial cells of fetal capillaries and also in the syncytiotrophoblast cells on the maternal side of the placenta where it regulates materno-fetal cholesterol transport.Citation24,Citation25 ABCA1 is thus a crucial regulator of the lipoprotein metabolism. Recently, our group reported that ABCA1 epipolymorphisms are associated with HDL particle profile (especially HDL cholesterol [HDL-C] concentrations) as well as history of coronary artery disease in adults with familial hypercholesterolemia.Citation26 Hence, ABCA1 DNA methylation profile appears to be sensitive to in utero environment and might have long-term functional impact on lipid profile and the onset of CVD. We have thus hypothesized that the newborn ABCA1 DNA methylation profile could be disturbed by the maternal metabolic status. Accordingly, the main objective of the current study was to determine the associations between the maternal glucose status and lipid profile and ABCA1 DNA methylation profile in placenta and cord blood.
Results
shows the characteristics of mothers and their newborns according to the maternal glucose tolerance status. Women were on average 29 y old, were slightly overweight and had normal fasting glucose concentrations and lipid profile. Of the 100 women recruited, 26 had impaired glucose tolerance (IGT), whereas 74 were normoglycemic (NGT). Among the women with IGT, 13 were recommended to follow a diet and 12 were treated with insulin in addition to the diet (treatment was unknown for one woman). Parity was higher in women with IGT compared with NGT women (P = 0.02). Women with IGT gained less weight on average than NGT women during pregnancy. ABCA1 DNA methylation levels were found to be significantly lower in the blood of IGT mothers than with NGT mothers (P = 0.04). Cord blood ABCA1 DNA methylation levels also tended to be lower in newborn exposed to IGT (P = 0.06) (). Moreover, newborns from the IGT and NGT groups had similar gestational age, birth weight, and cord blood lipid profile. Interestingly, children born to mothers with IGT had significantly higher cord blood c-peptide levels than those born to NGT.
Table 1. Maternal and newborn characteristics according to glucose tolerance status
ABCA1 genomic context and DNA methylation analyses
Overall, 22 CpG dinucleotides within four regions of the ABCA1 gene promoter CpG island were first epigenotyped in ten placenta samples (maternal and fetal sides), maternal blood at first trimester and cord blood samples (). The CpG sites that were found to be unmethylated (<10.0%) or fully methylated (>90.0%) were excluded from the current study as they showed low variability and were unlikely to explain the phenotypic variability. Hence, ABCA1 loci B, C, and D, mostly unmethylated (<10%) and almost invariable in all four tissues, were not further analyzed. ABCA1-A locus (8 CpGs), located just upstream the first exon, was the only region showing interindividual variability in DNA methylation levels across all tissues and was consequently analyzed in the remaining samples.
Figure 1.ABCA1 CpG island proximal promoter region and localization of the 4 loci epigenotyped in maternal and fetal placenta, as well as in maternal and cord blood samples. The ABCA1-A locus shows DNA methylation variability in both fetal and maternal sides of the placenta (especially at CpG5 and CpG6), as well as in maternal and cord blood samples.
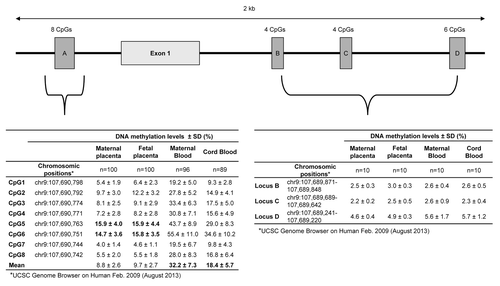
DNA methylation levels of maternal blood samples at the ABCA1-A locus were highly correlated between CpG sites 3 to 8 (r = 0.80 to 0.97; P < 0.001), whereas CpG sites 1 and 2 were less strongly correlated with the 6 others (r = 0.58 to 0.87; P < 0.001) (Fig. S1). In cord blood samples, DNA methylation levels of the 8 CpGs analyzed were found to be highly correlated with each other (r = 0.83 to 0.97; P < 0.001) (Fig. S1). Mean DNA methylation level values of the 8 CpGs epigenotyped were thus computed in maternal and cord blood samples and used for further analyses as previously described by Guay et al.Citation26 and Tobi et al.Citation22
Mean ABCA1 DNA methylation levels were higher in maternal blood as compared with cord blood (31.9 ± 7.3% vs. 18.0 ± 5.7%, P < 0.001). Interestingly, mean ABCA1 DNA methylation levels were found to be well correlated between maternal blood and cord blood samples (r = 0.50; P < 0.001). Maternal blood ABCA1 DNA methylation levels explained up to 20.0% (R2) of the DNA methylation variability at ABCA1 locus in cord blood.
On average, ABCA1-A locus DNA methylation was found to be lower than 10% in maternal (8.8 ± 2.6%) and fetal (9.7 ± 2.7%) placenta samples. Nevertheless, mean ABCA1-CpG5 and ABCA1-CpG6 DNA methylation levels were between 14.7% and 15.9% on average with good variability (s.d. > 3%) (). CpG sites 1 to 4, 7, and 8 were excluded from the analysis as they were considered unmethylated (DNA methylation < 10%) and showed low variability (s.d. < 3%) (). As they were only modestly correlated with each other (maternal side: r = 0.62 and fetal side: r = 0.54), ABCA1-CpG5 and ABCA1-CpG6 were investigated separately in both maternal and fetal side of the placenta. DNA methylation levels at ABCA1-CpG5 and CpG6 in maternal and cord blood were not associated to DNA methylation levels in either the maternal (r < |0.12|; P > 0.29) or fetal side of the placenta (r < |0.10|; P > 0.31).
Placental ABCA1 DNA methylation levels according to the maternal and newborn metabolic profiles
We first assessed whether the maternal metabolic profile might be associated with placental ABCA1 DNA methylation variability. On the maternal side of the placenta, we observed that higher maternal HDL-C concentrations at first trimester of pregnancy were associated with decreased DNA methylation levels at both ABCA1-CpG5 (r = –0.21; P = 0.04) and ABCA1-CpG6 (r = –0.24; P = 0.02) (). Consistently, the maternal placental ABCA1-CpG6 locus was found to be differentially methylated according to maternal HDL-C level tertiles at first trimester (right section of , P = 0.05). Indeed, mothers with the highest HDL-C levels (>1.67 mmol/L, n = 35) had 2.3% lower DNA methylation levels than women with the lowest concentrations (<1.44 mmol/L, n = 33).
Figure 2. Placental ABCA1-CpG5 and -CpG6 DNA methylation levels according to maternal and newborn metabolic profile. Higher maternal HDL-C levels at first trimester of pregnancy (A and B) and lower maternal glucose concentrations 2 h post-OGTT (C) were both associated with lower ABCA1 DNA methylation levels in the maternal side of the placenta. Panel D shows the negative correlation between ABCA1-CpG5 DNA methylation levels in the fetal side of placenta and cord blood triglyceride levels. aIncluded age, BMI, TG at first trimester and history of gestational diabetes as covariates. bAdjusted for gestational age and newborn sex. cCorrelation coefficient and P value obtained after log10-transformation of the cord blood triglyceride levels.
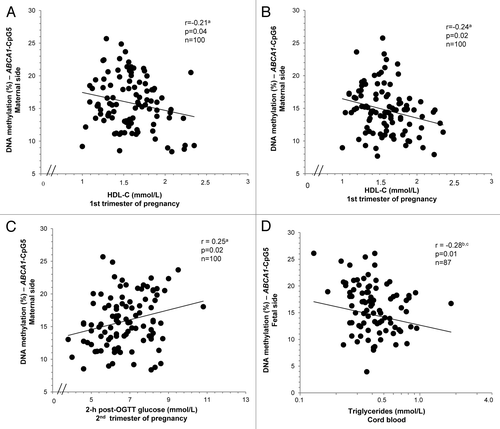
Figure 3. DNA methylation levels at ABCA1-CpG6 on the maternal side of the placenta according to glucose tolerance status and HDL-C levels. Women with impaired glucose tolerance (IGT) and low HDL-C levels (<1.44 mmol/L) had the highest ABCA1-CpG6 DNA methylation levels. Maternal glucose status and HDL-C levels interact to increase ABCA1 DNA methylation on maternal side of placenta (P = 0.04). aP values obtained in the Bonferroni post-hoc analysis by comparing low (<1.44 mmol/L) and medium (1.44–1.67 mmol/L) levels of HDL-C to levels >1.67 mmol/L in IGT group. bP value obtained in the Bonferroni post-hoc analysis by comparing HDL-C levels <1.44 mmol/L to levels >1.67 mmol/L in the entire cohort (n = 100).cANCOVA adjusted for age and BMI at first trimester and history of GDM.
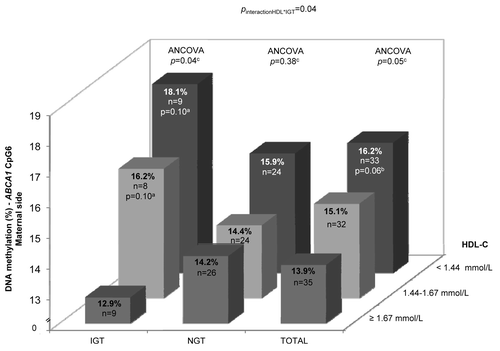
Moreover, we observed a positive correlation between maternal placenta ABCA1-CpG5 DNA methylation levels and maternal glucose 2 h post-OGTT levels (r = 0.25; P = 0.02) (). However, ABCA1 DNA methylation levels were found to be similar in the IGT and NGT groups on both sides of the placenta (). In addition, DNA methylation levels at ABCA1-CpG6 were significantly different among IGT women according to HDL-C level tertiles at first trimester (left section of ; P = 0.04). In women with IGT and high HDL-C levels (>1.67 mmol/L, n = 9), ABCA1-CpG6 was found to be hypomethylated when compared with the two lower tertiles of HDL-C (). For women with NGT, no significant difference in DNA methylation was observed among the HDL-C levels tertiles (middle section of the ). Of interest, an interaction effect was observed between maternal glucose status and HDL-C levels on DNA methylation levels at ABCA1-CpG6 (P = 0.04) (). A trend for interaction on DNA methylation levels at ABCA1-CpG5 was also observed between these two factors (P = 0.07), even though no significant DNA methylation difference was observed between groups (HDL-C levels and glucose tolerance status) for this CpG on the maternal side of placenta (data not shown). Results remained unchanged after consideration for potential confounding factors (age, BMI and triglycerides [TG] at first trimester of pregnancy, history of GDM, and weight gain between first and third trimester).
On the fetal side of the placenta, the maternal metabolic profile did not contribute significantly to ABCA1 DNA methylation level variability. Nevertheless, DNA methylation at fetal placental ABCA1-CpG5 was significantly correlated with cord blood TG concentrations (r = –0.28; P = 0.01) (), whereas a trend for association was also observed with cord blood HDL-C levels (r = 0.19; P = 0.09) when newborn sex and gestational age were taken into account. All the associations remained similar after statistical adjustments for maternal age, BMI at first trimester, history of GDM and weight gain between first and third trimester. DNA methylation levels on the maternal and fetal side of the placenta were not found to be associated with other maternal or fetal metabolic variables (Tables S2 and S3).
According to the results of the stepwise multivariable linear regression analyses of placental ABCA1 DNA methylation level predictors, maternal and fetal characteristics explained respectively up to 11.4% and 5.9% of the ABCA1 DNA methylation variability observed in the maternal or fetal side of placenta (). These results remained unchanged when the forward and backward multivariable linear regression models were applied (data not shown).
Table 2. Multivariable linear regression analyses of ABCA1 DNA methylation levels predictors in placenta and cord blood
Correlations between cord blood ABCA1 DNA methylation levels, maternal characteristics, and newborn metabolic profiles
Next, we investigated whether maternal and newborn characteristics might be associated with cord blood ABCA1 DNA methylation level variability. First, the maternal glucose metabolism at second trimester of pregnancy was found to be associated with the DNA methylation profile of the cord blood ABCA1-A locus. Indeed, we observed a negative correlation between cord blood ABCA1-A DNA methylation levels and maternal glucose concentrations (2 h post-OGTT) (), as well as an association with fasting TG at first trimester (r = –0.24; P = 0.03) when all maternal potential confounders variables were included in the statistical model. Moreover, higher cord blood ABCA1-A DNA methylation levels were significantly associated with higher placenta weight (r = 0.29; P = 0.01) (Table S2) and lower fetal-placental weight ratio (r = -0.25, P = 0.03; ) at term when both gestational age and newborn’s sex were considered. These correlations remained unchanged after the inclusion of the following variables: maternal age, BMI, blood ABCA1 DNA methylation, TG at first trimester, glucose levels 2 h post-OGTT at second trimester and weight gain between first and third trimester. No additional correlation was observed between cord blood ABCA1 DNA methylation levels, maternal and newborn characteristics (Tables S2 and S3). Overall, the combination of maternal and fetal characteristics might explain up to 34.0% of the cord blood ABCA1 DNA methylation variability ().
Figure 4. Cord blood ABCA1-A locus DNA methylation levels according to maternal and newborn characteristics. Pearson’s correlations between cord blood mean ABCA1 DNA methylation levels and: (A) 2 h post-OGTT maternal glucose concentrations, and (B) placental efficiency (estimated by fetal-placental weight ratio). aAdjusted for newborn’s sex and gestational age, as well as maternal age, BMI, TG at first trimester and history of gestational diabetes.
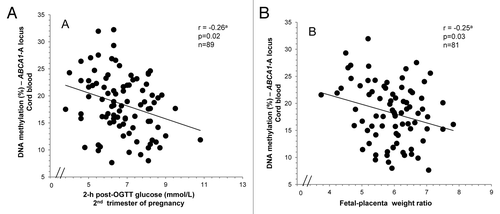
Correlation between ABCA1 DNA methylation and mRNA levels on the maternal and fetal side of the placenta
To assess the functional impact of the ABCA1 DNA methylation level variability, ABCA1 mRNA levels were quantified in maternal and fetal placenta. Relative ABCA1 gene expression was found to be negatively correlated with ABCA1-CpG6 DNA methylation levels on the maternal (r = –0.35; P = 0.05) and fetal side of the placenta (r = –0.36; P = 0.05) (). Moreover, a trend for association was observed between DNA methylation at ABCA1-CpG5 and ABCA1 gene expression in fetal placenta (r = –0.35; P = 0.06). Of note, potential binding sites for transcription factors such as AP-2, AP-4, ETS1, SRY, and STAT were found closed to ABCA1-CpG5 and CpG6, within or near ABCA1-A locus.Citation26
Discussion
Previous studies suggested that in utero environment perturbations, such as maternal dyslipidemia and hyperglycemia, contribute to the development of adult chronic diseases (obesity, diabetes, CVD),Citation5,Citation27 possibly through epigenetic adaptations in the placenta and offspring.Citation11,Citation12,Citation28 Leukocyte DNA methylation variability at ABCA1 gene locus was previously associated to prenatal famine exposure, blood lipid profile and CVD.Citation22,Citation26 These results suggest that ABCA1 epigenetic profile is sensitive to the in utero environment and that methylation at this locus could explain, to some extent, the link between suboptimal maternal conditions and the metabolic health programming of the newborn. In the current study, we showed that higher ABCA1 DNA methylation levels on the maternal side of the placenta were associated with lower maternal HDL-C levels and higher maternal glucose concentrations 2 h post-OGTT at first trimester and second trimester of pregnancy respectively ().
Figure 6. Maternal and fetal metabolic variables associated with placental and cord blood ABCA1 DNA methylation variations. Maternal (glucose 2 h post-OGTT and HDL-C levels) and fetal metabolic profile (cord blood triglyceride levels) are respectively associated with ABCA1 DNA methylation levels in the maternal and fetal side of the placenta. Higher maternal glucose 2 h post-OGTT and triglyceride levels are associated with lower cord blood ABCA1 DNA methylation levels, whereas maternal epigenetic profile is positively associated with cord blood ABCA1 DNA methylation.
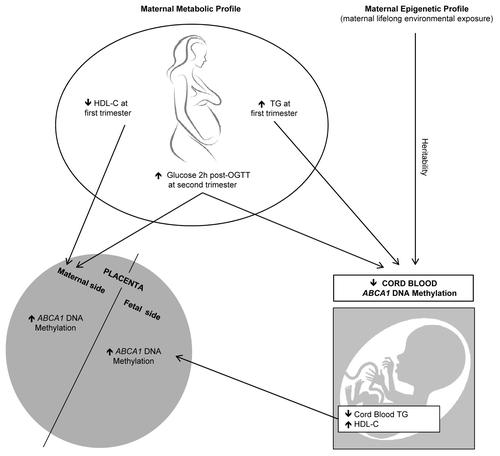
In our cohort, IGT women gave birth to children with higher cord blood c-peptide levels even if they gained less weight throughout their pregnancy possibly because of their required clinical follow-up.Citation29,Citation30 These results suggest that child born to IGT mother have higher insulin production and confirm that they were exposed to higher maternal glucose concentrations in utero. A hyperglycemic in utero environment has already been associated with impaired fetal and placental metabolism and epigenomic changes. Indeed, several epidemiological studies have associated maternal glucose with fetal overgrowth, excess adiposity in childhood and long-term susceptibility of offspring to obesity and metabolic disorders.Citation5,Citation31,Citation32 Moreover, a recent in vitro study has reported that chronic exposure to hyperglycemia leads to persistent DNA methylation modifications of key metabolic genes in Schwann cells.Citation14 Likewise, associations between maternal hyperglycemia exposure and placental DNA methylation variations at LEP, ADIPOQ and MEST gene loci have been previously reported by our group and El Hajj et al.Citation11-Citation13 The maternal lipid profile (HDL-C, total cholesterol, and TG), in pregnancies complicated or not by IGT, was also shown to be a determinant of birth weight and lifelong susceptibility to cardio-metabolic disorders.Citation33-Citation35 Our results support these observations and suggest that the effects of maternal lipid and glucose changes could partly be mediated through epigenetic modifications at specific loci, such as ABCA1 and other genes.
The exact role of ABCA1 is not clearly defined on the maternal side of the placenta. Indeed, ABCA1 appears to regulate both the placental cholesterol uptake and cholesterol efflux toward maternal circulation.Citation36,Citation37 We showed that mothers with low HDL-C and high glucose levels at first and second trimester of pregnancy respectively had higher ABCA1 DNA methylation levels and a concomitant reduction in ABCA1 expression in the placenta. This interaction might be attributed to an overall less favorable metabolic profile, often characterized by the combination of low HDL-C and high glucose levels. Moreover, the reduction of placental ABCA1 mRNA has already been reported in obese and normal weight subjects with IGT and suggested to impair placental cholesterol-uptake and transport.Citation38 Although ABCA1 DNA methylation levels on the maternal side of the placenta were not associated with birth weight or the cord blood lipid profile, this suggests that other key players of the placental cholesterol metabolism may compensate for the loss of function of ABCA1 (e.g., ATP-binding cassette G1 [ABCG1] or scavenger receptor class B member 1 [SRB1]). Furthermore, we cannot exclude that the ABCA1 DNA methylation epivariations we observed on the maternal side of the placenta may also contribute to the maternal HDL-C level variability.
The role of ABCA1 in the endothelial cells of the fetal side of the placenta is more clearly defined.Citation39 Indeed, Stefulj et al.Citation39 have reported that ABCA1 regulates the cholesterol efflux from the placenta to apo-A1 and apo-E for HDL biogenesis and subsequent release into fetal circulation. In the current study, ABCA1 DNA methylation levels on the fetal side of the placenta were not associated with maternal metabolic profile, but they were associated with cord blood lipid profile (). Increased ABCA1 DNA methylation levels and the expected decreased expression were observed when cord blood HDL-C concentrations were higher and TG levels were lower. Interestingly, ABCA1 DNA methylation on the maternal side of the placenta was not associated with newborn weight or cord blood lipid profile. As the fetus can synthesize de novo cholesterol, the existence of fetal stimuli to prevent excess cholesterol delivery from the placenta has been proposed.Citation40 Our results support this hypothesis and suggest that cord blood lipid concentrations could prevent excess cholesterol transfer from the fetal side of the placenta to fetal circulation through epigenetic modifications at the ABCA1 gene locus. The ABCA1 epigenetic profile on the fetal side of the placenta may be more susceptible to fetal lipid level adaptations than the maternal side since it appears to be one of the most important regulators of placental-fetal cholesterol transfer and likely of newborn cholesterol homeostasis. However, the cord blood lipid profile only explains a modest fraction of ABCA1 DNA methylation variability in the fetal side of the placenta suggesting that other factors might affect this epipolymorphism and the cholesterol transfer.
In a previous study carried on leukocytes, we failed to find a significant association between DNA methylation at the ABCA1-A gene promoter locus and ABCA1 mRNA levels.Citation26 The results of the current study are the first to suggest that the ABCA1 DNA methylation locus tested in our previous study (Guay et al.Citation26) and that of Tobi et al.Citation22 is associated with its gene expression regulation, at least in a tissue-specific manner in placenta. Further analyses in others tissue will be needed to confirm the possible tissue-specific impact of DNA methylation at ABCA1 gene promoter locus.
Cord blood samples were also epigenotyped to determine the relationship between the blood lipid profile and maternal IGT. We first observed that ABCA1 DNA methylation levels were associated with maternal blood leukocyte ABCA1 DNA methylation. Based on correlation analyses, we estimated that the familial resemblance or the multifactorial heritability of leukocyte ABCA1 DNA methylation might be as high as 20%. This is concordant with previous studies, which have lately estimated the whole-blood DNA methylation heritability to 18% at the genome-wide level in twin pairs, and 20% and 15–48% at LEP and FABP3 gene loci respectively in family member comparison studies.Citation41-Citation43 Furthermore, it suggests that the newborn epigenetic profile at the ABCA1 gene locus is partially inherited and thus likely affected by the environment to which the mother has been exposed throughout her own life ().
Although newborns’ methylome is only moderately inherited from the mother, our results also suggest that it may vary according to the intrauterine environment to which it has been exposed. Indeed, cord blood ABCA1 DNA methylation was found to be negatively correlated with maternal fasting triglyceridemia and glucose levels 2 h post-OGTT at first and second trimester of pregnancy respectively (). Interestingly, the correlation between maternal glucose and ABCA1 DNA methylation levels showed opposite associations in cord blood and on the maternal side of the placenta. Accordingly, newborns’ methylome may differently respond to maternal glucose in order to overcome placental ABCA1 DNA methylation modifications and impaired materno-fetal cholesterol transfer. On the maternal side of the placenta, we observed increased DNA methylation levels at ABCA1-A locus when maternal glucose concentrations were higher at second trimester. As increased placental ABCA1 DNA methylation levels are associated with decreased mRNA levels, this suggests that high maternal glucose concentrations could reduce materno-fetal cholesterol transfer. Hence, the newborn ABCA1 gene promoter locus might be less methylated in order to increase endogenous HDL-C biogenesis and to overcome the decrease in placental cholesterol transfer. Moreover, the association found between cord blood ABCA1 DNA methylation levels and the fetal-placental weight ratio (a placental efficiency marker representing the feto-placental development occurring throughout pregnancy to meet fetal growth demandsCitation44) also suggests that the ABCA1 epigenetic profile of newborns might adapt to or influence placental efficiency to ensure an adequate lipid supply. Therefore, as observed on the fetal side of the placenta, newborns’ ABCA1 DNA methylation profile may also serve as a regulatory mechanism to ensure that a sufficient quantity of endogenous and/or exogenous cholesterol is available to the fetus for its optimal growth and development. Nevertheless, the ABCA1 epigenetic “compensatory” signature acquired in utero may have lifelong consequences on newborns’ lipid metabolism possibly triggering their susceptibility to obesity, dyslipidemia, and CVD.Citation26
Interestingly, Tobi et al.Citation22 have observed that prenatal exposure to ending World War II-related famine in the Netherlands was associated with increased DNA methylation levels at the ABCA1 gene promoter locus in leukocytes of adults. This reinforces the potential role of ABCA1 epigenetic adaptations to in utero environment as DNA methylation changes seem to be opposing in conditions where nutrients are restricted (famine) or in excess (hyperglycemia); two conditions associated with a long-term increased susceptibility to CVD.Citation45-Citation49 Moreover, it suggests that dysregulation (hypo or hypermethylation) of the ABCA1 epigenetic profile could impair ABCA1 function and possibly increase the risk of CVD. Indeed, we have previously reported increased ABCA1 DNA methylation levels in patients with a previous history of coronary artery disease (Guay et al.).26 Further studies will be needed to assess whether epigenetic perturbations of ABCA1 gene observed in newborns might contribute or not to the development of chronic diseases in children and adults, but the current study and the literature provide good evidence in support of the existence of this relationship.
One strength of this study is the longitudinal follow-up of women from first trimester of pregnancy to delivery. It allowed us to accurately identify mothers developing IGT during pregnancy and avoid their misclassification with women who were diabetic pre-pregnancy. Moreover, the study design, along with our fairly large sample size, has allowed us to consider potential confounding factors in our statistical models. ABCA1 was epigenotyped in four different tissues thus providing insights on tissue-specific regulation. It also gives us the overall picture of the feedback regulatory mechanisms that might exist at epigenetic levels between these inter-connected tissues. Nevertheless, as placenta and cord blood samples were analyzed cross-sectionally at birth, the causal variable (placenta or cord blood) in the compensatory mechanisms discussed remains to be identified. Moreover, ABCA1 DNA methylation levels will need to be assessed in these children in a few years to determine methylation stability at this locus and its association with developmental indices and lipid profile throughout infancy and childhood. ABCA1 DNA methylation levels were tested for association with numerous closely related and highly correlated metabolic variables (e.g., HOMA-IR, insulin and glucose; or total cholesterol, HDL-C, LDL-C, and TG). Bonferroni correction for multiple testing would be likely considered too stringent in this context and would thus potentially generates false-negative results.Citation50 It remains that some of the reported associations are quite significant (P < 0.01) and that this study is the third one putting forward the association between ABCA1 DNA methylation levels and suboptimal in utero exposure or adverse cardiometabolic outcomes. In addition, as the results observed are associative, further studies (such as the one carried by Kim et al.Citation14) will need to assess the functional impact of the maternal metabolic profile on placental and newborn methylome.
The current study reports for the first time that maternal metabolic profile is associated with ABCA1 DNA methylation levels in the maternal side of placenta and cord blood. Our results also suggest that ABCA1 DNA methylation profile changes in response to in utero environment and fetal stimuli to ensure optimal cholesterol delivery to the fetus and then cellular intake. These epigenetic adaptations could potentially trigger a long-term susceptibility of the newborn to obesity, dyslipidemia and CVD. This study also suggests that epigenetic modifications are involved in fetal programming and raises the possibility that other gene loci will respond to maternal conditions and could explained the link between suboptimal in utero environment and metabolic health programming of the newborn. Our results thus emphasize the need for the instigation of better maternal care programs early during pregnancy.
Materials and Methods
Sample and clinical data
One hundred women with a singleton pregnancy were selected from a cohort recruited in a founder population of French-Canadian origin from the Saguenay-Lac-Saint-Jean region of Québec (Canada). The women included in this study were aged between 18–40 y, with no personal history of pre-gestational type 1 or 2 diabetes, polycystic ovary syndrome (PCOS), or familial hypercholesterolemia. Women with a positive history of alcohol and/or drug abuse during the current pregnancy were also excluded. All women provided a written informed consent before their inclusion, and all clinical data were de-identified. This project was approved by the Chicoutimi Hospital Ethics Committee, in accordance with the Declaration of Helsinki.
Women were met at the ECOGENE-21 Clinical Research Center at the first (11–14 wk), second (24–28 wk), and third (36–39 wk) trimester of pregnancy. A registered nurse performed a complete anthropometric and metabolic profiling. Anthropometric variables and blood pressure were measured using standardized procedures.Citation51 Blood samples were obtained after a 12 h overnight fast from the antecubical vein into vacutainer tubes containing EDTA at each gestational trimester and at the time of delivery (cord blood). Plasma cholesterol, TG, and glucose levels were enzymatically measured using a Beckman analyzer (models DXC and LX20). Total cholesterol was determined in plasma and HDL particles after precipitation of very low-density lipoprotein (VLDL) and low-density lipoprotein (LDL) particles (d > 1.006 g/ml) in the infranatant with heparin and MnCl2. Plasma LDL-cholesterol (LDL-C) levels were estimated using the Friedewald formula.Citation52 Insulin measurements were performed using a radioimmunoassay method (Advia Centaur, Simmens). IGT was defined as a 2 h glucose ≥ 7.8 mmol/L following a 75-g OGTT performed at 24–28 weeks’ gestation according to the WHO criteria.Citation53 Insulin resistance levels were assessed using HOMA-IR (fasting glucose [mmol/L] × fasting insulin [mU/L] / 22.5) at the second trimester.Citation54 Clinical information about the newborn was collected at birth from medical files and included gestational age, sex of the newborn and anthropometric measurements. The gestational age was calculated at the first visit from the date of the last menstrual period and was corrected afterward, as required, based on the date of the ultrasound scans. Cord blood lipid and glucose levels were assessed as described for the mother. In addition, cord blood C-peptide levels, measured by ELISA, were used as a marker of the newborn’ insulin-secretory activity.Citation55
ABCA1 DNA methylation and mRNA measurements
Placenta and cord blood samples were taken within minutes of delivery and placental expulsion (on average < 15 min) by well-trained clinicians (MD). Cord blood samples collected were mixed arterial and venous blood. On the fetal side of the placenta, biopsies were taken near the insertion point of the umbilical cord and consisted of the chorionic plate with the chorionic villi. Maternal placenta biopsies were sampled on the opposite side of the placental cord blood insertion point and were composed of the basal plate and the chorionic villi. The biopsies were washed in PBS 1× to remove cord/maternal blood, dissected to remove conjunctive tissues and kept in RNAlater (Qiagen, #76106) at –80 °C until nucleic acid extraction. Placental DNA and RNA were purified using the All Prep DNA/RNA/Protein Mini Kit (Qiagen, #80004). DNA was purified from whole blood and cord blood samples with the Gentra Puregen Blood Kit (Qiagen, #158389). Maternal blood samples at first trimester were available for 96 of the 100 mothers, whereas 89 cord blood samples were available for DNA methylation analysis. Placental RNA quality was assessed with Agilent 2100 Bioanalyser RNA Nano Chips (Agilent Technologies, #5067–1511). RNA samples on both the maternal and fetal side of the placenta showed good quality with a mean RNA integrity number (RIN) ranging from 6.9 to 9.3 (mean: 8.1 ± 0.7) and 6.5 to 9.5 (mean: 8.2 ± 0.9) respectively.
We used the gold-standard pyrosequencing technology, an accurate and quantitative sequencing assay, to determine base-specific cytosine methylation levels at four different loci within the CpG island of the ABCA1 gene proximal promoter (). The epigenotyped regions were the same as those previously described.Citation26 Pyrosequencing assays combine sodium bisulfite DNA conversion chemistry (EpiTech Bisulfite Kits; Qiagen; #59104), polymerase chain reaction (PCR) amplification (Pyromark PCR Kit; Qiagen; #978703), and sequencing by synthesis assay (Pyromark Gold Q24 Reagents; Qiagen; #978802) of the target sequence. Sodium bisulfite preferentially deaminates unmethylated cytosine residues to thymines (after PCR amplification), whereas methyl cytosines remain unmodified. Each of the pyrosequencing runs performed included a negative PCR control and a sequencing primer control as well as sodium bisulfite conversion controls. Additionally, pyrosequencing quality control (peak height, deviation from reference sequence pattern and unexpected peak height) was assessed for each sample using Pyromark Q24 Analysis Software (v1.0.10.134). Most of the samples were not run in replicates but 100% of the samples passed the pyrosequencing quality control. PCR primers were selected using the PyroMark Assay Design software v2.0.1.15. The PCR and pyrosequencing primers for the amplification of the ABCA1 gene CpG island loci are described in Table S1.
ABCA1 mRNA levels were measured in a randomly selected subsample of 31 placental samples from the maternal and fetal sides. One sample from the fetal side was not available for mRNA quantification. Complementary DNA (cDNA) was generated from placental total RNA using a random primer hexamer provided with the High Capacity cDNA Archive kit (Life Technologies, #4368814). Equal amounts of cDNA were run in duplicate and amplified in a 20 µl reaction containing 10 µL of 2X Universal PCR Master Mix (Life Technologies, #4366072). Primers and Taqman probes were designed to cover exon boundaries (Life Technologies, ABCA1: Hs01059118_m1). Each sample was calibrated to the YWHAZ housekeeping gene (endogenous control; YWHAZ: Hs00237047_m1).Citation56-Citation58 Relative quantification estimations were performed using an Applied Biosystems 7500 Real Time PCR System as recommended by the manufacturer. ABCA1/GAPDH Ct ratio (1/x) values were used in the analyses.
Statistical analyses
The normal distribution of all variables was assessed using the Kolmogorov-Smirnov test. The following variables were found normally distributed after they were log10-transformed: fasting TG levels, insulin levels and HOMA-IR. Partial Pearson correlation was used to investigate the association between DNA methylation levels and the main variables of interest: glucose concentrations 2 h post-OGTT, fasting glucose and insulin levels, maternal and cord blood lipid profile, as well as ABCA1 mRNA levels. Categorical variables were compared using a Pearson χ2-statistic, whereas mean differences between groups for continuous variables were compared with a Student t-test (NGT vs. IGT) or an ANOVA, followed by the Bonferroni’s post-hoc comparison test (between the HDL-C levels tertiles). The statistical models were controlled for the following potential confounding factors when appropriate: maternal age, BMI, and TG at first trimester and previous history of GDM as well as newborn’s sex and gestational age. Results remained unchanged after consideration for potential confounding factors (age, BMI, TG at first trimester of pregnancy, smoking, gravidity, parity, mode of delivery, newborn sex, history of GDM and weight gain between first and third trimester). Results were considered statistically significant when P values were <0.05 (two-sided).
Stepwise multivariable linear regression was used to identify predictors of the placental and cord blood ABCA1 DNA methylation level variability. The input-independent variables were: maternal characteristics (age, BMI, weight gain between first and third trimester, fasting triglyceridemia and HDL-C at first trimester, 2 h post OGTT glucose at second trimester and blood ABCA1 DNA methylation levels) and fetal characteristics (gestational age, sex, placental weight, cord blood HDL-C and TG levels). All the variables with a P ≤ 0.10 were included in the regression model. All statistical analyses were performed with the IBM SPSS Statistics 20 software (release 20.0.0, SPSS).
Additional material
Download Zip (83.1 KB)Acknowledgments
The authors acknowledge the contribution of Cécilia Légaré, Sébastien Claveau, MSc; Nadia Mior; Jeanine Landry, RN, and Chantal Aubut, RN from the ECOGENE-21 Clinical Research Center for their dedicated work in this study. The authors also express their gratitude to Céline Bélanger, Chicoutimi Hospital, for her thoughtful language revision of the manuscript. Bouchard L and Hivert MF are junior research scholars from the Fonds de la recherche du Québec en santé (FRQS). Baillargeon JP is senior research scholars from the FRQS. Hivert MF is also supported by a Canadian Diabetes Association clinical scientist award. Bouchard L, Hivert MF, Perron P, and Baillargeon JP are members of the FRQS-funded Centre de recherche clinique Étienne-Le Bel (affiliated to the Centre hospitalier universitaire de Sherbrooke). During this research, Guay SP was the recipient of a doctoral research award from the Canadian Institutes for Health and Research (CIHR) and Houde AA was a recipient of a FRQS doctoral training award. Houde AA and Desgagné V were supported by Diabète Québec. This project was supported by ECOGENE-21, the Canadian Institutes of Health Research (CIHR team in community genetics [grant #CTP-82941]), FRSQ, and Diabète Québec.
Disclosure of Potential Conflicts of Interest
No potential conflicts of interest were disclosed.
Authors’ Contributions
Houde AA and Guay SP contributed to the study design, performed the data collection, the data analysis/interpretation and wrote the manuscript. Desgagné V contributed to data collection and revised the manuscript. St-Pierre J, Hivert MF, Baillargeon JP, and Perron P participated in study design conception and revised the manuscript. Gaudet D contributed to the study design, supplied research infrastructure and revised the manuscript. Brisson D and Bouchard L conceived the study design, participated to data analysis/interpretation process and revised the manuscript.
Supplemental Materials
Supplemental materials may be found here: www.landesbioscience.com/journals/epigenetics/article/26554
References
- Butte NF. Carbohydrate and lipid metabolism in pregnancy: normal compared with gestational diabetes mellitus. Am J Clin Nutr 2000; 71:Suppl 1256S - 61S; PMID: 10799399
- Galtier F. Definition, epidemiology, risk factors. Diabetes Metab 2010; 36:628 - 51; http://dx.doi.org/10.1016/j.diabet.2010.11.014; PMID: 21163426
- Hillier TA, Pedula KL, Schmidt MM, Mullen JA, Charles MA, Pettitt DJ. Childhood obesity and metabolic imprinting: the ongoing effects of maternal hyperglycemia. Diabetes Care 2007; 30:2287 - 92; http://dx.doi.org/10.2337/dc06-2361; PMID: 17519427
- Dabelea D, Mayer-Davis EJ, Lamichhane AP, D’Agostino RB Jr., Liese AD, Vehik KS, Narayan KM, Zeitler P, Hamman RF. Association of intrauterine exposure to maternal diabetes and obesity with type 2 diabetes in youth: the SEARCH Case-Control Study. Diabetes Care 2008; 31:1422 - 6; http://dx.doi.org/10.2337/dc07-2417; PMID: 18375420
- Clausen TD, Mathiesen ER, Hansen T, Pedersen O, Jensen DM, Lauenborg J, Schmidt L, Damm P. Overweight and the metabolic syndrome in adult offspring of women with diet-treated gestational diabetes mellitus or type 1 diabetes. J Clin Endocrinol Metab 2009; 94:2464 - 70; http://dx.doi.org/10.1210/jc.2009-0305; PMID: 19417040
- Barker DJ. Intrauterine programming of adult disease. Mol Med Today 1995; 1:418 - 23; http://dx.doi.org/10.1016/S1357-4310(95)90793-9; PMID: 9415190
- Lillycrop KA, Burdge GC. Epigenetic changes in early life and future risk of obesity. Int J Obes (Lond) 2011; 35:72 - 83; http://dx.doi.org/10.1038/ijo.2010.122; PMID: 20548303
- Henikoff S, Matzke MA. Exploring and explaining epigenetic effects. Trends Genet 1997; 13:293 - 5; http://dx.doi.org/10.1016/S0168-9525(97)01219-5; PMID: 9260513
- Bird A. DNA methylation patterns and epigenetic memory. Genes Dev 2002; 16:6 - 21; http://dx.doi.org/10.1101/gad.947102; PMID: 11782440
- Bestor TH. The DNA methyltransferases of mammals. Hum Mol Genet 2000; 9:2395 - 402; http://dx.doi.org/10.1093/hmg/9.16.2395; PMID: 11005794
- Bouchard L, Hivert MF, Guay SP, St-Pierre J, Perron P, Brisson D. Placental adiponectin gene DNA methylation levels are associated with mothers’ blood glucose concentration. Diabetes 2012; 61:1272 - 80; http://dx.doi.org/10.2337/db11-1160; PMID: 22396200
- Bouchard L, Thibault S, Guay SP, Santure M, Monpetit A, St-Pierre J, Perron P, Brisson D. Leptin gene epigenetic adaptation to impaired glucose metabolism during pregnancy. Diabetes Care 2010; 33:2436 - 41; http://dx.doi.org/10.2337/dc10-1024; PMID: 20724651
- El Hajj N, Pliushch G, Schneider E, Dittrich M, Müller T, Korenkov M, Aretz M, Zechner U, Lehnen H, Haaf T. Metabolic programming of MEST DNA methylation by intrauterine exposure to gestational diabetes mellitus. Diabetes 2013; 62:1320 - 8; http://dx.doi.org/10.2337/db12-0289; PMID: 23209187
- Kim ES, Isoda F, Kurland I, Mobbs CV. Glucose-Induced Metabolic Memory in Schwann Cells: Prevention by PPAR Agonists. Endocrinology 2013; 154:3054 - 66; http://dx.doi.org/10.1210/en.2013-1097; PMID: 23709088
- Turan N, Ghalwash MF, Katari S, Coutifaris C, Obradovic Z, Sapienza C. DNA methylation differences at growth related genes correlate with birth weight: a molecular signature linked to developmental origins of adult disease?. BMC Med Genomics 2012; 5:10; http://dx.doi.org/10.1186/1755-8794-5-10; PMID: 22498030
- Yuen RK, Avila L, Peñaherrera MS, von Dadelszen P, Lefebvre L, Kobor MS, Robinson WP. Human placental-specific epipolymorphism and its association with adverse pregnancy outcomes. PLoS One 2009; 4:e7389; http://dx.doi.org/10.1371/journal.pone.0007389; PMID: 19838307
- Filiberto AC, Maccani MA, Koestler D, Wilhelm-Benartzi C, Avissar-Whiting M, Banister CE, Gagne LA, Marsit CJ. Birthweight is associated with DNA promoter methylation of the glucocorticoid receptor in human placenta. Epigenetics 2011; 6:566 - 72; http://dx.doi.org/10.4161/epi.6.5.15236; PMID: 21521940
- Ferreira JC, Choufani S, Grafodatskaya D, Butcher DT, Zhao C, Chitayat D, Shuman C, Kingdom J, Keating S, Weksberg R. WNT2 promoter methylation in human placenta is associated with low birthweight percentile in the neonate. Epigenetics 2011; 6:440 - 9; http://dx.doi.org/10.4161/epi.6.4.14554; PMID: 21474991
- Godfrey KM, Sheppard A, Gluckman PD, Lillycrop KA, Burdge GC, McLean C, Rodford J, Slater-Jefferies JL, Garratt E, Crozier SR, et al. Epigenetic gene promoter methylation at birth is associated with child’s later adiposity. Diabetes 2011; 60:1528 - 34; http://dx.doi.org/10.2337/db10-0979; PMID: 21471513
- Seki Y, Williams L, Vuguin PM, Charron MJ. Minireview: Epigenetic programming of diabetes and obesity: animal models. Endocrinology 2012; 153:1031 - 8; http://dx.doi.org/10.1210/en.2011-1805; PMID: 22253432
- Heijmans BT, Tobi EW, Stein AD, Putter H, Blauw GJ, Susser ES, Slagboom PE, Lumey LH. Persistent epigenetic differences associated with prenatal exposure to famine in humans. Proc Natl Acad Sci U S A 2008; 105:17046 - 9; http://dx.doi.org/10.1073/pnas.0806560105; PMID: 18955703
- Tobi EW, Lumey LH, Talens RP, Kremer D, Putter H, Stein AD, Slagboom PE, Heijmans BT. DNA methylation differences after exposure to prenatal famine are common and timing- and sex-specific. Hum Mol Genet 2009; 18:4046 - 53; http://dx.doi.org/10.1093/hmg/ddp353; PMID: 19656776
- Joyce C, Freeman L, Brewer HB Jr., Santamarina-Fojo S. Study of ABCA1 function in transgenic mice. Arterioscler Thromb Vasc Biol 2003; 23:965 - 71; http://dx.doi.org/10.1161/01.ATV.0000055194.85073.FF; PMID: 12615681
- Albrecht C, Soumian S, Tetlow N, Patel P, Sullivan MH, Lakasing L, Nicolaides K, Williamson C. Placental ABCA1 expression is reduced in primary antiphospholipid syndrome compared to pre-eclampsia and controls. Placenta 2007; 28:701 - 8; http://dx.doi.org/10.1016/j.placenta.2006.10.001; PMID: 17113147
- Nikitina L, Wenger F, Baumann M, Surbek D, Körner M, Albrecht C. Expression and localization pattern of ABCA1 in diverse human placental primary cells and tissues. Placenta 2011; 32:420 - 30; http://dx.doi.org/10.1016/j.placenta.2011.03.003; PMID: 21501868
- Guay SP, Brisson D, Munger J, Lamarche B, Gaudet D, Bouchard L. ABCA1 gene promoter DNA methylation is associated with HDL particle profile and coronary artery disease in familial hypercholesterolemia. Epigenetics 2012; 7:464 - 72; http://dx.doi.org/10.4161/epi.19633; PMID: 22419126
- van der Graaf A, Vissers MN, Gaudet D, Brisson D, Sivapalaratnam S, Roseboom TJ, Jansen AC, Kastelein JJ, Hutten BA. Dyslipidemia of mothers with familial hypercholesterolemia deteriorates lipids in adult offspring. Arterioscler Thromb Vasc Biol 2010; 30:2673 - 7; http://dx.doi.org/10.1161/ATVBAHA.110.209064; PMID: 20864670
- DeRuiter MC, Alkemade FE, Gittenberger-de Groot AC, Poelmann RE, Havekes LM, van Dijk KW. Maternal transmission of risk for atherosclerosis. Curr Opin Lipidol 2008; 19:333 - 7; http://dx.doi.org/10.1097/MOL.0b013e328304b670; PMID: 18607178
- Deveer R, Deveer M, Akbaba E, Engin-Üstün Y, Aydoğan P, Celikkaya H, Danişman N, Mollamahmutoğlu L. The effect of diet on pregnancy outcomes among pregnant with abnormal glucose challenge test. Eur Rev Med Pharmacol Sci 2013; 17:1258 - 61; PMID: 23690197
- Stewart ZA, Wallace EM, Allan CA. Patterns of weight gain in pregnant women with and without gestational diabetes mellitus: an observational study. Aust N Z J Obstet Gynaecol 2012; 52:433 - 9; http://dx.doi.org/10.1111/ajo.12001; PMID: 23045986
- Walsh JM, Mahony R, Byrne J, Foley M, McAuliffe FM. The association of maternal and fetal glucose homeostasis with fetal adiposity and birthweight. Eur J Obstet Gynecol Reprod Biol 2011; 159:338 - 41; http://dx.doi.org/10.1016/j.ejogrb.2011.09.022; PMID: 21982556
- Deierlein AL, Siega-Riz AM, Chantala K, Herring AH. The association between maternal glucose concentration and child BMI at age 3 years. Diabetes Care 2011; 34:480 - 4; http://dx.doi.org/10.2337/dc10-1766; PMID: 21216858
- Clausen T, Burski TK, Øyen N, Godang K, Bollerslev J, Henriksen T. Maternal anthropometric and metabolic factors in the first half of pregnancy and risk of neonatal macrosomia in term pregnancies. A prospective study. Eur J Endocrinol 2005; 153:887 - 94; http://dx.doi.org/10.1530/eje.1.02034; PMID: 16322395
- Misra VK, Trudeau S, Perni U. Maternal serum lipids during pregnancy and infant birth weight: the influence of prepregnancy BMI. Obesity (Silver Spring) 2011; 19:1476 - 81; http://dx.doi.org/10.1038/oby.2011.43; PMID: 21394096
- Palinski W, Yamashita T, Freigang S, Napoli C. Developmental programming: maternal hypercholesterolemia and immunity influence susceptibility to atherosclerosis. Nutr Rev 2007; 65:S182 - 7; http://dx.doi.org/10.1301/nr.2007.dec.S182-S187; PMID: 18240546
- Baardman ME, Kerstjens-Frederikse WS, Berger RM, Bakker MK, Hofstra RM, Plösch T. The role of maternal-fetal cholesterol transport in early fetal life: current insights. Biol Reprod 2013; 88:24; http://dx.doi.org/10.1095/biolreprod.112.102442; PMID: 23153566
- Aye IL, Waddell BJ, Mark PJ, Keelan JA. Placental ABCA1 and ABCG1 transporters efflux cholesterol and protect trophoblasts from oxysterol induced toxicity. Biochim Biophys Acta 2010; 1801:1013 - 24; http://dx.doi.org/10.1016/j.bbalip.2010.05.015; PMID: 20570635
- Dubé E, Ethier-Chiasson M, Lafond J. Modulation of cholesterol transport by insulin-treated gestational diabetes mellitus in human full-term placenta. Biol Reprod 2013; 88:16; http://dx.doi.org/10.1095/biolreprod.112.105619; PMID: 23221398
- Stefulj J, Panzenboeck U, Becker T, Hirschmugl B, Schweinzer C, Lang I, Marsche G, Sadjak A, Lang U, Desoye G, et al. Human endothelial cells of the placental barrier efficiently deliver cholesterol to the fetal circulation via ABCA1 and ABCG1. Circ Res 2009; 104:600 - 8; http://dx.doi.org/10.1161/CIRCRESAHA.108.185066; PMID: 19168441
- Palinski W. Maternal-fetal cholesterol transport in the placenta: good, bad, and target for modulation. Circ Res 2009; 104:569 - 71; http://dx.doi.org/10.1161/CIRCRESAHA.109.194191; PMID: 19286612
- Zhang Y, Kent JW 2nd, Lee A, Cerjak D, Ali O, Diasio R, Olivier M, Blangero J, Carless MA, Kissebah AH. Fatty acid binding protein 3 (fabp3) is associated with insulin, lipids and cardiovascular phenotypes of the metabolic syndrome through epigenetic modifications in a Northern European family population. BMC Med Genomics 2013; 6:9; http://dx.doi.org/10.1186/1755-8794-6-9; PMID: 23510163
- Bell JT, Tsai PC, Yang TP, Pidsley R, Nisbet J, Glass D, Mangino M, Zhai G, Zhang F, Valdes A, et al, MuTHER Consortium. Epigenome-wide scans identify differentially methylated regions for age and age-related phenotypes in a healthy ageing population. PLoS Genet 2012; 8:e1002629; http://dx.doi.org/10.1371/journal.pgen.1002629; PMID: 22532803
- Lesseur C, Armstrong DA, Paquette AG, Koestler DC, Padbury JF, Marsit CJ. Tissue-specific Leptin promoter DNA methylation is associated with maternal and infant perinatal factors. Mol Cell Endocrinol 2013; 381:160 - 7; http://dx.doi.org/10.1016/j.mce.2013.07.024; PMID: 23911897
- Fowden AL, Sferruzzi-Perri AN, Coan PM, Constancia M, Burton GJ. Placental efficiency and adaptation: endocrine regulation. J Physiol 2009; 587:3459 - 72; http://dx.doi.org/10.1113/jphysiol.2009.173013; PMID: 19451204
- Yang J, Cummings EA, O’connell C, Jangaard K. Fetal and neonatal outcomes of diabetic pregnancies. Obstet Gynecol 2006; 108:644 - 50; http://dx.doi.org/10.1097/01.AOG.0000231688.08263.47; PMID: 16946226
- Bunt JC, Tataranni PA, Salbe AD. Intrauterine exposure to diabetes is a determinant of hemoglobin A(1)c and systolic blood pressure in pima Indian children. J Clin Endocrinol Metab 2005; 90:3225 - 9; http://dx.doi.org/10.1210/jc.2005-0007; PMID: 15797952
- Boney CM, Verma A, Tucker R, Vohr BR. Metabolic syndrome in childhood: association with birth weight, maternal obesity, and gestational diabetes mellitus. Pediatrics 2005; 115:e290 - 6; http://dx.doi.org/10.1542/peds.2004-1808; PMID: 15741354
- Roseboom TJ, van der Meulen JH, Osmond C, Barker DJ, Ravelli AC, Schroeder-Tanka JM, van Montfrans GA, Michels RP, Bleker OP. Coronary heart disease after prenatal exposure to the Dutch famine, 1944-45. Heart 2000; 84:595 - 8; http://dx.doi.org/10.1136/heart.84.6.595; PMID: 11083734
- Roseboom TJ, van der Meulen JH, Ravelli AC, Osmond C, Barker DJ, Bleker OP. Effects of prenatal exposure to the Dutch famine on adult disease in later life: an overview. Twin Res 2001; 4:293 - 8; PMID: 11869479
- Hinault C, Van Obberghen E, Mothe-Satney I. Role of amino acids in insulin signaling in adipocytes and their potential to decrease insulin resistance of adipose tissue. J Nutr Biochem 2006; 17:374 - 8; http://dx.doi.org/10.1016/j.jnutbio.2006.02.008; PMID: 16644198
- Lohman TG. RAaMR. Anthropometric Standardization Reference Manual. Champaign, IL: Human Kinetics Books, 1988.
- Friedewald WT, Levy RI, Fredrickson DS. Estimation of the concentration of low-density lipoprotein cholesterol in plasma, without use of the preparative ultracentrifuge. Clin Chem 1972; 18:499 - 502; PMID: 4337382
- Ahima RS. Digging deeper into obesity. J Clin Invest 2011; 121:2076 - 9; http://dx.doi.org/10.1172/JCI58719; PMID: 21633174
- Matthews DR, Hosker JP, Rudenski AS, Naylor BA, Treacher DF, Turner RC. Homeostasis model assessment: insulin resistance and beta-cell function from fasting plasma glucose and insulin concentrations in man. Diabetologia 1985; 28:412 - 9; http://dx.doi.org/10.1007/BF00280883; PMID: 3899825
- Heding LG. Radioimmunological determination of human C-peptide in serum. Diabetologia 1975; 11:541 - 8; http://dx.doi.org/10.1007/BF01222104; PMID: 1239396
- Meller M, Vadachkoria S, Luthy DA, Williams MA. Evaluation of housekeeping genes in placental comparative expression studies. Placenta 2005; 26:601 - 7; http://dx.doi.org/10.1016/j.placenta.2004.09.009; PMID: 16085039
- Murthi P, Fitzpatrick E, Borg AJ, Donath S, Brennecke SP, Kalionis B. GAPDH, 18S rRNA and YWHAZ are suitable endogenous reference genes for relative gene expression studies in placental tissues from human idiopathic fetal growth restriction. Placenta 2008; 29:798 - 801; http://dx.doi.org/10.1016/j.placenta.2008.06.007; PMID: 18684503
- Drewlo S, Levytska K, Kingdom J. Revisiting the housekeeping genes of human placental development and insufficiency syndromes. Placenta 2012; 33:952 - 4; http://dx.doi.org/10.1016/j.placenta.2012.09.007; PMID: 23026742