Abstract
Colorectal cancer (CRC) is one of the common malignant tumors worldwide. Both genetic and epigenetic changes are regarded as important factors of colorectal carcinogenesis. Loss of DACH1 expression was found in breast, prostate, and endometrial cancer. To analyze the regulation and function of DACH1 in CRC, 5 colorectal cancer cell lines, 8 cases of normal mucosa, 15 cases of polyps and 100 cases of primary CRC were employed in this study. In CRC cell lines, loss of DACH1 expression was correlated with promoter region hypermethylation, and re-expression of DACH1 was induced by 5-Aza-2'-deoxyazacytidine treatment. We found that DACH1 was frequently methylated in primary CRC and this methylation was associated with reduction in DACH1 expression. These results suggest that DACH1 expression is regulated by promoter region hypermethylation in CRC. DACH1 methylation was associated with late tumor stage, poor differentiation, and lymph node metastasis. Re-expression of DACH1 reduced TCF/LEF luciferase reporter activity and inhibited the expression of Wnt signaling downstream targets (c-Myc and cyclinD1). In xenografts of HCT116 cells in which DACH1 was re-expressed, tumor size was smaller than in controls. In addition, restoration of DACH1 expression induced G2/M phase arrest and sensitized HCT116 cells to docetaxel. DACH1 suppresses CRC growth by inhibiting Wnt signaling both in vitro and in vivo. Silencing of DACH1 expression caused resistance of CRC cells to docetaxel. In conclusion, DACH1 is frequently methylated in human CRC and methylation of DACH1 may serve as detective and prognostic marker in CRC.
Introduction
Colorectal cancer (CRC) is the third most common cancer and the second leading cause of cancer-related death in the world.Citation1,Citation2 The incidence of CRC is increasing quickly in China, with the changing of life style. Even though the etiology of CRC remains unclear, aberrant genetic and epigenetic changes were regarded as important events of CRC development.Citation3-Citation6 Identification of novel epigenetic marker may improve the early detection, prognosis, and therapy.
TGF-β signaling plays a critical but paradoxical role in the predisposition and progression of colorectal cancer.Citation7 It involves several biological processes, including cell proliferation, differentiation, migration, and apoptosis. TGF-β signaling was regarded to play a tumor suppressor role in the early stages for most of tumors, whereas in a subset of advanced tumors, it facilitates EMT and TGF-β-dependent growth.Citation8-Citation11 The dichotomous nature of TGF-β in tumor development is known as the “TGF-β Paradox.”Citation12
Wnt signaling is involved in proliferation and differentiation of embryonic development and carcinogenesis and activating mutations of the Wnt signaling pathway may cause colorectal cancer.Citation13,Citation14 Activation of Wnt signaling will accumulate free β-catenin in cytoplasm and translocate it into the nucleus, and further cause transcription of downstream target genes.Citation15 In the absence of Wnt signal stimulation, β-catenin was regulated by a multiprotein complex, consisting of APC, axin, and the glycogen synthase kinase (GSK3β), leading to phosphorylation and proteasomal degradation.Citation16
Furuhashi M et al. reported stability of Smads in the TGF-β pathway can be regulated by axin.Citation17 Several studies have shown that TGF-β signaling synergizes with Wnt signaling in the regulation of cellular proliferation, differentiation, and colorectal cancer progression.Citation18-Citation22
Dachshund homolog 1 (DACH1), a homolog of Drosophila dac in human, is located in chromosome 13q22. Dac protein contains two conserved domains: dachbox-N and dachbox-C, both of which are highly conserved from Drosophila to humans. DACH1 is expressed widely in normal tissues and loss of DACH1 expression predicts poor outcome in breast, endometrial, and prostate cancers.Citation23-Citation26 It was reported that DACH1 inhibited the TGF-β pathway in breast cancer.Citation27
In this study, we analyzed epigenetic regulation and the function of DACH1 in human colorectal cancer, and we studied the chemosensitivity under epigenetic silencing DACH1 expression and restoration of DACH1 expression in colorectal cancer cells.
Results
DACH1 is silenced by promoter region hypermethylation in colorectal cancer cell line
Abnormal expression of DACH1 was found in some hormone-responsive cancers, but the regulation of DACH1 expression was unclear.Citation28 DACH1 expression was discovered in the human colorectal cancer cell lines LOVO, SW480, HT29, and RKO by semi-quantitative RT-PCR. Loss of DACH1 expression was detected in the HCT116 cell line (). Whereas complete methylation of the DACH1 genes was found in the HCT116 cell line, DACH1 was not methylated in LOVO, SW480, HT29, and RKO cell lines (). Loss of DACH1 expression was correlated with promoter region hypermethylation. To further study if DACH1 expression was regulated by promoter region hypermethylation, colorectal cancer cell lines were treated by 5-aza-2′-deoxycytidine (5-Aza). Re-expression of DACH1 was found in HCT116 cell line and no expression changes were revealed in LOVO, SW480, HT29, and RKO cell lines after 5-Aza treatment (). These results indicate that DACH1 expression is regulated by promoter region hypermethylation. To further validate the effect of methylation specific PCR (MSP) primer design and the methylation density of the DACH1 promoter region, we performed bisulfite sequencing (BSSQ; ). We observed dense methylation of the promoter region of DACH1 in HCT116 cells and no methylation of the same region in normal colonic mucosa and RKO cells.
Figure 1. Expression of DACH1 is downregulated by DNA methylation in colorectal cancer cell lines. (A) Expression of DACH1 was analyzed by semi-quantitative RT-PCR in colorectal cancer cell lines (LOVO, HT29, SW480, RKO, and HCT116). (B) CpG islands of the DACH1 gene locus predicted by “MethPrimer” (http://www.urogene.org/cgi-bin/methprimer/methprimer.cgi). CpG sites between -1000 and +1000 bp from the transcription start site (TSS) are presented by vertical bars. Sequence of methylation specific PCR (MSP) and bisulfite sequencing (BSSQ) products is shown. (C) Methylation status of the DACH1 promoter region in colorectal cancer cell lines. Primer efficiency was verified by positive control (in vitro methylated DNA, IVD) and negative control (normal blood lymphocyte DNA, NL). U indicates the presence of unmethylated alleles; M indicates the presence of methylated alleles. (D) Expression of DACH1 was analyzed by semi-quantitative RT-PCR in HCT116 and RKO cell lines in the absence or presence of 2 μmol/L 5-Aza (+) for 96 h. (E) Bisulfite sequencing of DACH1 was performed in HCT116, RKO cell lines, and normal colon mucosa. The region of CpG island studied by MSP is indicated by a double-headed arrow, spanning 130 bp. Filled circles represent methylated CpG sites within the DACH1 CpG island, and open circles denote unmethylated CpG sites. Bisulfite sequencing focused on a 286 bp (-426 bp to -140 bp) CpG island upstream of the DACH1 TST.
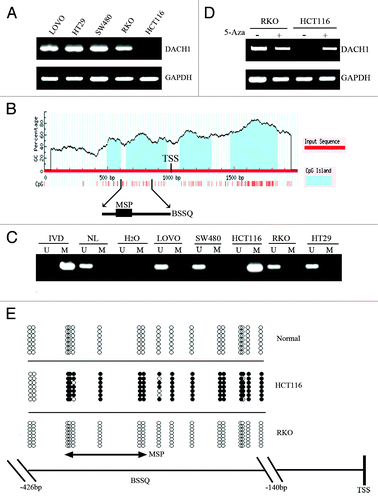
DACH1 is frequently methylated in primary colorectal cancer
To explore methylation changes in DACH1 during colorectal cancer development, 8 cases of normal colorectal mucosa, 15 cases of polyps and 100 cases of primary colorectal cancer were detected by MSP (). Whereas no methylation was found in normal colorectal mucosa, methylation was observed in 1 case of colon polyp (6.67%) and 48 cases of advanced colorectal cancer (48%). As shown in , methylation of DACH1 was significantly associated with late tumor stage (P < 0.001), poor differentiation (P < 0.001), and lymph node metastasis (P < 0.01) in CRC. No association was found between DACH1 methylation and age or gender. The results presented above suggest that methylation of DACH1 is associated with the progression of CRC. Methylation of DACH1 may serve as early detection and prognostic marker in CRC.
Figure 2. DNA methylation and expression of DACH1 in primary colorectal cancer. (A) Representative MSP results of DACH1 methylation status in normal colon mucosa (NC), colorectal adenomatous polyps (CP) and colorectal cancer tissues (CRC). (B) Representative images of DACH1 protein expression in colorectal cancer and their adjacent non-tumor tissues determined by IHC. (upper images, X200; lower images, X400). (C) DACH1 expression scores are shown as box plots, horizontal lines represent the median score; the bottom and top of the boxes representing the 25th and 75th percentiles, respectively; vertical bars represent the range of data. Expression of DACH1 was different between adjacent normal and tumor tissues in 30-matched primary CRCs. *P < 0.05. (D) The correlation between DACH1 hypermethylation and expression level was analyzed in 30-matched primary CRCs (**P < 0.01).
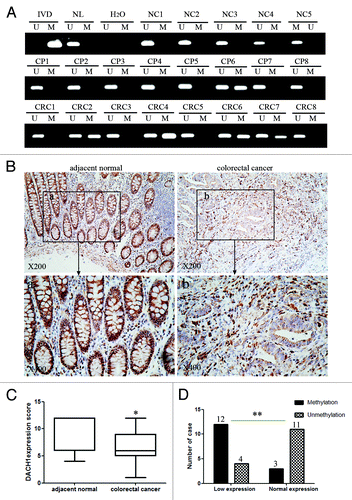
Table 1. Clinical characteristics and DACH1 methylation status of 100 patients with colorectal cancer
To explore the association of DACH1 expression and promoter region hypermethylation in primary colorectal cancer, DACH1 expression was evaluated by immunohistochemistry (IHC) in 30 cases of matched colorectal cancer and adjacent tissue samples. DACH1 staining was observed both in nucleus and cytoplasm. Reduced expression was found in 16 cases of cancer tissue, 12 of which were methylated (). Reduced DACH1 expression was significantly associated with methylation (P < 0.01; ). These results suggest that DACH1 expression is regulated by promoter region hypermethylation in primary colorectal cancer.
Restoration of DACH1 expression suppresses cell growth in CRC
To evaluate the effect of DACH1 on CRC carcinogenesis, cell viability and colony formation were evaluated before and after restoration of DACH1 expression in HCT116 cell line. Cell viability was reduced and colony formation was suppressed after re-expression of DACH1 in HCT116 cells (). These results indicate that DACH1 inhibits colorectal cancer cell proliferation.
Figure 3. Effect of DACH1 expression on tumor growth and cell cycle in HCT116 cells. (A) Growth curves, which represents the effect of DACH1 expression on cell viability in HCT116 cells was tested by CCK-8 kit daily for 96 h. (B) Colony formation assay shows the effect of DACH1 expression on cancer cell growth. The left panel shows the representative images of PCMV6-AC-DACH1-GFP or empty vector (PCMV6-AC-GFP) group. Right panel shows colony numbers (**P < 0.01). (C) Representative cell cycle and flow cytometry data (**P < 0.01). (D) western blot results in HCT116 cells with unexpressed DACH1 and re-expressed DACH1 . (E) The cell viability assay showed the effect of DACH1 restoration on the sensitivity of HCT116 cells to docetaxel (**P < 0.01).
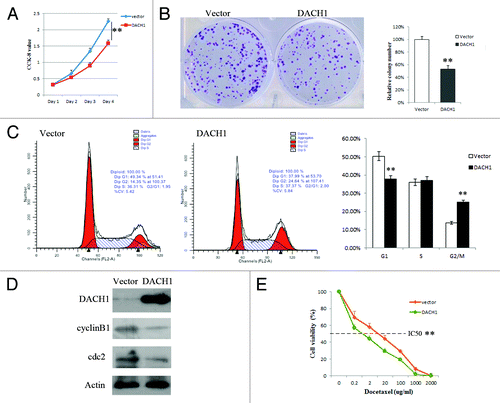
Restoration of DACH1 expression induced G2/M arrest and sensitivity to docetaxel in HCT116 cells
To explore the effect of DACH1 on cell cycle, we performed flow cytometry analyses () in HCT116 cells (not expressing DACH1) vs. HCT116 cells in which DACH1 was re-expressed. The distribution of cells in each cell cycle phase in these cells was as follows: G0/1 phase: 50.31 ± 1.01% vs. 37.83 ± 0.47%; S phase: 35.98 ± 0.47% vs. 37.13 ± 0.95%; G2/M phase: 13.71 ± 0.58% vs. 25.04 ± 0.59%. G0/1 phase was significantly reduced (P < 0.01) and G2/M phase was significantly increased (P < 0.01) after re-expression of DACH1. To further validate the effect of DACH1 expression on the cell cycle, the expression of cdc2 and cyclinB1, the mitosis initiators, was examined by western blot in HCT116 cells not expressing DACH1 and in cells in which DACH1 was re-expressed (). Expression of both cdc2 and cyclinB1 was apparently decreased after re-expression of DACH1. These results suggest that G2/M phase arrest is induced by suppression cdc2 and cyclinB1 expression after restoration of DACH1 expression. Docetaxel, a microtubule inhibitor, is related to G2/M checkpoint. To determine whether expression of DACH1 is related to docetaxel sensitivity, we examined the response to docetaxel in HCT116 cells not expressing DACH1 and cells in cells in which DACH1 was re-expressed (). The results showed that DACH1 expression increased the sensitivity of HCT116 cells to docetaxel (5.5 ± 0.378 vs. 1.069 ± 0.220 μg/ml, P < 0.01).
Silencing of DACH1 activates TGF-β signaling in CRC
DACH1 protein contains two domains: DachBox-N and DachBox-C. Human DachBox-N shares approximately 28% amino acid identity to SKI/SNO proteins, and is also known as SKI/SNO (or DS) domain.Citation29,Citation30 TGF-β signaling is inhibited by DACH1 DS domain in breast and ovarian cancer.Citation27,Citation31 To evaluate the effect of DACH1 on TGF-β signaling, dual-luciferase reporter assay was employed in HCT116 cells to analyze the function of full-length DACH1, DS (a DACH1 fragment that encodes the Smad binding protein domain), and ΔDS (fragment of DACH1 deleted for DS) (). SBE4-luciferase reporter activity was significantly inhibited by full-length DACH1 and DS, in a dose dependent manner (P < 0.01; ); SBE4 activity showed no changes before and after ΔDS transfection in different doses (P > 0.05). The results indicate that the effect of DACH1 in TGF-β signaling was dependent on the presence of the DS domain.
Figure 4. Effect of DACH1 on TGF-β signaling pathway. (A) Schematic structure of proteins expressed by DACH1, DS, and ΔDS vector. (B) Luciferase activity was detected in HCT116 cells transfected with empty vector and increasing doses of DACH1, DS, and ΔDS (50 ng, 100 ng, 200 ng/well for DACH1 and the same molecular for DS and ΔDS), with the addition of 5 ng/ml TGF-β1 in each group (**P < 0.01). (C) HCT116 cells were transfected with DACH1 with or without TGF-β1 addition, and indicated proteins were detected with the indicated antibodies by western blot. (D) western blot shows the expression of TGF-β signaling downstream genes before and after DACH1 was knocked down in HT29 cells.
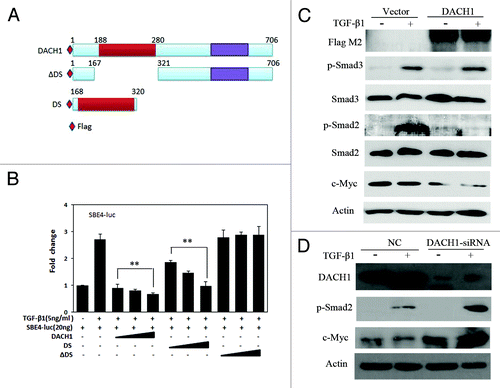
To further validate the effect of DACH1 on the TGF-β signaling pathway, phosphorylated Smad2, Smad3, and c-Myc were detected by western blot in HCT116 cells not expressing and expressing DACH1. Both phosphorylated Smad2 and c-Myc were apparently suppressed by DACH1 expression, but no correlation was found between the reduction of c-Myc expression and TGF-β1 stimulation (). The above results were further confirmed by DACH1 knock down in HT29 cells (). These results suggest that suppression of c-Myc expression by DACH1 may be mediated via a different pathway.
DACH1 inhibits Wnt/β-catenin signaling pathway in colorectal cancer
It was reported that TGF-β signaling could synergize with Wnt/β-catenin signaling through the activation of Wnt signaling directly by TGF-β1 protein in Xenopus and rat.Citation32,Citation33 As shown in , we looked at the activity of the Wnt signaling pathway using the Topflash/Fopflash reporter plasmid and the reporter (TCF/LEF) in HCT116 cells. Activity of Wnt signaling was significantly increased after co-transfection of the β-catenin expression vector with the Topflash reporter, while no change in Wnt signaling activity was observed after transfection of the β-catenin vector with Fopflash in HCT116 cells. TCF/LEF activity was increased by adding TGF-β1 in HCT116 cells not expressing DACH1, and this activation was inhibited by re-expression of DACH1 (). To further explore the effect of DACH1 on the Wnt/β-catenin signaling pathway, we looked at TCF/LEF luciferase reporter activity in HCT116 cells with silenced DACH1 and re-expressed DACH1. The activity of the TCF/LEF reporter was inhibited by co-transfection of β-catenin and full length DACH1 in a dose dependent manner ().
Figure 5. DACH1 represses Wnt/β-catenin signaling activity in colorectal cancer cells. (A) β-catenin expression vector was co-transfected with TCF/LEF Topflash reporter or its mutant, Fopflash, into HCT116 cells. Luciferase activity was normalized to Renilla luciferase activity (**P < 0.01). (B) Luciferase activity in mock, mock + TGF-β1, and DACH1 + TGF-β1 groups. (*P < 0.05, **P < 0.01). (C) Effect of increasing dose of DACH1 (50 ng, 100 ng, 200 ng/well) on Wnt signaling activity (**P < 0.01). (D) western blot showing the levels of p-β-catenin and Wnt signaling downstream genes, cyclinD1, and c-Myc, before and after DACH1 transfection in HCT116 cells. (E) western blot of HT29 cells before and after DACH1 was knocked down. The left panel shows the level of p-β-catenin was decreased after knocking down DACH1 in HT29 cells; the right panel shows the expression of c-Myc and cyclinD1, which was increased after knocking down DACH1 in HT29 cells.
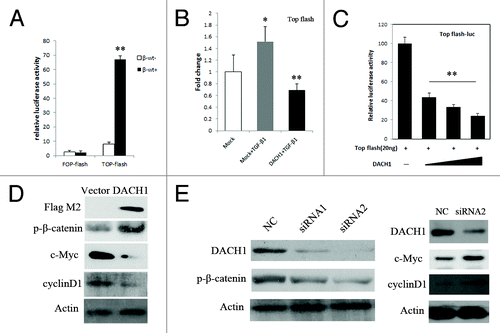
In the canonical Wnt/β-catenin pathway, increased β-catenin in the cytoplasm will promote translocation of β-catenin into the nucleus, and then activate TCF/LEF activity and downstream gene transcription. c-Myc and cyclinD1 are downstream targets of Wnt/β-catenin signaling. In our study, the expression levels of c-Myc and cyclinD1 were reduced, and phosphorylated β-catenin (p-β-catenin) was increased after re-expression of DACH1 (). P-β-catenin is the component representing degradation of β-catenin. The expression level of p-β-catenin was reduced, and the expression levels of c-Myc and cyclinD1 were increased in HT29 cells after DACH1 was knocked down (). These results suggest that DACH1 represseses the Wnt signaling pathway by phosphorylating β -catenin in colorectal cancer cell line.
DACH1 suppresses CRC growth in xenograft mice
To further explore the effect of DACH1 on CRC growth, a xenograft mice model was employed in which HCT116 cells not expressing and re-expressing DACH1 were used (). As shown in , the tumor size is smaller in HCT116 cell xenograft mice in which DACH1 was re-expressed than in non-expressing HCT116 cell xenograft mice (280.79 ± 90.27 mm3 vs 644.46 ± 227.55 mm3, P < 0.01), and the tumor weight is significantly lower in DACH1-expressing than in non-expressing HCT116 cell xenograft mice (274.29 ± 79.13 mg vs. 570.43 ± 64.25 mg, P < 0.001). We detected expression of DACH1 and increased p-β-catenin levels in DACH1-expressing HCT116 cells xenograft mice by IHC staining (). The above results suggest that DACH1 suppressed CRC growth in xenograft mice through the inhibition of Wnt signaling.
Figure 6. DACH1 retarded tumor growth in vivo. (A) Representative picture of tumor growth in nude mice subcutaneously inoculated with DACH1 expressing vector (right) and empty vector (left). (B) Subcutaneous tumor growth curve in xenograft mice with or without DACH1 expression. Retarded tumor growth was found in DACH1-expressing group. (C) Histogram represents average weight of xenograft in DACH1-expressing and DACH1-non-expressing groups. (D) Increased p-β-catenin was found in DACH1-expressing xenografts by IHC staining (x400).
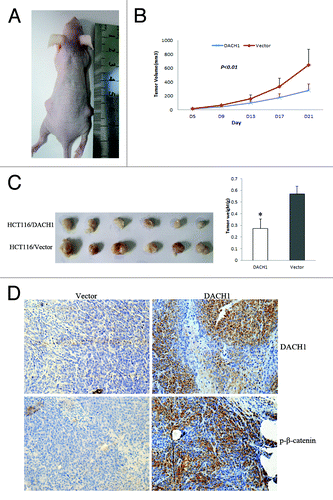
Discussion
Expression of DACH1 has previously been found to be reduced in breast, prostate, and endometrial cancer, but increased in ovarian cancer.Citation24,Citation27,Citation34,Citation35 In our study, we found that DACH1 expression was significantly reduced in human colorectal cancer, and that DACH1 was silenced by promoter region hypermethylation. We also report that DACH1 methylation was associated with tumor stage, poor differentiation, and lymph node metastasis. Methylation of DACH1 may therefore serve as potential detection and prognosis marker in colorectal cancer. DACH1 expression was found to suppress tumor growth both in vitro and in vivo in colorectal cancer. Our study suggests that DACH1 is a potential tumor suppressor in colorectal cancer.
Inhibition of the TGF-β signaling pathway by DACH1 has been found in both breast and ovarian cancer. DACH1 represses TGF-β signaling pathway by binding to Smad4 in breast cancer cells [27]. In our study, the level of phosphorylated Smad2 was reduced in colorectal cancer cells in which DACH1 was re-expressed. However, expression of c-Myc, the downstream gene of both TGF-β signaling and Wnt signaling, showed no obvious changes after addition of TGF-β1, indicating that c-Myc is not suppressed by TGF-β signaling. Cross-talk between the TGF-β and Wnt signaling pathways has been demonstrated during development and tumorigenesisCitation17-Citation19,Citation36-Citation38; therefore, DACH1 may be involved in Wnt signaling pathway in colorectal cancer. To test this hypothesis, the effect of DACH1 on Wnt signaling was analyzed. We employed the HCT116 cell line, in which DACH1 is methylated and silenced. Our results demonstrate that TCF/LEF reporter activity increased after addition TGF-β1 to HCT116 cells, and that activity was reduced after restoration of DACH1 expression. In addition to the TGF-β1-dependent effect, DACH1 inhibits the activity of TCF/LEF in a dose dependent manner in the absence of the TGF-β1 in HCT116 cells (). The HCT116 cell line carries one wild type and one mutant β-catenin allele.Citation39,Citation40 Methylation of DACH1 may cooperate with mutated β-catenin to stimulate Wnt signaling. After re-expression of DACH1 in HTC116 cells, the expression of c-Myc and cyclinD1 was reduced, whereas p-β-catenin was increased. The inhibitory effect of DACH1 on Wnt signaling was validated by knocking down DACH1 in HT29 cells. Increased p-β-catenin level by re-expression of DACH1 was also observed in vivo. Unlike β-catenin and APC mutations, which can occur in the adenomatous stage of colorectal neoplasia,Citation39,Citation40 DACH1 silencing was mostly observed in advanced tumors, which may contribute to invasive tumor phenotypes. DACH1 encodes a dachshund domain (DS domain), which binds to NCoR/HDAC. CyclinD1 and androgen receptor expression are regulated by DACH1/NCoR/HDAC complexes through their recruiting to promoter regions.Citation23,Citation36 It is possible that DACH1 regulates Wnt signaling by recruiting NCoR/HDAC to key pathway components. Understanding the function of major component in this signaling pathway may help design targeting therapies or preventive strategies in cancer.
Our study demonstrated that G2/M phase arrest was induced by DACH1 re-expression in HCT116 cells. As shown in , re-expression of DACH1 increased the sensitivity of HCT116 cells to docetaxel, a cytotoxic reagent that targets microtubules and causes cell cycle arrest at the G2/M phase.Citation41 This observation suggests that silencing of DACH1 by promoter region hypermethylation may induce resistance to chemotherapy in colorectal cancer cells.
In conclusion, DACH1 is frequently methylated in human colorectal cancer and hypermethylation of its promoter region silences DACH1 expression. DACH1 methylation is associated with tumor stage, poor differentiation and lymph node metastasis. Our results suggest that expression of DACH1 suppresses human colorectal cancer proliferation by inhibiting Wnt/β-catenin signaling pathways.
Material and Methods
Primary human colorectal cancer samples and cell lines
A total of 100 cases of primary colorectal cancer were collected as fresh frozen tissue from Chinese PLA General Hospital. All cases of colorectal cancer were classified by TNM stage, including stage I (n = 3), stage II (n = 50), stage III (n = 39), and stage IV (n = 8). Eight cases of normal colon tissue and 15 cases of colon polyps were collected as fresh frozen tissue by endoscopy biopsy in Chinese PLA General Hospital. Among 100 cancer samples, 30 cases of paraffin blocks are available with matched adjacent tissue. All samples were collected under the approved guidelines of the Chinese PLA General Hospital’s institutional review board.
Five colorectal cancer cell lines (LOVO, SW480, HT29, RKO, and HCT116) were included in this study. All colorectal cancer cell lines were previously established from primary colorectal cancer, and maintained in 90% RPMI 1640 (InvitrogenCA) supplementing with 10% fetal bovine serum. Cells were passaged 1:3 once total confluence (approximately 106 cells) was reached on a 75 cm2 culture flask (NEST Biotechnology).
5-aza-2′-deoxycytidine and docetaxel treatments
Colorectal cancer cell lines were split to low density (25% confluence) 12 h before treatment. Cells were treated with 5-aza-2′-deoxycytidine (5-Aza) (Sigma) at a concentration of 2 μM in the growth medium, which was exchanged every 24 h for a total 96-h treatment. At the end of treatment course, RNA was extracted. For the chemosensitivity assay, CRC cells, which had been transfected with DACH1-expression or control vectors 24 h before, were treated with docetaxcel at 7 concentrations (0, 0.02, 0.2, 2, 20, 100, 1000 μg/ml) for 48 h and then the cell viability was tested.
RNA isolation and semi-quantitative RT-PCR
Total RNA was isolated using Trizol reagent (Life Technologies). Agarose gel electrophoresis and spectrophotometric analysis were used to evaluate RNA quality and quantity. First strand cDNA was synthesized according to manufacturer’s instruction. A total of 5 μg total RNA was used to synthesize first strand cDNA using the Superscript III-reverse transcriptase kit (Invitrogen). The reaction mixture was then diluted to 100 μl with water. A total of 2.5 μl of diluted cDNA mixture was used for each 25-μl PCR reaction. PCR primers are as follow: 5′-CGTGAACAAG CAGAACAGAC G-3′(F) and 5′-CCCATGACGA ATGTCTGACT G-3′(R). A total of 30 cycles were performed. GAPDH was amplified using 25 cycles, as an internal control.
Bisulfite modification, methylation specific PCR (MSP) and bisulfite sequencing (BSSQ)
Genomic DNA was prepared by the proteinase-K method. Genomic DNA was bisulfite-modified as described previously.Citation42 MSP primers were designed according to genomic sequences around transcription start sites (TSS) and synthesized (Invitrogen) to detect unmethylated (U) and methylated (M) alleles. MSP primers are as follow: 5′- GGAAAAAATT ATTAGTTTTC GCGGAC-3′ (MF); 5′-AAACCGAAAA CACAAAAATA ACGATCG-3′ (MR); 5′- TTTGGAAAAA ATTATTAGTT TTTGTGGAT-3′ (UF) and 5′- AAAAAACCAA AAACACAAAA ATAACAATCA-3′ (UR). The expected sizes of the unmethylated and methylated PCR product are 130 bp and 124 bp, respectively. Bisulfite-treated DNA was subjected to PCR using primers flanking the targeted MSP regions. The sequencing primers used were: 5′-TGGYGGGYGA TTGTTTAGAA G-3′ (F) and 5′-ATATTAACCC AAATTATCCA ACC-3′ (R). Bisulfite sequencing was performed as previously described.Citation43
Immunohistochemistry (IHC)
Immunohistochemistry (IHC) was performed on 4 μm thick serial sections derived from formaldehyde-fixed paraffin blocks of colorectal cancer and paired adjacent tissue. After deparaffinization and rehydration, endogenous peroxidase activity was blocked for 30 min in methanol containing 0.3% hydrogen peroxide. After antigen retrieval, a cooling-off period of 20 min preceded the incubation with the primary antibody. Anti-DACH1 was used at a 1/400 dilution (Proteintech) overnight at 4 °C.
The staining intensity and extent of the stained area were graded according to the German semi-quantitative scoring system: staining intensity of the nucleus, cytoplasm, and/or membrane (no staining = 0; weak staining = 1; moderate staining = 2; strong staining = 3); extent of stained cells (0% = 0, 1–24% = 1, 25–49% = 2, 50–74% = 3, 75–100% = 4). The final immunoreactive score (0 to 12) was determined by multiplying intensity score to the extent of stained cells score.
Plasmid construction
The expression vectors for wild type DACH1 or mutants (DS and ΔDS) were gifts from Dr Cvekl and described previously.Citation44 Reporter constructs, including SBE-4-luc, which contains Smad-binding elements, TCF/LEF Topflash reporter, and Fopflash control, were described previously.Citation3 In addition, full-length DACH1 cDNA (GenBank accession number NM_080759.4) was cloned into the PCMV6-AC-GFP vector. The constructed plasmid was verified by DNA sequencing.
Cell viability assay
Cell viability was measured daily for 96 h using the Cell Counting Kit-8 (CCK8 Kit, Dojindo Laboratories) according to the manufacturer’s instruction. The results were plotted as means ± SD. For the chemosensitivity assay, HCT116 cells were seeded in 96-well plates and treated as above. The percentage of viable cells {[A450–630(treated) - A450–630(blank)]/ [A450–630(control) - A450–630(blank)] × 100%} was calculated. IC50 was deðned as the concentration that was required for 50% inhibition of cell growth. The values were calculated by nonlinear regression analysis using SPSS 15.0 software (SPSS Inc.).
Colony formation assay
HCT116 cells were grown in 6-well culture plates 24 h before transfection. Cells were transfected with PCMV6-AC-GFP vector or PCMV6-AC-DACH1-GFP, using FuGENE HD according to manufacturer’s instructions (Roche Applied Science). Cells were diluted and reseeded at 1500 cells per well in 6-well culture plates in triplicates 24 h later. Growth medium, conditioned with G418 (Invitrogen) at 500 μg/mL, was exchanged every 24 h. After 14 d, cells were fixed with 75% ethanol for 30 min and stained with 0.2% crystal violet for visualization and counting.
DACH1 knock down by siRNA
Four selected siRNAs targeting DACH1 and RNAi negative control duplex were used in this study. The sequences are as follows: siRNA duplex 1 (sense: 5′-GCCUCCUAAG AGGACUCAAT T-3′; antisense: 5′-UUGAGUCCUC UUAGGAGGCT T-3′); siRNA duplex 2 (sense: 5′-GCAGGAAGCA CUUGAGUUUT T-3′; antisense:5′-AAACUCAAGU GCUUCCUGCT T-3′). RNAi negative control duplex (sense: 5′-UUCUCCGAAC GUGUCACGUT T-3′; antisense: 5′-ACGUGACACG UUCGGAGAAT T-3). RNAi oligonucleotide or RNAi negative control duplex (GenePharma Co.) was transfected into HT29 cells according to the manufacturer’s instructions. The best result was obtained with siRNA duplex.
Flow cytometry analysis
Cell cycle analysis: after 12 h of synchronization by serum starvation, the stably transfected HCT116 cells (with PCMV6-AC-DACH1-GFP expression vector or PCMV6-AC-GFP empty vector) were re-stimulated with 10% fetal bovine serum (FBS) for 24 h. Cells were fixed in 70% ethanol and stained with 50 μg/ml propidium iodide (BD PharMingen). The cells were then sorted by FACS Calibur (BD Biosciences) and cell cycle profiles were analyzed by WinMDI v. 2.9 software (Scripps Research Institute).
Dual-Luciferase reporter assay
HCT116 cells were seeded at 1 × 104/well in 96-well plates and incubated for 24h, and then transiently transfected with an appropriate combination of the reporter, expression plasmids and control vector, including 20 ng/well SBE4-luc reporter plasmid, 2 ng/well pRL-TK as an internal reporter control and increasing amounts of full length DACH1, DS, and ΔDS (using 50 ng, 100 ng, 200 ng/well for DACH1 and 10 ng, 20 ng, 40 ng of well for DS, 40 ng, 80 ng, 160 ng of well for ΔDS). Then, cells were serum-starved for 36 h before they were stimulated with or without human recombinant TGF-β1 (Peprotech, Rocky Hill, NJ) for 12 h before luciferase assay.
In parallel, HCT16 cells were seeded at 5 × 103 cells/well in 96-well culture plates 24 h before transfection. To examine transcriptional activity driven by TCF/LEF, HCT116 cells were transfected with 20 ng/well Topflash reporter vector (TCF/LEF–responsive reporter); 2 ng/well pRL-TK and pCI-neo-β-catenin expressing vector (20 ng/well) were used to activate the reporter gene. As a negative control, another group of HCT116 cells were transfected with 20 ng/well Fopflash reporter vector, 2 ng/well pRL-TK control vector, and β-catenin expressing vector (20 ng/well). Then, increasing amounts of full length DACH1 (50 ng, 100 ng, 200 ng/well) were transfected into cells together with the Topflash reporter vector, pRL-TK control vector, and pCI-neo-β-catenin to evaluate the regulative function of DACH1 in the Wnt signaling pathway.
Forty-eight hours after transfection, relative luciferase activities were measured with the Dual Luciferase Reporter Assay System (Promega) according to the manufacturer’s protocol. For each experiment, the luciferase reporter assay was performed three times.
Protein preparation and western blotting
Transfected cells were lysed in ice-cold Tris buffer (20 mmol/L Tris; pH 7.5) containing 137 mmol/L NaCl, 2 mmol/L EDTA, 1% Triton X, 10% glycerol, 50 mmol/L NaF, 1 mmol/L DTT, and a protease inhibitor cocktail (Roche Applied Science). The protein lysates were then separated by SDS-PAGE and electroblotted onto PVDF membranes (Hybond-P). After blocking with 5% nonfat milk and 0.1%Tween-20 in TBS, the membranes were incubated with antibodies. The antibodies for immunoblot analysis were as follows: mouse anti-FLAG M2 (Sigma), rabbit anti-DACH1 (Proteintech), rabbit anti-cyclinD1 (Bioworld Technology), rabbit anti-c-Myc (Bioworld Technology), rabbit anti-cyclinB1 (Bioworld Technology), rabbit anto-cdc2 (Bioworld Technology), rabbit anti-phospho-Smad3 (Cell signaling Technology, Inc.), rabbit anti-phospho-Smad2 (Millipore), rabbit polyclonal anti-Smad3, anti-Smad2 (Bioworld Technology), and rabbit anti-phospho-β-catenin (Bioworld Technology).The blots were visualized using enhanced chemiluminescence (Pierce Bioscience).
In vivo tumorigenicity
HCT116 cells transfected with DACH1-expression vector or empty vector (2 × 106 cells in 0.2 ml phosphate-buffered saline) were injected subcutaneously into the dorsal right and left flank of 4-week-old male Balb/c nude mice (n = 6) respectively. Tumor volume was assessed every 2 d for 3 weeks starting 5 d after implantation. Tumor volume was calculated according to the formula: V = L × W2/2, where V indicates volume (mm3), L indicates biggest diameter (mm), and W indicates smallest diameter (mm). All procedures were approved by the Animal Ethics Committee of the Chinese PLA General Hospital.
Statistical Analysis
SPSS 15.0 software was employed and all data are presented as means ± standard deviation (SD) of at least three independent experiments. Statistical differences are presented as probability levels of P < 0.05, P < 0.01, and P < 0.001.
Abbreviations: | ||
DACH1 | = | Dachshund homolog 1 |
5-Aza | = | 5-aza-2’-deoxycytidine |
MSP | = | methylation specific polymerase chain reaction |
NL | = | normal lymphocyte DNA |
IVD | = | in vitro methylated DNA |
RT-PCR | = | reverse-transcription polymerase chain reaction |
TGF-β | = | transforming growth factor |
TCF | = | T-cell factor |
LEF | = | lymphoid enhancer factor |
Acknowledgments
Thanks Dr Cvekl for providing DACH1 expression vectors. This work was supported by grants from the National Basic Research Program of China (973 Program No. 2012CB934002, 2010CB912802); National High-tech R&D Program of China (863 Program No. SS2012AA020314, SS2012AA020821, SS2012AA020303); National Key Scientific instrument Special Programme of China (Grant No. 2011YQ03013405); National Science Foundation of China (Grant No. 81121004, 81071953, 81161120432, 81072169, 81172422, 81261120395).
Disclosure of Potential Conflicts of Interest
JGH is a consultant to MDxHealth. The other authors declare no conflict of interest.
Author Contributions
Yan W performed the experiments, analyzed the data and wrote the manuscript. Wu K, Herman JG, Brock MV, and Fuks F provided manuscript revision and experimental advice. Yang L, Zhu H, and Li Y helped with experimental procedures. Yang Y supervised the project. Guo M designed the project and edited the manuscript.
References
- Jemal A, Siegel R, Ward E, Hao Y, Xu J, Thun MJ. Cancer statistics, 2009. CA Cancer J Clin 2009; 59:225 - 49; http://dx.doi.org/10.3322/caac.20006; PMID: 19474385
- Ferlay J, Shin HR, Bray F, Forman D, Mathers C, Parkin DM. Estimates of worldwide burden of cancer in 2008: GLOBOCAN 2008. Int J Cancer 2010; 127:2893 - 917; http://dx.doi.org/10.1002/ijc.25516; PMID: 21351269
- Zhang W, Glöckner SC, Guo M, Machida EO, Wang DH, Easwaran H, Van Neste L, Herman JG, Schuebel KE, Watkins DN, et al. Epigenetic inactivation of the canonical Wnt antagonist SRY-box containing gene 17 in colorectal cancer. Cancer Res 2008; 68:2764 - 72; http://dx.doi.org/10.1158/0008-5472.CAN-07-6349; PMID: 18413743
- Riojas MA, Guo M, Glöckner SC, Machida EO, Baylin SB, Ahuja N. Methylation-induced silencing of ASC/TMS1, a pro-apoptotic gene, is a late-stage event in colorectal cancer. Cancer Biol Ther 2007; 6:1710 - 6; http://dx.doi.org/10.4161/cbt.6.11.4829; PMID: 17986858
- Hanada N, Takahata T, Zhou Q, Ye X, Sun R, Itoh J, Ishiguro A, Kijima H, Mimura J, Itoh K, et al. Methylation of the KEAP1 gene promoter region in human colorectal cancer. BMC Cancer 2012; 12:66; http://dx.doi.org/10.1186/1471-2407-12-66; PMID: 22325485
- Dallosso AR, Øster B, Greenhough A, Thorsen K, Curry TJ, Owen C, Hancock AL, Szemes M, Paraskeva C, Frank M, et al. Long-range epigenetic silencing of chromosome 5q31 protocadherins is involved in early and late stages of colorectal tumorigenesis through modulation of oncogenic pathways. Oncogene 2012; 31:4409 - 19; http://dx.doi.org/10.1038/onc.2011.609; PMID: 22249255
- Xu Y, Pasche B. TGF-beta signaling alterations and susceptibility to colorectal cancer. Hum Mol Genet 2007; 16:Spec No 1 R14 - 20; http://dx.doi.org/10.1093/hmg/ddl486; PMID: 17613544
- Massagué J, Blain SW, Lo RS. TGFbeta signaling in growth control, cancer, and heritable disorders. Cell 2000; 103:295 - 309; http://dx.doi.org/10.1016/S0092-8674(00)00121-5; PMID: 11057902
- Azuma H, Ehata S, Miyazaki H, Watabe T, Maruyama O, Imamura T, Sakamoto T, Kiyama S, Kiyama Y, Ubai T, et al. Effect of Smad7 expression on metastasis of mouse mammary carcinoma JygMC(A) cells. J Natl Cancer Inst 2005; 97:1734 - 46; http://dx.doi.org/10.1093/jnci/dji399; PMID: 16333029
- Leivonen SK, Ala-Aho R, Koli K, Grénman R, Peltonen J, Kähäri VM. Activation of Smad signaling enhances collagenase-3 (MMP-13) expression and invasion of head and neck squamous carcinoma cells. Oncogene 2006; 25:2588 - 600; http://dx.doi.org/10.1038/sj.onc.1209291; PMID: 16407850
- Leivonen SK, Kähäri VM. Transforming growth factor-beta signaling in cancer invasion and metastasis. Int J Cancer 2007; 121:2119 - 24; http://dx.doi.org/10.1002/ijc.23113; PMID: 17849476
- Tian M, Schiemann WP. The TGF-beta paradox in human cancer: an update. Future Oncol 2009; 5:259 - 71; http://dx.doi.org/10.2217/14796694.5.2.259; PMID: 19284383
- Kongkanuntn R, Bubb VJ, Sansom OJ, Wyllie AH, Harrison DJ, Clarke AR. Dysregulated expression of beta-catenin marks early neoplastic change in Apc mutant mice, but not all lesions arising in Msh2 deficient mice. Oncogene 1999; 18:7219 - 25; http://dx.doi.org/10.1038/sj.onc.1203181; PMID: 10602475
- Thorstensen L, Lind GE, Løvig T, Diep CB, Meling GI, Rognum TO, Lothe RA. Genetic and epigenetic changes of components affecting the WNT pathway in colorectal carcinomas stratified by microsatellite instability. Neoplasia 2005; 7:99 - 108; http://dx.doi.org/10.1593/neo.04448; PMID: 15802015
- Van der Flier LG, Sabates-Bellver J, Oving I, Haegebarth A, De Palo M, Anti M, Van Gijn ME, Suijkerbuijk S, Van de Wetering M, Marra G, et al. The Intestinal Wnt/TCF Signature. Gastroenterology 2007; 132:628 - 32; http://dx.doi.org/10.1053/j.gastro.2006.08.039; PMID: 17320548
- Orford K, Crockett C, Jensen JP, Weissman AM, Byers SW. Serine phosphorylation-regulated ubiquitination and degradation of beta-catenin. J Biol Chem 1997; 272:24735 - 8; http://dx.doi.org/10.1074/jbc.272.40.24735; PMID: 9312064
- Furuhashi M, Yagi K, Yamamoto H, Furukawa Y, Shimada S, Nakamura Y, Kikuchi A, Miyazono K, Kato M. Axin facilitates Smad3 activation in the transforming growth factor beta signaling pathway. Mol Cell Biol 2001; 21:5132 - 41; http://dx.doi.org/10.1128/MCB.21.15.5132-5141.2001; PMID: 11438668
- Nishita M, Hashimoto MK, Ogata S, Laurent MN, Ueno N, Shibuya H, Cho KW. Interaction between Wnt and TGF-beta signalling pathways during formation of Spemann’s organizer. Nature 2000; 403:781 - 5; http://dx.doi.org/10.1038/35001602; PMID: 10693808
- Labbé E, Lock L, Letamendia A, Gorska AE, Gryfe R, Gallinger S, Moses HL, Attisano L. Transcriptional cooperation between the transforming growth factor-beta and Wnt pathways in mammary and intestinal tumorigenesis. Cancer Res 2007; 67:75 - 84; http://dx.doi.org/10.1158/0008-5472.CAN-06-2559; PMID: 17210685
- Hussein SM, Duff EK, Sirard C. Smad4 and beta-catenin co-activators functionally interact with lymphoid-enhancing factor to regulate graded expression of Msx2. J Biol Chem 2003; 278:48805 - 14; http://dx.doi.org/10.1074/jbc.M305472200; PMID: 14551209
- Letamendia A, Labbé E, Attisano L. Transcriptional regulation by Smads: crosstalk between the TGF-beta and Wnt pathways. J Bone Joint Surg Am 2001; 83-A:Suppl 1 S31 - 9; PMID: 11263663
- Eger A, Stockinger A, Park J, Langkopf E, Mikula M, Gotzmann J, Mikulits W, Beug H, Foisner R. beta-Catenin and TGFbeta signalling cooperate to maintain a mesenchymal phenotype after FosER-induced epithelial to mesenchymal transition. Oncogene 2004; 23:2672 - 80; http://dx.doi.org/10.1038/sj.onc.1207416; PMID: 14755243
- Wu K, Li A, Rao M, Liu M, Dailey V, Yang Y, Di Vizio D, Wang C, Lisanti MP, Sauter G, et al. DACH1 is a cell fate determination factor that inhibits cyclin D1 and breast tumor growth. Mol Cell Biol 2006; 26:7116 - 29; http://dx.doi.org/10.1128/MCB.00268-06; PMID: 16980615
- Nan F, Lü Q, Zhou J, Cheng L, Popov VM, Wei S, Kong B, Pestell RG, Lisanti MP, Jiang J, et al. Altered expression of DACH1 and cyclin D1 in endometrial cancer. Cancer Biol Ther 2009; 8:1534 - 9; http://dx.doi.org/10.4161/cbt.8.16.8963; PMID: 19502783
- Wu K, Liu M, Li A, Donninger H, Rao M, Jiao X, Lisanti MP, Cvekl A, Birrer M, Pestell RG. Cell fate determination factor DACH1 inhibits c-Jun-induced contact-independent growth. Mol Biol Cell 2007; 18:755 - 67; http://dx.doi.org/10.1091/mbc.E06-09-0793; PMID: 17182846
- Yamada Y, Arao T, Gotoda T, Taniguchi H, Oda I, Shirao K, Shimada Y, Hamaguchi T, Kato K, Hamano T, et al. Identification of prognostic biomarkers in gastric cancer using endoscopic biopsy samples. Cancer Sci 2008; 99:2193 - 9; http://dx.doi.org/10.1111/j.1349-7006.2008.00935.x; PMID: 18957060
- Wu K, Yang Y, Wang C, Davoli MA, D’Amico M, Li A, Cveklova K, Kozmik Z, Lisanti MP, Russell RG, et al. DACH1 inhibits transforming growth factor-beta signaling through binding Smad4. J Biol Chem 2003; 278:51673 - 84; http://dx.doi.org/10.1074/jbc.M310021200; PMID: 14525983
- Popov VM, Wu K, Zhou J, Powell MJ, Mardon G, Wang C, Pestell RG. The Dachshund gene in development and hormone-responsive tumorigenesis. Trends Endocrinol Metab 2010; 21:41 - 9; http://dx.doi.org/10.1016/j.tem.2009.08.002; PMID: 19896866
- Kim SS, Zhang RG, Braunstein SE, Joachimiak A, Cvekl A, Hegde RS. Structure of the retinal determination protein Dachshund reveals a DNA binding motif. Structure 2002; 10:787 - 95; http://dx.doi.org/10.1016/S0969-2126(02)00769-4; PMID: 12057194
- Hammond KL, Hanson IM, Brown AG, Lettice LA, Hill RE. Mammalian and Drosophila dachshund genes are related to the Ski proto-oncogene and are expressed in eye and limb. Mech Dev 1998; 74:121 - 31; http://dx.doi.org/10.1016/S0925-4773(98)00071-9; PMID: 9651501
- Sunde JS, Donninger H, Wu K, Johnson ME, Pestell RG, Rose GS, Mok SC, Brady J, Bonome T, Birrer MJ. Expression profiling identifies altered expression of genes that contribute to the inhibition of transforming growth factor-beta signaling in ovarian cancer. Cancer Res 2006; 66:8404 - 12; http://dx.doi.org/10.1158/0008-5472.CAN-06-0683; PMID: 16951150
- Zhou B, Liu Y, Kahn M, Ann DK, Han A, Wang H, Nguyen C, Flodby P, Zhong Q, Krishnaveni MS, et al. Interactions between β-catenin and transforming growth factor-β signaling pathways mediate epithelial-mesenchymal transition and are dependent on the transcriptional co-activator cAMP-response element-binding protein (CREB)-binding protein (CBP). J Biol Chem 2012; 287:7026 - 38; http://dx.doi.org/10.1074/jbc.M111.276311; PMID: 22241478
- Labbé E, Letamendia A, Attisano L. Association of Smads with lymphoid enhancer binding factor 1/T cell-specific factor mediates cooperative signaling by the transforming growth factor-beta and wnt pathways. Proc Natl Acad Sci U S A 2000; 97:8358 - 63; http://dx.doi.org/10.1073/pnas.150152697; PMID: 10890911
- Wu K, Katiyar S, Witkiewicz A, Li A, McCue P, Song LN, Tian L, Jin M, Pestell RG. The cell fate determination factor dachshund inhibits androgen receptor signaling and prostate cancer cellular growth. Cancer Res 2009; 69:3347 - 55; http://dx.doi.org/10.1158/0008-5472.CAN-08-3821; PMID: 19351840
- Liang F, Lü Q, Sun S, Zhou J, Popov VM, Li S, Li W, Liu Y, Jiang J, Kong B. Increased expression of dachshund homolog 1 in ovarian cancer as a predictor for poor outcome. Int J Gynecol Cancer 2012; 22:386 - 93; http://dx.doi.org/10.1097/IGC.0b013e31824311e6; PMID: 22367319
- Dong Y, Drissi H, Chen M, Chen D, Zuscik MJ, Schwarz EM, O’Keefe RJ. Wnt-mediated regulation of chondrocyte maturation: modulation by TGF-beta. J Cell Biochem 2005; 95:1057 - 68; http://dx.doi.org/10.1002/jcb.20466; PMID: 15962307
- Minoo P, Li C. Cross-talk between transforming growth factor-beta and Wingless/Int pathways in lung development and disease. Int J Biochem Cell Biol 2010; 42:809 - 12; http://dx.doi.org/10.1016/j.biocel.2010.02.011; PMID: 20219694
- Caraci F, Gili E, Calafiore M, Failla M, La Rosa C, Crimi N, Sortino MA, Nicoletti F, Copani A, Vancheri C. TGF-beta1 targets the GSK-3beta/beta-catenin pathway via ERK activation in the transition of human lung fibroblasts into myofibroblasts. Pharmacol Res 2008; 57:274 - 82; http://dx.doi.org/10.1016/j.phrs.2008.02.001; PMID: 18346908
- Morin PJ, Sparks AB, Korinek V, Barker N, Clevers H, Vogelstein B, Kinzler KW. Activation of beta-catenin-Tcf signaling in colon cancer by mutations in beta-catenin or APC. Science 1997; 275:1787 - 90; http://dx.doi.org/10.1126/science.275.5307.1787; PMID: 9065402
- Sparks AB, Morin PJ, Vogelstein B, Kinzler KW. Mutational analysis of the APC/beta-catenin/Tcf pathway in colorectal cancer. Cancer Res 1998; 58:1130 - 4; PMID: 9515795
- Armand JP. [Focus on cellular pharmacology of docetaxel]. Bull Cancer 2003; 90:1067 - 70; PMID: 14715427
- Herman JG, Graff JR, Myöhänen S, Nelkin BD, Baylin SB. Methylation-specific PCR: a novel PCR assay for methylation status of CpG islands. Proc Natl Acad Sci U S A 1996; 93:9821 - 6; http://dx.doi.org/10.1073/pnas.93.18.9821; PMID: 8790415
- Jia Y, Yang Y, Liu S, Herman JG, Lu F, Guo M. SOX17 antagonizes WNT/β-catenin signaling pathway in hepatocellular carcinoma. Epigenetics 2010; 5:743 - 9; http://dx.doi.org/10.4161/epi.5.8.13104; PMID: 20716954
- Wu K, Yang Y, Wang C, Davoli MA, D’Amico M, Li A, Cveklova K, Kozmik Z, Lisanti MP, Russell RG, et al. DACH1 inhibits transforming growth factor-beta signaling through binding Smad4. J Biol Chem 2003; 278:51673 - 84; http://dx.doi.org/10.1074/jbc.M310021200; PMID: 14525983