Abstract
RNA silencing processes use exogenous or endogenous RNA molecules to specifically and robustly regulate gene expression. In C. elegans, initial mechanistic descriptions of the different silencing processes focused on posttranscriptional regulation. In this review, we discuss recent work showing that, in this model organism, RNA silencing also controls the transcription of target genes by inducing heterochromatin formation. Specifically, it has been shown that ribonucleoprotein complexes containing small RNAs, either processed from exogenous dsRNA or synthesized from the genome itself, and proteins of the Argonaute family, mediate the deposition of repressive histone marks at the targeted loci. Interestingly, the accumulation of repressive marks is required for the inheritance of the silencing effect and the establishment of an epigenetic memory that discriminates self- from non-self-RNAs.
Introduction
In 2006, Andrew Fire and Craig Mello were awarded the Nobel Prize in Medicine for their discovery of RNA interference (RNAi), a gene silencing process induced by double-stranded RNA (dsRNA). This discovery led to the development of novel methods to knockdown genes of interest and revolutionized the way researchers analyze gene function. Furthermore, it opened new therapeutic perspectives in clinical medicine.
The original studies, performed in the nematode Caenorhabditis elegans, described a posttranscriptional gene silencing (PTGS) mechanism triggered by the introduction (through feeding, soaking or injection) of long dsRNA molecules. Forward and reverse genetic screens identified a first set of factors required for efficient silencing, and based on their functional characterization, a model for RNAi was proposed.Citation1 According to this model, the processing of dsRNA into small interfering RNAs (known as primary siRNA) is mediated by a complex that includes DCR-1, an ATP-dependent ribonuclease, RDE-4, a dsRNA binding protein, and RDE-1, a member of the Argonaute family defined by the presence of a Piwi Argonaute Zwille (PAZ) and a PIWI (P-element induced wimpy testis) domain (). AGO proteins have been widely conserved during evolution and are involved in many RNAi related processes through binding of small RNAs. In C. elegans, RDE-1 binds single-stranded primary siRNAs and targets them to their complementary sequences present in mRNAs. Interaction between the primary siRNA/RDE-1 complex and target mRNAs promotes the recruitment of an RNA-directed RNA polymerase (RdRP), encoded by rrf-1 in somatic cells and ego-1 in the germline, which synthesizes de novo dsRNA using the targeted mRNA as template. A ribonuclease activity then processes the newly synthesized dsRNA into secondary siRNAs characterized by a 22-nucleotide long sequence and a 5′ guanosine (22G-RNA). This positive amplification loop triggered by RdRPs promotes the synthesis of a large number of secondary siRNAs that bind a group of AGO proteins including SAGO-1, SAGO-2, and PPW-1 to target their cognate mRNAs for degradation, resulting in PTGS.Citation2
Figure 1. RNAi and nuclear RNAi pathways in somatic cells. Exogenous dsRNAs enter the cytoplasm and are cleaved by DCR-1 to produce primary siRNAs that are targeted to cognate mRNA by the Argonaute protein RDE-1. Secondary siRNAs are synthesized by the RNA-dependent RNA polymerase RRF-1 using the targeted mRNA as a template. Secondary siRNAs bind to a group of Argonaute proteins including SAGO-1 and SAGO-2 and target mRNAs for degradation resulting in posttranscriptional gene silencing. Secondary siRNA can also bind NRDE-3 and translocate into the nucleus. If the secondary siRNAs bound to NRDE-3 are complementary to a nascent mRNA transcript, they will result in transcriptional gene silencing promoted by the NRDE machinery and mediated by histone 3 lysine 9 methylation.
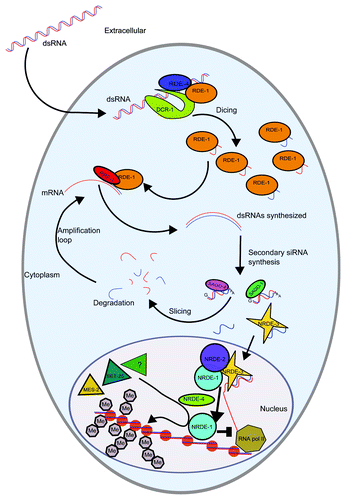
In yeast and Drosophila, RNAi and related silencing processes also induce heterochromatin formation.Citation3 Although in C. elegans, chromatin factors and chromatin modifying enzymes have previously been implicated in RNAi and related processes,Citation4-Citation7 until recently direct evidence that chromatin modifications are present at the targeted endogenous loci was lacking.
RNAi induces heterochromatin formation in C. elegans
Genome-wide chromatin profiling shows that RNAi induces heterochromatin formation
To investigate whether the formation of heterochromatin domains plays a role in the response to dsRNA treatment, the group of Andrew Fire performed chromatin immunoprecipitation of nucleosome core particles followed by high-throughput sequencing.Citation8 This work showed that RNAi can induce trimethylation of lysine 9 of histone H3 (H3K9me3), a mark associated with heterochromatin, specifically at the RNAi-targeted locus. This de novo heterochromatin formation was found to spread into the flanking sequences as far as 9kb from the targeted region. It was further shown that during this process, secondary siRNA accumulation precedes histone methylation, and that H3K9me3 deposition is dependent on (1) RDE-1, (2) secondary siRNAs-interacting Argonaute proteins and (3) the NRDE pathway,Citation9 discussed in the following section.
The NRDE machinery promotes heterochromatin formation during RNAi
Screens performed in the laboratory of Scott Kennedy for mutant animals that failed to silence nuclear-localized RNAs in response to RNAi, but retained the ability to silence cytoplasmic RNAs, identified the nrde (for nuclear RNAi defective) genes. nrde genes encode factors essential for RNAi-induced heterochromatin formation (). Based on analysis of nrde-1 to 4,Citation8-Citation10 a model was proposed for how the NRDE pathway contributes to nuclear RNAi (). Key to this process is NRDE-3/WAGO-12, an Argonaute protein that contains a Nuclear Localization Signal (NLS) in addition to the PAZ and PIWI domains and is exclusively expressed in somatic cells after the ~80 cells stage of embryogenesis.Citation9 NRDE-3 binding of cytoplasmic secondary-siRNAs produced by the RdRP RRF-1 triggers translocation of the complex from the cytoplasm to the nucleus. In the nucleus, the NRDE-3/siRNAs complex is bound to complementary nascent pre-mRNA transcripts synthesized by RNA polymerase II (RNAP II), and promotes NRDE-2 and NRDE-1 recruitment to nascent transcripts.Citation10,Citation11 NRDE-1 is then targeted to chromatin in an NRDE-4 dependent manner, followed by deposition of H3K9me3. H3K9me3 and NRDE-1 work together to inhibit RNAP II elongation, but whether NRDE-1 directly recruits the H3K9me3 HMT to the site of RNAi, or whether H3K9me3 is required to recruit NRDE-1 to chromatin, remains unknown.
Table 1. Brief description of the main proteins discussed in this review
The HMT responsible for catalyzing RNAi-induced H3K9me3 deposition remains to be identified. At least 35 proteins encoded by the C. elegans genome carry a catalytic SET domain responsible for lysine methylation. Based on sequence homology, 13 of these, including SET-25 and SET-32, are potential H3K9 HMTs. SET-25 was shown to efficiently catalyze H3K9me3 in embryos, and together with the H3K9me1/2 HMTase MET-2, is required to silence repetitive transgenes and sequester transcriptionally inactive heterochromatin at the nuclear periphery via binding to the nuclear lamin.Citation12 In the germline, H3K9me3 is partially dependent upon MES-2, a HMTase belonging to the conserved Polycomb Repressive Complex 2 (PRC2) and initially characterized for its role in the deposition of both H3K27 di- and tri-methylation, two additional repressive marks.Citation13,Citation14 In the absence of MES-2, most germline H3K9me3 disappears. However, residual H3K9me3 can still be detected on mitotic chromosomes and on meiotic chromosomes at diplotene and diakinesis, indicating that a subset of germline H3K9me3 is MES-2 independent. Although so far MES-2 has not been implicated in RNAi-induced heterochromatin formation, indirect evidence (see below) suggests that SET-25 and SET-32 may be required for this process.Citation15 In the future, it will be interesting to ask whether other repressive heterochromatin marks (such as H3K27 methylation) are also involved in RNAi-induced transcriptional silencing, and if so to test the mechanism and developmental timing of their establishment.
Interestingly, recent data shows that in C. elegans an endogenous nuclear RNAi pathway that relies on the NRDE machinery promotes olfactory adaptation to butanone in AWC olfactory sensory neurons ,Citation16 providing an exciting link between environmental cues and behavior. In AWC neurons, NRDE-3 loads with 22G-RNAs targeting the guanyl cyclase ODR-1 mRNAs, whose downregulation is required for odor adaptation. In addition, adaptation requires H3K9 methylation and binding of HPL-2, the homolog of the H3K9me3 reader HP1Citation17 , at the odr-1 locus. Altogether, these results suggest that NRDE-dependent heterochromatin formation is an essential part of transcriptional repression of odr-1 in the odor adaptation process.
Nuclear RNAi-induced H3K9 methylation mediates RNAi heritability
Following its discovery, RNAi quickly became a tool of choice to knockdown gene expression. In the course of RNAi experiments in C. elegans, it was observed that, at least in some case, the RNAi effect could persist for several generations in the progeny of dsRNA-treated animals, in the absence of the dsRNA trigger.Citation7,Citation18,Citation19
Small RNA-directed chromatin modifications appeared as likely candidates in mediating RNAi heritability. To directly test this, maintenance of the heterochromatin state at the RNAi target gene was monitored in the absence of continuous RNAi treatment, in the progeny of RNAi-treated animals. H3K9me3 levels in the F1 progeny of dsRNA-treated animals were significantly more abundant than in the parental RNAi-treated animalsCitation8,Citation20 showing that RNAi can induce heritable chromatin modifications.
The role of the NRDE machinery in RNAi heritability was assayed by testing animals defective for nuclear RNAi for their ability to inherit RNAi silencing.Citation20 In this experiment, a reporter transgene expressing GFP in all somatic cells from the later stages of embryogenesis to adulthood was introduced in nrde defective backgrounds. In a wild type background, GFP expression is silenced in the presence of a dsRNA trigger and silencing is transmitted to non-treated F1 progeny (). In nrde mutants, GFP silencing was also observed in the non-treated F1 progeny at the embryonic stage. However, in contrast to wild type, GFP silencing was not maintained during development of the F1 progeny, and GFP expression was observed in adult somatic cells. These results show that in the absence of the NRDE machinery, RNAi silencing is established and inherited, but cannot be maintained during larval development of the progeny. Consistent with the idea that nuclear RNAi-induced H3K9me3 mediates RNAi heritability, it was shown that nrde-1, nrde-3, and nrde-4 mutants defective for RNAi inheritance fail to induce heritable H3K9me3.
Figure 2. RNAi inheritance in the soma of C. elegans. At the P0 generation, RNAi is established by feeding wild type worms with a dsRNA molecule that specifically targets a single gene. Silencing can be inherited for several generations in the absence of the dsRNA trigger. Secondary siRNAs and H3K9me3 are required to transmit the RNAi silencing effect to the progeny. Gene expression is represented in green. In nrde defective animals, RNAi silencing is established in the treated animals and transmitted to the embryos, but fails to be maintained in later developemental stages.
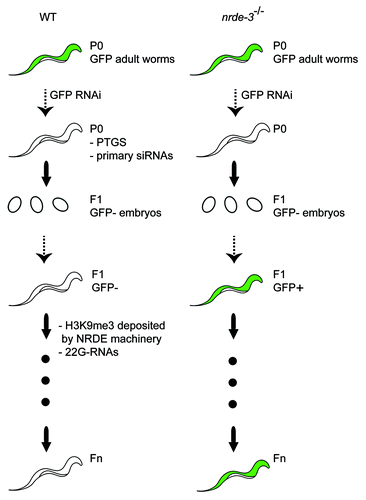
Formal proof for small RNAs being involved in heritable gene silencing came from high-throughput sequencing of small RNAs derived from dsRNA-treated animals and their non-treated silenced progeny.Citation15 In treated animals, primary siRNAs were mostly detected. In contrast, F4 animals contained only secondary siRNAs (22G-RNA), generated de novo at each generation. Interestingly, the NRDE machinery is not essential for 22G-RNA accumulation, showing that this pathway acts downstream of siRNA expression.
NRDE-3 is uniquely expressed in the soma. However, RNAi inheritance is also observed in the germline. Using reporter transgenes specifically expressing a GFP reporter gene in the germline, it was shown that in this tissue, NRDE-3 is replaced by HRDE-1 (for heritable RNAi deficient, also known as WAGO-9), another nuclear Argonaute protein.Citation11,Citation15 NRDE-3 and HRDE-1 seem to play very similar roles. However, whereas NRDE-3 can translocate from the cytoplasm to the nucleus, HRDE-1 seems to be constitutively localized in the nucleus. This observation leaves open the question as to how siRNAs are recruited to HRDE-1 and the NRDE machinery during nuclear RNAi in the germline, and strongly suggests that HRDE-1 might be constitutively linked to siRNA independently of RNAi treatment and play an essential role in C. elegans development. Consistent with such a function, endogenous 22G-RNAs are potentially able to target C. elegans genes through HRDE-1 binding (see below).
Genomically encoded PIWI-interacting small RNAs play cellular functions mediated by the NRDE machinery
The C. elegans genome encodes endogenous small RNAs that bind the PIWI protein PRG-1
Large-scale sequencing experiments of small RNAs in C. elegans revealed the existence of a class of non-coding small RNAs called 21U-RNAsCitation21 that structurally differ from other endogenous small RNAs. 21U-RNAs are precisely 21 nucleotides long, begin with a uracil 5′-monophosphate and are also modified on their 3′ ribose. Over 15 000 unique 21U-RNAs have been identified so far, and the large majority has been mapped to three regions on chromosome IV, respectively spanning coordinates 4.5–7.0 M, 9–9.7 M, and 13.5–17.2 M (). These 3 regions are relatively poor in genes compared with the whole genome and most of the 21U-RNAs mapped either within introns or in intergenic regions. Finally, 21U-RNAs were shown to be mostly expressed in the germline.Citation22 Accumulation of 21U-RNAs depends on PRG-1, an Argonaute protein expressed in the germline. Within the Argonaute family, PRG-1 is a member of the Piwi group known to interact with endogenously expressed small RNAs named piRNA (for Piwi interacting RNA). piRNA are found in many species and are involved in various silencing processes.Citation23 Based on their physical interaction with PRG-1, 21U-RNAs are now considered to be bona fide piRNAs. PRG-1 is localized in P-granules, perinuclear cytoplasmic “germ granules” implicated in germline specification and gene silencing process.
Figure 3. Genome-wide surveillance of germline transcripts by PRG-1 (and CSR-1). (A) Primary siRNAs (21U RNAs) are transcribed from three clusters on chromosome IV and bound by PRG-1. (B) The association between PRG-1/siRNAs and mRNA induces 22G-RNA synthesis and inhibition of targeted genes. In the absence of mismatches between CSR-1/22G-RNAs and mRNAs, mRNAs are protected against PRG-1. However, a binding without mismatch between PRG-1/siRNAs and an mRNA overcomes the CSR-1 protection.
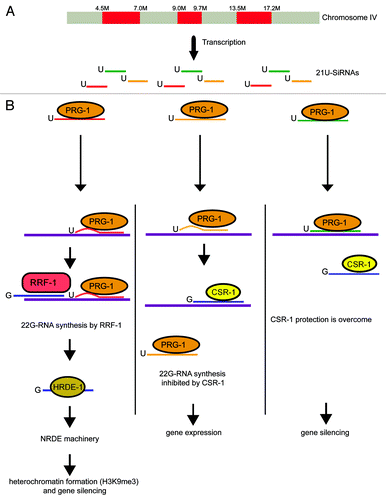
C. elegans piRNAs establish an epigenetic memory of nonself RNAs
Recent studiesCitation15,Citation22,Citation24-Citation26 using single-copy transgenes showed that PRG-1 and piRNA are also involved in an RNA-induced epigenetic silencing pathway named RNAe that permanently silences transgenes in the C. elegans germline. It was shown that RNAe (1) results in a decrease in both pre-mRNA and mRNA; (2) generates a dominant trans-acting silencing signal that can spread from a silenced to a non silenced loci; (3) correlates with the accumulation of 22G-RNAs targeting the silenced transgenes, and (4) is mediated by the NRDE/HRDE-1 machinery and H3K9me3 deposition at the targeted locus. During RNAe, piRNAs partially complementary to the transgenic sequence interact with PRG-1. The piRNA/PRG-1 complex is then thought to scan the transcriptome to initiate RdRP-dependent 22G-RNA biosynthesis within a 40nt window around the site of piRNA complementarity on the target RNA (). 22G-RNAs are then recognized by HRDE-1 and trigger NRDE-mediated H3K9me3 at the targeted locus, resulting in transcriptional silencing. Once established, H3K9me3 is transmitted to the progeny even in the absence of PRG-1. In this model, piRNAs are genomically encoded signals that are used by cellular machineries to recognize nonself from self sequences and silence them definitively by triggering the formation of heterochromatin.
C. elegans piRNA and the RNAi inheritance machinery are important actors in genome-wide surveillance of germline transcripts
In mutants lacking PRG-1, many loci that are silent in wild type germlines exhibit an increased level of mRNA expression. The absence of PRG-1 also correlates with the depletion of germline-expressed 22G-RNAs that are proximal to potential target sites that base pair imperfectly but extensively to 21U-RNAs. HRDE-1 interacts with endogenous germline 22G-RNA that are anti-sense to about 1500 coding genes.Citation8 320 predicted target genes showed a more than 2-fold depletion of H3K9me3 in both nrde-2 and nrde-4 depleted backgrounds. A significant number of these same genes are putative targets of HRDE-1-bound 22G-RNAs, and increased pre-mRNA expression was observed in a hrde-1 defective background. Finally, multigenerational monitoring of H3K9me3 at NRDE-2/4 target loci in a hrde-1 defective background showed that H3K9me3 is progressively lost at these targets over generations. Altogether, these results suggest that the RNAi inheritance machinery transmits endogenous gene regulatory information across generational boundaries. Interestingly, the SET-25 H3K9 HMTaseCitation12, SET-32, a putative H3K9 HMTase, and HPL-2, have all been shown to be required for RNAe. Considering the link between nuclear RNAi, RNAi inheritance and RNAe, it is likely that these chromatin factors may be part of the cellular machinery contributing to the establishment of RNAi-induced heterochromatin.Citation15
In hrde-1 and nrde-1/2/4 animals, loss of multigenerational epigenetic inheritance is associated with a mortal germline (Mrt) phenotype:Citation27 animals have wild type fertility in early generations following loss of HRDE/NRDE activities, but become sterile in subsequent generations. Progressive overexpression of genes that are normally silenced in the germline could be at least partially responsible for the Mrt phenotype of these animals. Altogether, these results strongly suggest that C. elegans uses the RNAi inheritance machinery to transmit epigenetic information to regulate important biological processes.
An anti-silencing pathway to protect germline expressed genes from PRG-1/HRDE/NRDE dependent silencing ?
In C. elegans, each germline-expressed gene can potentially be targeted by at least one 21U-RNA.Citation22 Based on this observation, the existence of an anti-silencing pathway to prevent 21U-RNAs mediated silencing of functional germline genes was hypothesized. CSR-1 is an Argonaute protein initially studied for its role in chromosome segregation.Citation28 CSR-1 interacts with 22G-RNAs that target endogenous germline-expressed protein-encoding mRNAs. Interestingly, mRNAs targeted by 22G-RNA associated with CSR-1 are not upregulated in the absence of CSR-1, showing that this Argonaute protein is not involved in the silencing of its target genes. This latter observation prompted Craig Mello and his collaborators to propose that CSR-1 prevents PRG-1 and 21U-RNAs from initiating silencing at germline-expressed genes. According to this model (), the perfect matching of CSR-1-bound 22G-RNAs to their target mRNA prevents the binding of partially complementary PRG-1-associated 21U-RNAs and the RdRP-dependent synthesis of 22G-RNAs required to amplify and maintain the silencing effect. Several lines of evidence support this model. For instance, it is observed that PRG-1 is required both to initiate and maintain silencing of transgenes that contain fragments of germline-expressed sequences.Citation29 By contrast, PRG-1 is only required to initiate, but not maintain silencing of transgenes that contain only somatically-expressed genes not targeted by CSR-1 22G-RNAs.Citation22 Furthermore, it has been shown that the protective effect provided by CSR-1 binding can be locally overcome by PRG-1 association with 21 U-RNAs perfectly complementary to their target sequences.Citation25
Conclusions and Perspectives
In this review, we have described recent work showing that two distinct and complementary mechanisms are successively required to establish and promote inheritance of gene expression in C. elegans. The establishment of gene silencing depends on PRG-1 and its associated endogenous small RNAs which prime the RdRP-dependent synthesis of secondary small RNAs; these in turn bind NRDE-3 in the soma and HRDE-1 in the germline to induce heterochromatin formation. RNA-induced mechanisms have been shown to be essential to transmit an epigenetic memory of non-self RNA and for the genome-wide surveillance of transcripts in the C. elegans germline.
In C. elegans, complex genetic traits including virus resistance,Citation30-Citation32 germline immortality,Citation33,Citation34 longevity,Citation35 and behavioral plasticityCitation36,Citation37 are submitted to long-term memory. Once these traits are established, they can persist for several generations even in the absence of the products required for their establishment. Other examples of transgenerational inheritance of an acquired trait have also been reported in other organisms such as drosophila, where it plays an essential role in transposon silencing,Citation38 and plants, where it is essential for flower symmetry and color.Citation39 In the future, it will be of utmost interest to establish the role of specific chromatin modifications in the inheritance of genetic traits, and how small RNA driven processes are important for the multigenerational maintenance of these modifications. Furthermore, the identification of molecular actors used by somatic RNAi for germline transmission will provide valuable insight into the complexity of heritability.
Abbreviations: | ||
RNAi | = | RNA interference |
dsRNA | = | double stranded RNA |
PTGS | = | Post transcriptional gene silencing |
siRNA | = | small interfering RNA |
AGO | = | Argonaute protein |
PAZ | = | Piwi Argonaute Zwille |
PIWI | = | P-element induced wimpy testis |
RDE | = | RNAi Deficient |
RdRP | = | RNA polymerase RNA dependent |
H3K9me3 | = | trimethylated lysine 9 of histone 3 |
NRDE | = | Nuclear RNAi Deficient |
HRDE | = | Heritable RNAi Deficient |
HMT | = | Histone Methyl Transferase |
RRF | = | RNA-dependent RNA polymerase Family |
SAGO | = | Synthetic secondary siRNA-deficient ArGOnaute mutant |
PPW | = | PAZ/PIWI domain-containing |
21U RNA | = | 21 nucleotide long RNA with a 5′ uridine |
22G RNA | = | 22 nucleotide long RNA with a 5′ guanosine |
CSR | = | Chromosome-Segregation and RNAi deficient |
RNAe | = | RNA-induced epigenetic silencing |
WAGO | = | Worms Argonaute |
SET domain | = | Su(var)3-9 |
Enhancer-of-zeste | = | Trithorax domain |
PRG | = | Piwi Related Gene |
piRNA | = | Piwi interacting RNAs |
Disclosure of Potential Conflicts of Interest
No potential conflicts of interest were disclosed.
Acknowledgments
Authors thank Anne Laurençon-Loviton and Steve Garvis for the careful reading of the manuscript. Research in the laboratory of F. Palladino is funded by the Association pour la Recherche contre le Cancer, the Ligue Contre le Cancer, the Centre Nationale de la Recherche Scientifique (CNRS) and the Agence Nationale pour la Recherche.
References
- Tijsterman M, Ketting RF, Plasterk RHA. The genetics of RNA silencing. Annu Rev Genet 2002; 36:489 - 519; http://dx.doi.org/10.1146/annurev.genet.36.043002.091619; PMID: 12429701
- Yigit E, Batista PJ, Bei Y, Pang KM, Chen C-CG, Tolia NH, Joshua-Tor L, Mitani S, Simard MJ, Mello CC. Analysis of the C. elegans Argonaute family reveals that distinct Argonautes act sequentially during RNAi. Cell 2006; 127:747 - 57; http://dx.doi.org/10.1016/j.cell.2006.09.033; PMID: 17110334
- Martienssen RA, Kloc A, Slotkin RK, Tanurdzić M. Epigenetic inheritance and reprogramming in plants and fission yeast. Cold Spring Harb Symp Quant Biol 2008; 73:265 - 71; http://dx.doi.org/10.1101/sqb.2008.73.062; PMID: 19329575
- Grishok A, Sinskey JL, Sharp PA. Transcriptional silencing of a transgene by RNAi in the soma of C. elegans. [cité 2013 sept 6 ] Genes Dev 2005; 19:683 - 96; http://dx.doi.org/10.1101/gad.1247705; PMID: 15741313
- Kim JK, Gabel HW, Kamath RS, Tewari M, Pasquinelli A, Rual J-F, Kennedy S, Dybbs M, Bertin N, Kaplan JM, et al. Functional genomic analysis of RNA interference in C. elegans. Science 2005; 308:1164 - 7; http://dx.doi.org/10.1126/science.1109267; PMID: 15790806
- Robert VJP, Sijen T, van Wolfswinkel J, Plasterk RHA. Chromatin and RNAi factors protect the C. elegans germline against repetitive sequences. Genes Dev 2005; 19:782 - 7; http://dx.doi.org/10.1101/gad.332305; PMID: 15774721
- Vastenhouw NL, Brunschwig K, Okihara KL, Müller F, Tijsterman M, Plasterk RHA. Gene expression: long-term gene silencing by RNAi. Nature 2006; 442:882; http://dx.doi.org/10.1038/442882a; PMID: 16929289
- Gu SG, Pak J, Guang S, Maniar JM, Kennedy S, Fire A. Amplification of siRNA in Caenorhabditis elegans generates a transgenerational sequence-targeted histone H3 lysine 9 methylation footprint. Nat Genet 2012; 44:157 - 64; http://dx.doi.org/10.1038/ng.1039; PMID: 22231482
- Guang S, Bochner AF, Pavelec DM, Burkhart KB, Harding S, Lachowiec J, Kennedy S. An Argonaute transports siRNAs from the cytoplasm to the nucleus. Science 2008; 321:537 - 41; http://dx.doi.org/10.1126/science.1157647; PMID: 18653886
- Burkhart KB, Guang S, Buckley BA, Wong L, Bochner AF, Kennedy S. A pre-mRNA-associating factor links endogenous siRNAs to chromatin regulation. PLoS Genet 2011; 7:e1002249; http://dx.doi.org/10.1371/journal.pgen.1002249; PMID: 21901112
- Guang S, Bochner AF, Burkhart KB, Burton N, Pavelec DM, Kennedy S. Small regulatory RNAs inhibit RNA polymerase II during the elongation phase of transcription. Nature 2010; 465:1097 - 101; http://dx.doi.org/10.1038/nature09095; PMID: 20543824
- Towbin BD, González-Aguilera C, Sack R, Gaidatzis D, Kalck V, Meister P, Askjaer P, Gasser SM. Step-wise methylation of histone H3K9 positions heterochromatin at the nuclear periphery. Cell 2012; 150:934 - 47; http://dx.doi.org/10.1016/j.cell.2012.06.051; PMID: 22939621
- Bessler JB, Andersen EC, Villeneuve AM. Differential localization and independent acquisition of the H3K9me2 and H3K9me3 chromatin modifications in the Caenorhabditis elegans adult germ line. PLoS Genet 2010; 6:e1000830; http://dx.doi.org/10.1371/journal.pgen.1000830; PMID: 20107519
- Bender LB, Cao R, Zhang Y, Strome S. The MES-2/MES-3/MES-6 complex and regulation of histone H3 methylation in C. elegans. Curr Biol 2004; 14:1639 - 43; http://dx.doi.org/10.1016/j.cub.2004.08.062; PMID: 15380065
- Ashe A, Sapetschnig A, Weick E-M, Mitchell J, Bagijn MP, Cording AC, Doebley A-L, Goldstein LD, Lehrbach NJ, Le Pen J, et al. piRNAs can trigger a multigenerational epigenetic memory in the germline of C. elegans. [cité 2012 nov 8 ] Cell 2012; 150:88 - 99; http://dx.doi.org/10.1016/j.cell.2012.06.018; PMID: 22738725
- Juang B-T, Gu C, Starnes L, Palladino F, Goga A, Kennedy S, L’Etoile ND. Endogenous nuclear RNAi mediates behavioral adaptation to odor. Cell 2013; 154:1010 - 22; http://dx.doi.org/10.1016/j.cell.2013.08.006; PMID: 23993094
- Couteau F, Guerry F, Muller F, Palladino F. A heterochromatin protein 1 homologue in Caenorhabditis elegans acts in germline and vulval development. EMBO Rep 2002; 3:235 - 41; http://dx.doi.org/10.1093/embo-reports/kvf051; PMID: 11850401
- Alcazar RM, Lin R, Fire AZ. Transmission dynamics of heritable silencing induced by double-stranded RNA in Caenorhabditis elegans. Genetics 2008; 180:1275 - 88; http://dx.doi.org/10.1534/genetics.108.089433; PMID: 18757930
- Grishok A, Tabara H, Mello CC. Genetic requirements for inheritance of RNAi in C. elegans. Science 2000; 287:2494 - 7; http://dx.doi.org/10.1126/science.287.5462.2494; PMID: 10741970
- Burton NO, Burkhart KB, Kennedy S. Nuclear RNAi maintains heritable gene silencing in Caenorhabditis elegans. Proc Natl Acad Sci U S A 2011; 108:19683 - 8; http://dx.doi.org/10.1073/pnas.1113310108; PMID: 22106253
- Batista PJ, Ruby JG, Claycomb JM, Chiang R, Fahlgren N, Kasschau KD, Chaves DA, Gu W, Vasale JJ, Duan S, et al. PRG-1 and 21U-RNAs interact to form the piRNA complex required for fertility in C. elegans. Mol Cell 2008; 31:67 - 78; http://dx.doi.org/10.1016/j.molcel.2008.06.002; PMID: 18571452
- Lee H-C, Gu W, Shirayama M, Youngman E, Conte D Jr., Mello CC. C. elegans piRNAs mediate the genome-wide surveillance of germline transcripts. [cité 2012 nov 8 ] Cell 2012; 150:78 - 87; http://dx.doi.org/10.1016/j.cell.2012.06.016; PMID: 22738724
- Olovnikov I, Aravin AA, Fejes Toth K. Small RNA in the nucleus: the RNA-chromatin ping-pong. Curr Opin Genet Dev 2012; 22:164 - 71; http://dx.doi.org/10.1016/j.gde.2012.01.002; PMID: 22349141
- Das PP, Bagijn MP, Goldstein LD, Woolford JR, Lehrbach NJ, Sapetschnig A, Buhecha HR, Gilchrist MJ, Howe KL, Stark R, et al. Piwi and piRNAs act upstream of an endogenous siRNA pathway to suppress Tc3 transposon mobility in the Caenorhabditis elegans germline. Mol Cell 2008; 31:79 - 90; http://dx.doi.org/10.1016/j.molcel.2008.06.003; PMID: 18571451
- Shirayama M, Seth M, Lee H-C, Gu W, Ishidate T, Conte D Jr., Mello CC. piRNAs initiate an epigenetic memory of nonself RNA in the C. elegans germline. [cité 2012 nov 8 ] Cell 2012; 150:65 - 77; http://dx.doi.org/10.1016/j.cell.2012.06.015; PMID: 22738726
- Luteijn MJ, van Bergeijk P, Kaaij LJT, Almeida MV, Roovers EF, Berezikov E, Ketting RF. Extremely stable Piwi-induced gene silencing in Caenorhabditis elegans. EMBO J 2012; 31:3422 - 30; http://dx.doi.org/10.1038/emboj.2012.213; PMID: 22850670
- Ahmed S, Hodgkin J. MRT-2 checkpoint protein is required for germline immortality and telomere replication in C. elegans. Nature 2000; 403:159 - 64; http://dx.doi.org/10.1038/35003120; PMID: 10646593
- Claycomb JM, Batista PJ, Pang KM, Gu W, Vasale JJ, van Wolfswinkel JC, Chaves DA, Shirayama M, Mitani S, Ketting RF, et al. The Argonaute CSR-1 and its 22G-RNA cofactors are required for holocentric chromosome segregation. Cell 2009; 139:123 - 34; http://dx.doi.org/10.1016/j.cell.2009.09.014; PMID: 19804758
- Bagijn MP, Goldstein LD, Sapetschnig A, Weick E-M, Bouasker S, Lehrbach NJ, Simard MJ, Miska EA. Function, targets, and evolution of Caenorhabditis elegans piRNAs. Science 2012; 337:574 - 8; http://dx.doi.org/10.1126/science.1220952; PMID: 22700655
- Félix M-A, Ashe A, Piffaretti J, Wu G, Nuez I, Bélicard T, Jiang Y, Zhao G, Franz CJ, Goldstein LD, et al. Natural and experimental infection of Caenorhabditis nematodes by novel viruses related to nodaviruses. [cité 2012 déc 13 ] PLoS Biol 2011; 9:e1000586; http://dx.doi.org/10.1371/journal.pbio.1000586; PMID: 21283608
- Lu R, Maduro M, Li F, Li HW, Broitman-Maduro G, Li WX, Ding SW. Animal virus replication and RNAi-mediated antiviral silencing in Caenorhabditis elegans. Nature 2005; 436:1040 - 3; http://dx.doi.org/10.1038/nature03870; PMID: 16107851
- Rechavi O, Minevich G, Hobert O. Transgenerational inheritance of an acquired small RNA-based antiviral response in C. elegans. Cell 2011; 147:1248 - 56; http://dx.doi.org/10.1016/j.cell.2011.10.042; PMID: 22119442
- Buckley BA, Burkhart KB, Gu SG, Spracklin G, Kershner A, Fritz H, Kimble J, Fire A, Kennedy S. A nuclear Argonaute promotes multigenerational epigenetic inheritance and germline immortality. Nature 2012; 489:447 - 51; http://dx.doi.org/10.1038/nature11352; PMID: 22810588
- Kelly WG, Katz DJ. The wisdom of Weismann: epigenetic erasure mechanisms and germ line immortality. Cell Cycle 2009; 8:2131 - 2; http://dx.doi.org/10.4161/cc.8.14.9083; PMID: 19556871
- Greer EL, Maures TJ, Ucar D, Hauswirth AG, Mancini E, Lim JP, Benayoun BA, Shi Y, Brunet A. Transgenerational epigenetic inheritance of longevity in Caenorhabditis elegans. Nature 2011; 479:365 - 71; http://dx.doi.org/10.1038/nature10572; PMID: 22012258
- Remy J-J. Stable inheritance of an acquired behavior in Caenorhabditis elegans. Curr Biol 2010; 20:R877 - 8; http://dx.doi.org/10.1016/j.cub.2010.08.013; PMID: 20971427
- Remy J-J, Hobert O. An interneuronal chemoreceptor required for olfactory imprinting in C. elegans. Science 2005; 309:787 - 90; http://dx.doi.org/10.1126/science.1114209; PMID: 16051801
- Grentzinger T, Armenise C, Brun C, Mugat B, Serrano V, Pelisson A, Chambeyron S. piRNA-mediated transgenerational inheritance of an acquired trait. Genome Res 2012; 22:1877 - 88; http://dx.doi.org/10.1101/gr.136614.111; PMID: 22555593
- Cubas P, Vincent C, Coen E. An epigenetic mutation responsible for natural variation in floral symmetry. Nature 1999; 401:157 - 61; http://dx.doi.org/10.1038/43657; PMID: 10490023
- Kamath RS, Fraser AG, Dong Y, Poulin G, Durbin R, Gotta M, Kanapin A, Le Bot N, Moreno S, Sohrmann M, et al. Systematic functional analysis of the Caenorhabditis elegans genome using RNAi. Nature 2003; 421:231 - 7; http://dx.doi.org/10.1038/nature01278; PMID: 12529635
- Piano F, Schetter AJ, Mangone M, Stein L, Kemphues KJ. RNAi analysis of genes expressed in the ovary of Caenorhabditis elegans. Curr Biol 2000; 10:1619 - 22; http://dx.doi.org/10.1016/S0960-9822(00)00869-1; PMID: 11137018
- Capowski EE, Martin P, Garvin C, Strome S. Identification of grandchildless loci whose products are required for normal germ-line development in the nematode Caenorhabditis elegans. Genetics 1991; 129:1061 - 72; PMID: 1783292
- Pothof J, van Haaften G, Thijssen K, Kamath RS, Fraser AG, Ahringer J, Plasterk RHA, Tijsterman M. Identification of genes that protect the C. elegans genome against mutations by genome-wide RNAi. Genes Dev 2003; 17:443 - 8; http://dx.doi.org/10.1101/gad.1060703; PMID: 12600937
- Smardon A, Spoerke JM, Stacey SC, Klein ME, Mackin N, Maine EM. EGO-1 is related to RNA-directed RNA polymerase and functions in germ-line development and RNA interference in C. elegans. Curr Biol 2000; 10:169 - 78; http://dx.doi.org/10.1016/S0960-9822(00)00323-7; PMID: 10704412
- Grishok A, Pasquinelli AE, Conte D, Li N, Parrish S, Ha I, Baillie DL, Fire A, Ruvkun G, Mello CC. Genes and mechanisms related to RNA interference regulate expression of the small temporal RNAs that control C. elegans developmental timing. Cell 2001; 106:23 - 34; http://dx.doi.org/10.1016/S0092-8674(01)00431-7; PMID: 11461699