Abstract
There is ample evidence that exposure to stress during gestation increases the risk of the offspring to develop mood disorders. Brain-derived neurotrophic factor (Bdnf) plays a critical role during neuronal development and is therefore a prime candidate to modulate neuronal signaling in adult offspring of rat dams that were stressed during gestation. In the current study, we tested the hypothesis that alterations in Bdnf expression in prenatally stressed (PNS) offspring are mediated by changes in DNA methylation in exons IV and VI of the Bdnf gene. We observed decreased Bdnf expression in the amygdala and hippocampus of prenatally stressed rats both at weaning and in adulthood. This decrease in Bdnf expression was accompanied by increased DNA methylation in Bdnf exon IV in the amygdala and hippocampus, suggesting that PNS-induced reduction in Bdnf expression may, at least in part, be mediated by increased DNA methylation of Bdnf exon IV. Expression of DNA methyltransferases (Dnmt) 1 and 3a was increased in PNS rats in the amygdala and hippocampus. Our data suggest that PNS induces decreases in Bdnf expression that may at least in part be mediated by increased DNA methylation of Bdnf exon IV.
Introduction
The prenatal environment has been suggested to play an important role in the development of mood- and stress-related disorders. In both humans and animals models, maternal stress exposure has been shown to affect the offspring in adulthood.Citation1 In humans, offspring from mothers that experienced severe stress during their pregnancy are more likely to develop neurodevelopmental and psychiatric problems, such as anxiety disorders, depression, attention deficit/hyperactivity disorder, and delayed language acquisition.Citation2 Additionally, exposure to maternal stress in rodents has been shown to induce a depressive-like phenotype in the adult offspring.Citation3 Rodent prenatal stress (PNS) models also indicate a higher vulnerability of offspring for drug addictionCitation4,Citation5 and anxiety.Citation6 During the perinatal period, the brain is highly plastic and is thus highly sensitive to stressors. The prenatal environment is hypothesized to influence the behavioral phenotype of the adult individual by altering expression of genes during the development of the brain and throughout the lifespan. These differences in gene expression, in turn, are hypothesized to be brought about by alterations in the epigenome, either through changes in DNA methylation or by histone modifications. Brain-derived neurotrophic factor (Bdnf) plays important roles in neuronal differentiation, proliferation, and synaptic plasticity.Citation7-Citation9 Given its importance in neuronal development, Bdnf is a prime candidate to modulate neuronal signaling in adult offspring of stressed dams. Bdnf has indeed been shown to be influenced by the early life environment. In the hippocampus, prefrontal cortex, and amygdala of mice, increased or decreased maternal care leads to increased or decreased Bdnf protein levels, respectively.Citation10-Citation12 Additionally, PNS has been shown to alter Bdnf expression levels in the rat hippocampus.Citation13,Citation14 In the current study, we investigated the effect of PNS on Bdnf expression at PND (postnatal day) 21 and PND80 in male rats. We chose the PND21 time point to target the peak expression levels of Bdnf during development.Citation15 More specifically, PND21 is the time point at which Bdnf exon IV expression peaks and is hypothesized to contribute most to Bdnf expression and protein levels.Citation15 To assess whether PNS induced alterations in Bdnf expression and DNA methylation carried through into adulthood brain samples of 80 d old rats were assessed. Studying the effect of PNS in both sexes is important and Bdnf expression has been shown to fluctuate across the estrous cycleCitation16 and estrogen and progesterone have been associated with those changes.Citation16 Therefore, when studying females, adequate controls for the stage of estrus and the different stages of development are required. For these reasons, we focus on male offspring in this study. Data obtained from the current studies will be used to design more targeted follow up studies that can be aimed at sex differences.
Previous studies showed that PNS predominantly affects the expression of Bdnf exon IV and exon VI.Citation17 Therefore, we tested the hypothesis that alterations in Bdnf expression in prenatally stressed offspring are mediated by variable DNA methylation of Bdnf exon IV and Bdnf exon VI. Because PNS seems to enhance vulnerability for anxiety- and depressive-like phenotypes, we not only analyzed Bdnf expression and DNA methylation in the hippocampus, but also in other brain areas involved in anxiety and depression including the amygdala and the prefrontal cortex. Within Bdnf, we measured the effects of PNS on DNA methylation in a region of exon IV that encompasses the transcription start site as well as a cyclic adenosine monophosphate (cAMP) response element. This region was chosen because this particular region of the exon may play an important role in neural activity-dependent Bdnf gene expression.Citation18-Citation20 Within Bdnf exon VI, we focused analysis on DNA methylation on a region of the exon that encompasses a glucocorticoid response element,Citation21 which may be particularly targeted by prenatal stress exposure. Furthermore, the chosen regions of Bdnf exon IV and VI have been previously studied for DNA methylation and DNA methylation was shown to be altered by early life stress in these regions.Citation14 Levenson and colleagues showed that alteration in Bdnf DNA methylation patterns in the hippocampus may be mediated by DNA (cytosine-5)-methyltransferases (Dnmt).Citation22 We therefore measured expression levels of Dnmt1, Dnmt3a, and Dnmt3b in control and PNS rat brains to evaluate the role these enzymes play in the methylation of Bdnf.
Results
Dam and pup characteristics
No differences in body weight were observed between stressed and control dams. There were no differences in litter size or weight. PNS and control pup weight did not significantly differ at any time point during the lactation period (). There were no differences in plasma corticosterone levels between PNS and control pups at sacrifice.
Table 2. Litter composition, and body weights of dams and pups
Expression of Bdnf at PND21
displays the relative expression of Bdnf in various brain regions in PNS and control offspring. PNS resulted in significantly decreased Bdnf expression in the amygdala, and hippocampus at PND 21 (AMY: t[22] = 3.88 P < 0.001; HPC: t[22] = 5.20 P < 0.001). In the prefrontal cortex, no between-groups difference in Bdnf expression was observed.
Figure 1.Bdnf mRNA expression in control and PNS rats. Bdnf expression is expressed as the normalized relative expression to the control proactive chow fed group. A: PND21 offspring, B: PND80 offspring. Control, open bars; PNS, hatched bars; AMY, amygdala; HPC, hippocampus; PFC, prefrontal cortex; *significant difference (P < 0.01).
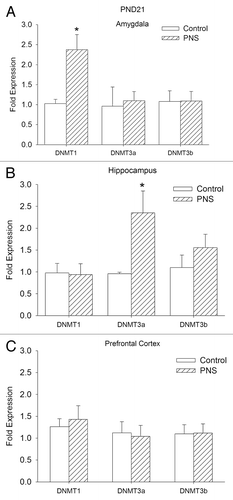
Expression of Bdnf at PND80
PNS resulted in significantly decreased Bdnf expression in the amygdala and hippocampus at PND 80 (AMY: t[22] = 3.15 P < 0.01; HPC: t[22] = 2.94 P < 0.05). In the prefrontal cortex no between-groups difference in Bdnf expression was observed.
DNA methylation of Bdnf exon IV promoter at PND21
The percentage of DNA methylation of the Bdnf exon IV region is presented in . Overall Bdnf exon IV DNA methylation at PND21 was significantly increased in the amygdala and hippocampus of PNS rats (AMY: t[22] = -6.74 P < 0.001; HPC: t[22] = -2.49, P < 0.05). More specifically, in the amygdala, a significant increase in DNA methylation was observed at the following individual CpGs: TTS-35: F[1,18] = 7.3063; TSS-23: F[1,18] = 19.8996 P < 0.05; TSS+19: F[1,18] = 32.7296; TSS+35: F[1,18] = 25.7465, P < 0.05, TSS+37: F[1,18] = 37.4617, P < 0.05, TSS+62: F[1,18] = 17.0308; TSS+65: F[1,18] = 6.50770, P < 0.05; TSS+124: F[1,18] = 46.3022, P < 0.01. In the hippocampus, significant differences were seen in TSS-35, TSS+35, and TSS+37 (TSS-35: F[1,18] = 5.9276, P < 0.05; TSS+35: F[1,18] = 19.4608; TSS+37: F[1,18] = 8.900, P < 0.01). In the prefrontal cortex no overall difference in DNA methylation of Bdnf exon IV was observed at PND21 though DNA methylation was significantly increased in the prefrontal cortex of PNS rats at TSS+35 (F[1,18] = 6.8428 P < 0.05).
Figure 2. DNA methylation of Bdnf exon IV promoter. The CpGs analyzed are presented relative to the transcription site (TSS) of Bdnf exon IV promoter. (A‒C) PND21 offspring, (D‒F): PND80 offspring. Control, open bars; PNS, hatched bars; *significant difference (P < 0.01); CRE, cyclic adenosine monophospate (cAMP) response element.
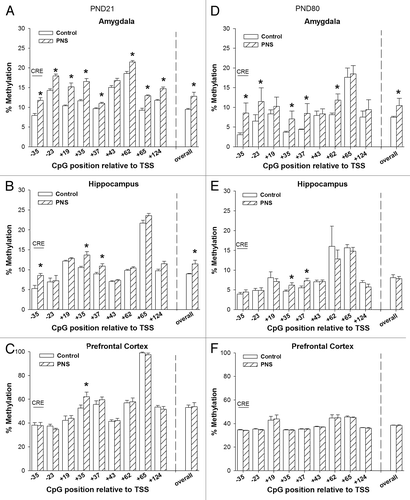
DNA methylation Bdnf exon IV promoter PND80
Overall, Bdnf exon IV DNA methylation at PND80 was significantly increased in the amygdala PNS rats (AMY: t[22] = 2.32 P < 0.05). More specifically, in the amygdala, a significant increase in DNA methylation in PNS rats was observed at the following individual CpGs (TTS-35: F[1,18] = 60.06 P < 0.001; TSS-23: F[1,18] = 13.82 P < 0.01; TSS+35: F[1,18] = 5.38, P < 0.05, TSS+37: F[1,18] = 4.84, P < 0.05, TSS+62: F[1,18] = 13.53 P < 0.01). Overall, Bdnf exon IV DNA methylation in the hippocampus and prefrontal cortex was not different for control and PNS offspring at PND80. In the hippocampus, there were, however, some CpG-specific increases in DNA methylation in PNS offspring at TSS+35, and TSS+37 (TSS+35: F[1,18] = 4.92, P < 0.05 ; TSS+37: F[1,18] = 10.18, P < 0.01).
DNA methylation of Bdnf exon VI promoter PND 21
displays the percentage of DNA methylation of the Bdnf exon VI region. Overall methylation of Bdnf exon VI was not different in control and PNS offspring in any of the tested brain areas at PND21. There were, however, some CpG-specific differences. In the amygdala, PNS led to increase in methylation of TSS+265 and decrease in methylation of TSS+195 and TSS+227 (TSS+195: F[1,18] = 11.75 P < 0.01, TSS+227: F[1,18] = 7.81 P < 0.05, TSS+265: F[1,18] = 16.38 P < 0.01). TSS+242, and TSS+273, TSS+294, and TSS+309 increased in methylation in the hippocampus of PNS offspring (TSS+242: F[1,18] = 7.35 P < 0.05, TSS+273: F[1,18] = 5.84 P < 0.05, TSS+294: F[1,18] = 10.97 P < 0.01, TSS+309: F[1,18]= 5.00 P < 0.05). In the prefrontal cortex TSS+195, TSS+227 and TSS+265 increased in methylation in PNS offspring (TSS+195: F[1,18] = 10.53 P < 0.01, TSS+227: F[1,18] = 8.78 P < 0.01, TSS+265: F[1,18] = 23.11 P < 0.01).
Figure 3. DNA methylation of Bdnf exon VI promoter. The CpGs analyzed are presented relative to the transcription site (TSS) of Bdnf exon VI promoter. (A‒C) PND21 offspring, (D‒F) PND80 offspring. Control, open bars; PNS, hatched bars; *significant difference (P < 0.01); GRE, Glucocorticoid response element.
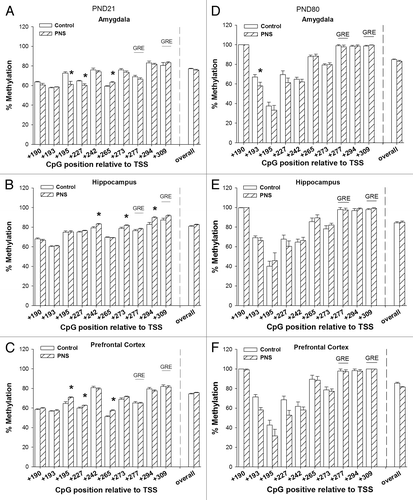
DNA methylation Bdnf exon VI promoter PND80
Overall methylation of Bdnf exon VI was not different in control and PNS offspring in any of the tested brain areas at PND80. Some CpG specific differences were observed in the amygdala and the prefrontal cortex. In the amygdala, PNS led to decrease in methylation of TSS+193 (TSS+193: F[1,18] = 17.71 P < 0.01). In the prefrontal cortex, a decrease in methylation in PNS offspring was observed at TSS+193 (TSS+193: F[1,18] = 30.71 P < 0.001).
Expression of Dnmts PND21
We asked whether there was a change in any of the DNA methylation enzymes that can account for the increased DNA methylation observed in the amygdala and the hippocampus in PNS rats at PND21. Relative expression of Dnmt1, Dnmt3a, and Dnmt3b is shown in . Dnmt1 expression is elevated in the amygdala of PNS rats, but there were no changes in expression of Dnmt3a or Dnmt3b (Dnmt1 AMY: t [22] = 3.18; P < 0.01). Dnmt3a expression was greater in hippocampus of PNS rats (Dnmt3a HPC: t [22] = 2.76; P < 0.05). No differences between control and PNS offspring were observed in Dnmt expression in the prefrontal cortex.
Discussion
Our study shows that variable PNS leads to decreased Bdnf gene expression in the amygdala and hippocampus of rats at both PND21 and PND80. We further found that DNA methylation of the Bdnf exon IV promoter is also altered in amygdala and hippocampus, suggesting that this may be a mechanism for changes in gene expression. Dnmt1 gene expression was higher in the amygdala of PNS offspring and Dnmt3a gene expression was higher in the hippocampus of PNS offspring. These increases in Dnmt expression could represent the initiating step for PNS-induced methylation changes in the brain.
Our findings support previously reported studies describing decreased Bdnf expression in the hippocampus as a result of PNS exposure.Citation23 In contrast, Neeley and colleagues showed increased hippocampal Bdnf expression after PNS in adult offspring. They also reported that PNS altered exon VI expression, without altering expression of exon IV.Citation17 In their study, PNS increased Bdnf exon VI expression under baseline conditions, and decreased expression after acute stress. We did not test the expression of specific exons, so it is possible that increased expression of exon VI does occur in our model, but that this does not alter overall expression of the Bdnf gene. The differences in BDNF expression between baseline and stress conditions described by Neeley and colleaguesCitation17 suggest that, at least in the adult rat hypothalamus, the conditions under which brains are collected are a crucial factor in the levels of BDNF expression.
Even though our data suggest that DNA methylation levels are similarly affected by PNS at PND21 and PND80, it is not unlikely that there are differential effects of PNS at different time points. As was shown by van den Hove et al., PNS resulted in decreased Bdnf mRNA levels at postnatal day (PND) 15, but not at earlier time points.Citation23 Bdnf expression has an inverted U-shape developmental pattern with peak levels between PND14 through PND21.Citation24 Expression of Bdnf exon IV increases strongly in the hippocampus in the first 14 d of life, and stabilizes from PND14 through PND21 then decreases throughout adulthood.Citation15 To our knowledge, developmental expression patterns of exon VI in rats have not been studied. However, most of the Bdnf exons studied follow a similar pattern, with peak levels during the first two weeks of life.Citation15 PNS may affect the developmental time-course of Bdnf expression leading to differential effects of PNS at different ages. These effects may be brain region- and exon-specific. Detailed investigation of Bdnf mRNA and protein levels throughout development in control and prenatally stressed rats is necessary to determine this. Nevertheless, our data suggest that alterations in Bdnf expression and Bdnf exon IV DNA methylation induced by prenatal stress exposure carry though from PND21 to PND80.
Our data suggest that differences in Bdnf gene expression in PNS rats may be a result of differential DNA methylation, at least in the amygdala and the hippocampus. In this study, PNS resulted in increased DNA methylation of Bdnf exon IV in the amygdala and hippocampus, which may be causally related to the observed decrease in Bdnf expression in these same brain regions. DNA methylation was not altered in the exon VI region. It is debatable if an increase in methylation of Bdnf exon IV alone would be sufficient to induce the observed decrease in Bdnf expression. Previous research has suggested that increases in expression of the coding Bdnf exon, exon IX, at this time point were predominantly mediated by increases in exon IV expression,Citation15 suggesting that difference in methylation of exon IV may indeed influence overall Bdnf expression. However, those studies did not measure the contribution of exons III, VI, VII, and VIII on overall Bdnf expression, leaving this hypothesis speculative. Nevertheless, studies in adult animals have shown that alterations in exon IV expression, without changes in expression of the other non-coding exons, are sufficient to generate changes is overall Bdnf expression.Citation25,Citation26 Changes in exon IV-specific Bdnf expression has been shown to be correlated with the degree of methylation of the corresponding exon IV-associated CpGs in rats exposed to associative fear conditioning.Citation27 Although our experimental set-up did not allow for direct correlative analysis of DNA methylation and gene expression with the individual pups, using pups from the same litter resulted in statistically significant correlations between DNA methylation of Bdnf exon IV and mRNA expression of Bdnf in the amygdala and hippocampus (R = -0.790, R2 = 0.625, P < 0.01). This suggests that the observed differences in DNA methylation in Bdnf exon IV may indeed have influenced expression of Bdnf exon IV. However, future experiments are needed to investigate the causal relationships between DNA methylation and mRNA expression of the Bdnf gene in the amygdala and hippocampus.
The observed alterations in DNA methylation and mRNA expression of Bdnf may contribute to the PNS phenotype as Bdnf plays a major role in neuronal development and previous studies have shown that prenatal stress affects neuronal development during this specific early postnatal period. For example, at PND25 the amygdala of offspring of rats exposed to PNS were shown to have a decreased number of neurons.Citation28 Additionally, decreases in neurogenesis and dendrite complexity after prenatal stress have been reported in the hippocampus during the early postnatal period.Citation29 While the direct role of epigenetic processes in PNS-induced changes in Bdnf expression has not been investigated in this study, our data do suggest that PNS may induce changes in DNA methylation of Bdnf exon IV, which may influence the expression of Bdnf throughout the rats’ development.
PNS decreased Bdnf expression specifically in the hippocampus and amygdala. Studies on the role of Bdnf in the amygdala are mostly correlative; however, they suggest that Bdnf in the amygdala plays a major role in anxiety-like behavior. Mice that are deficient in TrkB, the Bdnf receptor, show morphological alterations in the amygdala and increased anxiety-like behavior.Citation30 Additionally, Bdnf was shown to be critical for fluoxetine induced fear extinction.Citation31 Finally, in human carriers of the Bdnf Val66Met polymorphism, which is associated with depression and anxiety, show reductions in amygdala volume.Citation32 Future experiments are needed to further elucidate a causal role for amygdala Bdnf in depression. The role of Bdnf in the hippocampus in depression-like behavior is clearer. A study using overexpression and knockdown of Bdnf in rats showed that Bdnf overexpression could rescue depression-like behavior in chronically stressed rats, whereas knockdown of Bdnf in the hippocampus, at least in young animals, produced a depression-like phenotype.Citation33,Citation34
One of the limitations of the current study is that we were not able to analyze expression and DNA methylation for the specific subnuclei of the hippocampus and amygdala. There are functional differences among the subnuclei that may be reflected by differences in DNA methylation. Additionally, in our study we did not differentiate between neurons and glia cells. Since Bdnf is expressed in both cell types, understanding whether PNS induced alterations in DNA methylation occur in either neurons or glia or in both of these cell types would increase our knowledge about the potential functional impact of the alterations. Existing methodology does not allow for such fine analysis in a cost effective manner. Further studies using advanced brain microdissection and cell sorting techniques are required for a more refined analysis.
The increased methylation of Bdnf exon IV may be mediated by differential expression of Dnmts, as we found higher expression of Dnmt1 in the amygdala and of Dnmt3a in the hippocampus of PNS offspring. Evidence from in vitro studies in hippocampal slices suggests that Dnmts influence methylation patterns of the Bdnf promoter region,Citation22 which further strengthens the hypothesis that increased methylation in the Bdnf gene of PNS offspring may be induced by these enzymes. The observed brain region specific differences in the expression patterns of Dnmts may be indicative of the functional properties of these enzymes. Dnmt1 has been shown to selectively induce methylation of hemimethylated DNA, thereby ensuring the inheritance of appropriate methylation patterns.Citation35-Citation37 Dnmt3a, on the other hand, has been associated with de novo methylation.Citation38 Dnmt3a is hypothesized to be responsible for inducing methylation postnatally, and expression levels of Dnmt3a in the hippocampus have been reported to increase during the first 3 wk of life and decline thereafter.Citation39 Hippocampal developmental delays due to PNSCitation40 may delay the peak expression of Dnmt3a, explaining a difference of Dnmt3a expression at PND21 in the hippocampus. Dnmt1 expression in the hippocampus was shown to be relatively stable during this developmental period and may thus be less affected.Citation41 Dnmt1 expression in the amygdala is high during the first week of development and decreases thereafter.Citation41 Expression of Dnmt3a in the amygdala has not been extensively studied, but Dnmt3a expression in amygdala is thought to decrease from PND1 onward.Citation42 To our knowledge, the effects of PNS on neurodevelopmental patterns in the amygdala have not been studied. If, similar to the hippocampus, neurodevelopmental delays do occur in the amygdala in response to PNS, this may explain elevated Dnmt1 expression on PND21. In vitro studies using inhibition of Dnmt by zebularine lead to demethylation of a CpG island of the Bdnf promoter.Citation22 This suggests that alterations of Dnmt levels may affect the methylation of the Bdnf gene. However, to date, it is not known whether inhibition or activation of a specific Dnmt may affect DNA methylation at the level of a single CpG within the Bdnf gene. Nevertheless, increases in Dnmt levels are not the only mechanism through which changes in DNA methylation could be induced. For example, enzymes involved in DNA demethylation like ten-11 translocation proteins (TET) and the base excision repair proteins (BER) could influence DNA methylation levels independent of Dnmt (reviewed in ref. Citation43). Furthermore, alterations in histone modifications such as methylation of histone H3 lysine 9 (H3K9) and lysine 27 (H3K27) may act as signals to recruit DNA methylation, possibly through G9a, a methyltransferase.Citation44 Lastly, DNA binding factors like FOXA1, REST, and CTCFCitation45,Citation46 may alter DNA methylation at promoters and enhancers in the surrounding DNA. Therefore, research is needed to investigate the underlying mechanisms that lead to changes in DNA methylation at Bdnf exon IV in our study. Overall, the presented data suggest that Dnmts may have contributed to some of the observed alterations in DNA methylation after PNS. However, this is not likely the only mechanism underlying alterations in DNA methylation in response to PNS exposure. The extent to which altered expression of Dnmt in response to PNS leads to direct changes in DNA methylation of specific genes, such as Bdnf, merits further investigation.
Decreased expression of Bdnf at weaning may have several consequences for the adult animal. Bdnf increases synaptic plasticity and increases dendritic arborization.Citation47 This might be important in the depression-like phenotype of the adult PNS rat since during the early adolescent time period the connectivity between the amygdala and the prefrontal cortex matures. Disruption of amygdala-prefrontal cortex connectivity has been associated with depression and problems in emotionality.Citation48 Furthermore, the differences in Bdnf methylation and Dnmt expression suggest that differences in Bdnf expression might be present throughout neuronal development from G14 through PND21. Changes in Bdnf expression during the last week of gestation and the early postnatal period could alter neuronal development of several brain areas that are involved in mood disorders.
A question that remains to be answered is whether these alterations in Bdnf methylation and subsequent expression may also account for the anxiety- and depressive-like phenotypes observed in adult PNS rats. Further research using targeting increased DNA methylation of Bdnf exon IV during the early post-natal period are needed to elucidate the causal pathways. It is plausible that exposure to PNS may alter Bdnf developmental patterns and peak levels of Bdnf, leading to the differential Bdnf expression observed in this study. The data presented here indicate that PNS decreases Bdnf expression in the amygdala and hippocampus and both DNA methylation analysis and Dnmt expression analysis suggest that this decrease in Bdnf expression may be mediated by increased DNA methylation of, in particular, Bdnf exon IV.
Materials and Methods
Animals
Twenty-four timed-pregnant Sprague Dawley rats were received on gestational day (G) two (Charles River). The male offspring of these dams were used in the experiments. The dams were individually housed in standard tub cages withad libitum access to water and a standard chow diet (Harlan 2018). The room was maintained on a 12:12 light/dark cycle (Light onset: 6 AM). The offspring remained with the dams until PND21. Hereafter, the rats were group housed with 2 to 3 same sex litter mates until sacrifice. All animal protocols were approved by the Animal Care and Use Committee of the Johns Hopkins University School of Medicine.
Experimental set-up
Half of the dams (n = 12) were exposed to a variable stress paradigm. The offspring of the dams exposed to variable stress will be referred to as prenatally stressed (PNS), offspring of the control dams will be referred to as controls. Body weight and food intake of the dams were measured daily during the gestational period. The day a litter was found was designated PND0. On PND1, litters were culled to 10 pups each (5 male and 5 female). Only litters that contained > 10 pups were included. Pups and dams were weighed once weekly beginning on PND1. Two male pups per litter were sacrificed on PND21, and two male pups per litter were sacrificed on PND80. Their brains were used for expression and methylation analysis (1 pup per litter for mRNA expression, 1 pup per litter for DNA methylation).
Prenatal stress
Beginning on G14, 12 dams were subjected to an 8-d schedule of variable stress. Each stressor was applied during the light cycle unless noted otherwise (). We selected a variable stress paradigm for our studies to prevent the animals from habituating to the stress, as has been documented using predictable stressors such as repeated restraint stress.Citation49 Stress was restricted to the 3rd week of gestation because brain regions that regulate the HPA axis and mood, including the hypothalamus, amygdala, and hippocampus, are rapidly developing during this period.Citation50 The remaining control dams (n = 12) were exposed to normal animal room husbandry practices in the animal facility.
Table 1. Schematic overview of stressors applied during Gestation
Tissue collection
Rats were killed by decapitation at PND21 or PND80 between 10–11am. Brains were removed, frozen immediately on powdered dry ice, and stored at −80 °C. The, amygdala (AMY), prefrontal cortex (PFC), and hippocampus (HPC) were isolated from 500 μm thick frozen coronal sections using a blunted 16-gauge stainless steel needle (ID 1.65 mm) based on the coordinates for PND21 rat brainsCitation51 or for adult rat brains.Citation52 Trunk blood was collected at sacrifice and plasma corticosterone levels were determined by a commercially available radioimmunoassay kit for corticosterone (MP Biomedicals). Inter- and intra-assay variability for the assay was as follows: 6.5–7.1% and 4.4 –10.3%.
Gene expression
Total RNA from the tissue punches was obtained using the RNeasy Lipid Tissue Mini Kit (Qiagen) with the Qiazol reagent that allows for an efficient separation of RNA from contaminating proteins. QuantiTect Reverse Transcription Kit (Qiagen) was used to generate cDNA for subsequent quantitative real-time PCR. Negative RT samples were used to control for possible contamination of gDNA. All reactions were performed in triplicate using 1× Taqman master mix (Applied Biosystems), 1× Taqman probes for each gene (Bdnf, Dnmt1, Dnmt3a, Dnmt3b, and Beta-actin), and 2 μg of cDNA template in a total volume of 20 μL. Real-time reactions were performed on an Applied Biosystems 7900HT Fast Real-Time PCR System with standard PCR conditions (50 °C for 2 min; 95 °C for 10 min; and 95 °C for 15 s and 60 °C for 1 min for 40 cycles). Each set of triplicates was checked to ensure that the threshold cycle (Ct) values were all within 1 Ct of each other. To determine relative expression values, the -ΔΔCt method (Applied Biosystems) was used, where triplicate Ct values for each tissue sample were averaged and subtracted from those derived from the housekeeping gene β-actin. The average Ct difference for the control group (control proactive chow fed rats) was subtracted from those of the test samples, and the resulting -ΔΔCt values were raised to a power of two to determine normalized relative expression.
Bisulfite pyrosequencing Genomic DNA (gDNA) from rat brain tissue was isolated with the Masterpure DNA Purification Kit (Epicenter Biotechnologies) according to manufacturer’s protocol. The concentration of the gDNA was determined using a NanoDrop 1000 Spectrophotometer (Thermo Scientific). Five-hundred ng of the DNA was used for bisulfite conversion according to the manufacturer's protocol (EZ DNA Methylation-Gold Kit; Zymo Research). Twenty-five nanograms of bisulfite-treated DNA was used for each PCR reaction. An additional nested PCR was performed with 2 μL of the previous PCR reaction and one biotinylated primer (other primer being unmodified). Amplification for both PCR steps consisted of 40 cycles (95 °C for 1 min, 53 °C for 30 s, 72 °C for 1 min). PCR products were further purified using gel extraction. PCR products were separated on agarose gels and the bands corresponding to the sizes of region of interest (Bdnf exon IV; 271 bp, Bdnf exon VI; 162 bp) was cut out of the gel. PCR products were subsequently extracted from the gel fragments using Qiaquick gel extraction kit (Qiagen).
The design of the primers was based on the regions previously studied by Lubin et.al.Citation27 To assess DNA methylation of the Bdnf exon IV promoter region, two sets of primers were designed to amplify a 271-bp region of rat Bdnf exon IV:
outside forward 5′- AGGTAGAGGA GGTATTATAT GATAGT-3′
outside reverse 5′- ACTATATATT TCCCCTTCTC TTCAATT-3′
nested forward 5′- TTTTTTTGTT AGTGTGATAT ATTT-3′
nested reverse 5′- CACCTTTTTC AATCACTACT TATCA -3′
To assess DNA methylation of the Bdnf exon VI promoter region, two sets of primers were designed to ultimately amplify a 162-bp region of rat Bdnf exon VI:
outside forward 5′- GAAGTTTAAT YGAAGAGTTA AATAATG -3′
outside reverse 5′- ACTTCTCAAT CCCCTAACCA ACTTTC -3′
nested forward 5′- GGATTTTTYG GTTTGGAGAA GGAAAT -3′
nested reverse 5′- CCTACCTCAA AATCCACACA AAACTCTC -3′
Pyro Gold reagents were used to prepare samples for pyrosequencing according to manufacturer's instructions. For each sample, biotinylated PCR product was mixed with streptavidin coated sepharose beads (GE Healthcare), binding buffer, and Milli-Q water, and shaken at room temperature. A vacuum prep tool was used to isolate the sepharose bead-bound single-stranded PCR products. The attached DNAs were released into a PSQ HS 96-well plate containing pyrosequencing primers in annealing buffer. Pyrosequencing reactions were performed by PyroMark MD System (Qiagen). CpG methylation quantification was performed with Pyro Q-CpGt 1.0.9 software (Qiagen). An internal quality-control step was employed to disqualify any assays that contained unconverted DNA. Percentage of methylation at each CpG as determined by pyrosequencing was compared between DNA from control and PNS offspring. The positions of the CpGs analyzed for each exon relative to the transcription start site (TSS) are summarized in .
Table 3. Positions of analyzed CpGs relative to the transcription site
Statistical analysis
Data are expressed as averages with standard error of the mean. mRNA expression data are normalized against β-actin and are presented as the fold expression relative to the control proactive chow fed rats. Statistical differences were assessed using Statistica 7 (Stat Soft, Inc.). Differences in mRNA expression were statistically tested, for each brain area separately, using an unpaired T-test with PNS as between-subjects factors. Overall DNA methylation of a gene was calculated as the average % DNA methylation of all CpGs within the gene. To control for potential crossover effects between adjacent CpGs within a gene, statistical differences in DNA methylation for each CpG were tested using a multivariate ANOVA with PNS as between-subjects factor, followed by Bonferroni post hoc analysis. In all statistical tests, P < 0.05 was considered significant.
Disclosure of Potential Conflicts of Interest
No potential conflicts of interest were disclosed.
Financial Disclosures
The authors have no conflicts of interest to declare.
Acknowledgments
We are grateful to Bo Sun, Jennifer Albertz, and Nu-Chu Liang for their technical assistance. We would like to thank Timothy Moran for his valuable feedback to the manuscript. These studies were supported by NIH HD055030, NARSAD Young Investigator Award, and NWO Rubicon Post-Doctoral Fellowship (825.10.032) grants.
References
- Weinstock M. The potential influence of maternal stress hormones on development and mental health of the offspring. Brain Behav Immun 2005; 19:296 - 308; http://dx.doi.org/10.1016/j.bbi.2004.09.006; PMID: 15944068
- Talge NM, Neal C, Glover V, Early Stress, Translational Research and Prevention Science Network: Fetal and Neonatal Experience on Child and Adolescent Mental Health. Antenatal maternal stress and long-term effects on child neurodevelopment: how and why?. J Child Psychol Psychiatry 2007; 48:245 - 61; http://dx.doi.org/10.1111/j.1469-7610.2006.01714.x; PMID: 17355398
- Darnaudéry M, Dutriez I, Viltart O, Morley-Fletcher S, Maccari S. Stress during gestation induces lasting effects on emotional reactivity of the dam rat. Behav Brain Res 2004; 153:211 - 6; http://dx.doi.org/10.1016/j.bbr.2003.12.001; PMID: 15219722
- Deminière JM, Piazza PV, Guegan G, Abrous N, Maccari S, Le Moal M, Simon H. Increased locomotor response to novelty and propensity to intravenous amphetamine self-administration in adult offspring of stressed mothers. Brain Res 1992; 586:135 - 9; http://dx.doi.org/10.1016/0006-8993(92)91383-P; PMID: 1511342
- Kippin TE, Szumlinski KK, Kapasova Z, Rezner B, See RE. Prenatal stress enhances responsiveness to cocaine. Neuropsychopharmacology 2008; 33:769 - 82; http://dx.doi.org/10.1038/sj.npp.1301447; PMID: 17487224
- Vallée M, Mayo W, Dellu F, Le Moal M, Simon H, Maccari S. Prenatal stress induces high anxiety and postnatal handling induces low anxiety in adult offspring: correlation with stress-induced corticosterone secretion. J Neurosci 1997; 17:2626 - 36; PMID: 9065522
- Alderson RF, Alterman AL, Barde YA, Lindsay RM. Brain-derived neurotrophic factor increases survival and differentiated functions of rat septal cholinergic neurons in culture. Neuron 1990; 5:297 - 306; http://dx.doi.org/10.1016/0896-6273(90)90166-D; PMID: 2169269
- Hofer MM, Barde YA. Brain-derived neurotrophic factor prevents neuronal death in vivo. Nature 1988; 331:261 - 2; http://dx.doi.org/10.1038/331261a0; PMID: 3336438
- Kalcheim C, Gendreau M. Brain-derived neurotrophic factor stimulates survival and neuronal differentiation in cultured avian neural crest. Brain Res 1988; 469:79 - 86; http://dx.doi.org/10.1016/0165-3806(88)90171-X; PMID: 3401810
- Branchi I. The mouse communal nest: investigating the epigenetic influences of the early social environment on brain and behavior development. Neurosci Biobehav Rev 2009; 33:551 - 9; http://dx.doi.org/10.1016/j.neubiorev.2008.03.011; PMID: 18471879
- Chatterjee D, Chatterjee-Chakraborty M, Rees S, Cauchi J, de Medeiros CB, Fleming AS. Maternal isolation alters the expression of neural proteins during development: ‘Stroking’ stimulation reverses these effects. Brain Res 2007; 1158:11 - 27; http://dx.doi.org/10.1016/j.brainres.2007.04.069; PMID: 17555725
- Nair A, Vadodaria KC, Banerjee SB, Benekareddy M, Dias BG, Duman RS, Vaidya VA. Stressor-specific regulation of distinct brain-derived neurotrophic factor transcripts and cyclic AMP response element-binding protein expression in the postnatal and adult rat hippocampus. Neuropsychopharmacology 2007; 32:1504 - 19; http://dx.doi.org/10.1038/sj.npp.1301276; PMID: 17164818
- Neeley EW, Berger R, Koenig JI, Leonard S. Strain dependent effects of prenatal stress on gene expression in the rat hippocampus. Physiol Behav 2011; 104:334 - 9; http://dx.doi.org/10.1016/j.physbeh.2011.02.032; PMID: 21382392
- Roth TL, Lubin FD, Funk AJ, Sweatt JD. Lasting epigenetic influence of early-life adversity on the BDNF gene. Biol Psychiatry 2009; 65:760 - 9; http://dx.doi.org/10.1016/j.biopsych.2008.11.028; PMID: 19150054
- Timmusk T, Metsis M. Regulation of BDNF promoters in the rat hippocampus. Neurochem Int 1994; 25:11 - 5; http://dx.doi.org/10.1016/0197-0186(94)90046-9; PMID: 7950963
- Gibbs RB. Levels of trkA and BDNF mRNA, but not NGF mRNA, fluctuate across the estrous cycle and increase in response to acute hormone replacement. Brain Res 1998; 787:259 - 68; http://dx.doi.org/10.1016/S0006-8993(97)01511-4; PMID: 9518642
- Neeley EW, Berger R, Koenig JI, Leonard S. Prenatal stress differentially alters brain-derived neurotrophic factor expression and signaling across rat strains. Neuroscience 2011; 187:24 - 35; http://dx.doi.org/10.1016/j.neuroscience.2011.03.065; PMID: 21497180
- Aid T, Kazantseva A, Piirsoo M, Palm K, Timmusk T. Mouse and rat BDNF gene structure and expression revisited. J Neurosci Res 2007; 85:525 - 35; http://dx.doi.org/10.1002/jnr.21139; PMID: 17149751
- Bredy TW, Wu H, Crego C, Zellhoefer J, Sun YE, Barad M. Histone modifications around individual BDNF gene promoters in prefrontal cortex are associated with extinction of conditioned fear. Learn Mem 2007; 14:268 - 76; http://dx.doi.org/10.1101/lm.500907; PMID: 17522015
- Martinowich K, Hattori D, Wu H, Fouse S, He F, Hu Y, Fan G, Sun YE. DNA methylation-related chromatin remodeling in activity-dependent BDNF gene regulation. Science 2003; 302:890 - 3; http://dx.doi.org/10.1126/science.1090842; PMID: 14593184
- Suri D, Vaidya VA. Glucocorticoid regulation of brain-derived neurotrophic factor: relevance to hippocampal structural and functional plasticity. Neuroscience 2013; 239:196 - 213; http://dx.doi.org/10.1016/j.neuroscience.2012.08.065; PMID: 22967840
- Levenson JM, Roth TL, Lubin FD, Miller CA, Huang IC, Desai P, Malone LM, Sweatt JD. Evidence that DNA (cytosine-5) methyltransferase regulates synaptic plasticity in the hippocampus. J Biol Chem 2006; 281:15763 - 73; http://dx.doi.org/10.1074/jbc.M511767200; PMID: 16606618
- Van den Hove DL, Steinbusch HW, Scheepens A, Van de Berg WD, Kooiman LA, Boosten BJ, Prickaerts J, Blanco CE. Prenatal stress and neonatal rat brain development. Neuroscience 2006; 137:145 - 55; http://dx.doi.org/10.1016/j.neuroscience.2005.08.060; PMID: 16242847
- Das KP, Chao SL, White LD, Haines WT, Harry GJ, Tilson HA, Barone S Jr.. Differential patterns of nerve growth factor, brain-derived neurotrophic factor and neurotrophin-3 mRNA and protein levels in developing regions of rat brain. Neuroscience 2001; 103:739 - 61; http://dx.doi.org/10.1016/S0306-4522(01)00011-2; PMID: 11274792
- Lubin FD. Epigenetic gene regulation in the adult mammalian brain: multiple roles in memory formation. Neurobiol Learn Mem 2011; 96:68 - 78; http://dx.doi.org/10.1016/j.nlm.2011.03.001; PMID: 21419233
- Yasuda S, Liang MH, Marinova Z, Yahyavi A, Chuang DM. The mood stabilizers lithium and valproate selectively activate the promoter IV of brain-derived neurotrophic factor in neurons. Mol Psychiatry 2009; 14:51 - 9; http://dx.doi.org/10.1038/sj.mp.4002099; PMID: 17925795
- Lubin FD, Roth TL, Sweatt JD. Epigenetic regulation of BDNF gene transcription in the consolidation of fear memory. J Neurosci 2008; 28:10576 - 86; http://dx.doi.org/10.1523/JNEUROSCI.1786-08.2008; PMID: 18923034
- Kraszpulski M, Dickerson PA, Salm AK. Prenatal stress affects the developmental trajectory of the rat amygdala. Stress 2006; 9:85 - 95; http://dx.doi.org/10.1080/10253890600798109; PMID: 16895832
- Kawamura T, Chen J, Takahashi T, Ichitani Y, Nakahara D. Prenatal stress suppresses cell proliferation in the early developing brain. Neuroreport 2006; 17:1515 - 8; http://dx.doi.org/10.1097/01.wnr.0000236849.53682.6d; PMID: 16957600
- Carim-Todd L, Bath KG, Fulgenzi G, Yanpallewar S, Jing D, Barrick CA, Becker J, Buckley H, Dorsey SG, Lee FS, et al. Endogenous truncated TrkB.T1 receptor regulates neuronal complexity and TrkB kinase receptor function in vivo. J Neurosci 2009; 29:678 - 85; http://dx.doi.org/10.1523/JNEUROSCI.5060-08.2009; PMID: 19158294
- Karpova NN, Pickenhagen A, Lindholm J, Tiraboschi E, Kulesskaya N, Agústsdóttir A, Antila H, Popova D, Akamine Y, Bahi A, et al. Fear erasure in mice requires synergy between antidepressant drugs and extinction training. Science 2011; 334:1731 - 4; http://dx.doi.org/10.1126/science.1214592; PMID: 22194582
- Montag C, Weber B, Fliessbach K, Elger C, Reuter M. The BDNF Val66Met polymorphism impacts parahippocampal and amygdala volume in healthy humans: incremental support for a genetic risk factor for depression. Psychol Med 2009; 39:1831 - 9; http://dx.doi.org/10.1017/S0033291709005509; PMID: 19335934
- Taliaz D, Loya A, Gersner R, Haramati S, Chen A, Zangen A. Resilience to chronic stress is mediated by hippocampal brain-derived neurotrophic factor. J Neurosci 2011; 31:4475 - 83; http://dx.doi.org/10.1523/JNEUROSCI.5725-10.2011; PMID: 21430148
- Taliaz D, Stall N, Dar DE, Zangen A. Knockdown of brain-derived neurotrophic factor in specific brain sites precipitates behaviors associated with depression and reduces neurogenesis. Mol Psychiatry 2010; 15:80 - 92; http://dx.doi.org/10.1038/mp.2009.67; PMID: 19621014
- Bestor TH. Activation of mammalian DNA methyltransferase by cleavage of a Zn binding regulatory domain. EMBO J 1992; 11:2611 - 7; PMID: 1628623
- Kimura H, Shiota K. Methyl-CpG-binding protein, MeCP2, is a target molecule for maintenance DNA methyltransferase, Dnmt1. J Biol Chem 2003; 278:4806 - 12; http://dx.doi.org/10.1074/jbc.M209923200; PMID: 12473678
- Okano M, Xie S, Li E. Dnmt2 is not required for de novo and maintenance methylation of viral DNA in embryonic stem cells. Nucleic Acids Res 1998; 26:2536 - 40; http://dx.doi.org/10.1093/nar/26.11.2536; PMID: 9592134
- Okano M, Bell DW, Haber DA, Li E. DNA methyltransferases Dnmt3a and Dnmt3b are essential for de novo methylation and mammalian development. Cell 1999; 99:247 - 57; http://dx.doi.org/10.1016/S0092-8674(00)81656-6; PMID: 10555141
- Feng J, Chang H, Li E, Fan G. Dynamic expression of de novo DNA methyltransferases Dnmt3a and Dnmt3b in the central nervous system. J Neurosci Res 2005; 79:734 - 46; http://dx.doi.org/10.1002/jnr.20404; PMID: 15672446
- Mychasiuk R, Gibb R, Kolb B. Prenatal stress alters dendritic morphology and synaptic connectivity in the prefrontal cortex and hippocampus of developing offspring. Synapse 2012; 66:308 - 14; http://dx.doi.org/10.1002/syn.21512; PMID: 22121047
- Simmons RK, Howard JL, Simpson DN, Akil H, Clinton SM. DNA methylation in the developing hippocampus and amygdala of anxiety-prone versus risk-taking rats. Dev Neurosci 2012; 34:58 - 67; http://dx.doi.org/10.1159/000336641; PMID: 22572572
- Kolodkin MH, Auger AP. Sex difference in the expression of DNA methyltransferase 3a in the rat amygdala during development. J Neuroendocrinol 2011; 23:577 - 83; http://dx.doi.org/10.1111/j.1365-2826.2011.02147.x; PMID: 21518035
- Auclair G, Weber M. Mechanisms of DNA methylation and demethylation in mammals. Biochimie 2012; 94:2202 - 11; http://dx.doi.org/10.1016/j.biochi.2012.05.016; PMID: 22634371
- Dong KB, Maksakova IA, Mohn F, Leung D, Appanah R, Lee S, Yang HW, Lam LL, Mager DL, Schübeler D, et al. DNA methylation in ES cells requires the lysine methyltransferase G9a but not its catalytic activity. EMBO J 2008; 27:2691 - 701; http://dx.doi.org/10.1038/emboj.2008.193; PMID: 18818693
- Sérandour AA, Avner S, Percevault F, Demay F, Bizot M, Lucchetti-Miganeh C, Barloy-Hubler F, Brown M, Lupien M, Métivier R, et al. Epigenetic switch involved in activation of pioneer factor FOXA1-dependent enhancers. Genome Res 2011; 21:555 - 65; http://dx.doi.org/10.1101/gr.111534.110; PMID: 21233399
- Stadler MB, Murr R, Burger L, Ivanek R, Lienert F, Schöler A, van Nimwegen E, Wirbelauer C, Oakeley EJ, Gaidatzis D, et al. DNA-binding factors shape the mouse methylome at distal regulatory regions. Nature 2011; 480:490 - 5; PMID: 22170606
- Vigers AJ, Amin DS, Talley-Farnham T, Gorski JA, Xu B, Jones KR. Sustained expression of brain-derived neurotrophic factor is required for maintenance of dendritic spines and normal behavior. Neuroscience 2012; 212:1 - 18; http://dx.doi.org/10.1016/j.neuroscience.2012.03.031; PMID: 22542678
- Cunningham MG, Bhattacharyya S, Benes FM. Amygdalo-cortical sprouting continues into early adulthood: implications for the development of normal and abnormal function during adolescence. J Comp Neurol 2002; 453:116 - 30; http://dx.doi.org/10.1002/cne.10376; PMID: 12373778
- Barnum CJ, Blandino P Jr., Deak T. Adaptation in the corticosterone and hyperthermic responses to stress following repeated stressor exposure. J Neuroendocrinol 2007; 19:632 - 42; http://dx.doi.org/10.1111/j.1365-2826.2007.01571.x; PMID: 17620105
- Weinstock M. Gender differences in the effects of prenatal stress on brain development and behaviour. Neurochem Res 2007; 32:1730 - 40; http://dx.doi.org/10.1007/s11064-007-9339-4; PMID: 17406975
- Sherwood N, Timiras P. A stereotaxic atlas of the developing rat brain. Berkeley, Los Angeles, London, University of California Press 1970.
- Paxinos GW. C. The Rat Brain in Stereotaxic Coordinates. Sydney: Academic Press, 1982.