Abstract
“Fetal programming” is a term used to describe how early-life experience influences fetal development and later disease risk. In humans, prenatal stress-induced fetal programming is associated with increased risk of preterm birth, and a heightened risk of metabolic and neurological diseases later in life. A critical determinant of this is the regulation of fetal exposure to glucocorticoids by the placenta. Glucocorticoids are the mediators through which maternal stress influences fetal development. Excessive fetal glucocorticoid exposure during pregnancy results in low birth weight and abnormalities in a number of tissues. The amount of fetal exposure to maternal glucocorticoids depends on the expression of HSD11B2, an enzyme predominantly produced by the syncytiotrophoblast in the placenta. This protects the fetus by converting active glucocorticoids into inactive forms. In this review we examine recent findings regarding placental HSD11B2 that suggest that its epigenetic regulation may mechanistically link maternal stress and long-term health consequences in affected offspring.
Introduction
“Fetal programming” is a term used to describe how the early-life experience influences fetal development and later disease risk.Citation1 The glucocorticoid hypothesis describes how increased fetal exposure to maternal glucocorticoids, such as cortisol, as a result of prenatal stress during pregnancy is associated with a number of poor birth outcomes and increased risk for later-in-life neurological and cardiometabolic syndromes in exposed offspring.Citation1,Citation2 Recent clinical and epidemiological studies have shown that maternal prenatal stress is associated with increased risk for preterm birth, reduced birth weight,Citation3 smaller head circumferenceCitation4 and later-in-life cardiometabolicCitation5 and neuropsychiatric disorders,Citation6 such as obesity,Citation5,Citation7 type 1 diabetes,Citation8 schizophrenia,Citation6 autism, ADHD,Citation9 and impaired cognitive and language development.Citation10
As early as the 11–12th week of pregnancy, profound changes in the activity of the maternal hypothalamic-pituitary axis (HPA) occur that lead to increased production of maternal cortisol. The levels of cortisol continue to rise during pregnancy to reach almost three times those of non-pregnant women by the third trimester.Citation11-Citation13 The HPA axis is regulated by the release of corticotrophin-releasing hormone (CRH) from the hypothalamus, which stimulates the release of adrenocorticotrophic hormone (ACTH) from the anterior pituitary gland, which in turn stimulates the production of cortisol from the adrenal glands. Normally, cortisol regulates its own secretion through a negative feedback loop that inhibits the production of CRH and ACTH.Citation14 However, during pregnancy, the placenta begins to release CRH into the maternal bloodstream and levels reach 1000–10 000 times that of a non-pregnant woman. This further increases the production of cortisol by the adrenals.Citation15,Citation16 Although cortisol usually negatively regulates the secretion of hypothalamic CRH, a rise in maternal cortisol actively stimulates the release of placental CRH, which further raises the levels of maternal cortisol progressively during pregnancy.Citation11-Citation13 This increase in maternal glucocorticoid levels has been proposed to be required for normal fetal organogenesisCitation17 and, in keeping with this suggestion, antenatal synthetic glucocorticoid administration to preterm infants has been shown to significantly decrease mortality and morbidity in infants born before 34 wk of gestation by accelerating lung maturation.Citation18-Citation20 However, excessive fetal exposure to maternal glucocorticoids has been proposed to mediate the impact of prenatal stress on birth outcomes, resulting in long-term health consequences in affected infants.Citation21 Furthermore, elevated maternal cortisol levels during pregnancy is associated with a larger amygdala and more emotional disorders in girls aged 7, directly linking maternal cortisol to long-term programming effects.Citation22 However, no study to date has examined if maternal stress is directly associated with elevated fetal cortisol levels during pregnancy or at term.
Normally, the fetus is protected from the high levels of maternal cortisol by molecular mechanisms in the placenta that convert active cortisol to its inactive metabolite cortisone. Consequently, fetal cortisol levels have been shown to be ten to 13 times lower than those found in maternal circulation.Citation23,Citation24 The primary barrier between maternal and fetal circulation is provided by two epithelial layers that cover the chorionic villi of the human placenta.Citation25 The first of these epithelial layers is the syncytiotrophoblast, a large multi-nucleated terminally-differentiated syncytium (many nuclei in the same cytoplasmic mass), that is formed by differentiation and fusion of cells derived from the second epithelial layer, the underlying cytotrophoblast.Citation26 Within these trophoblast layers, an enzyme called 11β-hydroxysteroid dehydrogenase type-2 (HSD11B2) is highly expressed. HSD11B2 catalyzes the conversion of active cortisol into inactive cortisone, thus protecting the fetus from excessive cortisol exposure ().Citation27-Citation29
Figure 1. The placental HSD11B2 shield. (A) During pregnancy, the placenta acts as a critical regulator that limits fetal exposure to maternal glucorticoids. (B) Cross sectional image of the placenta. Blood travels from the fetus through the umbilical arteries (blue). Blood is carried to the chorionic villi, where exchange of nutrients and waste products with the mother occurs. The chorionic villi are made up of two epithelial layers, the syncytiotrophoblast and the cytotrophoblast. (C) Longitudinal section of the chorionic villi. HSD211B2 is expressed strongly in the syncytiotrophoblast and to a lesser extent in the cytotrophoblast, where it converts cortisol into inactive cortisone, thereby protecting the fetus from excessive cortisol exposure.
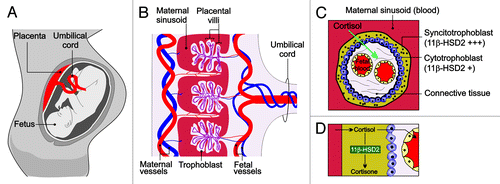
In this review we describe the impact of prenatal stress during pregnancy on placental HSD11B2 expression and its relationship to fetal health outcomes. We examine the available evidence that suggests that the programming effects of prenatal stress may be mediated through the regulation of placental HSD11B2 expression. We summarize recent data indicating that epigenetic regulation of placental HSD11B2 expression and/or function may be a mechanistic link between maternal stress and the resultant long-term health consequences for affected offspring. Finally, we offer a clinical perspective, highlighting the difficulties and promises of targeting placental HSD11B2 epigenetic change.
Effect of Prenatal Stress on HSD11B2 Expression
Increasing preclinical and clinical evidence suggest that maternal stress during pregnancy can lead to alterations in placental HSD11B2 expression. For example, direct cortisol infusion into pregnant mice between embryonic day (E)12 and E15 resulted in the upregulation of HSD11B2 mRNA and protein levels in the placenta at E14.5. However, by E17, placental HSD11B2 mRNA was significantly reduced, indicating an adaptive mechanism whereby acute cortisol exposure initially upregulates the placental barrier to protect the fetus, but chronic cortisol exposure reduces its expression, resulting in overexposure of the fetus to maternal glucocorticoids.Citation30 In agreement with these findings, it has also been shown that pregnant rats subjected to acute restraint stress for one hour on gestational day 20, show increased placental HSD11B2 expression.Citation31 In contrast, chronic restraint stress in pregnant rat dams from gestational day 14 to 20 caused a reduction of HSD11B2 protein and mRNA levels in the placenta.Citation31,Citation32 This was accompanied by reduced fetal birth weight, reduced circulating ACTH levels and lower adrenal weight.Citation32 Similarly, pregnant rat dams that were food restricted from gestational day 10 to 20, which causes an increase in maternal corticosterone levels, showed reduced placental HSD11B2 mRNA expression and lower fetal birth weight.Citation33 Furthermore, these offspring displayed reduced circulating ACTH, adrenal atrophy and decreased mineralocorticoid and glucocorticoid mRNA expression in the fetal hippocampus, indicating alterations in the fetal HPA axis.Citation34 Similar findings have been reported in human pregnancy. Amniocentesis in pregnant women, which is associated with increased anxiety levels and is a form of acute stress in humans, is associated with increased HSD11B2 activity in the placenta.Citation35 Prenatal anxiety and depression in humans, as determined by the Spielberger State Trait Anxiety Test and The Edinburgh postnatal depression test, is a form of chronic prenatal stress in humans. Consistent with preclinical data, chronic prenatal anxiety and depression were found to be associated with reduced activity and expression of placental HSD11B2.Citation36
The precise mechanism(s) by which prenatal stress reduces placental expression of HSD11B2 is unclear, but it has been shown that increased circulating glucocorticoid concentrations, as a result of stress, stimulate the sympathoadrenal system, causing elevated adrenaline and noradrenaline release from the adrenal medulla.Citation37 These stress-induced catecholamines have shown to decrease HSD11B2 mRNA in the BeWo human choriocarcinoma cell line and in primary trophoblastic cells.Citation38 Furthermore, women experiencing high levels of stress during pregnancy also show increased circulating levels of pro-inflammatory cytokines.Citation39 Pro-inflammatory cytokines TNF-α and IL-1β have been shown to decrease HSD11B2 mRNA levels and activity in vitro.Citation40-Citation42 These studies indicate that there are multiple mechanisms through which prenatal stress may affect placental HSD11B2 expression and/or activity. Collectively, these data highlight an important point: acute stress during pregnancy may not be harmful to the developing fetus due to the ability to upregulate the expression of HSD11B2; however, chronic stress can result in a significant decrease in placental HSD11B2 expression, causing the fetus to be exposed to excessive levels of maternal cortisol. Although the precise mechanisms mediating these changes in placental HSD11B2 expression remain to be fully elucidated, it has recently emerged that epigenetic regulation may play a crucial role.
Epigenetic Regulation of HSD11B2 Expression
It is now largely accepted that epigenetics can be modified by environmental exposures, particularly during development, when the epigenome undergoes profound changes.Citation43 Recently, it was suggested that the link between prenatal stress and the reduction in placental HSD11B2 expression may involve hypermethylation of the HSD11B2 promoter, leading to a corresponding reduction in HSD11B2 expression.
The human HSD11B2 gene contains four CpG islands, two located in the promoter and exon 1 and two in exon 5 and the downstream region.Citation44,Citation45 Hypermethylation of normally unmethylated CpG islands is indicative of transcriptional repression of the relevant gene.Citation46 Interestingly, hypermethylation of HSD11B2 CpG islands correlates with lower levels of HSD11B2 expression.Citation45 Low methylation levels of HSD11B2 were observed in the placenta (were HSD11B2 expression is high), whereas in skeletal muscle (low HSD11B2 expression) the HSD11B2 promoter was hypermethylated.Citation45 Furthermore, it has been shown that the activity of the HSD11B2 promoter is directly regulated by methylation.Citation45 These data show that exposures that change HSD11B2 methylation status in the placenta can result in transcriptional repression of the gene, causing the fetus to be exposed to higher levels of cortisol that may adversely affect fetal development. In agreement with this idea, Marsit and colleagues determined the methylation status of HSD11B2 in 186 placentae and examined any correlation with fetal birth and neurodevelopmental milestones. The methylation status of 4 CpG islands in placental HSD11B2 was examined, showing a statistically significant, albeit moderate, negative correlation with placental HSD11B2 mRNA expression, indicating that changes in the methylation status of placental HSD11B2 can regulate its expression.Citation44 Intriguingly, a negative correlation was found between infant birth weight and ponderal index (ratio of weight for length) and HSD11B2 methylation, demonstrating that smaller and leaner/thinner infants showed increased levels of HSD11B2 methylation.Citation44 Furthermore, when the extent of HSD11B2 methylation was examined in intrauterine growth restricted (IUGR) infants compared with non-IUGR infants, IUGR infants showed a significantly greater extent of HSD11B2 methylation than non-IUGR infants.Citation44 They also examined infant neurobehavioral outcomes and found that the extent of HSD11B2 methylation was greatest in infants with reduced quality of movement scores as assessed using the NICU Network Neurobehavioral Scales (NNNS) examination.Citation44 This study is important as it is the first one to link growth, epigenetic alterations of placental genes, and early-life neurobehavioral outcomes in humans. Clearly, longer follow-up of infants is required to determine if methylation status of placental HSD11B2 can help identify those children who may develop neurobehavioral or learning difficulties later in life.Citation44 It is possible that the epigenetic status of placental HSD11B2 could also be a useful predictor of other adverse outcomes.
New data using a rat model of prenatal anxiety has now demonstrated that prenatal maternal anxiety caused a reduction of HSD11B2 placental expression and, importantly, that this was associated with increased CpG methylation of placental HSD11B2.Citation47 This study also attempted to assess whether methylation at certain CpG sites within placental HSD11B2 positively predicted the methylation status of HSD11B2 in the fetal cortex and hippocampus. This raises the intriguing possibility of using the epigenetic status of placenta to predict corresponding changes in the fetal brain.Citation47 Prenatal maternal anxiety was reported to result in a small but statistically significant increase in HSD11B2 methylation at CpG sites 4, 7 and 8, and reduced expression of placental HSD11B2. When methylation at these same sites was examined in the fetal hypothalamus, significant changes in methylation were observed, but these were discordant and in the opposite direction as observed in the placenta, apparently causing no effect on HSD11B2 mRNA levels.Citation47 However, it should be noted that significant differences in methylation at other CpG sites in the placenta were also observed that were not found in the fetal brain, suggesting that there may not be a complete direct, inverse relationship between both tissues. Furthermore, no differences in DNA methylation were observed in the fetal cortex of stressed animals compared with controls,Citation47 suggesting that regional differences in the effects of prenatal maternal anxiety on the methylation status of HSD11B2 in the fetal brain may exist. This raises the intriguing possibility that the epigenetic status of HSD11B2 in the placenta could predict changes in the fetal brain. However, it is clear that much work remains to be done to investigate causality, and that the effects of prenatal maternal anxiety on the entire epigenome, rather than individual genes, need to be examined. Such studies are vital, given the recent demonstration that global epigenomic reconfiguration is observed during human brain development, which suggests a key role of DNA methylation in brain development and function.Citation46
Recently, the impact of maternal prenatal anxiety on the placental epigenetic status of HSD11B2 has been reported.Citation48 Conradt and colleagues used a structured chart review to collect information about whether a mother reported depression and/or anxiety during pregnancy. Of the 482 participants recruited for the study, 13.7% reported depression or anxiety during pregnancy, which is consistent with the estimated prevalence rates.Citation49 In agreement with findings from animal models of prenatal anxiety,Citation47 maternal anxiety during pregnancy resulted in a statistically significant increase in methylation of CpG site 4 of the HSD11B2 promoter.Citation48 Furthermore, infants of mothers who were anxious during pregnancy were more hypotonic, and placentae from these pregnancies had greater methylation of CpG site 4 in HSD11B2.Citation48 While correlations suggest that the epigenetic status of placental HSD11B2 may help identify infants at risk for poor neurodevelopmental outcomes, a key challenge that remains is to prove cause and effect. Specifically, whether the increase in HSD11B2 methylation in the placenta following prenatal anxiety in fact leads to the behavioral changes observed in affected infants needs to be further examined. One possible approach could be to examine fetal neurobehavioral outcomes following prenatal anxiety in mice that express a drug-inducible, conditional placental-specific knockdown of the methyltransferase DNMT3a, whose expression has been shown to be increased in the placenta following prenatal anxiety;Citation47 or, more specifically, the generation of mice carrying methylation resistant CpG sites in HSD11B2.
A Clinical Perspective: Extrinsic Factors That Can Modify Placental HSD11B2 Expression
If epigenetic downregulation of placental HSD11B2 expression is the mechanistic link between the experience of maternal stress and the resultant long-term health consequences for affected offspring, then strategies that increase placental HSD11B2 expression have the potential of improving fetal outcome. Here we highlight two ways in which extrinsic factors could modify the expression of placental HSD11B2: (1) the use of pharmacological epigenetic modifiers and; (2) dietary considerations that may affect placental HSD11B2.
Effects of Pharmacological Epigenetic Modifiers on HSD11B2 Expression
A defining feature of epigenetic modifications is that they are reversible. Thus, drugs that interfere with the enzymes that produce these changes may help maintain or restore normal gene expression levels. One class of drugs that alter DNA methylation is that of DNMT inhibitors. Many types of DNMT inhibitors have been produced and some of these have been used clinically for the treatment of leukemias, myelodysplastic syndromes and hemglobinopathies.Citation50 These compounds exert their effects by covalently trapping methyltransferases, thereby preventing full methylation of a DNA strand.Citation51,Citation52 A second class of molecules is that of HDAC inhibitors. These compounds inhibit the activity of HDACs, thus preventing histone deacetylation of lysine residues on histone tails and, as a consequence, enhancing gene transcription. HDAC inhibitors have also been used in epigenetic therapy and two of these compounds have recently gained FDA approval as anti-cancer therapies.Citation53
DNMT inhibitors 5-aza-2′-deoxycytidine (5-AZA) and procainamide have been shown to demethylate the HSD11B2 promoter, enhancing both HSD11B2 expression and activity in the SW620 human colon carcinoma cell line, MCF-7 breast adenocarcinoma cell line, and JEG-3 placental carcinoma cell line.Citation45 Furthermore, when given orally to rats for seven days, DNMT inhibitors were shown to reduce HSD11B2 methylation and to increase HSD11B2 expression,Citation45 which highlights the potential impact of such classes of epigenetic pharmacological modifiers on HSD11B2 expression. Similar studies have also examined the effects of HDAC inhibition on HSD11B2 expression and found that the HDAC inhibitor trichostatin A had no effect by itself on these cell lines, but increased HSD211B2 expression in SW620 and MCF-7 cells when added only 48 h after 5-AZA treatment, indicating that DNA methylation is the dominant form of epigenetic modification on this gene.Citation45
These classes of pharmacological inhibitors have been proposed to be potentially useful in reversing placental epigenetic change. However, a major challenge with these approaches will be to specifically target the placenta without affecting the developing fetus. This is particularly pertinent as recent data shows that a global reconfiguration of the fetal neural epigenome occurs during development,Citation46 suggesting that drugs that interfere with these processes may have profound consequences for normal fetal development. Furthermore, these drugs have significant detrimental effects on both the mother and fetus. For example, intraperitoneal (ip) injection of pregnant mice with 5-AZA on gestational day 10 has been shown to reduce birth weight in affected offspring, an effect that persists in males at 5 months of age.Citation54 This reduced weight is also associated with reduced IGF-1 levels in the serum of these offspring.Citation54 Furthermore, males and females who were exposed to 5-AZA in utero display a 70% reduction and a 30% reduction in mating capacity and fertility, respectively, as measured by the presence of a vaginal plug and viable pregnancy.Citation54 In a recent study by Ding and colleagues, Kunming mice were subjected to i.p. injection of 5-AZA or saline on gestational days 1, 2 and 4, followed by embryo implantation on day 4. Mice that received 5-AZA showed a significant reduction in embryo implantation, as compared with the saline group. This was associated with a cell specific reduction in mRNA levels and an increase in the methylation levels of many genes involved in endometrial change, as well as a decrease in stromal cell proliferation and differentiation.Citation55 Furthermore, intravenous infusion of 5-AZA in patients with metastatic lung carcinomas induced hematopoiesis toxicity,Citation56 thus limiting their use in pregnant women. Given these significant problems and challenges associated with their use, the administration of pharmacological epigenetic modifiers to modify the placental epigenome is not feasible at the present time.
Effects of Dietary Composition on Placental HSD11B2 Expression
The influence of dietary composition as a modifier of placental epigenetic machinery is becoming increasingly apparent.Citation57,Citation58 Mice fed a high fat diet showed reduced placental expression of a number of methyltransferases involved in epigenetic modification.Citation57,Citation58 This is a very important finding when one considers that, in western societies, 50% of all pregnant women are either overweight (BMI of 25–29.9 kg/m2) or obese (BMI of ≥30 kg/m2),Citation59 have a dietary caloric excess predominantly derived from fat,Citation60 or show a higher rate of adverse pregnancy outcomes.Citation61,Citation62
DNA methylationCitation45 is dependent on the availability of methyl group donors and cofactors provided by the methionine and folate metabolisms, as well as dietary-derived vitamin B6 and B12, suggesting that dietary modifications can affect the fetal and placental epigenome.Citation63 This is highlighted by a 12-wk dose-response choline feeding study conducted in third-trimester pregnant women that investigated the effect of a maternal choline intake of 930 mg or 480 mg per day. Higher maternal choline intake significantly increased the average methylation levels in the glucocorticoid receptor gene promoter in placenta and fetal leukocytes, with infants showing lower levels of circulating cortisol in the higher choline group.Citation64 Although limited data exist regarding the impact of dietary composition on the epigenetic modification of placental HSD11B2, Takaya et al. examined the methylation of individual CpG dinucleotides in the promoter region of hepatic HSD11B2 in offspring of pregnant rats fed a magnesium-deficient diet from 2 wk prior to mating and throughout the gestational period.Citation65 The average methylation of the HSD11B2 promoter in the offspring of dams fed a magnesium deficient diet was significantly higher than that from controls.Citation65 However, it is important to note that contrary to what was expected given previous findings in the placenta,Citation44 HSD11B2 methylation was positively correlated with HSD11B2 mRNA expression.Citation65 However, responsiveness to environmental exposures in utero cannot be generalized across genes and tissues,Citation66 suggesting that epigenetic modification of the HSD11B2 promoter in different tissues may result in different effects on gene expression. Together, these studies show that maternal diet can exert gene-specific epigenetic changes that can effect placental mRNA expression. The effect of diet on placental epigenetic regulation of HSD11B2 is a key question for future research. However, a number of studies have examined the effect of both dietary intake and composition on placental HSD11B2.
A recent study investigating the impact of maternal caloric restriction on placental HSD11B2 expression reported that when dietary intake was reduced by 50% in Sprague Dawley rats from gestational day 10 to 20, these rats showed higher maternal corticosterone levels than controls and a reduction in HSD11B2 expression in the labyrinth zone of the placenta.Citation33,Citation67 Furthermore, specific reduction in protein content of the maternal diet during early and mid, but not late, gestation in Wistar rats caused a reduction of placental HSD11B2 expression.Citation68 These findings have been supported by some,Citation69-Citation71 though not all,Citation72 studies and collectively suggest that adequate protein intake is required to maintain optimal expression of placental HSD11B2. However, an excess of calories, predominantly derived from high fat and high sugar foods largely define Western dietary habits.Citation60 Therefore, an area that demands further investigation is the effect of such high calories, high fat/sugar diets on placental HSD11B2 expression. A recent study by Sferruzzi-Perri et al. reported altered placental phenotypes in mice fed a high fat, high sugar diet that affected placental nutrient handling, which has implications for fetal development and pregnancy outcomes.Citation73 In addition, the high fat, high sugar diet influenced fetal growth trajectory (initial growth restriction followed by catch-up growth), which may have implications for later-life outcomes. However, one potential caveat of this well-designed study (acknowledged by its authors) was the difference in protein content between the two diets (the standard diet consisted of 26% protein compared with the high fat, high sugar diet, which consisted of 17% protein).Citation73 It has previously been shown that even mild protein restriction can contribute to changes in placental expression of HSD211B2 and development of HPA dysfunction and hypertension.Citation60 Therefore, the data from Sferruzzi-Perri and colleagues need to be interpreted within the context of unmatched maternal protein intakes. However, given that mice and rats fed a high fat diet during pregnancy display alterations in the placental transcriptome and have dysregulated placental epigenetic machinery,Citation57,Citation58 a much greater understanding of the effects of diet on placental HSD11B2 is required. When we analyzed the supplementary data available in the study by Mao et al. looking at the placental transcriptome at day 12.5 of gestation in mice fed a high fat diet, robust changes were found in the placental transcriptome however HSD11B2 was not in their list of differentially expressed genes.Citation74 This does not mean that this gene does not change in response to a high fat diet, it only means that changes in HSD11B2 were not detected at the particular time at which they did their microarray profiling. In addition, transient changes with long-lasting effects cannot be ruled out, especially given the data from Sferruzzi-Perri and colleagues.Citation73
Future research should focus on addressing the effects of such diets, as well as on understanding the correct balance of nutrients that may mitigate the effects of increased maternal stress. For instance, could optimal protein levels throughout pregnancy protect against the placental morphological and phenotypical changes associated with a high fat, high sugar diet? In addition, we know that a reduction in total maternal calories downregulates HSD11B2 in the labyrinth zone and preliminary evidence suggests that a high fat, high sugar diet influences labyrinth zone development; therefore, devising maternal dietary interventions that may influence both placental development and ensure optimal fetal outcomes may be an exciting area of research. Similarly, could maternal dietary manipulation represent a mechanism that could protect or counteract the effect of maternal stress (environmental or emotional) on HSD11B2 expression and fetal outcomes? These questions warrant further investigation.
Whether nutritional interventions can specifically prevent or reverse prenatal stress-induced epigenetic change in placental HSD11B2 remains to be elucidated, but it represents an exciting and, more importantly, feasible approach to potentially reverse or prevent the programming effect of prenatal stress on the placental and fetal epigenome.
Abbreviations: | ||
ACTH | = | adrenocorticotrophic hormone |
5-AZA | = | 5-aza-2′-deoxycytidine |
CRH | = | Corticotrophin-releasing hormone |
DMNT | = | DNA methyltransferase |
E | = | embryonic day |
HAT | = | histone acetyltransferase |
HDAC | = | histone deacetylases |
HSD11B2 | = | 11β-hydroxysteroid dehydrogenase type-2 |
HPA | = | hypothalamic-pituitary axis |
IUGR | = | intrauterine growth restricted |
NNNS examination | = | NICU Network Neurobehavioral Scales examination |
Disclosure of Potential Conflicts of Interest
No potential conflicts of interest were disclosed.
Acknowledgments
The authors acknowledge grant support in the form of a Research Frontiers Program grant (Grant#: 10/RFP/NES2786) (G.O.’K.) and a Research Centres grant (Grant#: INFANT-12/RC/2272) (L.K.) from Science Foundation Ireland.
References
- Entringer S, Buss C, Wadhwa PD. Prenatal stress, telomere biology, and fetal programming of health and disease risk. Sci Signal 2012; 5:pt12; http://dx.doi.org/10.1126/scisignal.2003580; PMID: 23112344
- Reynolds RM. Glucocorticoid excess and the developmental origins of disease: two decades of testing the hypothesis--2012 Curt Richter Award Winner. Psychoneuroendocrinology 2013; 38:1 - 11; http://dx.doi.org/10.1016/j.psyneuen.2012.08.012; PMID: 22998948
- Hobel CJ, Goldstein A, Barrett ES. Psychosocial stress and pregnancy outcome. Clin Obstet Gynecol 2008; 51:333 - 48; http://dx.doi.org/10.1097/GRF.0b013e31816f2709; PMID: 18463464
- Dancause KN, Laplante DP, Oremus C, Fraser S, Brunet A, King S. Disaster-related prenatal maternal stress influences birth outcomes: project Ice Storm. Early Hum Dev 2011; 87:813 - 20; http://dx.doi.org/10.1016/j.earlhumdev.2011.06.007; PMID: 21784587
- Dancause KN, Veru F, Andersen RE, Laplante DP, King S. Prenatal stress due to a natural disaster predicts insulin secretion in adolescence. Early Hum Dev 2013; 89:773 - 6; http://dx.doi.org/10.1016/j.earlhumdev.2013.06.006; PMID: 23830724
- Khashan AS, Abel KM, McNamee R, Pedersen MG, Webb RT, Baker PN, Kenny LC, Mortensen PB. Higher risk of offspring schizophrenia following antenatal maternal exposure to severe adverse life events. Arch Gen Psychiatry 2008; 65:146 - 52; http://dx.doi.org/10.1001/archgenpsychiatry.2007.20; PMID: 18250252
- Dancause KN, Vilar M, Chan C, DeHuff C, Wilson M, Soloway LE, Tarivonda L, Regenvanu R, Kaneko A, Garruto RM, et al. Patterns of childhood and adolescent overweight and obesity during health transition in Vanuatu. Public Health Nutr 2012; 15:158 - 66; http://dx.doi.org/10.1017/S1368980011001662; PMID: 21835097
- Hägglöf B, Blom L, Dahlquist G, Lönnberg G, Sahlin B. The Swedish childhood diabetes study: indications of severe psychological stress as a risk factor for type 1 (insulin-dependent) diabetes mellitus in childhood. Diabetologia 1991; 34:579 - 83; http://dx.doi.org/10.1007/BF00400277; PMID: 1936661
- Ronald A, Happé F, Dworzynski K, Bolton P, Plomin R. Exploring the relation between prenatal and neonatal complications and later autistic-like features in a representative community sample of twins. Child Dev 2010; 81:166 - 82; http://dx.doi.org/10.1111/j.1467-8624.2009.01387.x; PMID: 20331660
- King S, Laplante DP. The effects of prenatal maternal stress on children’s cognitive development: Project Ice Storm. Stress 2005; 8:35 - 45; http://dx.doi.org/10.1080/10253890500108391; PMID: 16019596
- D’Anna-Hernandez KL, Ross RG, Natvig CL, Laudenslager ML. Hair cortisol levels as a retrospective marker of hypothalamic-pituitary axis activity throughout pregnancy: comparison to salivary cortisol. Physiol Behav 2011; 104:348 - 53; http://dx.doi.org/10.1016/j.physbeh.2011.02.041; PMID: 21397617
- Demey-Ponsart E, Foidart JM, Sulon J, Sodoyez JC. Serum CBG, free and total cortisol and circadian patterns of adrenal function in normal pregnancy. J Steroid Biochem 1982; 16:165 - 9; http://dx.doi.org/10.1016/0022-4731(82)90163-7; PMID: 7078155
- Jung C, Ho JT, Torpy DJ, Rogers A, Doogue M, Lewis JG, Czajko RJ, Inder WJ. A longitudinal study of plasma and urinary cortisol in pregnancy and postpartum. J Clin Endocrinol Metab 2011; 96:1533 - 40; http://dx.doi.org/10.1210/jc.2010-2395; PMID: 21367926
- Myers B, McKlveen JM, Herman JP. Neural Regulation of the Stress Response: The Many Faces of Feedback. Cell Mol Neurobiol 2012; Forthcoming http://dx.doi.org/10.1007/s10571-012-9801-y; PMID: 22302180
- Duthie L, Reynolds RM. Changes in the maternal hypothalamic-pituitary-adrenal axis in pregnancy and postpartum: influences on maternal and fetal outcomes. Neuroendocrinology 2013; 98:106 - 15; http://dx.doi.org/10.1159/000354702; PMID: 23969897
- Petraglia F, Sawchenko PE, Rivier J, Vale W. Evidence for local stimulation of ACTH secretion by corticotropin-releasing factor in human placenta. Nature 1987; 328:717 - 9; http://dx.doi.org/10.1038/328717a0; PMID: 3039377
- Smith ID, Shearman RP. Fetal plasma steroids in relation to parturition. I. The effect of gestational age upon umbilical plasma corticosteroid levels following vaginal delivery. J Obstet Gynaecol Br Commonw 1974; 81:11 - 5; http://dx.doi.org/10.1111/j.1471-0528.1974.tb00357.x; PMID: 4818310
- Block MF, Kling OR, Crosby WM. Antenatal glucocorticoid therapy for the prevention of respiratory distress syndrome in the premature infant. Obstet Gynecol 1977; 50:186 - 90; PMID: 327364
- Doyle LW, Kitchen WH, Ford GW, Rickards AL, Kelly EA. Antenatal steroid therapy and 5-year outcome of extremely low birth weight infants. Obstet Gynecol 1989; 73:743 - 6; PMID: 2704500
- Eriksson L, Haglund B, Ewald U, Odlind V, Kieler H. Health consequences of prophylactic exposure to antenatal corticosteroids among children born late preterm or term. Acta Obstet Gynecol Scand 2012; 91:1415 - 21; http://dx.doi.org/10.1111/aogs.12014; PMID: 23006062
- Barbazanges A, Piazza PV, Le Moal M, Maccari S. Maternal glucocorticoid secretion mediates long-term effects of prenatal stress. J Neurosci 1996; 16:3943 - 9; PMID: 8656288
- Buss C, Davis EP, Shahbaba B, Pruessner JC, Head K, Sandman CA. Maternal cortisol over the course of pregnancy and subsequent child amygdala and hippocampus volumes and affective problems. Proc Natl Acad Sci U S A 2012; 109:E1312 - 9; http://dx.doi.org/10.1073/pnas.1201295109; PMID: 22529357
- Edwards CR, Benediktsson R, Lindsay RS, Seckl JR. Dysfunction of placental glucocorticoid barrier: link between fetal environment and adult hypertension?. Lancet 1993; 341:355 - 7; http://dx.doi.org/10.1016/0140-6736(93)90148-A; PMID: 8094124
- Gitau R, Cameron A, Fisk NM, Glover V. Fetal exposure to maternal cortisol. Lancet 1998; 352:707 - 8; http://dx.doi.org/10.1016/S0140-6736(05)60824-0; PMID: 9728994
- Huppertz B. The anatomy of the normal placenta. J Clin Pathol 2008; 61:1296 - 302; http://dx.doi.org/10.1136/jcp.2008.055277; PMID: 18755720
- Huppertz B, Borges M. Placenta trophoblast fusion. Methods Mol Biol 2008; 475:135 - 47; http://dx.doi.org/10.1007/978-1-59745-250-2_8; PMID: 18979242
- Brown RW, Chapman KE, Kotelevtsev Y, Yau JL, Lindsay RS, Brett L, Leckie C, Murad P, Lyons V, Mullins JJ, et al. Cloning and production of antisera to human placental 11 beta-hydroxysteroid dehydrogenase type 2. Biochem J 1996; 313:1007 - 17; PMID: 8611140
- Krozowski Z, MaGuire JA, Stein-Oakley AN, Dowling J, Smith RE, Andrews RK. Immunohistochemical localization of the 11 beta-hydroxysteroid dehydrogenase type II enzyme in human kidney and placenta. J Clin Endocrinol Metab 1995; 80:2203 - 9; PMID: 7608280
- Sun K, Yang K, Challis JR. Differential expression of 11 beta-hydroxysteroid dehydrogenase types 1 and 2 in human placenta and fetal membranes. J Clin Endocrinol Metab 1997; 82:300 - 5; PMID: 8989277
- Cuffe JS, O’Sullivan L, Simmons DG, Anderson ST, Moritz KM. Maternal corticosterone exposure in the mouse has sex-specific effects on placental growth and mRNA expression. Endocrinology 2012; 153:5500 - 11; http://dx.doi.org/10.1210/en.2012-1479; PMID: 22919064
- Welberg LA, Thrivikraman KV, Plotsky PM. Chronic maternal stress inhibits the capacity to up-regulate placental 11beta-hydroxysteroid dehydrogenase type 2 activity. J Endocrinol 2005; 186:R7 - 12; http://dx.doi.org/10.1677/joe.1.06374; PMID: 16135661
- Mairesse J, Lesage J, Breton C, Bréant B, Hahn T, Darnaudéry M, Dickson SL, Seckl J, Blondeau B, Vieau D, et al. Maternal stress alters endocrine function of the feto-placental unit in rats. Am J Physiol Endocrinol Metab 2007; 292:E1526 - 33; http://dx.doi.org/10.1152/ajpendo.00574.2006; PMID: 17264224
- Belkacemi L, Desai M, Beall MH, Liu Q, Lin JT, Nelson DM, Ross MG. Early compensatory adaptations in maternal undernourished pregnancies in rats: role of the aquaporins. J Matern Fetal Neonatal Med 2011; 24:752 - 9; http://dx.doi.org/10.3109/14767058.2010.521870; PMID: 20958229
- Lesage J, Blondeau B, Grino M, Bréant B, Dupouy JP. Maternal undernutrition during late gestation induces fetal overexposure to glucocorticoids and intrauterine growth retardation, and disturbs the hypothalamo-pituitary adrenal axis in the newborn rat. Endocrinology 2001; 142:1692 - 702; PMID: 11316731
- Ghaemmaghami P, Dainese SM, La Marca R, Zimmermann R, Ehlert U. The association between the acute psychobiological stress response in second trimester pregnant women, amniotic fluid glucocorticoids, and neonatal birth outcome. Dev Psychobiol 2013; Forthcoming PMID: 23775363
- O’Donnell KJ, Bugge Jensen A, Freeman L, Khalife N, O’Connor TG, Glover V. Maternal prenatal anxiety and downregulation of placental 11β-HSD2. Psychoneuroendocrinology 2012; 37:818 - 26; http://dx.doi.org/10.1016/j.psyneuen.2011.09.014; PMID: 22001010
- Kvetnanský R, Pacák K, Fukuhara K, Viskupic E, Hiremagalur B, Nankova B, Goldstein DS, Sabban EL, Kopin IJ. Sympathoadrenal system in stress. Interaction with the hypothalamic-pituitary-adrenocortical system. Ann N Y Acad Sci 1995; 771:131 - 58; PMID: 8597393
- Sarkar S, Tsai SW, Nguyen TT, Plevyak M, Padbury JF, Rubin LP. Inhibition of placental 11beta-hydroxysteroid dehydrogenase type 2 by catecholamines via alpha-adrenergic signaling. Am J Physiol Regul Integr Comp Physiol 2001; 281:R1966 - 74; PMID: 11705783
- Coussons-Read ME, Okun ML, Schmitt MP, Giese S. Prenatal stress alters cytokine levels in a manner that may endanger human pregnancy. Psychosom Med 2005; 67:625 - 31; http://dx.doi.org/10.1097/01.psy.0000170331.74960.ad; PMID: 16046378
- Chisaka H, Johnstone JF, Premyslova M, Manduch Z, Challis JR. Effect of pro-inflammatory cytokines on expression and activity of 11beta-hydroxysteroid dehydrogenase type 2 in cultured human term placental trophoblast and human choriocarcinoma JEG-3 cells. J Soc Gynecol Investig 2005; 12:303 - 9; http://dx.doi.org/10.1016/j.jsgi.2005.02.003; PMID: 15979541
- Kossintseva I, Wong S, Johnstone E, Guilbert L, Olson DM, Mitchell BF. Proinflammatory cytokines inhibit human placental 11beta-hydroxysteroid dehydrogenase type 2 activity through Ca2+ and cAMP pathways. Am J Physiol Endocrinol Metab 2006; 290:E282 - 8; http://dx.doi.org/10.1152/ajpendo.00328.2005; PMID: 16174654
- Suzuki S, Tsubochi H, Ishibashi H, Matsuda Y, Suzuki T, Krozowski ZS, Sasano H, Kondo T. Inflammatory mediators down-regulate 11beta-hydroxysteroid dehydrogenase type 2 in a human lung epithelial cell line BEAS-2B and the rat lung. Tohoku J Exp Med 2005; 207:293 - 301; http://dx.doi.org/10.1620/tjem.207.293; PMID: 16272800
- Reamon-Buettner SM, Mutschler V, Borlak J. The next innovation cycle in toxicogenomics: environmental epigenetics. Mutat Res 2008; 659:158 - 65; http://dx.doi.org/10.1016/j.mrrev.2008.01.003; PMID: 18342568
- Marsit CJ, Maccani MA, Padbury JF, Lester BM. Placental 11-beta hydroxysteroid dehydrogenase methylation is associated with newborn growth and a measure of neurobehavioral outcome. PLoS One 2012; 7:e33794; http://dx.doi.org/10.1371/journal.pone.0033794; PMID: 22432047
- Alikhani-Koopaei R, Fouladkou F, Frey FJ, Frey BM. Epigenetic regulation of 11 beta-hydroxysteroid dehydrogenase type 2 expression. J Clin Invest 2004; 114:1146 - 57; http://dx.doi.org/10.1172/JCI21647; PMID: 15489962
- Lister R, Mukamel EA, Nery JR, Urich M, Puddifoot CA, Johnson ND, Lucero J, Huang Y, Dwork AJ, Schultz MD, et al. Global epigenomic reconfiguration during mammalian brain development. Science 2013; 341:1237905; http://dx.doi.org/10.1126/science.1237905; PMID: 23828890
- Jensen Peña C, Monk C, Champagne FA. Epigenetic effects of prenatal stress on 11β-hydroxysteroid dehydrogenase-2 in the placenta and fetal brain. PLoS One 2012; 7:e39791; http://dx.doi.org/10.1371/journal.pone.0039791; PMID: 22761903
- Conradt E, Lester BM, Appleton AA, Armstrong DA, Marsit CJ. The roles of DNA methylation of NR3C1 and 11β-HSD2 and exposure to maternal mood disorder in utero on newborn neurobehavior. Epigenetics 2013; 8:1321 - 9; http://dx.doi.org/10.4161/epi.26634; PMID: 24135662
- Nylen KJ, Williamson JA, O’Hara MW, Watson D, Engeldinger J. Validity of somatic symptoms as indicators of depression in pregnancy. Arch Womens Ment Health 2013; 16:203 - 10; http://dx.doi.org/10.1007/s00737-013-0334-2; PMID: 23456541
- Goffin J, Eisenhauer E. DNA methyltransferase inhibitors-state of the art. Ann Oncol 2002; 13:1699 - 716; http://dx.doi.org/10.1093/annonc/mdf314; PMID: 12419742
- Santini V, Gozzini A, Scappini B, Grossi A, Rossi Ferrini P. Searching for the magic bullet against cancer: the butyrate saga. Leuk Lymphoma 2001; 42:275 - 89; http://dx.doi.org/10.3109/10428190109064584; PMID: 11699392
- Santini V, Kantarjian HM, Issa JP. Changes in DNA methylation in neoplasia: pathophysiology and therapeutic implications. Ann Intern Med 2001; 134:573 - 86; http://dx.doi.org/10.7326/0003-4819-134-7-200104030-00011; PMID: 11281740
- Wagner JM, Hackanson B, Lübbert M, Jung M. Histone deacetylase (HDAC) inhibitors in recent clinical trials for cancer therapy. Clin Epigenetics 2010; 1:117 - 36; http://dx.doi.org/10.1007/s13148-010-0012-4; PMID: 21258646
- Cisneros FJ, Wilson R, Travlos G, Anderson LM, Branch S. Susceptibility to postnatal growth retardation induced by 5-AZA-2′-deoxycytidine in utero: gender specificity and correlation with reduced insulin-like growth factor 1. Life Sci 2003; 72:2887 - 94; http://dx.doi.org/10.1016/S0024-3205(03)00229-7; PMID: 12697271
- Ding YB, Long CL, Liu XQ, Chen XM, Guo LR, Xia YY, He JL, Wang YX. 5-aza-2′-deoxycytidine leads to reduced embryo implantation and reduced expression of DNA methyltransferases and essential endometrial genes. PLoS One 2012; 7:e45364; http://dx.doi.org/10.1371/journal.pone.0045364; PMID: 23028963
- Momparler RL, Bouffard DY, Momparler LF, Dionne J, Belanger K, Ayoub J. Pilot phase I-II study on 5-aza-2′-deoxycytidine (Decitabine) in patients with metastatic lung cancer. Anticancer Drugs 1997; 8:358 - 68; http://dx.doi.org/10.1097/00001813-199704000-00008; PMID: 9180389
- Gabory A, Ferry L, Fajardy I, Jouneau L, Gothié JD, Vigé A, Fleur C, Mayeur S, Gallou-Kabani C, Gross MS, et al. Maternal diets trigger sex-specific divergent trajectories of gene expression and epigenetic systems in mouse placenta. PLoS One 2012; 7:e47986; http://dx.doi.org/10.1371/journal.pone.0047986; PMID: 23144842
- Gallou-Kabani C, Gabory A, Tost J, Karimi M, Mayeur S, Lesage J, Boudadi E, Gross MS, Taurelle J, Vigé A, et al. Sex- and diet-specific changes of imprinted gene expression and DNA methylation in mouse placenta under a high-fat diet. PLoS One 2010; 5:e14398; http://dx.doi.org/10.1371/journal.pone.0014398; PMID: 21200436
- Ogden CL, Carroll MD, Curtin LR, McDowell MA, Tabak CJ, Flegal KM. Prevalence of overweight and obesity in the United States, 1999-2004. JAMA 2006; 295:1549 - 55; http://dx.doi.org/10.1001/jama.295.13.1549; PMID: 16595758
- Allegri C, Turconi G, Cena H. Dietary attitudes and diseases of comfort. Eat Weight Disord 2011; 16:e226 - 35; http://dx.doi.org/10.1007/BF03327465; PMID: 22526128
- Smith GC, Shah I, Pell JP, Crossley JA, Dobbie R. Maternal obesity in early pregnancy and risk of spontaneous and elective preterm deliveries: a retrospective cohort study. Am J Public Health 2007; 97:157 - 62; http://dx.doi.org/10.2105/AJPH.2005.074294; PMID: 17138924
- Triunfo S, Lanzone A. Impact of overweight and obesity on obstetric outcomes. J Endocrinol Invest 2014; Forthcoming http://dx.doi.org/10.1007/s40618-014-0058-9; PMID: 24515300
- West AA, Caudill MA. Applied Choline-Omics: Lessons from Human Metabolic Studies for the Integration of Genomics Research into Nutrition Practice. J Acad Nutr Diet 2014
- Jiang X, Yan J, West AA, Perry CA, Malysheva OV, Devapatla S, Pressman E, Vermeylen F, Caudill MA. Maternal choline intake alters the epigenetic state of fetal cortisol-regulating genes in humans. FASEB J 2012; 26:3563 - 74; http://dx.doi.org/10.1096/fj.12-207894; PMID: 22549509
- Takaya J, Iharada A, Okihana H, Kaneko K. Magnesium deficiency in pregnant rats alters methylation of specific cytosines in the hepatic hydroxysteroid dehydrogenase-2 promoter of the offspring. Epigenetics 2011; 6:573 - 8; http://dx.doi.org/10.4161/epi.6.5.15220; PMID: 21406963
- Loke YJ, Galati JC, Morley R, Joo EJ, Novakovic B, Li X, Weinrich B, Carson N, Ollikainen M, Ng HK, et al. Association of maternal and nutrient supply line factors with DNA methylation at the imprinted IGF2/H19 locus in multiple tissues of newborn twins. Epigenetics 2013; 8:1069 - 79; http://dx.doi.org/10.4161/epi.25908; PMID: 23917818
- Belkacemi L, Jelks A, Chen CH, Ross MG, Desai M. Altered placental development in undernourished rats: role of maternal glucocorticoids. Reprod Biol Endocrinol 2011; 9:105; http://dx.doi.org/10.1186/1477-7827-9-105; PMID: 21806804
- Bertram C, Trowern AR, Copin N, Jackson AA, Whorwood CB. The maternal diet during pregnancy programs altered expression of the glucocorticoid receptor and type 2 11beta-hydroxysteroid dehydrogenase: potential molecular mechanisms underlying the programming of hypertension in utero. Endocrinology 2001; 142:2841 - 53; PMID: 11416003
- Langley-Evans SC, Gardner DS, Jackson AA. Maternal protein restriction influences the programming of the rat hypothalamic-pituitary-adrenal axis. J Nutr 1996; 126:1578 - 85; PMID: 8648431
- Whorwood CB, Donovan SJ, Wood PJ, Phillips DI. Regulation of glucocorticoid receptor alpha and beta isoforms and type I 11beta-hydroxysteroid dehydrogenase expression in human skeletal muscle cells: a key role in the pathogenesis of insulin resistance?. J Clin Endocrinol Metab 2001; 86:2296 - 308; PMID: 11344242
- Whorwood CB, Firth KM, Budge H, Symonds ME. Maternal undernutrition during early to midgestation programs tissue-specific alterations in the expression of the glucocorticoid receptor, 11beta-hydroxysteroid dehydrogenase isoforms, and type 1 angiotensin ii receptor in neonatal sheep. Endocrinology 2001; 142:2854 - 64; PMID: 11416004
- Garbrecht MR, Lamb FS. Placental HSD2 Expression and Activity Is Unaffected by Maternal Protein Consumption or Gender in C57BL/6 Mice. ISRN Endocrinol 2013; 2013:867938; http://dx.doi.org/10.1155/2013/867938; PMID: 23781346
- Sferruzzi-Perri AN, Vaughan OR, Haro M, Cooper WN, Musial B, Charalambous M, Pestana D, Ayyar S, Ferguson-Smith AC, Burton GJ, et al. An obesogenic diet during mouse pregnancy modifies maternal nutrient partitioning and the fetal growth trajectory. FASEB J 2013; 27:3928 - 37; http://dx.doi.org/10.1096/fj.13-234823; PMID: 23825226
- Mao J, Zhang X, Sieli PT, Falduto MT, Torres KE, Rosenfeld CS. Contrasting effects of different maternal diets on sexually dimorphic gene expression in the murine placenta. Proc Natl Acad Sci U S A 2010; 107:5557 - 62; http://dx.doi.org/10.1073/pnas.1000440107; PMID: 20212133