Abstract
Epigenetic alterations occur in tumor-associated vessels in the tumor microenvironment. Methylation of the CYP24A1 gene promoter differs in endothelial cells isolated from tumors and non-tumor microenvironments in mice. The epigenetic makeup of endothelial cells of human tumor-associated vasculature is unknown due to difficulty of isolating endothelial cells populations from a heterogeneous tissue microenvironment. To ascertain CYP24A1 promoter methylation in tumor-associated endothelium, we utilized laser microdissection guided by CD31 immunohistochemistry to procure endothelial cells from human prostate tumor specimens. Prostate tissues were obtained following robotic radical prostatectomy from men with clinically localized prostate cancer. Adjacent histologically benign prostate tissues were used to compare endothelium from benign versus tumor microenvironments. Sodium bisulfite sequencing of CYP24A1 promoter region showed that the average CYP24A1 promoter methylation in the endothelium was 20% from the tumor microenvironment compared with 8.2% in the benign microenvironment (p<0.05). A 2-fold to 17-fold increase in CYP24A1 promoter methylation was observed in the prostate tumor endothelium compared with the matched benign prostate endothelium in four patient samples, while CYP24A1 remained unchanged in two patient sample. In addition, there is no correlation of the level of CYP24A1 promoter methylation in prostate tumor-associated endothelium with that of epithelium/stroma. This study demonstrates that the CYP24A1 promoter is methylated in tumor-associated endothelium, indicating that epigenetic alterations in CYP24A1 may play a role in determining the phenotype of tumor-associated vasculature in the prostate tumor microenvironment.
Introduction
The tissue levels of the biologically active form of vitamin D3 (calcitriol, 1α,25-(OH)2D3) are tightly controlled through feedback regulation of its biosynthesis by α-hydroxylase (CYP27B1) and catabolism by the cytochrome P450 enzyme 25-hydroxyvitamin D 24-hydroxylase (24-hydroxylase). The 24-hydroxylase enzyme, encoded by the CYP24A1 gene, is primarily responsible for catabolism of calcitriol to the less active vitamin D metabolites 1α,Citation24,Citation25-(OH)2D3 and calcitroic acid.Citation1 Therefore, CYP24A1 enzyme expression may impact the regulation of vitamin D homeostasis, calcitriol bioavailability and exposure, and the therapeutic potential of calcitriol in different tumor cell types.Citation2–Citation4 Amplification of the CYP24A1 gene copy in chromosomal region 20q13.2 was observed in breast cancer.Citation5 Overexpression of CYP24A1 is associated with poor prognosis in esophageal cancerCitation6 and poorly differentiated colon carcinoma.Citation7 Studies have shown that epigenetic modulation is involved in regulation of CYP24A1 expression in human placenta and prostate cancer.Citation8–Citation10
Angiogenesis, the development of new blood vessels, plays a major role in tumor growth and metastasis.Citation11 Tumor angiogenesis involves capillary sprouting and abnormal branching, abnormal pericyte coat and loss of pericyte-endothelial cell adhesion, defects in the basement membrane and endothelial monolayer, and increased permeability, vasodilation and leakiness.Citation12,Citation13 Endothelial cells from tumor blood vessels, once thought to be genetically normal and stable compared to genetically unstable tumor cells, demonstrate differences in their transcriptome,Citation14 cytogenetic abnormalitiesCitation15 and functional abnormalities.Citation16 Epigenetic modifications are also implicated in regulating gene expression in the vascular endothelium.Citation17 Genes reported to be epigenetically silenced in the endothelial cells through promoter modification are ICAM-1,Citation18 endothelial nitric oxide synthaseCitation19 and estrogen receptor-β.Citation20 Higher GSTP1 and RARβ2 promoter methylation were observed in tumor-associated endothelium compared to normal prostate endothelium.Citation21
Our laboratory developed a system for isolation of fresh endothelial cells from murine tumors (TDEC) and normal tissue (Matrigel or normal tissue, MDEC) using dissociation of tissues and flow cytometric analysis. In TDEC the CYP24a1 is silenced and its promoter CpG island is hypermethylated. Treatment of TDEC with a DNA methyltransferase inhibitor restores calcitriol-mediated induction of CYP24a1. In contrast, CYP24a1 in endothelial cells isolated from normal tissues/MDEC are not silenced and its promoter CpG island is not methylated. This suggests that hypermethylation may contribute to gene silencing of CYP24a1. More importantly, treatment of TDEC with calcitriol induces G0/G1 arrest, modulates p27 and p21, induces apoptotic cell death and decreases P-Erk and P-Akt. However, endothelial cells isolated from normal tissues/MDEC are unresponsive to calcitriol-mediated anti-proliferative effects despite intact signaling through the vitamin D receptor (VDR). These data suggest that epigenetic silencing of CYP24A1 modulates cellular responses to calcitriol.Citation22,Citation23
In the current study, we established a protocol for laser microdissection (LMD) by CD31 immunophenotyping to identify and procure endothelium from regions of human benign and tumor tissues from patients with clinically localized prostate cancer. Laser-assisted microdissection is a commonly used method to study the cellular and molecular interactions of different cell populations within their tissue microenvironment.Citation24,Citation25 Using CD31-guided LMD, we compared CYP24A1 methylation status between endothelium from benign and tumor microenvironments for each prostate cancer patient specimen.
Results
Frozen prostate specimens obtained from robotic radical prostatectomy were evaluated by hematoxylin and eosin for benign prostate and prostate adenocarcinoma (tumor) regions ( and B). The matched prostate adenocarcinomas consisted of moderate grade tumors (Gleason = 7, n = 5) and high-grade tumors (Gleason 8+, n = 1). Immunohistochemical (IHC) staining of tissues prior to LMD enabled the identification of endothelial cells in the tumor microenvironment by combining morphologic and immunophenotypic criteria.Citation21,Citation24,Citation26,Citation27 Cryosections of the benign prostate and prostate tumor tissues were stained for the CD31 endothelial marker as shown in and D, respectively, and laser microdissection was performed with an ultraviolet laser using the Leica LMD6000.
Endothelial cells of the blood vessels can be easily distinguished from other cells by the brown staining of their cell surface membranes in contrast to the background (–F, H and I). Benign prostate and prostate tumor sections selected for UV laser dissection (outlined) before and after dissection are shown in . Endothelial cells that are dissected from the tissue section maintained their architecture and are shown in . The brown staining is no long visible on the tissue section after microdissection, indicating that the endothelium was removed (). Due to differences among samples in specimen size, endothelium architecture and vascular density, the total square microns of material collected ranged from 6 to 34 million µm2 for benign prostate specimens and 13 to 76 million µm2 for tumor specimens (). Overall DNA yields attained for DNA methylation analysis ranged from 152 to 2,086 ng for benign prostate specimens and 274 to 1,498 ng for tumor specimens ().
The methylation status of the 5′ CpG island region of CYP24A1 from microdissected samples was determined using bisulfite sequencingCitation8 (). We sequenced the 5′ CpG-rich region 2 of the CYP24A1 gene promoter since DNA hypermethylation had previously been observed in other human prostate cancer specimens ().Citation8 Bisulfite DNA sequencing of twenty individual clones for benign endothelium and tumor endothelium from Patient #1 show 7.3% and 35.6% methylation of the CYP24A1 promoter CpG island region 2, respectively (). Consistent with our previous findings, the positive and negative methylation cell line controls for CYP24A1 methylation show 84.5% methylation in the PC-3 cell line and no methyation in the DU145 cell line ().Citation8 Of our six matched sample sets, the CYP24A1 promoter sites were on average 20% methylated in the endothelium from the tumor microenvironment compared with 8.2% in endothelium from the benign microenvironment (p = 0.045) ( and ). There was no correlation between CYP24A1 methylation and Gleason score. CYP24A1 methylation varied between 8% to 36% methylation in tumor endothelium of patient matched prostate specimens. Four of six prostate cancer patient samples showed a 2-fold to 16-fold increase in CYP24A1 promoter CpG island region 2 methylation in the prostate tumor endothelium compared with the matched benign endothelium (, and B and Sup. Fig. 1). Overall, the tumor-associated endothelium demonstrated an average 4.8-fold increase in CYP24A1 promoter methylation when compared to benign endothelium ( and ). Finally, the CYP24A1 promoter methylation in prostate tumor-associated endothelium and remaining de-vascularized tissue sections did not correlate ().
Discussion
We have previously shown that the CYP24a1 gene promoter is hypermethylated in endothelial cells derived from different tumor cell types in a mouse model.Citation23 Epigenetic silencing of CYP24a1 in TDEC from a mouse syngeneic tumor model contributes to selective growth inhibition by calcitriol.Citation23 To investigate whether the CYP24A1 gene is differentially methylated in endothelial cells from human cancers, we examined the methylation status of the CYP24A1 promoter of tumor-associated and benign-associated endothelium from prostate cancer tissues. We examined CYP24A1 methylation status in prostate cancer specimens for these reasons: (1) CYP24A1 expression is epigenetically regulated in human prostate cancer cell lines,Citation8 (2) TDEC from PC-3 xenograft shows increased CYP24a1 promoter methylationCitation23 and (3) methylation of GSTP1 and RARβ2 promoters was reported in tumor-associated endothelium and stroma of localized human prostate cancer.Citation21,Citation28 We are unaware of previous studies demonstrating differential CYP24A1 promoter methylation in the endothelium associated with distinct malignant and benign human prostate microenvironments.
CD31-guided LMD was utilized to identify and enrich the collection of endothelium from benign prostate and tumor microenvironments. LMD enables the isolation of an intact endothelium with no obvious contamination from other cell population. Immunohistochemical staining with the CD31 antibody limited our ability to perform mRNA analysis due to degradation and loss of RNA through activation tissue. Although rapid immunostaining techniques reduce specimen preparation time and lessen mRNA degradation, β-actin mRNA levels were reduced by 99%, indicating that endogenous RNAses were not inhibited.Citation29 DNA markers that discriminate among endothelial, stromal and epithelial cells do not exist. Given these limitations, CD31-stained and LMD-captured endothelial tissue was compared to the remaining de-vascularized tissue sections to assess the ability of LMD to enrich our tissue material for endothelial cells. If endothelial populations were not enriched, the methylation status of de-vascularized tissue sections would be very similar to that of laser-captured endothelial tissues. We found that de-vascularized tumor tissue sections demonstrated different methylation compared to the tumor endothelium.
Epigenetic differences between tumor and stromal elements, consisting of fibroblasts, inflammatory cells and endothelial cells, have been reported in breast tumorsCitation30 and in distinct prostate tumor sub-regions.Citation31 The lack of expression of the CYP24a1 gene in TDEC is associated with DNA methylation of the upstream promoter and the first exon of the CYP24a1 gene in TDEC.Citation23 It is unclear what level of methylation affects CYP24A1 gene expression in human tumor-associated endothelium. While methylation of CYP24A1 may not silence the CYP24A1 gene, the extent of methylation modifies the level of expression and activity of the gene upon calcitriol induction in prostate cancer cell lines and may be regulated by repressive histone modifications.Citation8 Downregulated genes (ICAM-1, clusterin, fibrillin and quiescin Q6) in tumor-conditioned versus quiescent endothelial cells were associated with promoter histone modifications and not with promoter DNA methylation.Citation32 These genes could be reactivated by DNA methyltransferase (DNMT) and histone deacetylase (HDAC) inhibitors,Citation32 thus providing a mechanism for the epigenetic regulation of tumor endothelial cells. In human microvascular endothelial cells and pericytes, gene silencing of stromal cell-derived factor 1 (SDF-1), which regulates neovascularization, is regulated by histone modification and not by DNA promoter methylation.Citation33 The association of CYP24A1 expression in tumor endothelium with CYP24A1 promoter histone modifications or methylation has not been studied in clinical specimens of human tumor endothelium and warrants further investigation.
The mechanisms that cause epigenetic alterations in the tumor microenvironment are unclear. One possibility is that tumor cells can secrete factors into the microenvironment which affect surrounding cells and modulate the epigenetic status of genes. Interleukin-6 (IL-6), which is secreted by tumor cells, has been shown to silence the expression of p53 through promoter methylation in tumor cells.Citation34 Bao et al. demonstrated that conditioned medium from LNCaP, PC-3 and DU145 prostate cancer cell lines stimulated human umbilical vein endothelial cells (HUVEC) tube formation and migration.Citation35 Phenotypical and biological changes observed in the endothelial cells were inhibited by calcitriol treatment, suggesting that the tumor microenvironment can impact endothelial cell function, modulate endothelial cell response to calcitriol, and regulate other angiogenic factors by calcitriol.Citation35 However, secreted factors that can epigenetically regulate genes in tumor endothelial cells have not been identified. While it is unclear why CYP24A1 is methylated in tumor endothelium, preliminary results from our microarray gene expression analysis indicate that a number of cytochrome P450 enzymes were downregulated in TDEC when compared to MDEC. This may be due to the hypoxic tumor microenvironment, where cytochrome P450 enzyme expression and activity are downregulated under hypoxic conditions.Citation36 Freshly isolated endothelial cells from the tumor microenvironment of xenografts have demonstrated that the epigenetic regulation of CYP24A1 affected both gene expression and activity.Citation23 In prostate tissue samples studied by Luo et al. there was no overall correlation between the level of CYP24A1 expression and methylation.Citation8 However, mechanistic studies demonstrated that activation of CYP24A1 expression by epigenetic modulatory drugs, trichostatin A and 5-aza-2′-deoxycytidine, in prostate cancer cells increased CYP24A1 activity.Citation8
The role of methylation of the CYP24A1 promoter in the tumor vasculature remains unknown due to the difficulty of showing the expression or activity of CYP24A1 in prostate cancer associated vessels. To show this in human tissues would be difficult experimentally as one would have to give patients calcitriol and then isolate tumor endothelium in such a way that expression can be evaluated. CYP24A1 promoter methylation affected vitamin D bioavailability and activity in placenta.Citation9 Since maternal circulating 1,25(OH)2D levels increase dramatically during pregnancyCitation37 with ∼50% of 1,25(OH)2D derived from decidua and placenta,Citation38 epigenetic downregulation of the CYP24A1 gene in the placenta may be physiologically necessary to modulate 1,25-(OH)2D levels at the fetomaternal interface to sustain fetal growth. Although the ability of CYP24A1 to regulate tissue level of 25(OH)D and 1,25(OH)2D has not been determined in tumorigenesis, one can speculate that CYP24A1 methylation in the tumor endothelium may play a physiological role in modulating vitamin D bioavailability to the tumor because low serum 25(OH)D levels might be associated with increased risk and mortality of some cancers.Citation39 CYP24A1 promoter methylation may be a component in which the tumor microenvironment maintains adequate vitamin D levels to support endothelial integrity and normal function of vessels and sustain tumor growth. Therefore, if a defect in the regulation of CYP24A1 in tumor-associated endothelium can facilitate the growth of tumor, determining ways to modulate CYP24A1 expression or activity in the tumor microenvironment could lead to a more effective treatment.
Elucidating the physical and molecular interactions in the tumor microenvironment will contribute to our understanding of angiogenesis. Epigenetics of the tumor vasculature provide a new perspective on transcription control paradigms in vascular endothelial cells and provide a molecular basis for how the environment impacts disease progression. Genome-wide methylation analyses coupled with LMD of immunostained cells may allow us to further define the epigenome of tumor vasculature. Deciphering the extent of the epigenetic changes and identifying other potential targets of altered methylation in tumor endothelium are likely to lead to more effective clinical interventions directed to the tumor vasculature.
Materials and Methods
Human tissues specimen and cell lines.
Matched pairs of tumor and benign prostate tissues were obtained from robotic radical prostatectomy specimens from men (n = 6) with clinically organ-confined prostate cancer as previously described in reference Citation40. The research was approved by the Roswell Park Cancer Institute Institutional Review Board, and all patients provided written informed consent. Tumor size varies in prostate cancer and we required sufficient tumor for our analysis. Regions of benign and prostatic adenocarcinoma (tumor) for all specimens were graded by Gleason scoring by pathologists, macro-dissected and mounted in OCT. PC-3 and DU145 prostate adenocarcinoma cell lines were obtained from American Type Culture Collection (Manassas, VA) and cultured under manufacturer's conditions.
Immunohistochemistry and laser microdissection (LMD) of endothelium.
Eight-micron thick histological sections were mounted on polyethylene naphthalate (PEN)-membrane coated slides (Leica, Germany) and air dried for 10 min. Cryostat blades were replaced between OCT frozen specimens to prevent carryover and cross-contamination of previous samples. Slides were fixed in ice-cold acetone for 20 min, air dried for 5 min, washed with ddH2O and blocked with peroxidase for 5 min. Slides were washed thrice with PBS/Tween (PBS/T) for 5 min each wash. For endothelial cell staining, 1:50 dilution (1.25 µg/ml) of mouse anti-human CD31 primary antibody (clone WM59) (BD Pharmingen™, San Diego, CA USA) was applied for 30 min and washed thrice with PBS/T for 5 min each time. The anti-mouse secondary antibody from the DAKO Envision(+) System-HRP (DAKO/Cytomation, Carpinteria, CA) was incubated for 30 min and washed thrice with PBS/T. The DAB chromogen was applied for 5 min. Sections were washed with ddH2O, dehydrated in graded alcohols, immersed in xylene for 20 seconds and air dried. The sections were not counterstained to enhance the contrast between the stained and unstained areas. Pathologists identified the presence of prostate tumor from hematoxylin and eosin (H&E) and CD31 stained sections; H&E stained sections were performed on the first and last sections of the samples that were subjected to LMD.
Laser microdissection of CD31 stained endothelium was performed at 200× magnification with the following laser control setting parameters: power (80), speed (2) and specimen balance (11), using the Leica LMD6000 (Leica, Germany). CD31+ stained endothelium were collected into the lid of a 0.5-ml microcentrifuge tube. Tissue sections post-laser microdissected for CD31+ endothelium were retained as de-vascularized controls, which contain remaining epithelial and stromal cell types.
DNA extraction and sodium bisulfite DNA sequencing.
DNA from microdissected CD31+ endothelium from benign and tumor tissues was extracted with QIAamp DNA Micro Kit (QIAGEN, Valencia, CA). Final eluted DNA from the same benign or tumor tissue section was pooled and quantified with the NanoDrop 8000 Spectrophotometer (Thermo Scientific, Wilmington, DE). DNA was bisulfite modified using the EZ DNA Methylation Kit™ (Zymo Research, Orange, CA). The CYP24A1 5′ CpG island region was amplified and cloned into pCR®4-TOPO using the TOPO TA cloning kit for sequencing (Invitrogen, Carlsbad, CA), and sequenced (DNA Sequencing Core, RPCI, Buffalo, NY) as previously described in reference Citation8.
Disclosure of Potential Conflicts of Interest
No potential conflicts of interest were disclosed.
Financial Support
This research was supported by NIH/NCI grants CA067267, CA085142 and CA095045 (C.S.J., D.L.T.) and by the American Cancer Society Institutional Research Grant 02-197-04 (K.K.D.).
Abbreviations
TDEC | = | endothelial cells derived from tumors |
MDEC | = | endothelial cells derived from matrigel |
VDR | = | vitamin D receptor |
LMD | = | laser microdissection |
IHC | = | immunohistochemical |
SDF-1 | = | stromal cell-derived factor 1 |
Figures and Tables
Figure 1 Representative photomicrographs of matched Hematoxylin and Eosin stained frozen sections of benign (A) and malignant (B) prostatic tissues and CD31 immunostained frozen sections of benign (C, E and F) and malignant (D, H–J) prostatic tissues prepared for LMD. The benign prostatic tissue shows stromal and glandular hyperplasia and the malignant prostatic tissue shows well-differentiated adenocarcinoma. Endothelial cells are highlighted by CD31 immunostaining of matched benign (C) and malignant (D) prostate frozen tissue on a PEN membrane slides. It was a common observation that malignant prostatic tissues had higher microvascular density than benign prostatic tissues. (E and H) represent CD31 immunostained tissue sections before microdissection. (F and I) represent outlined endothelium to be dissected. (G) represents captured endothelium from the slide in a collection cap. (J) represents the remaining tissue sections after microdissection.
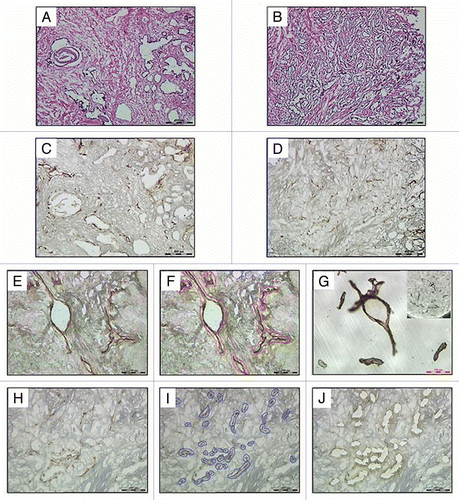
Figure 2 CYP24A1 promoter DNA methylation of endothelium associated with benign and malignant prostate tissues. (A) Diagram of the CYP24A1 promoter CpG-rich region. Twenty individual clones were sequenced from the CYP24A1 promoter region and shown from a representative patient sample (left). Positive (+) and negative (−) methylation controls from PC-3 and DU145, respectively, are shown (right). The methylation percentage indicates total proportion of methylated CpGs in the indicated CYP24A1 promoter region for each sample, taking into account all sequenced alleles. (B) Results indicate that the CYP24A1 promoter is methylated in the CD31 enriched endothelium associated with human prostate tumor compared to benign prostate (p = 0.045, paired, two-tailed Student's t-Test). Mean ± SEM values are shown. Matched patient pair of prostate tumor and benign prostate endothelium are shown with symbols: Patient #1 (■), Patient #2 (△), Patient #3 (○), Patient #4 (◊), Patient #5 (●) and Patient #6 (▲). (C) Lack of correlation of CYP24A1 promoter region 2 methylation between CD31+ endothelium from LMD and remaining epithelium/stroma from the de-vascularized sections.
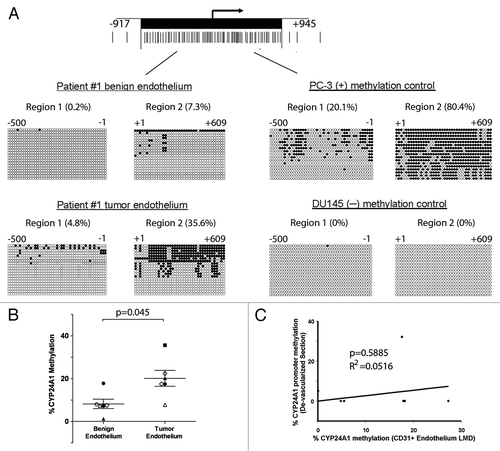
Table 1 Laser microdissection of CD31+ cells from prostate tumor and matched histologically benign prostatic tissues from radical prostectectomy
Table 2 Percent CYP24A1 promoter methylation of CD31+ endothelium from matched benign and tumor prostatic tissues
Additional material
Download Zip (2.2 MB)Acknowledgments
We thank Philip Ferenczy and Nancy Reska for their assistance in prostate tissue procurement. We also acknowledge Michelle Panasiewicz and Karen Head for their technical assistance.
References
- Omdahl JL, Bobrovnikova EA, Choe S, Dwivedi PP, May BK. Overview of regulatory cytochrome P450 enzymes of the vitamin D pathway. Steroids 2001; 66:381 - 389
- Kasuga H, Hosogane N, Matsuoka K, Mori I, Sakura Y, Shimakawa K, et al. Characterization of transgenic rats constitutively expressing vitamin D-24-hydroxylase gene. Biochem Biophys Res Commun 2002; 297:1332 - 1338
- Masuda S, Byford V, Arabian A, Sakai Y, Demay MB, St-Arnaud R, et al. Altered pharmacokinetics of 1alpha,25-dihydroxyvitamin D3 and 25-hydroxyvitamin D3 in the blood and tissues of the 25-hydroxyvitamin D-24-hydroxylase (Cyp24a1) null mouse. Endocrinology 2005; 146:825 - 834
- Deeb KK, Trump DL, Johnson CS. Vitamin D signalling pathways in cancer: potential for anticancer therapeutics. Nat Rev Cancer 2007; 7:684 - 700
- Albertson DG, Ylstra B, Segraves R, Collins C, Dairkee SH, Kowbel D, et al. Quantitative mapping of amplicon structure by array CGH identifies CYP24 as a candidate oncogene. Nat Genet 2000; 25:144 - 146
- Mimori K, Tanaka Y, Yoshinaga K, Masuda T, Yamashita K, Okamoto M, et al. Clinical significance of the overexpression of the candidate oncogene CYP24 in esophageal cancer. Ann Oncol 2004; 15:236 - 241
- Bareis P, Bises G, Bischof MG, Cross HS, Peterlik M. 25-hydroxy-vitamin d metabolism in human colon cancer cells during tumor progression. Biochem Biophys Res Commun 2001; 285:1012 - 1017
- Luo W, Karpf AR, Deeb KK, Muindi JR, Morrison CD, Johnson CS, et al. Epigenetic regulation of vitamin D 24-hydroxylase/CYP24A1 in human prostate cancer. Cancer Res 2010; 70:5953 - 5962
- Novakovic B, Sibson M, Ng HK, Manuelpillai U, Rakyan V, Down T, et al. Placenta-specific methylation of the vitamin D 24-hydroxylase gene: implications for feedback autoregulation of active vitamin D levels at the fetomaternal interface. J Biol Chem 2009; 284:14838 - 14848
- Khorchide M, Lechner D, Cross HS. Epigenetic regulation of vitamin D hydroxylase expression and activity in normal and malignant human prostate cells. J Steroid Biochem Mol Biol 2005; 93:167 - 172
- Carmeliet P. Angiogenesis in life, disease and medicine. Nature 2005; 438:932 - 936
- Li Y, Cozzi PJ. Angiogenesis as a strategic target for prostate cancer therapy. Med Res Rev 30:23 - 66
- Baluk P, Hashizume H, McDonald DM. Cellular abnormalities of blood vessels as targets in cancer. Curr Opin Genet Dev 2005; 15:102 - 111
- St. Croix B, Rago C, Velculescu V, Traverso G, Romans KE, Montgomery E, et al. Genes expressed in human tumor endothelium. Science 2000; 289:1197 - 1202
- Akino T, Hida K, Hida Y, Tsuchiya K, Freedman D, Muraki C, et al. Cytogenetic abnormalities of tumor-associated endothelial cells in human malignant tumors. Am J Pathol 2009; 175:2657 - 2667
- Hashizume H, Baluk P, Morikawa S, McLean JW, Thurston G, Roberge S, et al. Openings between defective endothelial cells explain tumor vessel leakiness. Am J Pathol 2000; 156:1363 - 1380
- Yan MS, Matouk CC, Marsden PA. Epigenetics of the vascular endothelium. J Appl Physiol 2010; 109:916 - 926
- Hellebrekers DM, Castermans K, Vire E, Dings RP, Hoebers NT, Mayo KH, et al. Epigenetic regulation of tumor endothelial cell anergy: silencing of intercellular adhesion molecule-1 by histone modifications. Cancer Res 2006; 66:10770 - 10777
- Fish JE, Marsden PA. Endothelial nitric oxide synthase: insight into cell-specific gene regulation in the vascular endothelium. Cell Mol Life Sci 2006; 63:144 - 162
- Zhu X, Leav I, Leung YK, Wu M, Liu Q, Gao Y, et al. Dynamic regulation of estrogen receptor-beta expression by DNA methylation during prostate cancer development and metastasis. Am J Pathol 2004; 164:2003 - 2012
- Grover AC, Tangrea MA, Woodson KG, Wallis BS, Hanson JC, Chuaqui RF, et al. Tumor-associated endothelial cells display GSTP1 and RARbeta2 promoter methylation in human prostate cancer. J Transl Med 2006; 4:13
- Chung I, Wong MK, Flynn G, Yu WD, Johnson CS, Trump DL. Differential antiproliferative effects of calcitriol on tumor-derived and matrigel-derived endothelial cells. Cancer Res 2006; 66:8565 - 8573
- Chung I, Karpf AR, Muindi JR, Conroy JM, Nowak NJ, Johnson CS, et al. Epigenetic silencing of CYP24 in tumor-derived endothelial cells contributes to selective growth inhibition by calcitriol. J Biol Chem 2007; 282:8704 - 8714
- Espina V, Wulfkuhle JD, Calvert VS, VanMeter A, Zhou W, Coukos G, et al. Laser-capture microdissection. Nat Protoc 2006; 1:586 - 603
- Eberle FC, Hanson JC, Willian JK, Wei L, Ylaya K, Hewitt SM, et al. Immunoguided laser assisted microdissection techniques for DNA methylation analysis of archival tissue specimens. J Mol Diagn 12:394 - 401
- Kinnecom K, Pachter JS. Selective capture of endothelial and perivascular cells from brain microvessels using laser capture microdissection. Brain Res Brain Res Protoc 2005; 16:1 - 9
- Buckanovich RJ, Sasaroli D, O'Brien-Jenkins A, Botbyl J, Conejo-Garcia JR, Benencia F, et al. Use of immuno-LCM to identify the in situ expression profile of cellular constituents of the tumor microenvironment. Cancer Biol Ther 2006; 5:626 - 633
- Hanson JA, Gillespie JW, Grover A, Tangrea MA, Chuaqui RF, Emmert-Buck MR, et al. Gene promoter methylation in prostate tumor-associated stromal cells. J Natl Cancer Inst 2006; 98:255 - 261
- Kohda Y, Murakami H, Moe OW, Star RA. Analysis of segmental renal gene expression by laser capture microdissection. Kidney Int 2000; 57:321 - 331
- Hu M, Yao J, Cai L, Bachman KE, van den Brule F, Velculescu V, et al. Distinct epigenetic changes in the stromal cells of breast cancers. Nat Genet 2005; 37:899 - 905
- Rodriguez-Canales J, Hanson JC, Tangrea MA, Erickson HS, Albert PS, Wallis BS, et al. Identification of a unique epigenetic sub-microenvironment in prostate cancer. J Pathol 2007; 211:410 - 419
- Hellebrekers DM, Melotte V, Vire E, Langenkamp E, Molema G, Fuks F, et al. Identification of epigenetically silenced genes in tumor endothelial cells. Cancer Res 2007; 67:4138 - 4148
- Seo J, Kim YO, Jo I. Differential expression of stromal cell-derived factor 1 in human brain microvascular endothelial cells and pericytes involves histone modifications. Biochem Biophys Res Commun 2009; 382:519 - 524
- Hodge DR, Peng B, Cherry JC, Hurt EM, Fox SD, Kelley JA, et al. Interleukin 6 supports the maintenance of p53 tumor suppressor gene promoter methylation. Cancer Res 2005; 65:4673 - 4682
- Bao BY, Yao J, Lee YF. 1alpha,25-dihydroxyvitamin D3 suppresses interleukin-8-mediated prostate cancer cell angiogenesis. Carcinogenesis 2006; 27:1883 - 1893
- Fradette C, Du Souich P. Effect of hypoxia on cytochrome P450 activity and expression. Curr Drug Metab 2004; 5:257 - 271
- Kovacs CS, Kronenberg HM. Maternal-fetal calcium and bone metabolism during pregnancy, puerperium and lactation. Endocr Rev 1997; 18:832 - 872
- Delvin EE, Arabian A, Glorieux FH, Mamer OA. In vitro metabolism of 25-hydroxycholecalciferol by isolated cells from human decidua. J Clin Endocrinol Metab 1985; 60:880 - 885
- Giovannucci E, Liu Y, Rimm EB, Hollis BW, Fuchs CS, Stampfer MJ, et al. Prospective study of predictors of vitamin D status and cancer incidence and mortality in men. J Natl Cancer Inst 2006; 98:451 - 459
- Morrison C, Cheney R, Johnson CS, Smith G, Mohler JL. Central quadrant procurement of radical prostatectomy specimens. Prostate 2009; 69:770 - 773