Abstract
Loss-of-function mutations in the methyl-DNA binding protein MeCP2 are associated with neurological dysfunction and impaired neural plasticity. However, the transcriptional changes that underlie these deficits remain poorly understood. Here, we show that mice bearing a C-terminal truncating mutation in Mecp2 (Mecp2308) are hypersensitive to the locomotor stimulating effects of cocaine. Furthermore, these mice have gene-specific alterations in striatal immediate-early gene (IEG) induction following cocaine administration. MeCP2 mutant mice show normal levels of baseline and cocaine-induced striatal Fos expression compared with their wild-type littermates. However, the mutant mice have enhanced cocaine-induced transcription of Junb and Arc. At the chromatin level, we find increased histone H3 acetylation at gene promoters in the Mecp2 mutant mice compared with their wild-type littermates, whereas two sites of repressive histone methylation are unchanged. Interestingly, we find that MeCP2 mutant mice show increased steady-state association of elongation-competent RNA Polymerase II (RNAP II) with the Junb and Arc promoters, whereas levels of RNAP II association at the Fos promoter are unchanged. These data reveal a gene-specific effect of MeCP2 on the recruitment of RNAP II to gene promoters that may modulate the inducibility of IEGs. In addition, our findings raise the possibility that aberrant regulation of IEGs including Junb and Arc may contribute to altered cocaine-induced neuronal and behavioral plasticity in Mecp2 mutant mice.
Introduction
In mammalian cells, genomic DNA is extensively modified by the addition of methyl-groups, predominantly at cytosine residues in CpG dinucleotides.Citation1 DNA methylation impacts transcription by inhibiting or recruiting the association of DNA binding proteins to methylated regions of the genome.Citation2 Methyl-CpG binding protein 2 (MeCP2) is a methyl-DNA binding protein of particular interest in the CNS because loss-of-function mutations in human MECP2 cause the neurodevelopmental disorder Rett Syndrome (RTT).Citation3,Citation4 In addition to its roles during brain development, conditional deletion of Mecp2 in the brains of adult mice causes neurological dysfunction indicating that MeCP2 has essential maintenance functions in mature neurons.Citation5,Citation6 Several lines of evidence suggest that MeCP2 modulates both synapse development and function.Citation7–Citation13 However, the MeCP2-dependent transcriptional changes that underlie the neuronal and synaptic abnormalities observed in MeCP2 mutant mice remain to be fully defined.
We and others have shown that MeCP2 expression in the adult brain is important for modulating the neural and behavioral plasticities induced by psychostimulant drugs of abuse.Citation7,Citation14 We have studied the effects of amphetamine administration in a transgenic strain of mice that express a C-terminally truncated form of MeCP2 (Mecp2308).Citation15,Citation16 This truncation is thought to be a partial loss-of-function mutation that mimics MECP2 mutations associated with less severe variants of RTT.Citation15,Citation16 Mecp2308/Y mutant mice (MUT) are hypersensitive to the locomotor stimulating effects of acute amphetamine compared with their Mecp2+/Y wild-type (WT) littermates.Citation7,Citation17 Furthermore, they fail to show behavioral sensitization or conditioned place preference upon repeated amphetamine administration.Citation7
The psychostimulants amphetamine and cocaine drive long-lasting changes in the function of brain reward circuits by inducing the transcription of new gene products.Citation18,Citation19 Psychostimulant-regulated immediate-early genes (IEGs) include both transcription factors of the Fos/Jun/Egr families as well neuronally-enriched genes such as Arc, Bdnf and Cdk5 that play direct roles in the plasticity of synaptic strength and structure.Citation19–Citation22 We have shown that amphetamine increases expression of Fos/Jun family transcription factors in the striatum of both WT and MUT mice of the Mecp2308 strain, demonstrating that expression of wild-type MeCP2 is not required for the IEG response.Citation7 However, both the magnitude of FosB and JunB induction following a single dose of amphetamine and the plasticity of Fos, FosB and JunB inducibility following repeated amphetamine is significantly altered in Mecp2 MUT mice. Nonetheless, it was unknown whether changes in transcription underlie these changes in Fos/Jun protein expression, whether synaptic IEGs are also affected, and whether IEG dysregulation in Mecp2 MUT mice also occurs in response to other drugs of abuse such as cocaine.
Because MeCP2 is known to associate with a number of chromatin modifying enzymes,Citation23–Citation25 we hypothesized that mutation of MeCP2 may modulate the inducibility of IEGs by altering chromatin-based regulation of these genes. Here, we have investigated this possibility by comparing cocaine-induced transcriptional regulation and chromatin modifications at IEG promoters in the striatum of Mecp2 WT and MUT mice. Our data show that Mecp2308 MUT mice have increased RNA polymerase II (RNAP II) recruitment at the Junb and Arc promoters that correlates with enhanced cocaine-inducibility of these genes in the striatum. These data support a role for MeCP2 as a modulator of stimulus-regulated gene expression, and raise the possibility that impairments in the fidelity of inducible IEG transcription may contribute to neural plasticity deficits in the absence of MeCP2.
Results
Mutation of MeCP2 enhances cocaine-inducibility of Junb and Arc but not Fos.
The psychostimulants amphetamine and cocaine have both overlapping and distinct mechanisms of action. Importantly both of these drugs increase extracellular dopamine levels in the striatum and both are known to induce striatal IEG expression.Citation7,Citation19,Citation26 To determine whether the Mecp2308 mutation affects neural processes that are common to the actions of both amphetamine and cocaine, we first monitored the locomotor response of Mecp2 WT and MUT mice to administration of a single dose of 20 mg/kg cocaine (i.p.) in the open field (). A repeated measures ANOVA revealed a main effect of genotype (F1,27 = 4.526, p = 0.043) and treatment (F1,27 = 117.811, p < 0.001), and a significant genotype times treatment interaction (F1,27 = 9.478, p = 0.005). Both WT and MUT mice showed a significant increase in locomotor activity following cocaine administration. However, the locomotor response of the MUT mice was significantly greater than that of their WT littermates. The magnitude and time course of this hypersensitive locomotor response to cocaine is similar to that seen following administration of amphetamine.Citation7 These data indicate that MeCP2-dependent processes in the brain are important for the behavioral effects of both amphetamine and cocaine.
To determine whether mutation of MeCP2 affects cocaine-induced changes in IEG transcription, we used quantitative PCR to measure the expression of mRNAs in striatal tissue of Mecp2 WT and MUT mice before or 1 h after cocaine administration. Consistent with previous studies in reference Citation27–Citation29, we found that levels of Fos, Junb, and Arc mRNA were significantly higher in the striatum of Mecp2 WT mice 1 h after cocaine administration compared with basal levels of these RNAs (). Cocaine also induced expression of Fos, Junb and Arc mRNA in the striatum of Mecp2 MUT mice, confirming that wild-type MeCP2 function is not required for this IEG response. Levels of Fos, Junb and Arc mRNAs were not different between WT and MUT mice under control conditions. Furthermore, Fos expression was induced by cocaine to equivalent levels in both WT and MUT mice (). These data mirror our observations of normal acute amphetamine-regulated Fos protein induction in Mecp2 WT and MUT mice,Citation7 and suggest that acute induction of Fos transcription is not sensitive to the Mecp2308 mutation. By contrast, cocaine-induced levels of Junb were significantly enhanced in Mecp2 MUT mice compared with their WT littermates (). This superinduction mirrors the enhanced expression of JunB protein we observed in Mecp2 MUT mice in response to acute amphetamine administration,Citation7 suggesting a role for MeCP2 in the regulation of Junb inducibility. We also found a significant enhancement of the cocaine-induced levels of Arc mRNA in the Mecp2 MUT mice compared with their WT littermates (). Our evidence of enhanced Arc expression is particularly intriguing because this is the first evidence that stimulus-inducible transcription of an IEG with synaptic functions is dysregulated in the brains of Mecp2308 mutant mice. Finally, as a control we measured expression of the constitutively expressed gene Tubb5, which encodes a neuronal β-tubulin subunit. Tubb5 expression did not change following cocaine administration and was not different between Mecp2 WT and MUT mice (). Taken together these data suggest that loss of Mecp2 modulates psychostimulant-inducible transcription of a subset of IEGs.
Enhanced promoter histone H3 acetylation in MeCP2 mutant mice.
The transcription of IEGs is very rapidly induced upon neuronal stimulation via the posttranslational modification of transcription factors pre-bound to IEG promoters.Citation30 Given the evidence that IEG promoters maintain a permissive chromatin state under both basal and stimulus-induced conditions,Citation31 we hypothesized that the differential inducibility of IEGs following cocaine administration might reflect a different basal state of chromatin at these gene promoters in MUT mice prior to cocaine administration. To assess steady-state histone modifications at IEG promoters we used chromatin immunoprecipitation (ChIP) to quantify levels of histone H3 acetylation (at K9 and K14) and two sites of repressive histone methylation (H3K9me2 and H3K27me3) at gene promoters in striatal lysates from Mecp2 WT and MUT mice.
As controls for our ChIP studies of IEGs, we assessed histone modifications at the constitutively active promoter of Tubb5 and the silenced promoter for the β-globin gene Hbb-bh1, RNA for which is undetectable in the striatum. Relative expression levels of all genes analyzed in this study are shown in . We found comparatively high levels of histone H3 acetylation (H3Ac) on the Tubb5 promoter and comparatively low levels on the promoter of Hbb-bh1 (). These data reflect the positive correlation between promoter histone acetylation and gene expression that has been found in genome-scale studies.Citation32
Levels of H3Ac at the Fos, Junb and Arc promoters approach those at the Tubb5 promoter (). These data show that although productive transcription of IEGs is stimulus-dependent, their promoters maintain a permissive chromatin state at baseline.Citation31 When we compared histone H3 acetylation between Mecp2 WT and MUT mice at each promoter, we found a trend toward an increase in promoter acetylation in the Mecp2 MUT mice that reached statistical significance at the Arc and Tubb5 promoters. These data are similar in magnitude to the global hyperacetylation that has been found by western blot analysis of lysates from brain tissue of Mecp2308 MUT mice and by ChIP in Mecp2 null strains.Citation15,Citation33 Taken together, these observations suggest that loss-of-function mutations in MeCP2 enhance steady-state levels of histone acetylation across the genome including certain IEG promoter regions.
We next examined two histone methylation modifications—H3K27me3 and H3K9me2. These histone modifications are associated with gene repressionCitation32 and we found high levels of both modifications at the promoter for the silenced gene Hbb-bh1 and low levels at the promoter for the constitutively expressed gene Tubb5 ( and C). Intermediate levels of H3K27me3 (), and very low levels of H3K9me2 were found at the Fos, Junb and Arc promoters (). When we compared histone methylation levels between WT and MUT mice we found no significant differences in these modifications at any of the gene promoters studied. Thus, the C-terminal truncation of MeCP2 in the Mecp2308/Y mice does not appear to alter levels of repressive histone methylation at these gene promoters.
Enhanced RNAP II association with the Junb and Arc promoters in Mecp2 MUT mice.
Chromatin state is translated into changes in gene expression by affecting the recruitment and activity of RNA polymerase. To determine whether mutation of MeCP2 has an effect on RNAP II recruitment at IEG promoters, we performed ChIP using an antibody against the large subunit of RNAP II (RPB1). RNAP II was found at the Fos, Junb and Arc promoters in both Mecp2 WT and MUT mice at levels similar to that seen at the constitutively active gene Tubb5 (). RNAP II was not detectable at the promoter of the silenced gene Hbb-bh1 (data not shown). When we compared the levels of RNAP II at these promoters between Mecp2 WT and MUT mice we found a significant increase in RNAP II bound to the Junb and Arc promoters in the MUT mice compared with their WT littermates. By contrast, levels of RNAP II at the Fos promoter were not different between genotypes. These data show that mutation of MeCP2 is associated with gene-specific differences in RNAP II recruitment.
RNAP II can exist in at least three activation states at gene promoters—preinitiation, stalled and elongation-competent.Citation34 Following recruitment to gene promoters, sequential phosphorylation of two Ser residues in the heptapeptide repeats of the C-terminal domain of the RBP1 subunit of RNAP II is required first for initiation (pSer5) and then elongation (pSer2) of transcription. Many IEGs have inactive, non-phosphorylated RNAP II pre-initiation complexes bound to their promoters under baseline conditions, which is thought to prime these promoters to rapidly initiate transcription following reception of an appropriate stimulus.Citation31,Citation35,Citation36 In addition, very rapidly responsive IEG promoters, including those of Fos, Junb and Arc, are bound under baseline conditions by Ser5 phosphorylated RNAP II that initiates transcription but then stalls and remains paused just after the transcription start site.Citation35 Phosphorylation of pSer2 on the stalled RNAP II relieves the elongation block and pausing is required for the most rapid phase of IEG transcription.Citation35 In the absence of Ser2 phosphorylation, Ser5 phosphorylated RNAP II drives unproductive transcription.Citation31,Citation37
To determine the activation state of RNAP II at IEG promoters we performed ChIP with antibodies selective for RPB1 phosphorylated at either Ser5 or Ser2. Both phosphorylated forms of RPB1 were detected at Fos, Junb and Arc. Comparing the levels of Ser5 and Ser2 phosphorylated forms of RPB1 between Mecp2 WT and MUT mice revealed that on the Junb and Arc promoters there was a significant increase in the phosphorylated forms in MUT mice relative to WT, and that both pSer5 and pSer2 levels were increased in MUT relative to WT ( and C). By contrast, there was no difference in the phosphorylation state of RNAP II at the Fos promoter between genotypes.
Genes that have stalled RNAP II show an elevated pSer5:pSer2 ratio compared with genes that have only the unphosphorylated pre-initiation or doubly phosphorylated elongating forms.Citation31 We found pSer5:pSer2 ratios greater than 1 at the Fos, Junb and Arc promoters (), consistent with the evidence from other studies that these genes have stalled PolII at their gene promoters.Citation35 However, the pSer5:pSer2 ratio at these IEG promoters was not different between Mecp2 MUT mice and their WT littermates suggesting that mutation of MeCP2 does not alter the regulation of RNAP II stalling.
Finally, because we found significant increases in all three forms of RNAP II (total, pSer5 and pSer2) on the Junb and Arc promoters in Mecp2 MUT mice, we asked whether this reflected a change in the relative levels of the pre-initiation (unphosphorylated) or elongation-competent (pSer2 phosphorylated) form of RNAP II. We found that the pSer2/total RNAP II ratios were not significantly different between Mecp2 WT and MUT mice at IEG promoters (). These data indicate that a similar fraction of the RNAP II found at the Junb and Arc promoters in both WT and MUT mice is in the dually phosphorylated form. Because there is significantly more total RNAP II on the Junb and Arc promoters in the Mecp2 MUT mice, these data imply that there is also more elongation-competent RNAP II at these genes. This gene-specific increase in RNAP II recruitment may underlie the enhanced inducibility of Junb and Arc we observe following cocaine administration.
Discussion
The plasticity of an animal's behavioral repertoire depends in large part on its ability to convert environmental stimuli into changes in neuronal function. One significant way that neurons accomplish this task is through the stimulus-regulated modulation of gene transcription. A growing body of evidence suggests that activity-dependent changes in both DNA methylation and histone modifications play a causative role in this process, and reveal that chromatin regulation is far more dynamic than once believed. We have used MeCP2 mutant mice as a means to investigate the relationship between DNA methylation-dependent gene transcription and the neural and behavioral plasticity induced by psychostimulant drugs of abuse. In this study, we used RNA expression analysis and ChIP to reveal gene-specific changes in the transcriptional regulation of IEGs in the striatum of Mecp2308 MUT mice. These data show that loss of MeCP2 function is associated with cocaine-inducible IEG transcription that is normal (Fos) or enhanced (Junb and Arc). An important finding of this study is that we observe an increase in RNAP II binding at the Junb and Arc promoters in Mecp2 MUT mice suggesting a mechanism that may underlie the enhanced cocaine-inducibility of these IEGs.
In neurons, the expression level of MeCP2 approaches that of the histones, and the overall distribution of MeCP2 binding across the genome closely parallels that of methylated DNA, which is very broadly distributed.Citation33,Citation38–Citation40 In this context, it is interesting to consider what mechanisms may explain our observation of distinct, gene-specific effects on the inducibility of Fos, Junb and Arc in Mecp2 MUT mice. Despite the relative lack of DNA methylation at actively transcribed, CpG rich gene promoters like those of the IEGs, it is possible that MeCP2 has specific functions at certain subsets of gene promoters. ChIP-PCR studies have indicated that MeCP2 is bound to the sparsely-methylated Junb promoter in neurons,Citation41 whereas at least one studyCitation42 directly tested but failed to find a significant association of MeCP2 with the Fos promoter. ChIP-Seq data reveal a more complex picture, with relatively uniform and similar binding profiles of MeCP2 distributed across the proximal promoters of the Fos, Junb and Arc genes.Citation40 Interestingly the functional consequences of MeCP2 for transcription of specific gene products has been suggested to correlate with the CpG content of gene promoters, such that genes with CpG-rich promoters were found to be enriched among the set of genes whose expression is reduced by loss-of-function mutations in MeCP2.Citation43 Fos, Junb and Arc all have CpG islands overlapping their proximal promoters making CpG content alone insufficient to explain the differential regulation of these IEGs in the Mecp2 mutant mice. However, other sequence features, such as the relative methylation of CpGs within these promoters, may contribute to the differential ways in which the association of MeCP2 with these promoter regions impacts transcription.
Alternatively, the consequences of loss of MeCP2 function for specific target genes may depend on regional MeCP2-dependent effects on the local secondary and/or tertiary structure of chromatin.Citation24,Citation44,Citation45 This model would offer a potential explanation for why genetic manipulations of Mecp2 have been associated with subtle increases and decreases in expression of a large number of coding and non-coding RNA transcripts.Citation43,Citation46 The rapid neuronal activity-dependent induction of IEGs has been shown to be associated with stimulus-dependent recruitment of transcriptional coactivators and RNAP II not only to IEG promoters but also to more distant enhancer elements that neighbor IEGs.Citation36 If long-distance chromatin interactions are important for induction of select IEGs, then chromatin changes outside of promoters in Mecp2 MUT mice that influence enhancer activation or that affect the formation of tertiary chromatin loops could have a significant impact on transcriptional induction of these genes. For example, DNA methylation and MeCP2 binding at or around enhancer elements may serve to modulate their functional relevance for IEG transcription. Importantly, the brains of Mecp2 null mice have been shown to lack a known long-distance interaction between a silencing element and the Dlx5 gene promoter, consistent with a potential role for MeCP2 in chromatin looping.Citation45
Brains of Mecp2 null mice show enhanced transcription of repetitive genomic elements and increased L1 retrotransposition, both of which are subject to DNA methylation-dependent repression.Citation33,Citation49 MeCP2 can be coimmunoprecipitated from neurons with mSin3A and the histone deacetylase HDAC1, suggesting that MeCP2 may repress transcription by deacetylating histones.Citation25,Citation47,Citation48 The functional importance of MeCP2 as a regulator of histone acetylation in vivo was first demonstrated by the observation of elevated histone H3Ac levels in nuclear extracts from the brain of mutant mice of the Mecp2308 strain.Citation15 The fact that this enhanced histone H3 acetylation could be observed in a tissue extract suggested that loss of MeCP2 resulted in genomewide rather than gene-specific increases in histone acetylation. Further support for this model came from the results of a histone H3Ac ChIP study that examined 100 loci and covered over 90 kb of genomic DNA from brains of Mecp2 null mice.Citation33 These results showed a general increase of about 2-fold in the level of H3Ac across the genome that was greatest in regions of the genome where MeCP2 binding is highest. Consistent with these data, we find a general trend toward elevated histone acetylation at the gene promoters tested in this study that reaches significance at the Arc and Tubb5 promoters. However, we note that enhanced promoter histone acetylation alone is not sufficient to cause increased gene expression as exemplified by the unchanged expression of Tubb5 in the striatum of Mecp2 MUT mice ().
In addition to its association with histone deacetylases, MeCP2 has also been shown to co-immunoprecipitate with a histone H3K9 methyltransferase.Citation23 A previous study reported no difference between Mecp2 null and WT brains in global levels of three repressive histone methylation marks as assessed by western blotting of nuclear extracts.Citation50 We took a more focused approach by quantifying levels of two repressive sites of histone methylation (H3K9me2 and H3K27me3) at IEG promoters. We found no significant differences in the levels of these modifications at the gene promoters studied here. Our findings do not rule out a function for MeCP2 in the regulation of other sites of histone methylation or in regulation of these histone methylation events elsewhere in the genome. However, our data do indicate that changes in the levels of repressive promoter histone methylation are unlikely to explain the alterations in IEG inducibility we observe in the Mecp2308 MUT mice.
Changes in chromatin due to loss of MeCP2 may influence RNAP II recruitment. It is a novel finding of this study that we observe increased association of RNAP II with the promoters of the Junb and Arc genes in Mecp2 MUT mice compared with their WT littermates. By contrast WT and MUT mice showed similar levels of RNAP II at the Fos promoter demonstrating that this effect is gene-specific. The correlation between increased RNAP II recruitment and enhanced cocaine-inducibility of the Junb and Arc genes suggests that having more RNAP II at these promoters may sensitize these genes to induction by a subsequent stimulus. It is interesting that we observe enhanced binding of pSer5/pSer2 elongation-competent RNAP II at these promoters prior to cocaine administration even though expression of Junb and Arc do not differ between WT and MUT mice in the striatum under these basal conditions. This observation highlights the complexity of transcriptional regulation and suggests that additional regulatory events beyond RNAP II recruitment and Ser5/Ser2 phosphorylation at gene promoters are required for the activation of productive transcription.
Importantly, our data suggest that impaired fidelity of the IEG response may contribute to the lack of psychostimulant-induced behavioral and neural plasticity we have observed in the Mecp2 MUT mice.Citation7 Specifically, we have shown that Mecp2 MUT mice have impaired structural plasticity of dendritic spines on striatal medium spiny neurons (MSNs). MSN dendritic spine density is not different between Mecp2 WT and MUT mice under basal conditions; however, the Mecp2 MUT mice fail to show the increase in MSN spine density seen in their WT littermates following repeated amphetamine exposure. Psychostimulantdependent MSN structural synapse plasticity is driven at least in part by the induced transcription of genes whose products function at synapses, which include the actin binding protein Arc.Citation51 Arc regulates the endocytosis of AMPA-type glutamate receptors and is required for long-lasting, activity-dependent changes in synaptic strength.Citation52,Citation53 Little is known about the role of Arc in structural synapse plasticity; however, both overexpression and knockout of Arc in the hippocampus induce significant changes in dendritic spine morphology.Citation54 Future studies that address the role of Arc in MSN structural synaptic plasticity may shed new light on the mechanisms that contribute to abnormal synapse development and plasticity in the absence of normal MeCP2 function.
Materials and Methods
Animals and drug treatments.
Mecp2308/Y mice (B6.129S-Mecp2tm1Hzo/J, stock number 005439) were purchased from Jackson laboratories (Bar Harbor, ME). This strain is congenic on a C57BL/6J background. Heterozygous Mecp2308/+ female mice in our breeding colony were crossed to C57BL/6J male mice (Jackson Laboratories) to generate WT (Mecp2+/Y) and MUT (Mecp2308/Y) male littermates. Adult (8–12 week old) male MUT and WT littermates were used in all experiments. Cocaine-HCl was purchased from Sigma (St. Louis, MO) and dissolved in saline. All injections were given i.p. and a dose of 20 mg/kg cocaine was used in all experiments. Mice were moved to the procedure room and weighed the day before the experiment. Mice were habituated to the open field (Accuscan Instruments, Columbus, OH) for 1 h to establish baseline locomotor activity before cocaine administration. Following injection, mice were immediately returned to the open field and the stimulation of locomotor activity was recorded for 1 h (horizontal distance in cm) under 340 lx illumination before tissue harvesting to ensure all animals used for biochemistry responded to the drug administered. In some experiments, as indicated in the text, mice were taken directly from the home cage for tissue harvesting. All animal procedures were performed under an approved protocol from the Duke University Institutional Animal Care and Use Committee.
RNA expression analyses.
Mice were deeply anesthetized with isofluorane, brains were removed, and a region of the ventral striatum containing the nucleus accumbens shell was rapidly dissected in 10 mM pH 7.4 HEPES-buffered Hank's Basic Salt Solution (Gibco/Invitrogen, Carlsbad, CA) on ice. Dissected tissue was snap frozen in liquid nitrogen and stored at −80° prior to use. Tissue was thawed on ice and homogenized in lysis buffer using a motorized Kontes pestle. Total RNA was extracted using the Absolutely RNA kit (Stratagene/Agilent, Santa Clara, CA), which contains an on-column DNase treatment step to eliminate genomic DNA contamination. Five hundred nanogram total RNA per sample was used for reverse transcription with Superscript II (Invitrogen), using random hexamer based priming. Quantitative PCR was run on an ABI 7300 PCR machine (Applied Biosystems, Foster City, CA) using gene-specific primers and Power SYBR Green master mix (Applied Biosystems). Each sample was run in triplicate and relative expression levels of each gene were quantified against a standard curve for each primer pair. Standard curve slopes were calculated for all primer pairs to validate their efficiency for use in ΔCt analysis, which was employed to compare expression levels between different gene products. Unique PCR product generation was confirmed by analyzing melting curves for a single dissociation peak. All samples were normalized to expression of the housekeeping gene Gapdh to control for sample preparation and handling. All primer pairs for mRNA expression were designed spanning an intron to eliminate any signal from genomic DNA. A complete list of PCR primers used for gene expression in this study is found in .
Chromatin immunoprecipitation (ChIP).
ChIP was performed essentially as described in reference Citation55, with minor modifications. Following isofluorane anesthesia and decapitation of mice the striatum was rapidly dissected from each hemisphere, then the tissue was snap frozen in liquid nitrogen and stored at −80° prior to use. To limit the effects of animal-to-animal variation on our data sets, for each set of ChIP experiments, striata from 3–4 mice were pooled. Thawed tissue was homogenized with 90 strokes in a 2 mL Dounce homogenizer in phosphate buffered saline (PBS) containing 1% formaldehyde (Sigma) to crosslink DNA with DNA binding proteins. After 10 min the formaldehyde was quenched by the addition of 200 mM glycine. Chromatin was sonicated to an average size of 500–1,000 bp in a Misonix 6000 (Farmingdale, NY) for 5 pulses of 15 sec at a power of 27 W. The sample was cleared of insoluble material by spinning at 13,000x g for 10 min. One-tenth of the starting material was set aside for measurement of input DNA. 25 µg of sonicated chromatin was used for each immunoprecipitation with 5 µg of the following antibodies: Anti-Lys9/14 acetylated histone H3 (cat. # 06-599; Millipore, Bilerica, MA), Anti-trimethyl-histone H3 Lys 27 (cat. # 07-449; Millipore), Anti-dimethyl-Histone H3 Lys 9 (cat. # 07-441; Millipore), Anti-RNAP II N-20 (cat. # sc-899; Santa Cruz, Santa Cruz, CA), Anti-RNAP II phospho-Ser2 CTD repeat YpSPTSPS (cat. # Ab5095; Abcam, Cambridge, MA), Anti-RNAP II phosphoSer5 CTD repeat YSPTpSPS (cat. # Ab5131; Abcam), Non-Immune Rabbit IgG (cat. # 12-370; Millipore). Multiple immunoprecipitations with different antibodies were performed from each single pool of chromatin to allow for direct comparisons of distinct DNA binding proteins in a common sample. All immunoprecipitations were performed as technical duplicates within a single experiment, and each ChIP was repeated from at least two independent pooled sets of samples. For statistical calculations the n was defined as the number of independent pools (for all ChIP experiments reported n = 2). Normal Rabbit IgG was used as a negative control and only pulldown values at least 3.3 cycles (∼10-fold) above those seen with IgG were accepted as being above nonspecific background. Immunoprecipitated material was collected using BSA-blocked Protein A Sepharose beads (GE, Uppsala Sweden), and repeated washing was used to remove nonspecific binding. Chromatin was eluted from the beads in SDS and crosslinks were reversed by incubating at 65°C overnight followed by treatment with proteinase K. DNA was purified by phenol/chloroform extraction and precipitation or by using a Qiaquick spin kit (Qiagen, Valencia, CA) and measured by quantitative PCR as described above. Immunoprecipitated quantities were calculated as percent of input and scaled for presentation relative to the pulldown levels on Tubb5 or Hbb-bh1 as described in the text. Primers were designed to amplify regions near the transcription start site of each gene and are listed in .
Statistical analyses.
For the locomotor data statistical significance was determined by ANOVA followed by Bonferroni post-hoc comparisons using SPSS v11.0 software (SPSS, Chicago, IL). For the gene expression and ChIP data significance was determined by conducting Student's unpaired two-tailed t-tests. In all cases p < 0.05 was considered significant. Data are shown as the mean ± SEM.
Disclosure of Potential Conflicts of Interest
No potential conflicts of interest were disclosed.
Figures and Tables
Figure 1 Mecp2 mutant mice are hypersensitive to the locomotor stimulating effects of cocaine. (A) Open field horizontal locomotor activity in Mecp2 WT and MUT mice. Mice were acclimated to the chamber then administered vehicle (saline) or 20 mg/kg cocaine (i.p.) after 60 min (black arrow). Horizontal locomotor activity was monitored for an additional 60 min. Lines show mean and error bars show SEM n = 9 WT vehicle, 5 MUT vehicle, 9 WT cocaine and 8 MUT cocaine. *p < 0.05 MUT cocaine vs. WT cocaine. (B) Quantification of cumulative locomotor activity in the 30 min following vehicle or cocaine administration. §p < 0.05 MUT vehicle vs. WT vehicle, *p < 0.05 WT cocaine vs. WT vehicle, #p < 0.05 MUT cocaine vs. WT cocaine, ^p < 0.05 MUT cocaine vs. MUT vehicle.
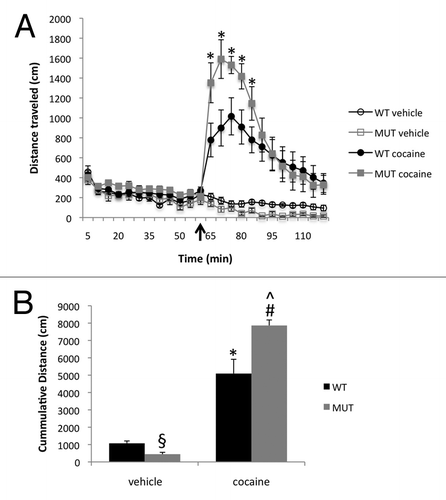
Figure 2 Enhanced cocaine-inducibility of Junb and Arc but not Fos following cocaine administration in Mecp2 mutant mice. Quantitative PC R was run on striatal lysates made from WT and MUT mice before or 1 h after administration of 20 mg/kg cocaine (i.p.). mRNA expression normalized to expression of the housekeeping gene Gapdh was scaled to basal levels in WT mice and presented as fold change. (A) Fos expression is induced by cocaine but does not differ between Mecp2 WT and MUT mice. (B) Junb expression is induced by cocaine and shows significantly greater induction by cocaine in Mecp2 MUT mice compared with WT. (C) Arc expression is induced by cocaine and shows significantly greater expression induction by cocaine in Mecp2 MUT mice compared with WT. (D) Tubb5 expression is not changed by cocaine administration and does not differ between WT and MUT mice. n = 6–8/group, *p < 0.05 WT cocaine vs. WT control, #p < 0.05 MUT cocaine vs. MUT control, ^p < 0.05 MUT cocaine vs. WT cocaine.
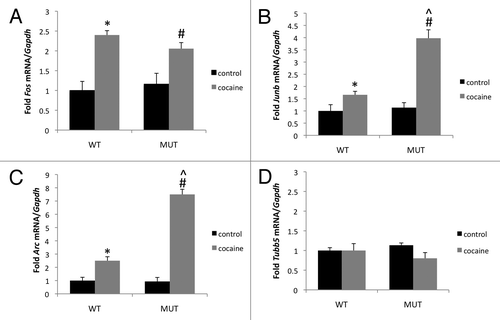
Figure 3 Enhanced histone H3 acetylation but no change in two sites of repressive histone H3 methylation at gene promoters in Mecp2 mutant mice. (A) ChIP was performed from striatum with an antibody that selectively recognizes histone H3 acetylated at lysine 9 and lysine 14 (H3K9/K14Ac). Pulldown quantities were normalized to the input for each experiment and relative quantities on each promoter were scaled to the constitutively transcribed gene Tubb5. (B) ChIP was performed from striatum with an antibody that selectively recognizes histone H3 trimethylated at lysine 27 (H3K27me3). Pulldown quantities were normalized to the input for each experiment and relative quantities on each promoter were scaled to the constitutively repressed gene Hbb-bh1. (C) ChIP was performed from striatum from an antibody that selectively recognizes histone H3 dimethylated at lysine 9 (H3K9me2). Pulldown quantities were normalized to the input for each experiment and relative quantities on each promoter were scaled to the constitutively repressed gene Hbb-bh1. n = 2 independent pools of 3–4 mice/group, *p < 0.05 MUT vs. WT.
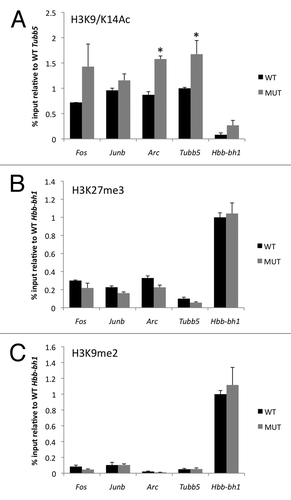
Figure 4 Enhanced association of elongation-competent RNA polymerase II with the Junb and Arc promoters in Mecp2 mutant mice. (A) ChIP was performed from striatum with an antibody that recognizes the large subunit of RNA Polymerase II. Pulldown quantities were normalized to the input for each experiment and relative quantities on each promoter were scaled to the constitutively expressed gene Tubb5. (B and C) ChIP was performed from striatum with antibodies that selectively recognize the Ser5 (B) or Ser2 (C) phosphorylated forms of the large subunit of RNA polymerase II. Pulldown quantities were normalized to the input for each experiment and relative quantities on each promoter are scaled to the constitutively expressed gene Tubb5. (D) No difference in pSer5:pSer2 ratio at IEG promoters in Mecp2 WT and MUT mice. (E) No difference in percentage of total PolII phosphorylated at Ser2 at IEG promoters in Mecp2 WT and MUT mice. n = 2 independent pools of 3–4 mice/group, *p < 0.05 MUT vs. WT.
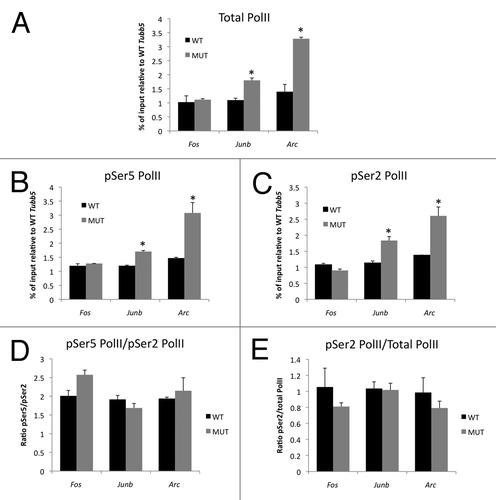
Table 1 Relative gene expression levels
Table 2 Gene expression primers used in this study
Table 3 ChIP primers used in this study
Acknowledgments
We thank A.N. Hutchinson, J.V. Deng and W.C. Wetsel for assistance with the animal experiments. This work was supported by NIH grant R01-DA022202 to A.E.W.
References
- Lister R, Pelizzola M, Dowen RH, Hawkins RD, Hon G, Tonti-Filippini J, et al. Human DNA methylomes at base resolution show widespread epigenomic differences. Nature 2009; 462:315 - 322; PMID: 19829295; http://dx.doi.org/10.1038/nature08514
- Klose RJ, Bird AP. Genomic DNA methylation: the mark and its mediators. Trends Biochem Sci 2006; 31:89 - 97; PMID: 16403636; http://dx.doi.org/10.1016/j.tibs.2005.12.008
- Chahrour M, Zoghbi HY. The story of Rett syndrome: from clinic to neurobiology. Neuron 2007; 56:422 - 437; PMID: 17988628; http://dx.doi.org/10.1016/j.neuron.2007.10.001
- Amir RE, Van den Veyver IB, Wan M, Tran CQ, Francke U, Zoghbi HY. Rett syndrome is caused by mutations in X-linked MECP2, encoding methyl-CpG-binding protein 2. Nat Genet 1999; 23:185 - 188; PMID: 10508514; http://dx.doi.org/10.1038/13810
- Chen RZ, Akbarian S, Tudor M, Jaenisch R. Deficiency of methyl-CpG binding protein-2 in CNS neurons results in a Rett-like phenotype in mice. Nat Genet 2001; 27:327 - 331; PMID: 11242118; http://dx.doi.org/10.1038/85906
- McGraw CM, Samaco RC, Zoghbi HY. Adult neural function requires MeCP2. Science 2011; 333:186; PMID: 21636743; http://dx.doi.org/10.1126/science.1206593
- Deng JV, Rodriguiz RM, Hutchinson AN, Kim IH, Wetsel WC, West AE. MeCP2 in the nucleus accumbens contributes to neural and behavioral responses to psychostimulants. Nat Neurosci 2010; 13:1128 - 1136; PMID: 20711186; http://dx.doi.org/10.1038/nn.2614
- Armstrong DD. Neuropathology of Rett syndrome. J Child Neurol 2005; 20:747 - 753; PMID: 16225830; http://dx.doi.org/10.1177/08830738050200082401
- Medrihan L, Tantalaki E, Aramuni G, Sargsyan V, Dudanova I, Missler M, et al. Early defects of GABAergic synapses in the brain stem of a MeCP2 mouse model of Rett syndrome. J Neurophysiol 2008; 99:112 - 121; PMID: 18032561; http://dx.doi.org/10.1152/jn.00826.2007
- Nelson ED, Kavalali ET, Monteggia LM. MeCP2-dependent transcriptional repression regulates excitatory neurotransmission. Curr Biol 2006; 16:710 - 716; PMID: 16581518; http://dx.doi.org/10.1016/j.cub.2006.02.062
- Dani VS, Chang Q, Maffei A, Turrigiano GG, Jaenisch R, Nelson SB. Reduced cortical activity due to a shift in the balance between excitation and inhibition in a mouse model of Rett syndrome. Proc Natl Acad Sci USA 2005; 102:12560 - 12565; PMID: 16116096; http://dx.doi.org/10.1073/pnas.0506071102
- Chao HT, Zoghbi HY, Rosenmund C. MeCP2 controls excitatory synaptic strength by regulating glutamatergic synapse number. Neuron 2007; 56:58 - 65; PMID: 17920015; http://dx.doi.org/10.1016/j.neuron.2007.08.018
- Tropea D, Giacometti E, Wilson NR, Beard C, McCurry C, Fu DD, et al. Partial reversal of Rett Syndrome-like symptoms in MeCP2 mutant mice. Proc Natl Acad Sci USA 2009; 106:2029 - 2034; PMID: 19208815; http://dx.doi.org/10.1073/pnas.0812394106
- Im HI, Hollander JA, Bali P, Kenny PJ. MeCP2 controls BDNF expression and cocaine intake through homeostatic interactions with microRNA-212. Nat Neurosci 2010; 13:1120 - 1127; PMID: 20711185; http://dx.doi.org/10.1038/nn.2615
- Shahbazian MD, Young JI, Yuva-Paylor LA, Spencer CM, Antalffy BA, Noebels JL, et al. Mice with truncated MeCP2 recapitulate many Rett syndrome features and display hyperacetylation of histone H3. Neuron 2002; 35:243 - 254; PMID: 12160743; http://dx.doi.org/10.1016/S0896-6273(02)00768-7
- Stearns NA, Schaevitz LR, Bowling H, Nag N, Berger UV, Berger-Sweeney J. Behavioral and anatomical abnormalities in Mecp2 mutant mice: a model for Rett syndrome. Neuroscience 2007; 146:907 - 921; PMID: 17383101; http://dx.doi.org/10.1016/j.neuroscience.2007.02.009
- De Filippis B, Ricceri L, Laviola G. Early postnatal behavioral changes in the Mecp2-308 truncation mouse model of Rett syndrome. Genes Brain Behav 2010; 9:213 - 223; PMID: 19958389; http://dx.doi.org/10.1111/j.1601-183X.2009.00551.x
- Kauer JA, Malenka RC. Synaptic plasticity and addiction. Nat Rev Neurosci 2007; 8:844 - 858; PMID: 17948030; http://dx.doi.org/10.1038/nrn2234
- Maze I, Covington HE 3rd, Dietz DM, LaPlant Q, Renthal W, Russo SJ, et al. Essential role of the histone methyltransferase G9a in cocaine-induced plasticity. Science 2010; 327:213 - 216; PMID: 20056891; http://dx.doi.org/10.1126/science.1179438
- Norrholm SD, Bibb JA, Nestler EJ, Ouimet CC, Taylor JR, Greengard P. Cocaine-induced proliferation of dendritic spines in nucleus accumbens is dependent on the activity of cyclin-dependent kinase-5. Neuroscience 2003; 116:19 - 22; PMID: 12535933; http://dx.doi.org/10.1016/S0306-4522(02)00560-2
- Parkitna JR, Bilbao A, Rieker C, Engblom D, Piechota M, Nordheim A, et al. Loss of the serum response factor in the dopamine system leads to hyperactivity. FASEB J 2010; 24:2427 - 2435; PMID: 20223941; http://dx.doi.org/10.1096/fj.09-151423
- Leslie JH, Nedivi E. Activity-regulated genes as mediators of neural circuit plasticity. Prog Neurobiol 2011; 94:223 - 237; PMID: 21601615; http://dx.doi.org/10.1016/j.pneurobio.2011.05.002
- Fuks F, Hurd PJ, Wolf D, Nan X, Bird AP, Kouzarides T. The methyl-CpG-binding protein MeCP2 links DNA methylation to histone methylation. J Biol Chem 2003; 278:4035 - 4040; PMID: 12427740; http://dx.doi.org/10.1074/jbc.M210256200
- Nan X, Hou J, Maclean A, Nasir J, Lafuente MJ, Shu X, et al. Interaction between chromatin proteins MECP2 and ATRX is disrupted by mutations that cause inherited mental retardation. Proc Natl Acad Sci USA 2007; 104:2709 - 2714; PMID: 17296936; http://dx.doi.org/10.1073/pnas.0608056104
- Nan X, Ng HH, Johnson CA, Laherty CD, Turner BM, Eisenman RN, et al. Transcriptional repression by the methyl-CpG-binding protein MeCP2 involves a histone deacetylase complex. Nature 1998; 393:386 - 389; PMID: 9620804; http://dx.doi.org/10.1038/30764
- Kuczenski R, Segal DS, Aizenstein ML. Amphetamine, cocaine and fencamfamine: relationship between locomotor and stereotypy response profiles and caudate and accumbens dopamine dynamics. J Neurosci 1991; 11:2703 - 2712; PMID: 1715389
- Hope B, Kosofsky B, Hyman SE, Nestler EJ. Regulation of immediate early gene expression and AP-1 binding in the rat nucleus accumbens by chronic cocaine. Proc Natl Acad Sci USA 1992; 89:5764 - 5768; PMID: 1631058; http://dx.doi.org/10.1073/pnas.89.13.5764
- Graybiel AM, Moratalla R, Robertson HA. Amphetamine and cocaine induce drug-specific activation of the c-fos gene in striosome-matrix compartments and limbic subdivisions of the striatum. Proc Natl Acad Sci USA 1990; 87:6912 - 6916; PMID: 2118661; http://dx.doi.org/10.1073/pnas.87.17.6912
- Fumagalli F, Bedogni F, Frasca A, Di Pasquale L, Racagni G, Riva MA. Corticostriatal upregulation of activity-regulated cytoskeletal-associated protein expression after repeated exposure to cocaine. Mol Pharmacol 2006; 70:1726 - 1734; PMID: 16908598; http://dx.doi.org/10.1124/mol.106.026302
- Lyons MR, West AE. Mechanisms of specificity in neuronal activity-regulated gene transcription. Prog Neurobiol 2011; 94:259 - 295; PMID: 21620929; http://dx.doi.org/10.1016/j.pneurobio.2011.05.003
- Hargreaves DC, Horng T, Medzhitov R. Control of inducible gene expression by signal-dependent transcriptional elongation. Cell 2009; 138:129 - 145; PMID: 19596240; http://dx.doi.org/10.1016/j.cell.2009.05.047
- Ernst J, Kheradpour P, Mikkelsen TS, Shoresh N, Ward LD, Epstein CB, et al. Mapping and analysis of chromatin state dynamics in nine human cell types. Nature 2011; 473:43 - 49; PMID: 21441907; http://dx.doi.org/10.1038/nature09906
- Skene PJ, Illingworth RS, Webb S, Kerr AR, James KD, Turner DJ, et al. Neuronal MeCP2 is expressed at near histone-octamer levels and globally alters the chromatin state. Mol Cell 2010; 37:457 - 468; PMID: 20188665; http://dx.doi.org/10.1016/j.molcel.2010.01.030
- Buratowski S. Progression through the RNA polymerase II CTD cycle. Mol Cell 2009; 36:541 - 546; PMID: 19941815; http://dx.doi.org/10.1016/j.mol-cel.2009.10.019
- Saha RN, Wissink EM, Bailey ER, Zhao M, Fargo DC, Hwang JY, et al. Rapid activity-induced transcription of Arc and other IEGs relies on poised RNA polymerase II. Nat Neurosci 2011; 14:848 - 856; PMID: 21623364; http://dx.doi.org/10.1038/nn.2839
- Kim TK, Hemberg M, Gray JM, Costa AM, Bear DM, Wu J, et al. Widespread transcription at neuronal activity-regulated enhancers. Nature 2010; 465:182 - 187; PMID: 20393465; http://dx.doi.org/10.1038/nature09033
- Sims RJ 3rd, Belotserkovskaya R, Reinberg D. Elongation by RNA polymerase II: the short and long of it. Genes Dev 2004; 18:2437 - 2468; PMID: 15489290; http://dx.doi.org/10.1101/gad.1235904
- Guy J, Cheval H, Selfridge J, Bird A. The Role of MeCP2 in the Brain. Ann Rev Cell Dev Biol 2011;
- Meissner A, Mikkelsen TS, Gu H, Wernig M, Hanna J, Sivachenko A, et al. Genome-scale DNA methylation maps of pluripotent and differentiated cells. Nature 2008; 454:766 - 770; PMID: 18600261
- Cohen S, Gabel HW, Hemberg M, Hutchinson AN, Sadacca LA, Ebert DH, et al. Genome-wide activity-dependent MeCP2 phosphorylation regulates nervous system development and function. Neuron 2011; 72:72 - 85; PMID: 21982370; http://dx.doi.org/10.1016/j.neuron.2011.08.022
- Yasui DH, Peddada S, Bieda MC, Vallero RO, Hogart A, Nagarajan RP, et al. Integrated epigenomic analyses of neuronal MeCP2 reveal a role for long-range interaction with active genes. Proc Natl Acad Sci USA 2007; 104:19416 - 19421; PMID: 18042715; http://dx.doi.org/10.1073/pnas.0707442104
- Chen WG, Chang Q, Lin Y, Meissner A, West AE, Griffith EC, et al. Derepression of BDNF transcription involves calcium-dependent phosphorylation of MeCP2. Science 2003; 302:885 - 889; PMID: 14593183; http://dx.doi.org/10.1126/science.1086446
- Chahrour M, Jung SY, Shaw C, Zhou X, Wong ST, Qin J, et al. MeCP2, a key contributor to neurological disease, activates and represses transcription. Science 2008; 320:1224 - 1229; PMID: 18511691; http://dx.doi.org/10.1126/science.1153252
- Chandler SP, Guschin D, Landsberger N, Wolffe AP. The methyl-CpG binding transcriptional repressor MeCP2 stably associates with nucleosomal DNA. Biochemistry 1999; 38:7008 - 7018; PMID: 10353812; http://dx.doi.org/10.1021/bi990224y
- Horike S, Cai S, Miyano M, Cheng JF, Kohwi-Shigematsu T. Loss of silent-chromatin looping and impaired imprinting of DLX5 in Rett syndrome. Nat Genet 2005; 37:31 - 40; PMID: 15608638
- Wu H, Tao J, Chen PJ, Shahab A, Ge W, Hart RP, et al. Genome-wide analysis reveals methyl-CpG-binding protein 2-dependent regulation of microRNAs in a mouse model of Rett syndrome. Proc Natl Acad Sci USA 2010; 107:18161 - 18166; PMID: 20921386; http://dx.doi.org/10.1073/pnas.1005595107
- Stancheva I, Collins AL, Van den Veyver IB, Zoghbi H, Meehan RR. A mutant form of MeCP2 protein associated with human Rett syndrome cannot be displaced from methylated DNA by notch in Xenopus embryos. Mol Cell 2003; 12:425 - 435; PMID: 14536082; http://dx.doi.org/10.1016/S1097-2765(03)00276-4
- Jones PL, Veenstra GJ, Wade PA, Vermaak D, Kass SU, Landsberger N, et al. Methylated DNA and MeCP2 recruit histone deacetylase to repress transcription. Nat Genet 1998; 19:187 - 191; PMID: 9620779; http://dx.doi.org/10.1038/561
- Muotri AR, Marchetto MC, Coufal NG, Oefner R, Yeo G, Nakashima K, et al. L1 retrotransposition in neurons is modulated by MeCP2. Nature 2010; 468:443 - 446; PMID: 21085180; http://dx.doi.org/10.1038/nature09544
- Urdinguio RG, Pino I, Ropero S, Fraga MF, Esteller M. Histone H3 and H4 modification profiles in a Rett syndrome mouse model. Epigenetics 2007; 2:11 - 14; PMID: 17965622; http://dx.doi.org/10.4161/epi.2.1.3698
- Russo SJ, Dietz DM, Dumitriu D, Morrison JH, Malenka RC, Nestler EJ. The addicted synapse: mechanisms of synaptic and structural plasticity in nucleus accumbens. Trends Neurosci 2010; 33:267 - 276; PMID: 20207024; http://dx.doi.org/10.1016/j.tins.2010.02.002
- McCurry CL, Shepherd JD, Tropea D, Wang KH, Bear MF, Sur M. Loss of Arc renders the visual cortex impervious to the effects of sensory experience or deprivation. Nat Neurosci 2010; 13:450 - 457; PMID: 20228806; http://dx.doi.org/10.1038/nn.2508
- Shepherd JD, Rumbaugh G, Wu J, Chowdhury S, Plath N, Kuhl D, et al. Arc/Arg3.1 mediates homeostatic synaptic scaling of AMPA receptors. Neuron 2006; 52:475 - 484; PMID: 17088213; http://dx.doi.org/10.1016/j.neuron.2006.08.034
- Peebles CL, Yoo J, Thwin MT, Palop JJ, Noebels JL, Finkbeiner S. Arc regulates spine morphology and maintains network stability in vivo. Proc Natl Acad Sci USA 2010; 107:18173 - 18178; PMID: 20921410; http://dx.doi.org/10.1073/pnas.1006546107
- Gillespie RF, Gudas LJ. Retinoid regulated association of transcriptional co-regulators and the polycomb group protein SUZ12 with the retinoic acid response elements of Hoxa1, RARbeta(2) and Cyp26A1 in F9 embryonal carcinoma cells. J Mol Biol 2007; 372:298 - 316; PMID: 17663992; http://dx.doi.org/10.1016/j.jmb.2007.06.079