Abstract
Hedgehog (Hh) signaling is essential for proper tissue patterning and maintenance and has a substantial impact on human disease. While many of the main components and mechanisms involved in transduction of the Hh signal have been identified, the details of how the pathway functions are continually being refined. One aspect that has attracted much attention recently is the involvement of G-protein-coupled receptor kinases (GRKs) in the pathway. These regulators of G-protein-coupled receptor (GPCR) signaling have an evolutionarily-conserved function in promoting high-threshold Hh target gene expression through regulation of Smoothened (Smo), a GPCR family member that activates intracellular Hh signaling. Several models of how GRKs impact on Smo to increase downstream signaling have been proposed. Recently, we demonstrated that these kinases have surprisingly complex and conflicting roles, acting to limit signaling through the pathway while also promoting Smo activity. In addition to the previously described direct effects of Gprk2 on Smo activation, Gprk2 also indirectly affects Hh signaling by controlling production of the second messenger cyclic AMP to influence Protein kinase A activity.
The overall growth and patterning of embryonic tissues is largely governed by signaling pathways that are activated in response to secreted growth factors, or morphogens. The Hedgehog (Hh) proteins are one of the principal families of morphogens that are required for the normal development of most tissues in animals ranging from flies to humans.Citation1 The activity of Hh proteins is not limited to development, as they also play important roles in stem cell maintenance, and thus tissue homeostasis, in adults.Citation2 Not surprisingly given its prominence, misregulation of Hh signaling has wide-ranging biological consequences, and has been identified as the cause of a variety of congenital syndromes and cancers.Citation3,Citation4
A number of conserved proteins required for transducing the signal downstream of Hh have been identified by genetic studies in model organisms, and these have been assembled into a core “canonical” pathway (reviewed in refs. Citation5,Citation6). The functioning of this pathway is best understood in Drosophila. As in mammals, the key initiator of intracellular signaling in flies is Smoothened (Smo), a seven-pass transmembrane protein that, together with Frizzled proteins, forms a distinct class of the G-protein-coupled receptor (GPCR) protein family.Citation7 Under basal conditions, Smo activity is kept low by the Hh receptor Patched. Hh binding relieves Patched-mediated repression of Smo. Smo in turn becomes highly phosphorylated, translocates to the cell surface, and signals through a complex of proteins that includes the serine/threonine kinase Fused (Fu), the scaffold protein Costal-2 (Cos2), and Suppressor of Fused (SuFu) to control the proteolytic processing and activity of the transcription factor Cubitus interruptus (Ci). Pathway activation leads to stabilization of Ci, reducing the levels of a proteolytically-cleaved 75 kDa transcriptional repressor form (Ci75) and increasing the levels of a full-length transcriptional activator form (Ci155), thereby promoting Hh target gene expression.
Like all morphogens, Hh proteins form concentration gradients in tissues centered on their sites of production, and differentially activate target genes in a concentration-dependent manner. This means that the ligand distribution gradient has to be converted to graded pathway activity in the field of target cells. This appears to be achieved in large part through graded activity of Smo. Like other GPCRs, Smo can exist in at least two conformational states with different signaling capacities.Citation8,Citation9 Transition between these states is controlled by phosphorylation of the cytoplasmic tail of Smo. More extensive phosphorylation either increases the probability of Smo adopting an active conformation or induces a greater conformational shift, leading to higher levels of Smo activity and higher-threshold target gene activation.Citation8 Smo phosphorylation is thus an important determinant of Hh activity.
G-Protein-Coupled Receptor Kinases: Conserved Regulators of Hh Signaling
In Drosophila, Protein kinase A (Pka) and Casein kinase I (CkI) are the main kinases responsible for activating Smo. In response to Hh, Smo is phosphorylated at three sets of clustered Pka and CkI sites.Citation10-Citation12 Phosphorylation at the Pka sites is sufficient to drive low-threshold target gene expression, and primes Smo for further phosphorylation by CkI to promote high-threshold target gene expression.Citation13 At least 18 additional Hh-responsive phosphorylation sites have been identified in Drosophila Smo, suggesting that additional kinases are involved in Smo regulation.Citation11 A growing body of evidence clearly implicates G-protein-coupled receptor kinases (GRKs) in this process in both flies and mammals. The mammalian GRKs are well-known regulators of GPCR signaling. The best-characterized function of these kinases is to dampen GPCR signaling in a process known as homologous desensitization.Citation14 They do this by binding to and phosphorylating a wide variety of GPCRs specifically in their agonist-occupied activated conformations, creating high-affinity binding sites for β-arrestins. β-arrestin recruitment to phosphorylated receptors interferes with heterotrimeric G-protein coupling and promotes clathrin-dependent receptor internalization, effectively shutting off G-protein-dependent signaling and limiting the capacity for reactivation of the same receptor molecule. For some receptors, β-arrestin binding facilitates G-protein-independent signaling by acting as a scaffold for the assembly of signaling complexes.Citation14
The first indication that GRKs participate in Hh signaling came from studies in mammalian cells. Smo internalization and phosphorylation, as well as activation of a Hh-responsive reporter gene, were found to require GRK2, one of seven mammalian GRK family kinases.Citation15,Citation16 Further support came from the characterization of GRK2 morphant zebrafish and GRK2 knockout mouse embryos, which displayed phenotypes consistent with moderate impairment of Hh signaling and reduced Hh target gene expression.Citation17 Several lines of evidence indicate that β-arrestins are also involved. β-arrestin is found in complex with active Smo.Citation16,Citation18,Citation19 Manipulation of β-arrestin levels has effects similar to GRK2 on Smo trafficking, and reduction of β-arrestin levels in cells and in vivo impairs Hh signaling.Citation15,Citation16,Citation18,Citation20 GRK2 facilitates the association between Smo and β-arrestin,Citation16 consistent with it regulating Smo in a similar manner as it does other GPCRs. This regulation is important for translocation of Smo to the primary cilium, its apparent site of action, upon pathway activation.Citation18,Citation19,Citation21
The role of GRKs in Hh signaling is conserved in lower organisms. Three separate studies have demonstrated that Gprk2, one of the two GRKs found in Drosophila, is required for efficient high-threshold target gene expression. Elimination of Gprk2 in the developing fly wing primordium (or imaginal disc), either by double-stranded RNA-mediated depletionCitation22 or in genetic mutants,Citation23,Citation24 leads to a moderate Hh loss-of-function phenotype, characterized by a reduction in the area of the central region of the adult wing (). This phenotype results from the loss of high- but not low-threshold target gene expression (), indicating that Gprk2 is required for high-level signaling. The requirement for Gprk2 is not unique to signaling in the developing wing, as mutant embryos derived from gprk2 homozygous mutant mothers show cuticle patterning defects typical of moderate Hh signaling impairment (). Interestingly, gprk2 is a Hh target gene in flies, suggesting that it may participate in a feedback loop required to amplify pathway activity.Citation22 At a cellular level, loss of Gprk2 leads to excessive accumulation of a hypophosphorylated form of Smo at the cell surface,Citation24 consistent with an impairment of receptor phosphorylation and internalization. Overexpression of Gprk2 has the opposite effect, downregulating endogenous Smo levels in cells in which Smo is strongly activated.Citation24 Perhaps surprisingly given the evidence in mammals, loss of the single β-arrestin (called Kurtz) in flies does not noticeably affect Hh signaling or Smo accumulation.Citation24,Citation26 However, Kurtz can associate with and regulate Smo when overexpressed,Citation24,Citation26,Citation27 leaving open the possibility that the role of endogenous Kurtz is masked by functional redundancy.
Figure 1.gprk2 mutants show moderate Hh loss-of-function phenotypes. (A) Wild-type Drosophila wing. The region patterned by Hh signaling, bounded by the third and fourth wing veins, is shaded pink. (B) A gprk2 mutant wing, showing a reduction of the territory between veins three and four that is characteristic of moderate Hh signaling impairment. (C) Schematic diagram of anterior-posterior patterning of the wing by Hh. The wing disc is divided into two non-mixing cell compartments, anterior (A, shaded blue) and posterior (P, shaded purple), divided by a compartment boundary (dotted line). Hh is produced by P compartment cells, which do not express Ci and are thus unable to mount a transcriptional response. Instead, Hh diffuses into the Ci-expressing A compartment, inducing Hh target gene expression in a stripe of cells just A to the compartment boundary. High threshold target genes like ptc, which require high levels of Hh for activation, are expressed in a narrow stripe (green). Low threshold target genes like decapentaplegic (dpp) are expressed in a wider stripe of cells (red). (D) Wild-type wing disc, showing the typical pattern of expression of Ptc (green) and dpp (red, visualized by LacZ expression from an enhancer trap). (E) In a gprk mutant wing disc, high but not low threshold target gene expression is lost. (F) Wing disc in which an activated form of Gαs is expressed in the dorsal half (D, above dotted line) in an otherwise wild-type background. The resulting increase in cAMP production in dorsal cells has little effect on Ptc expression (compare with wild-type ventral cells, V), indicating that cAMP levels are not normally limiting for this response. (G) In a gprk2 mutant background, expression of activated Gαs in the dorsal half (D, above dotted line) specifically rescues ptc expression (compare with gprk2 mutant ventral cells, V). This indicates that cAMP levels are limiting for Hh responses in the absence of Gprk2. (H) Ventral cuticle of a wild-type embryo, showing the typical segmentally-repeated pattern of denticle belts. (I) In a gprk2 maternal and zygotic mutant embryo, the denticle belts are frequently fused (yellow arrowheads). This phenotype is similar to that observed with hypomorphic alleles of smo,Citation25 and indicates that Gprk2 is also required for normal Hh signaling in the embryonic epidermis.
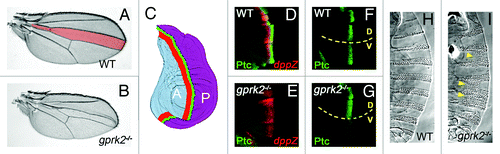
How Do GRKs Affect Transduction of the Hh Signal?
The evidence from several systems linking GRKs and β-arrestins with Smo accumulation and trafficking strongly suggests that GRKs regulate Smo in a manner typical of other GPCRs. This implies that GRKs should function to desensitize, or shut off, Smo. With other GPCRs, this is manifested in several ways, including increased cell surface receptor levels and prolonged or excessive signaling responses in the absence of the regulating GRK.Citation28 In the case of Smo, cell surface protein levels are substantially increased in gprk2 mutant Hh-responding cells, which is usually a sign of high-level signaling.Citation29,Citation30 Yet phenotypically, the loss of GRKs would appear to have the opposite effect, rendering Smo less active. Several explanations for this contradiction have been proposed. There is strong evidence that direct phosphorylation of Smo by GRKs contributes to the conformational shift associated with Smo activation in both flies and mammals. Thus the loss of GRKs can impair Hh responses by preventing Smo from reaching its most active state.Citation19,Citation23 Gprk2 can also facilitate Smo dimerization in a manner independent of its kinase activity.Citation23 It has also been suggested that GRK-dependent β-arrestin-binding and/or subsequent internalization of Smo may be required for efficient signaling. This could reflect the need for receptor recycling or ciliary translocation (in mammals) for high-level signaling, or by analogy with other GPCRs, the participation of β-arrestins in assembly of a Smo-associated signaling complex (for example, with Fu and Cos2 in flies).Citation14,Citation31-Citation33
Our recent analysis of Gprk2 in fliesCitation34 suggests that the role of GRKs in Hh signaling is more complex than envisioned. As summarized below, we found evidence that they act as both positive and negative regulators of signaling. Furthermore, they appear to have indirect effects on pathway activity in addition to the more direct effects on Smo noted above.
Gprk2 Suppresses Canonical Pathway Activation but Promotes Hh Target Gene Expression
We initially set out to assess how the loss of Gprk2 affects Smo activity. Rather than using Hh target gene expression as a readout, we focused instead on more proximal signaling events. In order for high-threshold target genes to be expressed, Ci155 must be stabilized and its retention in the cytoplasm by SuFu and Cos2 must be neutralized to enable it to enter the nucleus.Citation35-Citation37 Despite the loss of high-threshold target gene expression in gprk2 mutant wing discs, Smo-dependent stabilization and nuclear accumulation of Ci155 in Hh-responding cells appeared normal, indicating that signaling from Smo to Ci was intact. This signal is transduced by Fu, Cos2, and SuFu, which all become phosphorylated in response to Smo activation.Citation38,Citation39 Unexpectedly, Smo-dependent phosphorylation of all three proteins was increased in the absence of Gprk2. This indicates that total Smo activity is higher rather than lower without Gprk2, and is the first sign that Gprk2 acts negatively in the pathway to limit Smo activity, as predicted if it desensitizes Smo in the classical sense (). Furthermore, it argues against the possibility that Gprk2/β-arrestin-dependent Smo trafficking or signaling complex formation is strictly required for activation of canonical signaling.
Figure 2. Multiple effects of Gprk2 on the Hh signaling pathway. (A) Gprk2 “desensitizes” Smo like a typical GPCR, phosphorylating the active form of Smo (Smo**) to promote its internalization and degradation. When Gprk2 is missing active Smo accumulates, leading to increased canonical pathway activation. (B) Gprk2 directly promotes Smo activation by phosphorylating Pka- and CkI-phosphorylated Smo, increasing its conformational shift and enhancing its activity.Citation23 Loss of Gprk2 thus prevents the full activation of Smo required for high-threshold target gene expression. (C) Gprk2 indirectly promotes Smo activation via Pka. Gprk2 activity helps to maintain high cellular cAMP levels, most likely through its role in limiting GPCR signaling. Loss of Gprk2 leads to a decrease in cAMP production and reduced Pka activity. This in turn decreases Pka-dependent Smo phosphorylation and activation, leading to a loss of high threshold target gene expression.
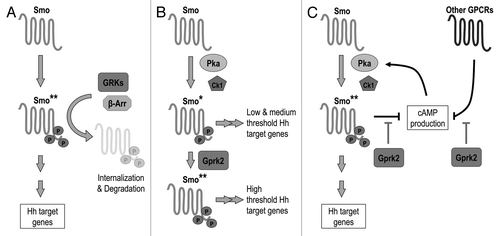
If GRKs are shutting off Smo signaling, why is high threshold target gene expression lost in their absence? One answer, mentioned above, is that they play dual roles when it comes to Smo. In addition to desensitizing Smo, direct GRK-mediated phosphorylation is required for Smo to reach its most active state (with activity assessed by target gene expression) ().Citation19,Citation23 Taking this into consideration, a likely explanation for the increase in canonical pathway activity observed in the absence of Gprk2 is that it results from the ectopic accumulation of a sub-maximally activated form of Smo. Although total pathway activity is higher because of the increase in cell-surface Smo levels and/or the duration of its activity in gprk2 mutant cells, the reduced per-molecule activity of Smo may affect the dynamics of activation in a way that prevents high-threshold target gene expression. In this interpretation, the indicators of pathway activity that increase in the absence of Gprk2 may effectively be measures of low-threshold signaling.
Connecting GRKs, Second Messenger Regulation and Hh Signaling
Direct GRK-mediated activation of Smo provides a straightforward explanation for the effects of these kinases on Hh target gene expression. However, we found that the situation is more complicated, with the loss of Gprk2 also indirectly impacting on the Hh pathway through altered second messenger signaling. This is not entirely unexpected. In both flies and mammals, a relatively small number of GRKs (two and seven, respectively) is tasked with regulating a huge complement of receptors—more than one hundred in flies and one thousand in mammals,Citation7,Citation40 the majority of which are thought likely to be GRK substrates.Citation41 In mammals, a single GRK can regulate many receptors, and one receptor can be regulated by several kinases.Citation41 The situation is presumably similar in Drosophila. Indeed, we have observed some functional redundancy between Gprk1 and Gprk2 in flies, at least during development, suggesting that they regulate some common targets.Citation24 Given this level of interconnectedness, the removal of a single GRK has the potential to simultaneously affect the activity of multiple GPCRs, and by extension second messenger dynamics, within a single cell.
Does this actually occur? Although the effects of GRK elimination are to some extent mitigated by functional redundancy, selective defects in receptor desensitization and second messenger production are observed in the absence of individual GRKs, both in cell culture models and in mouse knockouts.Citation28 For example, in addition to impairing Hh signaling, depletion of GRK2 in mammalian cells also elevates production of the second messengers cyclic AMP (cAMP) and diacylglycerol in response to activation of the V2 Vasopressin and Angiotensin II Type 1A GPCRs, respectively,Citation42,Citation43 whereas some other GPCR responses are normal.Citation44 Similarly, elimination of GRK6 causes exaggerated second messenger responses upon activation of a subset of GPCRs,Citation44-Citation46 without affecting others.Citation42 Because second messenger-dependent kinases also feed back to regulate diverse GPCR responses through heterologous desensitization,Citation47 there is also the potential for misregulation of GPCRs controlled by a given GRK to indirectly affect the activity of GPCRs not regulated by that GRK. Thus, individual GRKs can and do control a complex set of receptor responses, with consequent effects on second messenger production.
This is particularly relevant in the case of Hh signaling, where the cAMP-regulated kinase Pka plays an integral role in the pathway. Pka actually has dual functions, acting as both a silencer and activator of the pathway. On one hand, Pka phosphorylation promotes the proteolytic cleavage of Ci and one of its mammalian orthologs, Gli3, to their shorter transcriptional repressor forms,Citation48,Citation49 and also directly inhibits the transcriptional activator functions of full-length Ci and Gli proteins,Citation50 both of which serve to keep target genes off in the absence of Hh. Consequently, strong inhibition or elimination of Pka leads to constitutive Ci/Gli activation and ectopic target gene activation in both Drosophila and vertebrates.Citation51-Citation54 On the other hand, Pka activation can induce ectopic target gene expression and gain-of-function phenotypes in flies and vertebrates,Citation10,Citation55-Citation57 whereas Pka inhibition can block Hh-induced activation of target genes,Citation10,Citation57 indicating that Pka also has a positive function. Smo is one identified target of positive regulation by Pka in flies, through its C-terminus.Citation10-Citation12 Comparable Pka sites are not present in vertebrate Smo proteins, and there is no evidence that they are directly regulated by Pka, leaving the identity of the positive target in vertebrates unknown. The existence of another target is further supported by evidence from Drosophila embryos, where Pka appears to stimulate Hh target gene expression not only through Smo, but through another unknown target acting in parallel to Smo and Ci regulation as well.Citation58
The fact that Pka regulates critical pathway components with such opposite outcomes would suggest that its activity must be tightly controlled and perhaps itself regulated by Hh in order for the pathway to function properly. However, the evidence in this regard is equivocal. Smo has long been suspected to function as a GPCR, and there is evidence that in some systems it can couple to Gαi, a heterotrimeric G-protein α-subunit that inhibits adenylate cyclase activity to suppress cAMP productionCitation59 (further reviewed in Ref.Citation33). In other systems this seems not to be the case. Furthermore, Hh responsiveness in Pka mutant cells can be restored by reintroduction of a cAMP-insensitive form of the kinase, which has been interpreted as meaning that Pka activity need not be regulated by Hh.Citation53,Citation54 Whether or not it is directly regulated by Hh, it is clear that Pka activity needs to fall within a limited range to allow optimal functioning of the pathway. In Drosophila, no Pka activity leads to constitutive Ci activation,Citation53,Citation54 too little limits Smo activity,Citation10 a moderate increase enhances Hh responsiveness,Citation10,Citation55 and too much inhibits target gene activation.Citation54,Citation58
cAMP Misregulation Impairs Hh Target Gene Expression in gprk2 Mutants
Like its mammalian counterparts, Gprk2 has been implicated in second messenger regulation in Drosophila. In analyzing animals homozygous for a mutant allele of gprk2 that disrupts expression in the ovary, Lanutti et al.Citation60 found that the loss of Gprk2 was associated with a 70% drop in ovarian cAMP levels. By genetically manipulating enzymes involved in cAMP metabolism to increase or further decrease cAMP levels, they also showed that the reduction in cAMP levels was a significant contributor to the germline defects and reduced fertility of the mutant flies. This implies that Gprk2 affects egg production indirectly, through its effects on second messenger signaling. Using null alleles of gprk2, we found that cAMP levels in gprk2 homozygous mutant larvae were similarly reduced compared with wild-type animals. This seems to be a more general effect, as it could be reproduced by Gprk2 depletion in cultured cells. We therefore hypothesized that the low levels of cAMP/Pka activity may be limiting for efficient functioning of the Hh pathway in gprk2 mutants.
To test this hypothesis, we examined the effects of manipulating cAMP levels and Pka activity by expressing transgenic regulators of each on Hh target gene expression in the mutants. Lowering the already low levels of cAMP or Pka activity in the gprk2 mutants further impaired Hh-dependent high-threshold target gene expression in gprk2 mutant flies, but not wild-type flies. Conversely, increasing cAMP levels or Pka activity efficiently and specifically rescued Hh-dependent expression of high-threshold target genes to apparently normal levels in the absence of Gprk2 (). Both results indicate that the low levels of cAMP in gprk2 mutants are limiting for Hh target gene expression. Thus, as with its role in egg production, Gprk2 has indirect effects on the Hh pathway through second messenger signaling. Although Smo can itself signal through Gαi to lower cAMP levels, double knockdown experiments suggest that the cAMP defect in gprk2 mutants is independent of Smo activity. The changes in cAMP regulation could result from a broader misregulation of GPCR signaling in the gprk2 mutants.
Further support for an indirect effect comes from an analysis of Smo itself. Smo is less highly phosphorylated in the absence of Gprk2.Citation24 We found that this Gprk2-dependent effect on Smo phosphorylation persists when the direct Gprk2 phosphoacceptor sites in SmoCitation23 are eliminated. While there may be additional unmapped Gprk2 phosphorylation sites in Smo, it could also be that another kinase phosphorylates Smo in a Gprk2-dependent manner. Two pieces of evidence suggest that this latter explanation is at least partly correct and that the kinase involved is Pka, as our model would predict. First, Pka activation largely restores Smo phosphorylation in the absence of Gprk2. Second, a Pka phosphomimetic form of Smo rescues Hh target gene expression in gprk2 mutants. Interestingly, the phosphomimetic Smo is less effective than cAMP in rescuing target gene expression, suggesting that Pka may act through a target in addition to Smo in wing discs, as it does in embryos.Citation58 Taken together, our results suggest that Gprk2 helps maintain cellular cAMP levels high enough to permit full phosphorylation and activation of Smo, and possibly another target, by Pka (). How this indirect function of Gprk2 fits with its direct effects on Smo phosphorylation and dimerizationCitation23 is not clear. While the pathway can clearly function in the absence of Gprk2 if Pka activity is high enough, both mechanisms may be important for efficient Hh signaling under normal circumstances.
Implications for cAMP and PKA Function in Hh Signaling
Our study reveals an interesting connection between endogenous cAMP levels and pathway function. Despite the fact that Pka phosphorylation activates Smo, under normal circumstances moderate activation of Pka has only a small effect in extending the range of high threshold target gene expression in the wing disc.Citation10 This is true even when controlling for the negative effect of Pka on Ci.Citation58 This is consistent with our observation that manipulating cAMP production has little effect on high-threshold target gene activation in wild-type discsCitation34 (though lowering cAMP levels can de-repress low-threshold targetsCitation59). Thus Pka activity, and by extension cAMP levels, are not major limiting factors for Hh pathway activation in the wing disc. In contrast, Pka activity is limiting for pathway activation in the embryonic epidermis, as expression of constitutively active Pka leads to a dramatic expansion in Hh target gene expression due to dual effects on Smo and at least one other target.Citation55,Citation58 The functioning of the pathway in the embryonic epidermis in some ways resembles the situation in gprk2 mutant wing discs in this regard. One explanation for the observed difference in pathway function between discs and embryos could thus be that Gprk2 normally participates in Hh signaling only in discs. However, the fact that gprk2 maternal/zygotic mutant embryos show patterning defects typical of reduced Hh signaling () suggests that this is unlikely. An alternate explanation could be that baseline cAMP levels are higher in wild-type wing discs than they are in embryonic epidermal cells or gprk2 mutant discs, so that cAMP levels limit signaling only in the latter. Differences in the complement of GPCRs that are expressed and active in a given tissue could contribute to such intrinsic differences in cAMP levels. While this is speculative, there is evidence that regulation of cAMP levels by different GPCRs can influence Hh pathway-dependent responses in the vertebrate nervous system.Citation61,Citation62 If cellular cAMP levels do set thresholds for Hh pathway activation, then cell- or tissue-type-dependent differences in cAMP levels could provide a simple explanation for the often-conflicting results concerning the importance of heterotrimeric G-proteins in functioning of the pathway.
Abbreviations: | ||
cAMP | = | 3′-5′-cyclic adenosine monophosphate |
Ci | = | Cubitus interruptus |
CkI | = | Casein kinase I |
Cos2 | = | Constal-2 |
Fu | = | Fused |
GPCR | = | G-protein-coupled receptor |
Gprk2 | = | G-protein-coupled receptor kinase 2 |
GRK | = | G-protein-coupled receptor kinase |
Hh | = | Hedgehog |
Pka | = | Protein kinase A |
Smo | = | Smoothened |
SuFu | = | Suppressor of Fused |
Acknowledgments
This work was supported by funding from the Canadian Institutes of Health Research.
References
- McMahon AP, Ingham PW, Tabin CJ. Developmental roles and clinical significance of hedgehog signaling. Curr Top Dev Biol 2003; 53:1 - 114; http://dx.doi.org/10.1016/S0070-2153(03)53002-2; PMID: 12509125
- Beachy PA, Karhadkar SS, Berman DM. Tissue repair and stem cell renewal in carcinogenesis. Nature 2004; 432:324 - 31; http://dx.doi.org/10.1038/nature03100; PMID: 15549094
- Nieuwenhuis E, Hui CC. Hedgehog signaling and congenital malformations. Clin Genet 2005; 67:193 - 208; http://dx.doi.org/10.1111/j.1399-0004.2004.00360.x; PMID: 15691355
- Scales SJ, de Sauvage FJ. Mechanisms of Hedgehog pathway activation in cancer and implications for therapy. Trends Pharmacol Sci 2009; 30:303 - 12; http://dx.doi.org/10.1016/j.tips.2009.03.007; PMID: 19443052
- Hooper JE, Scott MP. Communicating with Hedgehogs. Nat Rev Mol Cell Biol 2005; 6:306 - 17; http://dx.doi.org/10.1038/nrm1622; PMID: 15803137
- Jiang J, Hui CC. Hedgehog signaling in development and cancer. Dev Cell 2008; 15:801 - 12; http://dx.doi.org/10.1016/j.devcel.2008.11.010; PMID: 19081070
- Foord SM, Bonner TI, Neubig RR, Rosser EM, Pin JP, Davenport AP, et al. International Union of Pharmacology. XLVI. G protein-coupled receptor list. Pharmacol Rev 2005; 57:279 - 88; http://dx.doi.org/10.1124/pr.57.2.5; PMID: 15914470
- Zhao Y, Tong C, Jiang J. Hedgehog regulates smoothened activity by inducing a conformational switch. Nature 2007; 450:252 - 8; http://dx.doi.org/10.1038/nature06225; PMID: 17960137
- Rosenbaum DM, Rasmussen SG, Kobilka BK. The structure and function of G-protein-coupled receptors. Nature 2009; 459:356 - 63; http://dx.doi.org/10.1038/nature08144; PMID: 19458711
- Jia J, Tong C, Wang B, Luo L, Jiang J. Hedgehog signalling activity of Smoothened requires phosphorylation by protein kinase A and casein kinase I. Nature 2004; 432:1045 - 50; http://dx.doi.org/10.1038/nature03179; PMID: 15616566
- Zhang C, Williams EH, Guo Y, Lum L, Beachy PA. Extensive phosphorylation of Smoothened in Hedgehog pathway activation. Proc Natl Acad Sci U S A 2004; 101:17900 - 7; http://dx.doi.org/10.1073/pnas.0408093101; PMID: 15598741
- Apionishev S, Katanayeva NM, Marks SA, Kalderon D, Tomlinson A. Drosophila Smoothened phosphorylation sites essential for Hedgehog signal transduction. Nat Cell Biol 2005; 7:86 - 92; http://dx.doi.org/10.1038/ncb1210; PMID: 15592457
- Su Y, Ospina JK, Zhang J, Michelson AP, Schoen AM, Zhu AJ. Sequential phosphorylation of smoothened transduces graded hedgehog signaling. Sci Signal 2011; 4:ra43; http://dx.doi.org/10.1126/scisignal.2001747; PMID: 21730325
- Reiter E, Lefkowitz RJ. GRKs and beta-arrestins: roles in receptor silencing, trafficking and signaling. Trends Endocrinol Metab 2006; 17:159 - 65; http://dx.doi.org/10.1016/j.tem.2006.03.008; PMID: 16595179
- Chen W, Ren XR, Nelson CD, Barak LS, Chen JK, Beachy PA, et al. Activity-dependent internalization of smoothened mediated by beta-arrestin 2 and GRK2. Science 2004; 306:2257 - 60; http://dx.doi.org/10.1126/science.1104135; PMID: 15618519
- Meloni AR, Fralish GB, Kelly P, Salahpour A, Chen JK, Wechsler-Reya RJ, et al. Smoothened signal transduction is promoted by G protein-coupled receptor kinase 2. Mol Cell Biol 2006; 26:7550 - 60; http://dx.doi.org/10.1128/MCB.00546-06; PMID: 16908539
- Philipp M, Fralish GB, Meloni AR, Chen W, MacInnes AW, Barak LS, et al. Smoothened signaling in vertebrates is facilitated by a G protein-coupled receptor kinase. Mol Biol Cell 2008; 19:5478 - 89; http://dx.doi.org/10.1091/mbc.E08-05-0448; PMID: 18815277
- Kovacs JJ, Whalen EJ, Liu R, Xiao K, Kim J, Chen M, et al. Beta-arrestin-mediated localization of smoothened to the primary cilium. Science 2008; 320:1777 - 81; http://dx.doi.org/10.1126/science.1157983; PMID: 18497258
- Chen Y, Sasai N, Ma G, Yue T, Jia J, Briscoe J, et al. Sonic Hedgehog dependent phosphorylation by CK1α and GRK2 is required for ciliary accumulation and activation of smoothened. PLoS Biol 2011; 9:e1001083; http://dx.doi.org/10.1371/journal.pbio.1001083; PMID: 21695114
- Wilbanks AM, Fralish GB, Kirby ML, Barak LS, Li YX, Caron MG. Beta-arrestin 2 regulates zebrafish development through the hedgehog signaling pathway. Science 2004; 306:2264 - 7; http://dx.doi.org/10.1126/science.1104193; PMID: 15618520
- Corbit KC, Aanstad P, Singla V, Norman AR, Stainier DY, Reiter JF. Vertebrate Smoothened functions at the primary cilium. Nature 2005; 437:1018 - 21; http://dx.doi.org/10.1038/nature04117; PMID: 16136078
- Molnar C, Holguin H, Mayor F Jr., Ruiz-Gomez A, de Celis JF. The G protein-coupled receptor regulatory kinase GPRK2 participates in Hedgehog signaling in Drosophila. Proc Natl Acad Sci U S A 2007; 104:7963 - 8; http://dx.doi.org/10.1073/pnas.0702374104; PMID: 17483466
- Chen Y, Li S, Tong C, Zhao Y, Wang B, Liu Y, et al. G protein-coupled receptor kinase 2 promotes high-level Hedgehog signaling by regulating the active state of Smo through kinase-dependent and kinase-independent mechanisms in Drosophila. Genes Dev 2010; 24:2054 - 67; http://dx.doi.org/10.1101/gad.1948710; PMID: 20844016
- Cheng S, Maier D, Neubueser D, Hipfner DR. Regulation of smoothened by Drosophila G-protein-coupled receptor kinases. Dev Biol 2010; 337:99 - 109; http://dx.doi.org/10.1016/j.ydbio.2009.10.014; PMID: 19850026
- van den Heuvel M, Ingham PW. smoothened encodes a receptor-like serpentine protein required for hedgehog signalling. Nature 1996; 382:547 - 51; http://dx.doi.org/10.1038/382547a0; PMID: 8700230
- Molnar C, Ruiz-Gómez A, Martín M, Rojo-Berciano S, Mayor F, de Celis JF. Role of the Drosophila non-visual ß-arrestin kurtz in hedgehog signalling. PLoS Genet 2011; 7:e1001335; http://dx.doi.org/10.1371/journal.pgen.1001335; PMID: 21437272
- Li S, Chen Y, Shi Q, Yue T, Wang B, Jiang J. Hedgehog-regulated ubiquitination controls smoothened trafficking and cell surface expression in Drosophila. PLoS Biol 2012; 10:e1001239; http://dx.doi.org/10.1371/journal.pbio.1001239; PMID: 22253574
- Premont RT, Gainetdinov RR. Physiological roles of G protein-coupled receptor kinases and arrestins. Annu Rev Physiol 2007; 69:511 - 34; http://dx.doi.org/10.1146/annurev.physiol.69.022405.154731; PMID: 17305472
- Denef N, Neubüser D, Perez L, Cohen SM. Hedgehog induces opposite changes in turnover and subcellular localization of patched and smoothened. Cell 2000; 102:521 - 31; http://dx.doi.org/10.1016/S0092-8674(00)00056-8; PMID: 10966113
- Zhu AJ, Zheng L, Suyama K, Scott MP. Altered localization of Drosophila Smoothened protein activates Hedgehog signal transduction. Genes Dev 2003; 17:1240 - 52; http://dx.doi.org/10.1101/gad.1080803; PMID: 12730121
- Moore CA, Milano SK, Benovic JL. Regulation of receptor trafficking by GRKs and arrestins. Annu Rev Physiol 2007; 69:451 - 82; http://dx.doi.org/10.1146/annurev.physiol.69.022405.154712; PMID: 17037978
- Rohatgi R, Scott MP. Cell biology. Arrestin’ movement in cilia. Science 2008; 320:1726 - 7; http://dx.doi.org/10.1126/science.1160448; PMID: 18583599
- Ayers KL, Thérond PP. Evaluating Smoothened as a G-protein-coupled receptor for Hedgehog signalling. Trends Cell Biol 2010; 20:287 - 98; http://dx.doi.org/10.1016/j.tcb.2010.02.002; PMID: 20207148
- Cheng S, Maier D, Hipfner DR. Drosophila G-protein-coupled receptor kinase 2 regulates cAMP-dependent Hedgehog signaling. Development 2012; 139:85 - 94; http://dx.doi.org/10.1242/dev.068817; PMID: 22096079
- Aza-Blanc P, Ramírez-Weber FA, Laget MP, Schwartz C, Kornberg TB. Proteolysis that is inhibited by hedgehog targets Cubitus interruptus protein to the nucleus and converts it to a repressor. Cell 1997; 89:1043 - 53; http://dx.doi.org/10.1016/S0092-8674(00)80292-5; PMID: 9215627
- Méthot N, Basler K. Suppressor of fused opposes hedgehog signal transduction by impeding nuclear accumulation of the activator form of Cubitus interruptus. Development 2000; 127:4001 - 10; PMID: 10952898
- Wang G, Amanai K, Wang B, Jiang J. Interactions with Costal2 and suppressor of fused regulate nuclear translocation and activity of cubitus interruptus. Genes Dev 2000; 14:2893 - 905; http://dx.doi.org/10.1101/gad.843900; PMID: 11090136
- Lum L, Zhang C, Oh S, Mann RK, von Kessler DP, Taipale J, et al. Hedgehog signal transduction via Smoothened association with a cytoplasmic complex scaffolded by the atypical kinesin, Costal-2. Mol Cell 2003; 12:1261 - 74; http://dx.doi.org/10.1016/S1097-2765(03)00426-X; PMID: 14636583
- Nybakken KE, Turck CW, Robbins DJ, Bishop JM. Hedgehog-stimulated phosphorylation of the kinesin-related protein Costal2 is mediated by the serine/threonine kinase fused. J Biol Chem 2002; 277:24638 - 47; http://dx.doi.org/10.1074/jbc.M110730200; PMID: 11934882
- Brody T, Cravchik A. Drosophila melanogaster G protein-coupled receptors. J Cell Biol 2000; 150:F83 - 8; http://dx.doi.org/10.1083/jcb.150.2.F83; PMID: 10908591
- Pitcher JA, Freedman NJ, Lefkowitz RJ. G protein-coupled receptor kinases. Annu Rev Biochem 1998; 67:653 - 92; http://dx.doi.org/10.1146/annurev.biochem.67.1.653; PMID: 9759500
- Ren XR, Reiter E, Ahn S, Kim J, Chen W, Lefkowitz RJ. Different G protein-coupled receptor kinases govern G protein and beta-arrestin-mediated signaling of V2 vasopressin receptor. Proc Natl Acad Sci U S A 2005; 102:1448 - 53; http://dx.doi.org/10.1073/pnas.0409534102; PMID: 15671180
- Violin JD, Dewire SM, Barnes WG, Lefkowitz RJ. G protein-coupled receptor kinase and beta-arrestin-mediated desensitization of the angiotensin II type 1A receptor elucidated by diacylglycerol dynamics. J Biol Chem 2006; 281:36411 - 9; http://dx.doi.org/10.1074/jbc.M607956200; PMID: 17008309
- Violin JD, DiPilato LM, Yildirim N, Elston TC, Zhang J, Lefkowitz RJ. beta2-adrenergic receptor signaling and desensitization elucidated by quantitative modeling of real time cAMP dynamics. J Biol Chem 2008; 283:2949 - 61; http://dx.doi.org/10.1074/jbc.M707009200; PMID: 18045878
- Kavelaars A, Vroon A, Raatgever RP, Fong AM, Premont RT, Patel DD, et al. Increased acute inflammation, leukotriene B4-induced chemotaxis, and signaling in mice deficient for G protein-coupled receptor kinase 6. J Immunol 2003; 171:6128 - 34; PMID: 14634128
- Vroon A, Heijnen CJ, Raatgever R, Touw IP, Ploemacher RE, Premont RT, et al. GRK6 deficiency is associated with enhanced CXCR4-mediated neutrophil chemotaxis in vitro and impaired responsiveness to G-CSF in vivo. J Leukoc Biol 2004; 75:698 - 704; http://dx.doi.org/10.1189/jlb.0703320; PMID: 14704365
- Freedman NJ, Lefkowitz RJ. Desensitization of G protein-coupled receptors. Recent Prog Horm Res 1996; 51:319 - 51, discussion 352-3; PMID: 8701085
- Price MA, Kalderon D. Proteolysis of cubitus interruptus in Drosophila requires phosphorylation by protein kinase A. Development 1999; 126:4331 - 9; PMID: 10477300
- Wang B, Fallon JF, Beachy PA. Hedgehog-regulated processing of Gli3 produces an anterior/posterior repressor gradient in the developing vertebrate limb. Cell 2000; 100:423 - 34; http://dx.doi.org/10.1016/S0092-8674(00)80678-9; PMID: 10693759
- Marks SA, Kalderon D. Regulation of mammalian Gli proteins by Costal 2 and PKA in Drosophila reveals Hedgehog pathway conservation. Development 2011; 138:2533 - 42; http://dx.doi.org/10.1242/dev.063479; PMID: 21610030
- Epstein DJ, Marti E, Scott MP, McMahon AP. Antagonizing cAMP-dependent protein kinase A in the dorsal CNS activates a conserved Sonic hedgehog signaling pathway. Development 1996; 122:2885 - 94; PMID: 8787761
- Hammerschmidt M, Bitgood MJ, McMahon AP. Protein kinase A is a common negative regulator of Hedgehog signaling in the vertebrate embryo. Genes Dev 1996; 10:647 - 58; http://dx.doi.org/10.1101/gad.10.6.647; PMID: 8598293
- Jiang J, Struhl G. Protein kinase A and hedgehog signaling in Drosophila limb development. Cell 1995; 80:563 - 72; http://dx.doi.org/10.1016/0092-8674(95)90510-3; PMID: 7867064
- Li W, Ohlmeyer JT, Lane ME, Kalderon D. Function of protein kinase A in hedgehog signal transduction and Drosophila imaginal disc development. Cell 1995; 80:553 - 62; http://dx.doi.org/10.1016/0092-8674(95)90509-X; PMID: 7867063
- Ohlmeyer JT, Kalderon D. Dual pathways for induction of wingless expression by protein kinase A and Hedgehog in Drosophila embryos. Genes Dev 1997; 11:2250 - 8; http://dx.doi.org/10.1101/gad.11.17.2250; PMID: 9303540
- Tiecke E, Turner R, Sanz-Ezquerro JJ, Warner A, Tickle C. Manipulations of PKA in chick limb development reveal roles in digit patterning including a positive role in Sonic Hedgehog signaling. Dev Biol 2007; 305:312 - 24; http://dx.doi.org/10.1016/j.ydbio.2007.02.017; PMID: 17376427
- Milenkovic L, Scott MP, Rohatgi R. Lateral transport of Smoothened from the plasma membrane to the membrane of the cilium. J Cell Biol 2009; 187:365 - 74; http://dx.doi.org/10.1083/jcb.200907126; PMID: 19948480
- Zhou Q, Apionishev S, Kalderon D. The contributions of protein kinase A and smoothened phosphorylation to hedgehog signal transduction in Drosophila melanogaster. Genetics 2006; 173:2049 - 62; http://dx.doi.org/10.1534/genetics.106.061036; PMID: 16783001
- Ogden SK, Fei DL, Schilling NS, Ahmed YF, Hwa J, Robbins DJ. G protein Galphai functions immediately downstream of Smoothened in Hedgehog signalling. Nature 2008; 456:967 - 70; http://dx.doi.org/10.1038/nature07459; PMID: 18987629
- Lannutti BJ, Schneider LE. Gprk2 controls cAMP levels in Drosophila development. Dev Biol 2001; 233:174 - 85; http://dx.doi.org/10.1006/dbio.2001.0219; PMID: 11319866
- Klein RS, Rubin JB, Gibson HD, DeHaan EN, Alvarez-Hernandez X, Segal RA, et al. SDF-1 alpha induces chemotaxis and enhances Sonic hedgehog-induced proliferation of cerebellar granule cells. Development 2001; 128:1971 - 81; PMID: 11493520
- Lelievre V, Seksenyan A, Nobuta H, Yong WH, Chhith S, Niewiadomski P, et al. Disruption of the PACAP gene promotes medulloblastoma in ptc1 mutant mice. Dev Biol 2008; 313:359 - 70; http://dx.doi.org/10.1016/j.ydbio.2007.10.031; PMID: 18036580