Abstract
The Drosophila embryonic salivary gland is a powerful system to analyze the cellular and molecular mechanisms of tubulogenesis in vivo. The secretory portion of the salivary gland (referred to here as the salivary gland), is a pair of elongated epithelial tubes with a single central lumen, that is formed by the invagination and collective migration of gland cells from the ventral surface of the embryo.Citation1,Citation2 As the salivary gland elongates during migration, the central lumen elongates and narrows at the proximal end.Citation3 A number of genes are known to regulate salivary gland migration and/or lumen size (); however, it is only recently that we have begun to analyze how control of salivary gland migration is coordinated with control of lumen size. To understand coordinated control of salivary gland migration and lumen size, we analyzed the role of the single Drosophila Rho GTPase, Rho1, in salivary gland tubulogenesis. In addition to the requirement of Rho1 in salivary gland invagination and migration (Xu et al., 2008), our recent studies show that Rho1 controls salivary gland lumen width and length during the migration process (). These studies reveal that Rho1-mediated processes at the proximal end of the migrating salivary gland, such as cell shape change, cell rearrangement and apical domain elongation, contribute to collective migration, and narrowing and elongation of the lumen.Citation4
Table 1. Genes that control salivary gland migration and/or lumen size
Figure 1. Rho1 is required for salivary gland migration and correct lumen size. In Rho11B heterozygous embryos (A and B), the salivary gland lumen elongates and narrows at the proximal end as the gland migrates (A and B, arrows). In Rho11B homozygous embryos, the salivary gland fails to migrate and elongate, and lumen width is expanded throughout the gland (C, arrow). Embryos were stained for dCREB-A (green) to label salivary gland nuclei, E-cadherin (E-cad) (white) to label the lumen and β-galactosidase (not shown) to distinguish heterozygous from homozygous embryos. The lumen in all panels is outlined in red and shaded in red. Scale bar in A represents 5 μm.
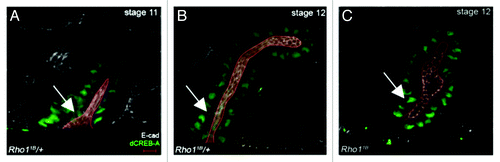
Rho1 Function in Actin Dynamics in Salivary Gland Cells
For many types of epithelia, the apical actin cytoskeleton and its associated proteins play a crucial role in numerous morphogenetic events. In the salivary gland, we found that Rho1 limits actin polymerization at the apical membrane and promotes actin polymerization at the basolateral membrane. In Rho1 zygotic loss of function mutants, excessive F-actin accumulates in the apical domain whereas F-actin is lost from the basolateral domain of salivary gland cells.Citation4 We quantified this difference in apical and basolateral F-actin by measuring the ratio of fluorescent intensity of apical to basolateral actin and observed an increase in the F-actin ratio in Rho1 mutant salivary gland cells. Thus, the salivary gland migration and lumen size defects observed in Rho1 mutant embryos may be due to perturbing this apical to basolateral actin ratio and/or the distinct enrichment of actin at the apical membrane and loss at the basolateral membrane.
Rho1 controls the differential localization of F-actin in salivary gland cells through Rho-kinase (Rok)-dependent inhibition of the actin-severing protein Cofilin. However, it is also possible that Rho1 simultaneously promotes actin polymerization by affecting Profilin, an actin polymerizing protein, either directly or indirectly. Although it is not clear how Rho1-regulated basolateral F-actin contributes to salivary gland collective migration and lumen size control, we hypothesize that it may regulate the transmission of forces within the epithelium to coordinate salivary gland morphogenesis. Although levels of the cell-cell adhesion protein, E-cadherin, are not changed in Rho1 mutant salivary gland cells, Rho1-dependent regulation of the basolateral F-actin cytoskeleton may indirectly affect E-cadherin activity at adhesion sites.
Independent of Rok-mediated regulation of Cofilin, Rho1 regulates actin dynamics at the apical membrane through downregulation of Moesin (Moe), an Ezrin-Radixin-Moesin family protein that links the actin cytoskeleton to the plasma membrane.Citation4 This regulation of Moe by Rho1 is necessary for the apical domains of salivary gland cells to elongate and for the proximal gland lumen to narrow. Rho1 regulates Moe in parallel to Ribbon (Rib), a BTB/POZ-domain transcription factor required for salivary gland elongation.Citation5,Citation6 Based on computer modeling, it is thought that rib mutant salivary glands fail to elongate because of increased stiffness of the apical membrane.Citation7 Thus, Rho1 may act in a similar manner to Rib whereby the presence of increased apical F-actin and apical active Moe in Rho1 mutant salivary gland cells makes the apical membrane stiff and prevents apical membrane remodeling and narrowing of the gland lumen.
Rho1-dependent Cellular Rearrangements at the Proximal Salivary Gland
Salivary gland cells in the proximal region of the gland are the last group of cells to invaginate from the embryo surface. After invagination, the epithelium of the proximal gland consists of irregularly shaped cells and appears pseudostratified with nuclei located at different positions along the apical-basal length of the cell (). As the salivary gland migrates in a dorsal direction, the proximal gland cells change shape from columnar to cuboidal, rearrange, transition from a pseudostratified to a simple epithelium and elongate their apical domains in the direction of migration ( and ). We show that salivary gland migration and lumen size defects of Rho1 mutant embryos are accompanied by defects in each of these processes.Citation4 Furthermore, tissue-specific rescue experiments indicate that Rho1 acts cell-autonomously to regulate these processesCitation4 and non-autonomously in the surrounding mesoderm-derived tissues for proper migration of the gland.Citation8 Thus, we are only beginning to understand these Rho1-regulated dynamic processes at the proximal end of the salivary gland and their role in collective migration and lumen size control. Future studies using live-imaging with multi-photon microscopy and 3D reconstruction of these processes are necessary to gain a better understanding of how these distinct processes are coordinated temporally and spatially to result in collective migration of the gland and correct lumen size. It is also necessary to identify regulatory proteins of each of these processes and the underlying molecular mechanisms. Such studies are bound to yield significant insight not only into Drosophila salivary gland morphogenesis but also into similar types of cell rearrangements that drive development of the vertebrate kidney and mammary gland.Citation9,Citation10
Figure 2. Salivary gland cells rearrange and transition from a pseudostratified to a simple epithelium during collective migration. In wild-type embryos (A-C), salivary gland cells rearrange as indicated by a decrease in the number of nuclei that surrounds the lumen. In Rho11B homozygous embryos at stage 12 (D), salivary gland cells fail to rearrange. In salivary glands of stage 11 wild-type embryos (E), the epithelium is pseudostratified with nuclei located at different positions along the length of the cell. By stage 12 in wild-type embryos (F), the salivary gland epithelium has transitioned to a simple epithelium with nuclei located at a similar position in all cells. All embryos shown were stained for dCREB-A (green in A-D; red in E and F) to label salivary gland nuclei; embryos in A-D were also stained for E-cadherin (E-cad; white) and those in E and F for aPKC (green) to label the lumen and Neurotactin (Nrt; white) to label the basolateral membrane. Scale bar in A represents 3 μm.
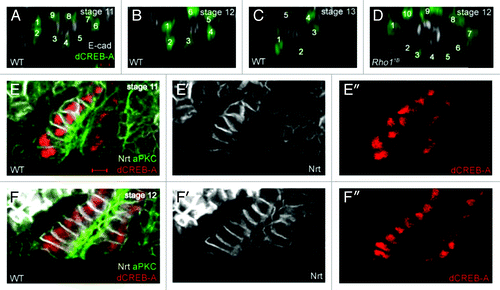
Figure 3. Cellular processes that control salivary gland migration and lumen size. At stage 11, all salivary gland cells have invaginated and the gland is oriented dorsally. Cells in the proximal part of the gland are columnar shaped with isotropic apical domains, and form a pseudostratified epithelium with nuclei at different positions along the apical-basolateral axis of the cell (A). As the salivary gland migrates dorsally at stage 12, the distal tip contacts the overlying circular visceral mesoderm (CVM) and proximal gland cells begin to change shape from columnar to cuboidal (B). It is also during stage 12 that proximal gland cells transition from a pseudostratified to a simple epithelium, apical domains elongate in the direction of migration (B) and cells rearrange to form a narrower tube (C). By stage 13, distal gland cells extend basal membrane protrusions as they migrate along the overlying CVM and underlying fat body (FB) and somatic mesoderm (SM) (D). Salivary gland cells are depicted in green and salivary duct cells in red. D: dorsal; V: ventral; A: anterior; P: posterior. Di: distal; Pr: proximal. VE: ventral ectoderm; VNC: ventral nerve cord; NB: neuroblasts. Diagram is not drawn to scale.
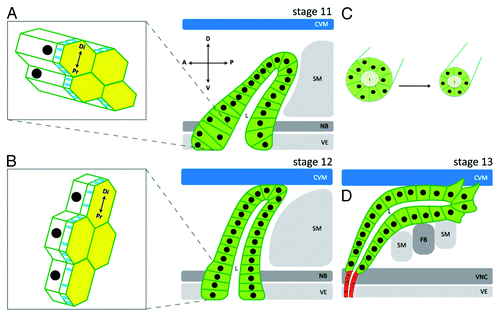
Salivary Gland Lumen Width and Collective Migration are Controlled by Distinct Mechanisms
Because the salivary gland migration defect occurs concomitantly with lumen size defects in Rho1 mutant embryos, one might predict that salivary gland migration and lumen size are controlled by the same mechanisms and that lumen size depends on extent of gland migration. However, this does not appear to be the case as demonstrated by our studies of embryos mutant for the serine-threonine kinase, p21-activated kinase (Pak1) (). In embryos mutant for a null allele of Pak1, the salivary gland migrates normally; however, lumen width in the medial gland is expanded.Citation3 This phenotype demonstrates that control of salivary gland migration and lumen width can be genetically separated. This conclusion is also supported by our tissue-specific rescue studies of Rho1 mutant embryos showing that expression of wild-type Rho1 specifically in the salivary gland rescues the cell rearrangement and apical domain elongation defects, whereas expression of wild-type Rho1 in the mesoderm does notCitation4 even though it rescues gland migration.Citation8 Moreover, our recent unpublished observations of embryos mutant for the integrin αPS1 subunit, encoded by multiple edematous wings (mew), suggest that control of lumen length is dependent on extent of salivary gland migration whereas lumen width is not (C. Pirraglia and M.M.Myat, unpublished observations). These studies suggest that not only is salivary gland migration and lumen width regulated by distinct mechanisms, but regulation of lumen length and lumen width also appear to be distinct. Thus, the embryonic salivary glands provide a unique model system to dissect cellular and molecular mechanisms by which collective migration and lumen size are controlled in a developing tubular organ.
References
- Pirraglia C, Myat MM. Genetic Regulation of Salivary Gland Development in Drosophila melanogaster. in Salivary Glands: Development, Adaptations and Disease., Vol. 14 (eds. Tucker, A. & Miletich, I.) 32-47 (S. Karger, 2010).
- Maruyama R, Andrew DJ. Drosophila as a model for epithelial tube formation. Dev Dyn 2012; 241:119 - 35; http://dx.doi.org/10.1002/dvdy.22775; PMID: 22083894
- Pirraglia C, Walters J, Myat MM. Pak1 control of E-cadherin endocytosis regulates salivary gland lumen size and shape. Development 2010; 137:4177 - 89; http://dx.doi.org/10.1242/dev.048827; PMID: 21068057
- Xu N, Bagumian G, Galiano M, Myat MM. Rho GTPase controls Drosophila salivary gland lumen size through regulation of the actin cytoskeleton and Moesin. Development 2011; 138:5415 - 27; http://dx.doi.org/10.1242/dev.069831; PMID: 22071107
- Bradley PL, Andrew DJ. ribbon encodes a novel BTB/POZ protein required for directed cell migration in Drosophila melanogaster.. Development 2001; 128:3001 - 15; PMID: 11532922
- Kerman BE, Cheshire AM, Myat MM, Andrew DJ. Ribbon modulates apical membrane during tube elongation through Crumbs and Moesin. Dev Biol 2008; 320:278 - 88; http://dx.doi.org/10.1016/j.ydbio.2008.05.541; PMID: 18585700
- Cheshire AM, Kerman BE, Zipfel WR, Spector AA, Andrew DJ. Kinetic and mechanical analysis of live tube morphogenesis. Dev Dyn 2008; 237:2874 - 88; http://dx.doi.org/10.1002/dvdy.21709; PMID: 18816822
- Xu N, Keung B, Myat MM. Rho GTPase controls invagination and cohesive migration of the Drosophila salivary gland through Crumbs and Rho-kinase. Dev Biol 2008; 321:88 - 100; http://dx.doi.org/10.1016/j.ydbio.2008.06.007; PMID: 18585373
- Chi X, Michos O, Shakya R, Riccio P, Enomoto H, Licht JD, et al. Ret-dependent cell rearrangements in the Wolffian duct epithelium initiate ureteric bud morphogenesis. Dev Cell 2009; 17:199 - 209; http://dx.doi.org/10.1016/j.devcel.2009.07.013; PMID: 19686681
- Ewald AJ, Brenot A, Duong M, Chan BS, Werb Z. Collective epithelial migration and cell rearrangements drive mammary branching morphogenesis. Dev Cell 2008; 14:570 - 81; http://dx.doi.org/10.1016/j.devcel.2008.03.003; PMID: 18410732
- Chandrasekaran V, Beckendorf SK. Tec29 controls actin remodeling and endoreplication during invagination of the Drosophila embryonic salivary glands. Development 2005; 132:3515 - 24; http://dx.doi.org/10.1242/dev.01926; PMID: 16000381
- Bradley PL, Myat MM, Comeaux CA, Andrew DJ. Posterior migration of the salivary gland requires an intact visceral mesoderm and integrin function. Dev Biol 2003; 257:249 - 62; http://dx.doi.org/10.1016/S0012-1606(03)00103-9; PMID: 12729556
- Myat MM, Andrew DJ. Epithelial tube morphology is determined by the polarized growth and delivery of apical membrane. Cell 2002; 111:879 - 91; http://dx.doi.org/10.1016/S0092-8674(02)01140-6; PMID: 12526813
- Pirraglia C, Jattani R, Myat MM. Rac function in epithelial tube morphogenesis. Dev Biol 2006; 290:435 - 46; http://dx.doi.org/10.1016/j.ydbio.2005.12.005; PMID: 16412417