Abstract
Individuals within species and populations vary. Such variation arises through environmental and genetic factors and ensures that no two individuals are identical. However, it is clear that not all traits show the same degree of intraspecific variation. Some traits, in particular secondary sexual characteristics used by males to compete for and attract females, are extremely variable among individuals in a population. Other traits, for example brain size in mammals, are not. Recent research has begun to explore the possibility that the extent of phenotypic variation (here referred to as “variability”) may be a character itself and subject to natural selection. While these studies support the concept of variability as an evolvable trait, controversy remains over what precisely the trait is. At the heart of this controversy is the fact that there are very few examples of developmental mechanisms that regulate trait variability in response to any source of variation, be it environmental or genetic. Here, we describe a recent study from our laboratory that identifies such a mechanism. We then place the study in the context of current research on the regulation of trait variability, and discuss the implications for our understanding of the developmental regulation and evolution of phenotypic variation.
Variability and Sources of Variation
Any discussion of variation must first define what variation is. Broadly speaking there are two sources of phenotypic trait variation: genetic and environmental.Citation1 Genetic variation is evident as phenotypic variation among individuals with different genotypes reared in identical environments. Environmental variation is evident as phenotypic variation among individuals with identical genotypes reared in different environments, also known as phenotypic plasticity. Traits that have evolved to be resistant to these sources of variation are said to be genetically and environmentally canalized.Citation2-Citation4 In addition to genetic and environmental sources of variation, however, there is also variation that arises from stochastic developmental processes among individuals with identical genotypes reared in the same environment.Citation4-Citation6 This variation is called developmental instability and is evident as fluctuating asymmetry (FA) in bilaterally symmetrical individuals.Citation6 Traits that have evolved to be resistant to developmental instability are said to be developmentally stable.Citation4 A useful perspective on the relationship between developmental instability and phenotypic plasticity is that they are a response to micro- and macroenvironmental perturbation, respectively.Citation4
An ongoing question in the study of variability is whether the same buffering mechanisms that regulate genetic variability also regulate environmental variability and developmental instability.Citation7-Citation11 Data from our laboratory is equivocal on this issue. We have shown that environmental variability in trait size in Drosophila depends on the source of environmental variation.Citation12 For example, wing size is much more variable in response to changes in developmental temperature (smaller at higher temperatures) than to changes in developmental nutrition (smaller at lower nutrition), when normalized against variation in overall body size.Citation12 Thus there does not appear to be a single buffering mechanism for wing size. On the other hand, male genital size shows low levels of variability in response to both sources of environmental variation and also in response to genetic variation, supporting a common mechanism.Citation13 To muddy the waters further, wing size is more developmental stable, and shows lower levels of fluctuating asymmetry, than genital size.Citation13
The ambiguity of our data concerning the existence of a universal buffering mechanism reflects the wider literature, with some studies supporting the hypothesisCitation9,Citation11,Citation14 and others rejecting it.Citation7,Citation8,Citation15 These studies (ours included) are essentially correlative, comparing and contrasting variability in different traits in response to different environmental and genetic perturbations. What is needed is an elucidation of the developmental mechanisms that regulate trait variability. Once these mechanisms are identified it will be possible to determine whether they regulate trait variability in response to different environmental and genetic factors, and to stochastic developmental processes.
The Developmental Regulation of Nutritional Variability
Perhaps the best studied form of environmental variation is the positive relationship between developmental nutrition and adult body and organ size: it is almost universally true among animals that malnutrition during development results in a reducing in adult body size.Citation16,Citation17 Not only is the phenomenon extremely evolutionarily conserved, but so too are the developmental mechanisms that regulate the response. From nematodes to humans, developmental nutrition affects body and organ size via the insulin/IGF-signaling (IIS) pathway ().Citation19-Citation24 Nevertheless, not all traits show the same variability in response to variation in developmental nutrition. Some organs, for example the human brain, are able to protect themselves from the effects of malnutrition and grow to more or less the same size in both well fed and poorly fed individuals.Citation12,Citation16 Our hypothesis was that an organ’s nutritional sensitivity is regulated by its individual response to changes in IIS.Citation25
Figure 1. Key components of the insulin-signaling pathway in Drosophila. The pathway regulates growth by suppressing the activity of the transcription factor FOXO. When nutrition and IIS is low FOXO is activated and targets the expression of growth inhibitors. For a more detailed version of the IIS in Drosophila see www.reactome.org.Citation18
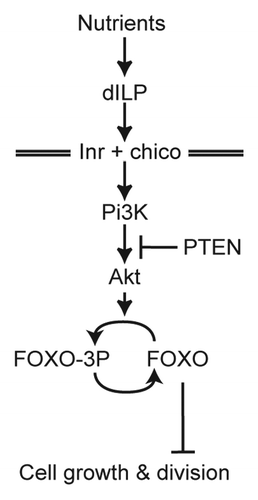
We used the different adult organs of Drosophila to test this hypothesis, comparing size regulation of the nutritionally plastic wings with size regulation of the nutritionally implastic male genitalia.Citation12,Citation25 Because adult insects have a hard exoskeleton, the size of the adult organs is controlled by their growth during the feeding larval stages. In holometabolous insects, adult organs grow as imaginal discs within the developing larva, which are sacks of cells sequestered from the epidermis during embryogenesis. For each adult organ there is a corresponding imaginal disc. We therefore compared how nutrition and IIS regulated growth in the wing imaginal disc compared with the male genital imaginal disc.Citation26
Our first experiment was to establish whether the low nutritional variability of the genitalia reflected their relative insensitivity to changes in IIS. To test this we examined flies that were mutant for the insulin-receptor (Inr). Mutations of the insulin receptor (Inr) genocopy starvation and, consistent with our hypothesis, caused a more substantial reduction in final wing size than final genital size. This corresponded to the differential effects of the mutation on the rate of cell division in the wing and genital imaginal discs. These data verified that the nutritional insensitivity of the genitalia reflected the insulin-insensitivity of the growing genital discs. Perhaps more importantly, they also indicated the mechanisms that account for this insensitivity act downstream of Inr in the IIS pathway. Such mechanisms modify systemic inputs into the IIS into organ-specific outputs. It follows that one can identify where in the IIS these mechanisms act by perturbing the pathway at different points and asking whether the growth effects are the same or different across all organs. If they are the same then we have perturbed the pathway above the mechanisms that regulate insulin sensitivity. If they are different then we have not.
Using this logic we were able to show that the mechanisms that regulate the nutritional and insulin-insensitivity of the genitalia act at FOXO in the pathway. FOXO is a transcription factor that, when active, targets the expression of negative growth regulators. However, it is itself suppressed by IIS, through phosphorylation by Akt, so that it can only slow growth when nutrition and IIS activity are low. A simple analogy is that growth is a car driving down a freeway, and FOXO is the foot brake, which when applied in low nutritional conditions slows the car down. Our experiments showed that the reason that the genitals are insensitive to changes in nutrition and IIS activity is because they only express very low levels of FOXO. In other words, they do not apply the “foot brake” on growth in low nutritional conditions because they do not have the foot brake. Finally, to confirm that it was indeed FOXO expression that regulated different organs’ response to changes in nutrition, we showed that changes in FOXO expression was sufficient to change an organ’s nutritional variability, while complete loss of FOXO expression eliminated differential nutritional variability among organs.
A General Mechanism for Regulating Variability?
The regulation of nutritional plasticity by FOXO expression adds to a very short list of developmental mechanisms that regulate trait variability. Perhaps the most well-known member of this list is the heat-shock protein HSP90, a chaperone that keeps unstable signaling proteins ready for activation and signal transduction.Citation27,Citation28 Mutation of HSP90 in flies destabilizes these proteins and makes activation of their signaling pathways more susceptible to genetic and environmental perturbation. Consequently, mutations and environmental stresses that would previously have been hidden by HSP90 are revealed, resulting in an increase in the production of abnormal traits and phenotypic variability.Citation27-Citation30
Similar to HSP90, microRNAs (miRNAs) are also thought to reduce phenotypic variability, specifically by increasing developmental stability through buffering stochastic perturbations in gene expression. miRNAs are short single stranded RNA molecules about 19–24 base pairs long that bind to complementary sequences in the 3′-UTRs of target mRNAs. The result is a double-stranded RNA molecule that is eliminated by the RNA-induced silencing proteins (RISC) complex, downregulating the expression of the target mRNA. miRNAs are thought to buffer gene expression by providing a negative-feedback loop or an incoherent feed-forward loop to a signaling pathway.Citation31 An example of this, again in Drosophila, is the miRNA miR-7, which stabilizes the expression of genes involved in specifying sensory organ precursor cells in various organs including the eye and antenna.Citation32 As for HSP90, loss of miR-7 increases the occurrence of abnormal phenotypes in flies subjected to environmental perturbations. Increases in abnormal phenotypes are also seen in flies lacking miR-263a/b.Citation33 Whether miRNAs also buffer against genetic perturbations is unknown.
A third mechanism that regulates trait variability has recently be discovered, also in Drosophila. Like the male genitalia, the size of the central nervous system (CNS) in developing Drosophila larvae is also resistant to changes in nutrition and IIS. However, in this case, the decreased sensitivity is because downstream components of the IIS pathway are activated by a receptor tyrosine kinase other than Inr.Citation34 This receptor, anaplastic lymphoma kinase (Alk), responds to its ligand Jelly Belly (Jeb) that is synthesized in a nutrition independent manner. The result is that CNS growth continues even when a larva is starved.Citation34
So what do these mechanisms tell us about the existence of a universal buffering mechanism? At first glance, it appears that they do not support the hypothesis. While HSP90 and miRNAs likely buffer against signaling perturbations that are genetic, macroenvironmental and microenvironmental in origin, the same does not appear to be true for FOXO expression and Alk activity, which ostensibly regulate trait variability in response to macroenvironmental variation (nutrition) alone. However, the types of trait variability regulated by HSP90/miRNA and FOXO/Alk appear to be qualitatively different. FOXO and Alk regulate trait variability that is “normal” and where the variation is an appropriate and evolved response to the environment, for example reduced organ size at low nutrition. In contrast, HSP90 and miRNAs appear to regulate trait variability that is “abnormal” and is not an appropriate or evolved response to the environment, for example the effect of temperature on the penetrance of a deformed-eye trait.Citation27,Citation35 Thus there may exist general buffering mechanisms against “abnormal” variation but more particular mechanisms against “normal” variation. Of course, what is “normal” and “abnormal” variation is a difficult question, but I suggest that ‘normal’ variation is generated and maintained by natural selection, for example phenotypic plasticity, while “abnormal” variation is almost always opposed by natural selection, for example congenital birth defects. This view is supported by data showing that mutation of HSP90 in Drosophila increases the variability of traits that are typically invariant among individuals (for example scutellar bristle number) but does not affect the variability of traits that are typically variant among individuals (for example sternopleural bristle number).Citation35
Having said this, it is unlikely that “normal” buffering mechanisms, for example reduced FOXO expression, reduce trait variability solely in response to a single environmental or genetic factor. Indeed, changes in FOXO expression are likely to influence a traits response to a variety of environmental factors. FOXO sits at the nexus of a suite of signaling pathways, including JNK signalingCitation36 and Hippo signaling,Citation37 each of which has some environmental input. Thus reduced FOXO expression may not only reduce the response to the genitals to changes in developmental nutrition, but other environmental factors also. Further, there is evidence that genetic variation in body and organ size is mediated by allelic variation in the insulin signaling pathway and other pathways that are mediated by FOXO.Citation38 Consequently, reduced FOXO expression may also reduce trait variation in response to these genetic factors. More generally, if genetic variation in body and organ size is primarily mediated by genes involved in the environmental regulation of size, then (macro)environmental and genetic canalization may reflect the same developmental processes.
There is some correlative evidence that this is the case. The genitalia of Drosophila not only show reduced variability to changes in nutrition, but also temperature.Citation12 They also show reduced variability in response to genetic variation, relative to other organs.Citation13 Importantly, this reduction in variability is only in response to genetic variation that acts systemically, that is variation that affects the size of all organs in the body in a coordinated manner.Citation13 Such variation may result from, for example, allelic variation in the IIS pathway. In contrast, the genitals do not show reduced variability in response to genetic factors that affect organ size autonomously, that is variation that is not correlated with variation in the size of any other organ.Citation13 Such variation may result from, for example, allelic variation in the genes that pattern specific organs. The genitalia also do not show reduced levels of developmental instability.Citation13 This is somewhat surprising, since developmental instability may arise through stochastic changes in the signaling pathways mediated by FOXO, and so reduced FOXO expression might be expected to mitigate the effects of these changes also. Nevertheless, it seems likely that, while not completely general, the mechanisms that regulate nutritional variability also regulate variability in response to other genetic and environmental factors.
Finally, of the mechanisms that regulate trait variability listed above, FOXO expression is the only one that is an integral part of the signaling pathway that it canalizes. In contrast, HSP90, miRNAs and Alk are additions to, and lie outside of the pathways that they canalize. It has been suggested that genes important for reducing developmental instability lie at the hubs of complex signaling networks.Citation39,Citation40 Indeed, the two best examples of genes whose miss-expression elevates fluctuating asymmetry, NotchCitation41 and Cyclin G 39, appear to fit this critereon. As mentioned above, FOXO also sits at the hub of a number of developmentally important signaling pathways. We have not assayed the effect of FOXO expression on fluctuating asymmetry and developmental instability. Neither has the effect of Notch or Cyclin G expression on genetic and environmental canalization been measured. However, collectively these observations support the hypothesis that trait variability in general is regulated by the expression of genes at the center of the signaling networks that underlie trait development.
The Evolution of Trait Variability
The evolutionary implications of canalization and developmental stability are myriad and have been dealt with extensively elsewhere. However, one evolutionary aspect of trait variability that is of particular interest is its role in controlling morphological scaling relationships. Indeed, it was the desire to elucidate the developmental mechanisms that regulate scaling that was the impetus for our study on the regulation of organ plasticity.
Morphological scaling relationship describe that relationship between organ size and body size as both vary among individuals within a species or population (formally referred to as static allometry).Citation42 Such scaling relationships regulate body proportion and shape, and it is no exaggeration to state that the evolution of morphology is the evolution of scaling relationships. While most organs scale proportionally with body size (a phenomenon called isometry), some traits are relatively larger in large individuals (hyperallometry) while others are relatively smaller (hypoallometry). It is a truism to state that, insomuch as organ size variation is coordinated across the body, traits that are more variable relative to other traits and body size are hyperallometric, while traits that are less variable are hypoallometric.Citation42
The relationship between trait variability and morphological scaling relationships provides an important perspective on the selective pressures that underlie the evolution of trait variability. For example, the elevated level of variability in secondary sexual characteristics may be a consequence of selection for hyperallometry in those traits. Because hyperallometric traits are proportionally larger in large individuals, body shape can be used as an indication of body size. If a male’s size is in turn an indication of his condition, then the relative size of a hyperallometric trait reflects this and can be used by females to select the fittest mate. As long as a trait is hyperallometric then it will, by definition, be an intrinsically honest indicator of body size and its hyperallometry should be favored by selection.Citation43 Cheating, that is the production of a proportionally large trait in small individuals, would necessarily eliminate the hyperallometry of the trait, render it uninformative and hence relax selection for elevated trait variability. Consequently, exaggerated secondary sexual characteristics—such as the disproportionately large antlers of the Irish elk, the bizarrely wide eyes of the stalk-eyed fly, and the elongated tail feathers of the widow bird—may evolve through selection by females not on disproportionally bigger traits per se, but on hyperallometric traits which are disproportionally bigger in larger males.Citation43
Our study provides a specific mechanism—insulin sensitivity—that may be the proximate target of selection by females on hyperallometry. Although our research concentrated on the hypoallometric genitalia, we also showed that an increase in FOXO expression in the wing increased its nutritional variability and made it hyperallometric to body size. This hyperallometry was achieved by making the wing disproportionally small in smaller individuals, relative to controls. (). This does not appear to be the pattern observed in many examples of hyperallometric traits, which are exaggerated in larger individuals . Nevertheless, additional data suggests a mechanism for this pattern. We found that increasing the expression of Inr also increased the nutritional variability of the wing. In this case, however, the resulting hyperallometry was achieved by making the wings disproportionally larger in large individuals, that is exaggerated, relative to controls (). Because the IIS pathway is so conserved, one can imagine that the evolution of exaggerated traits in other animals was a consequence of selection acting on the same or similar mechanisms. Indeed, the exaggerated horns of the Japanese rhinoceros beetle show elevated levels of insulin sensitivity,Citation43 while IIS appears necessary for the growth of exaggerated soldier morphologies in termitesCitation44 and for antler growth in red and fallow deer.Citation45,Citation46
Figure 2. Organ-autonomously altering the expression of IIS genes affects an organ’s nutritional variability and its scaling relationship with body size. (A) Increasing FOXO expression in the wing using an imaginal-disc specific driver (NP6333 > FOXO.wt) makes the wing significantly more hyperallometric to body size, by decreasing wing size in smaller individuals (data from Figure 5 Tang et al.,Citation26 common slope test, p < 0.01) (B) Increasing Inr expression (NP6333 > Inr) also makes the wing significantly more hyperallometric but by increasing wing size in larger individuals (common slope test, p < 0.01). Flies were reared and statistical analysis conducted as described in Tang et al.Citation26 Flies for Inr overexpression were from the Bloomington Stock Center (stock no. 8262). All lines are standardized major axes.
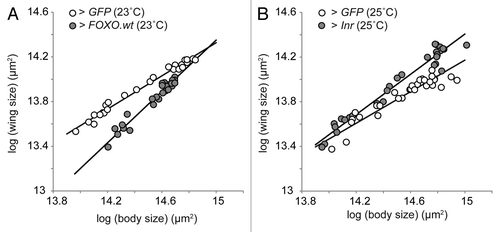
One final point worth noting is that, because the mechanisms that regulate a trait’s nutritional variability may also regulate its variability in response to genetic factors, changes in a trait’s insulin sensitivity may provide a mechanistic solution to the “lek paradox.” A long standing question in evolutionary biology is why, if females prefer a male trait that indicates condition (for example, exaggerated antlers), sexual selection does not exhaust genetic variation in the trait and drive it to fixation.Citation47 Since the utility of the trait as an indicator of male condition lies in its variability, how can a trait be both an indicator of a male’s genetic condition and be strongly selected by females? This is the paradox.Citation47 A theoretical solution to the paradox is the “genic capture hypothesis”: that male traits are dependent on many underlying physiological and developmental processes such that they capture variation over multiple loci and so are resistant to the erosive effects of strong directional selection on genetic variation.Citation48,Citation49 Because changes in FOXO expression may influence a trait’s variability in response to allelic variation in possibly a large number of signaling pathways, FOXO may be a regulator of genic capture.
Future Directions
The relationship between trait variability, genetic and environmental canalization and developmental stability remains contentious, but it is clear that a mechanistic understanding of how variability is regulated at a developmental level is central to resolving ongoing controversies. Further, as the example of the evolution of morphological scaling relationships illustrates, a mechanistic understanding of how trait variability is regulated illuminates how trait variability evolves. Consequently, further examples of mechanisms that regulate trait variability are needed, as is a deeper understanding of the few examples that already exist. With regards to IIS and trait variability, ongoing studies should explicitly test the hypothesis that changes in FOXO expression regulate a trait’s genetic variability and hence control genic capture. Further, comparative studies in other animals should continue to determine whether variation in insulin sensitivity is a general mechanism that regulates trait variability and morphological scaling relationships, particularly of hyperallometric traits. Finally, we should incorporate mechanistic understanding of trait variability into evolutionary models of how variability evolves and how it influences phenotypic evolution.
Acknowledgments
We are grateful to S. G. Modak and R.C. Stillwell for comments on early drafts of this manuscript. This work was supported by the National Science Foundation under Grant No. IOS-0845847 and Cooperative Agreement No. DBI-0939454.
References
- Falconer DS, Mackay TF. Introduction to Quantitave Genetics. New York: The Ronald Press Company, 1996.
- Waddington CH. Canalization of development and the inheritance of acquired characters. Nature 1942; 150:536 - 65; http://dx.doi.org/10.1038/150563a0
- Flatt T. The evolutionary genetics of canalization. Q Rev Biol 2005; 80:287 - 316; http://dx.doi.org/10.1086/432265; PMID: 16250465
- Debat V, David P. Mapping phenotypes: canalization, plasticity and developmental stability. Trends Ecol Evol 2001; 16:555 - 61; http://dx.doi.org/10.1016/S0169-5347(01)02266-2
- Dongen SV. Fluctuating asymmetry and developmental instability in evolutionary biology: past, present and future. J Evol Biol 2006; 19:1727 - 43; http://dx.doi.org/10.1111/j.1420-9101.2006.01175.x; PMID: 17040371
- Klingenberg C, Nijhout H. Genetics of fluctuating asymmetry: A developmental model of developmental instability. Evolution 1999; 53:358 - 75; http://dx.doi.org/10.2307/2640773
- Pélabon C, Hansen TF, Carter AJ, Houle D. Evolution of variation and variability under fluctuating, stabilizing, and disruptive selection. Evolution 2010; 64:1912 - 25; PMID: 20199560
- Debat V, Debelle A, Dworkin I. Plasticity, canalization, and developmental stability of the Drosophila wing: joint effects of mutations and developmental temperature. Evolution 2009; 63:2864 - 76; http://dx.doi.org/10.1111/j.1558-5646.2009.00774.x; PMID: 19624729
- Breuker CJ, Patterson JS, Klingenberg CP. A single basis for developmental buffering of Drosophila wing shape. PLoS One 2006; 1:e7; http://dx.doi.org/10.1371/journal.pone.0000007; PMID: 17183701
- Klingenberg CP. A developmental perspective on developmental insta- bility: theory, models and mechanisms. In: Polak M, ed. Developmental Instability: causes and consequences. Oxford, UK: Oxford Univ. Press, 2003.
- Willmore KE, Klingenberg CP, Hallgrímsson B. The relationship between fluctuating asymmetry and environmental variance in rhesus macaque skulls. Evolution 2005; 59:898 - 909; PMID: 15926699
- Shingleton AW, Estep CM, Driscoll MV, Dworkin I. Many ways to be small: different environmental regulators of size generate distinct scaling relationships in Drosophila melanogaster. Proc Biol Sci 2009; 276:2625 - 33; http://dx.doi.org/10.1098/rspb.2008.1796; PMID: 19386657
- Dreyer AP, Shingleton AW. The effect of genetic and environmental variation on genital size in male Drosophila: canalized but developmentally unstable. PLoS One 2011; 6:e28278; http://dx.doi.org/10.1371/journal.pone.0028278; PMID: 22174784
- Clarke G. The genetic basis of developmental stability. V. Inter- and intra-individual character variation. Heredity 1998; 80:562 - 7; http://dx.doi.org/10.1046/j.1365-2540.1998.00294.x
- Rego C, Matos M, Santos M. Symmetry breaking in interspecific Drosophila hybrids is not due to developmental noise. Evolution 2006; 60:746 - 61; PMID: 16739456
- Guthrie HA, Brown ML. Effect of severe undernutrition in early life on growth, brain size and composition in adult rats. J Nutr 1968; 94:419 - 26; PMID: 5653258
- Robertson FW. Ecological Genetics of Growth in Drosophila. 6. Genetic Correlation between Duration of Larval Period and Body Size in Relation to Larval Diet. Genet Res 1963; 4:74 - 92; http://dx.doi.org/10.1017/S001667230000344X
- Croft D, O’Kelly G, Wu G, Haw R, Gillespie M, Matthews L, et al. Reactome: a database of reactions, pathways and biological processes. Nucleic Acids Res 2011; 39:Database issue D691 - 7; http://dx.doi.org/10.1093/nar/gkq1018; PMID: 21067998
- Clemmons DR, Underwood LE. Nutritional regulation of IGF-I and IGF binding proteins. Annu Rev Nutr 1991; 11:393 - 412; http://dx.doi.org/10.1146/annurev.nu.11.070191.002141; PMID: 1716449
- Ikeya T, Galic M, Belawat P, Nairz K, Hafen E. Nutrient-dependent expression of insulin-like peptides from neuroendocrine cells in the CNS contributes to growth regulation in Drosophila. Curr Biol 2002; 12:1293 - 300; http://dx.doi.org/10.1016/S0960-9822(02)01043-6; PMID: 12176357
- Straus DS. Nutritional regulation of hormones and growth factors that control mammalian growth. FASEB J 1994; 8:6 - 12; PMID: 8299891
- Kimura KD, Tissenbaum HA, Liu Y, Ruvkun G. daf-2, an insulin receptor-like gene that regulates longevity and diapause in Caenorhabditis elegans. Science 1997; 277:942 - 6; http://dx.doi.org/10.1126/science.277.5328.942; PMID: 9252323
- Oldham S, Hafen E. Insulin/IGF and target of rapamycin signaling: a TOR de force in growth control. Trends Cell Biol 2003; 13:79 - 85; http://dx.doi.org/10.1016/S0962-8924(02)00042-9; PMID: 12559758
- Claeys I, Simonet G, Poels J, Van Loy T, Vercammen L, De Loof A, et al. Insulin-related peptides and their conserved signal transduction pathway. Peptides 2002; 23:807 - 16; http://dx.doi.org/10.1016/S0196-9781(01)00666-0; PMID: 11897402
- Shingleton AW, Das J, Vinicius L, Stern DL. The temporal requirements for insulin signaling during development in Drosophila. PLoS Biol 2005; 3:e289; http://dx.doi.org/10.1371/journal.pbio.0030289; PMID: 16086608
- Tang HY, Smith-Caldas MS, Driscoll MV, Salhadar S, Shingleton AW. FOXO regulates organ-specific phenotypic plasticity in Drosophila. PLoS Genet 2011; 7:e1002373; http://dx.doi.org/10.1371/journal.pgen.1002373; PMID: 22102829
- Rutherford SL, Lindquist S. Hsp90 as a capacitor for morphological evolution. Nature 1998; 396:336 - 42; http://dx.doi.org/10.1038/24550; PMID: 9845070
- Samakovli D, Thanou A, Valmas C, Hatzopoulos P. Hsp90 canalizes developmental perturbation. J Exp Bot 2007; 58:3513 - 24; http://dx.doi.org/10.1093/jxb/erm191; PMID: 18057034
- Queitsch C, Sangster TA, Lindquist S. Hsp90 as a capacitor of phenotypic variation. Nature 2002; 417:618 - 24; http://dx.doi.org/10.1038/nature749; PMID: 12050657
- Sollars V, Lu X, Xiao L, Wang X, Garfinkel MD, Ruden DM. Evidence for an epigenetic mechanism by which Hsp90 acts as a capacitor for morphological evolution. Nat Genet 2003; 33:70 - 4; http://dx.doi.org/10.1038/ng1067; PMID: 12483213
- Hornstein E, Shomron N. Canalization of development by microRNAs. Nat Genet 2006; 38:Suppl S20 - 4; http://dx.doi.org/10.1038/ng1803; PMID: 16736020
- Li X, Cassidy JJ, Reinke CA, Fischboeck S, Carthew RW. A microRNA imparts robustness against environmental fluctuation during development. Cell 2009; 137:273 - 82; http://dx.doi.org/10.1016/j.cell.2009.01.058; PMID: 19379693
- Hilgers V, Bushati N, Cohen SM. Drosophila microRNAs 263a/b confer robustness during development by protecting nascent sense organs from apoptosis. PLoS Biol 2010; 8:e1000396; http://dx.doi.org/10.1371/journal.pbio.1000396; PMID: 20563308
- Cheng LY, Bailey AP, Leevers SJ, Ragan TJ, Driscoll PC, Gould AP. Anaplastic lymphoma kinase spares organ growth during nutrient restriction in Drosophila. Cell 2011; 146:435 - 47; http://dx.doi.org/10.1016/j.cell.2011.06.040; PMID: 21816278
- Milton CC, Ulane CM, Rutherford S. Control of canalization and evolvability by Hsp90. PLoS One 2006; 1:e75; http://dx.doi.org/10.1371/journal.pone.0000075; PMID: 17183707
- Luo X, Puig O, Hyun J, Bohmann D, Jasper H. Foxo and Fos regulate the decision between cell death and survival in response to UV irradiation. EMBO J 2007; 26:380 - 90; http://dx.doi.org/10.1038/sj.emboj.7601484; PMID: 17183370
- Lehtinen MK, Yuan Z, Boag PR, Yang Y, Villén J, Becker EB, et al. A conserved MST-FOXO signaling pathway mediates oxidative-stress responses and extends life span. Cell 2006; 125:987 - 1001; http://dx.doi.org/10.1016/j.cell.2006.03.046; PMID: 16751106
- Sutter NB, Bustamante CD, Chase K, Gray MM, Zhao K, Zhu L, et al. A single IGF1 allele is a major determinant of small size in dogs. Science 2007; 316:112 - 5; http://dx.doi.org/10.1126/science.1137045; PMID: 17412960
- Rutherford S, Knapp JR, Csermely P. Hsp90 and developmental networks. Adv Exp Med Biol 2007; 594:190 - 7; http://dx.doi.org/10.1007/978-0-387-39975-1_16; PMID: 17205685
- Debat V, Bloyer S, Faradji F, Gidaszewski N, Navarro N, Orozco-Terwengel P, et al. Developmental stability: a major role for cyclin G in drosophila melanogaster. PLoS Genet 2011; 7:e1002314; http://dx.doi.org/10.1371/journal.pgen.1002314; PMID: 21998598
- Indrasamy H, Woods RE, McKenzie JA, Batterham P. Fluctuating asymmetry for specific bristle characters in notch mutants of Drosophila melanogaster. Genetica 2000; 109:151 - 9; http://dx.doi.org/10.1023/A:1017535930657; PMID: 11430479
- Shingleton AW, Frankino WA, Flatt T, Nijhout HF, Emlen DJ. Size and shape: the developmental regulation of static allometry in insects. Bioessays 2007; 29:536 - 48; http://dx.doi.org/10.1002/bies.20584; PMID: 17508394
- Emlen D, Warren I, Johns A, Dworkin I, Corley-Lavine L. Personal Communication. 2011.
- Miura T. Molecular Basis Underlying Caste Differentiation in Termites. In: Bignell DE, ed. Biology of Termites: A Modern Synthesis: Springer Netherlands, 2011.
- Suttie JM, Fennessy PF, Corson ID, Laas FJ, Crosbie SF, Butler JH, et al. Pulsatile growth hormone, insulin-like growth factors and antler development in red deer (Cervus elaphus scoticus) stags. J Endocrinol 1989; 121:351 - 60; http://dx.doi.org/10.1677/joe.0.1210351; PMID: 2754367
- Suttie JM, Gluckman PD, Butler JH, Fennessy PF, Corson ID, Laas FJ. Insulin-like growth factor 1 (IGF-1) antler-stimulating hormone?. Endocrinology 1985; 116:846 - 8; http://dx.doi.org/10.1210/endo-116-2-846; PMID: 3881250
- Kirkpatrick M, Ryan MJ. The evolution of mating preference and the paradox of the lek. Nature 1991; 350:33 - 8; http://dx.doi.org/10.1038/350033a0
- Tomkins JL, Radwan J, Kotiaho JS, Tregenza T. Genic capture and resolving the lek paradox. Trends Ecol Evol 2004; 19:323 - 8; http://dx.doi.org/10.1016/j.tree.2004.03.029; PMID: 16701278
- Rowe L, Houle D. The lek paradox and the capture of genetic variance by condition dependent traits. Proc R Soc Lond B Biol Sci 1996; 263:1415 - 21; http://dx.doi.org/10.1098/rspb.1996.0207