Abstract
Polycomb group (PcG) proteins were originally identified as negative regulators of HOX genes in Drosophila but have since emerged as a widely used transcriptional repression system that controls a variety of developmental processes in animals and plants. PcG proteins exist in multi-protein complexes that comprise specific chromatin-modifying enzymatic activities. Genome-wide binding studies in Drosophila and in mammalian cells revealed that these complexes co-localize at a large set of genes encoding developmental regulators. Recent analyses in Drosophila have begun to explore how the different chromatin-modifying activities of PcG protein complexes contribute to the repression of individual target genes. These studies suggest that monoubiquitination of histone H2A (H2Aub) by the PcG protein Sce is only essential for repression of a subset of PcG target genes but is not required for the Polycomb-mediated repression of other targets. Calypso/dBap1, a major deubiquitinase for H2Aub is also critically needed for repression of a subset of PcG target genes. Here, we review our current understanding of the role of H2A monoubiquitination and deubiquitination in Polycomb repression in Drosophila. We discuss unresolved issues concerning the immunological detection of H2Aub and critically evaluate experiments that used Sce and Ring1B point mutants with impaired H2A ubiquitinase activity to study H2Aub-dependent and -independent functions of these proteins in transcriptional repression.
Dissecting the Role of Polycomb Complex Subunits by Biochemistry and Genetics
PcG proteins were originally identified through genetic approaches in Drosophila. Biochemical studies subsequently established that in Drosophila these proteins comprise the subunits of at least four distinct multiprotein complexes: Polycomb repressive complex 1 (PRC1), Polycomb repressive complex 2 (PRC2), Polycomb repressive deubiquitinase (PR-DUB) and Pho repressive complex (PhoRC).Citation1-Citation7 Three of these complexes contain specific enzymatic activities for covalent modifications on histone proteins in nucleosomes. PRC2 is a histone methyltransferase that methylates histone H3 at lysine 27 (H3-K27me3), PRC1 and related PRC1-type complexes harbor an E3 ligase activity for the monoubiquitination of histone H2A (H2Aub), and PR-DUB is a deubiquitinase for H2Aub. For all three complexes, comparable or related protein assemblies are present in mammals.Citation8-Citation15 However, in mammals, the system tends to be more complex than in Drosophila. This is partly due to the existence of multiple paralogues for most complex subunits. Furthermore, some components of the mammalian complexes have evolved to bind additional proteins. For example, PR-DUB in Drosophila comprises Calypso (also called dBap1) and Asx () and a reconstituted orthologous human complex containing BAP1 and ASXL1 has the same H2Aub deubiquitinase activity like the Drosophila complex.Citation7 However, BAP1 complexes purified from mammalian cells contain not only ASXL1 or AXL2 but also several other proteins, including HCF-1Citation12–Citation14, and the association of HCF-1 with BAP1 was shown to be mediated by BAP1 sequences that are absent in the Drosophila Calypso/dBap1 protein.Citation12,Citation16 In this article, we focus on our current understanding of the role of H2A ubiquitination and deubiquitination in Drosophila and on how these activities contribute to the repression of target genes.
Figure 1. Drosophila PcG protein complexes involved in H2A ubiquitination and deubiquitination. (A) PRC1 and dRAF core complexes both contain the Sce:Psc subcomplex (dark orange) that has E3 ligase activity for H2A monoubiquitination (H2Aub). The presence of Psc or its paralogue Su(z)2 in both complexes suggests that both complexes are also able to compact chromatin. Subunits that are encoded by PcG genes are labeled in orange; Kdm2 mutants are not known to show PcG mutant phenotypes. PR-DUB (blue), containing the catalytic subunit Calypso/dBap1, is a major H2Aub deubiquitinase in Drosophila. See text for details. (B) Alignment of the C-termini of fly and human H2A showing the lysine that is ubiquitinated by PRC1-type complexes. Note that the C-terminal residues of H2A are different in flies and humans.
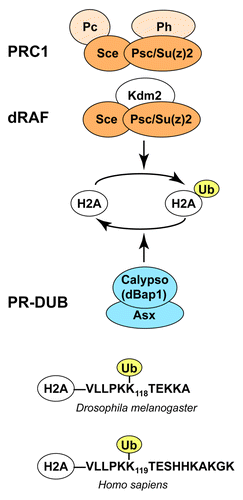
We start by discussing a few general aspects of the PRC1, PRC2 and PR-DUB enzyme complexes. Biochemical studies show that the non-catalytic subunits are critical for the enzymatic activity of these multi-protein complexes. Specifically, the catalytic subunit of each of these complexes exhibits only poor enzyme activity on nucleosomes in vitro and needs to be assembled with additional complex subunits to become fully active.Citation3,Citation7,Citation17-Citation19 At present, we still have only a limited understanding of how the non-catalytic subunits function to create an active enzyme. In the case of PRC2, there is experimental evidence that the non-catalytic subunits Suppressor of zeste 12 (Su(z)12) and Nurf55 are critical for docking the catalytic subunit Enhancer of zeste (E(z)) on the nucleosome substrate.Citation20 Furthermore, several of the non-catalytic subunits contain domains that recognize post-translational modifications (PTMs) present in nucleosomes. Specific PTMs are therefore thought to affect binding to the nucleosome substrate or modulate the enzymatic activity of the complex in a more intricate manner (e.g., refs. Citation21 and Citation22). The association of other complex subunits may also directly affect catalysis by inducing a conformational change in the catalytic subunit. However, at present there is no clearly established example for such an allosteric activation mechanism in any of these complexes. Structural studies of these complexes and of their interaction with nucleosomes will be required to fully understand the mechanisms that allow these enzymes to function on chromatin.
The essential role of the non-catalytic subunits for complex activity in vitro is strongly supported by genetic studies. Animals that lack non-catalytic subunits typically show phenotypes that are indistinguishable from those of mutants that lack the catalytic subunit of the complex. For example, PR-DUB comprises the catalytic subunit Calypso/dBap1 and its cofactor Asx ().Citation7 Calypso and Asx are both essential for H2A deubiquitination in vitro and in vivo, and animals lacking either protein show virtually identical morphological defects and misexpression of HOX target genes.Citation7 Similarly, animals that lack the PRC2 subunits E(z), Su(z)12 or the two paralogues Esc and Esc-like show comparable HOX misexpression phenotypes and complete loss of H3-K27 methylation.Citation3,Citation23-Citation26 This comparison of mutant phenotypes becomes more complicated in cases where a particular complex subunit is a component of several different chromatin complexes. For example, the PRC2 subunit Caf1 (also known as Nurf55) is also a core subunit of NURF, CAF-1 and several other chromatin-modifying complexes. Unlike cells that lack E(z) or Su(z)12, the cells that lack Caf1 cease to proliferate.Citation27
H2A Monoubiquitination and Non-covalent Chromatin Modification by PRC1 Complexes
In the case of PRC1 and the related dRAF complex (), the relationship between the phenotype of mutants lacking individual complex subunits and the chromatin-modifying activities of these protein complexes has been a long-standing puzzle. The core of Drosophila PRC1 comprises the proteins Sex combs extra (Sce; also known as dRing), Polycomb (Pc), the two highly related proteins Polyhomeotic-proximal (Ph-p) and Polyhomeotic-distal (Ph-d), and Posterior sex combs (Psc) or its paralogue Su(z)2 ().Citation1,Citation28-Citation31 The dRAF complex comprises Sce, Psc and KDM2 but lacks Ph and Pc ().Citation6 The Sce and Psc proteins form a stable dimeric complex that corresponds to the murine Ring1b:Bmi1 complex.Citation18,Citation19,Citation32 The Sce:Psc heterodimer therefore forms the core of both the PRC1 and the dRAF complex and it provides two distinct chromatin-modifying activities to theses complexes (). First, the Ring finger domains of Sce and of its mammalian orthologs Ring1b and Ring1a function as E3 ligases for the monoubiquitination of histone H2A in nucleosomes. This activity is greatly enhanced by the association with the Ring finger domain of Psc and Bmi1, respectively ().Citation6,Citation18,Citation19 Second, the C-terminal portion of Psc, an intrinsically disordered region, is responsible for the ability of PRC1 to inhibit nucleosome remodeling, repress transcription on chromatin templates and compact chromatin in vitro.Citation28,Citation30,Citation33,Citation34
Drosophila embryos that lack Psc protein (i.e., animals that are homozygous for a Psc null mutation and lack maternally-deposited Psc protein because they were obtained as progeny of females with Psc mutant germ cells) show severe developmental defects and lack most landmark structures in the embryonic cuticle.Citation35 Embryos that lack both Psc and its partially redundant paralogue Su(z)2 show even more extreme phenotypes; these animals arrest development during gastrulation and do not produce any embryonic cuticle.Citation36 These phenotypes are considerably more severe than the phenotype of Sce mutants. Sce mutant embryos undergo normal gastrulation and die at the end of embryogenesis with a fully developed cuticle showing the hallmark of the Polycomb phenotype: homeotic transformations of multiple body segments.Citation32,Citation36-Citation38 It is important to note that the differences between the Sce and Psc-Su(z)2 mutant phenotypes are not due to residual Sce+ activity in the Sce mutants. The Sce mutant phenotype described above was observed in animals homozygous for a molecularly defined Sce null allele with a deletion of the entire Sce coding region.Citation36 Hence, even though animals that lack Sce display severe homeotic phenotypes, they do not show the extreme developmental abnormalities that are observed when the Sce partner proteins Psc and Su(z)2 are absent. Psc-Su(z)2 evidently must be essential for processes and/or the regulation of target genes that do not require the function of Sce.
Consistent with the genetic data described above, Psc protein levels are undiminished in animals lacking Sce.Citation36 The Psc protein is therefore stable in the absence of its partner protein Sce. However, the Sce protein is unstable if it is not associated with Psc. A mutant Sce protein with a substitution in a Ring finger residue that is thought to be critical for the interaction with Psc and Su(z)Citation18,Citation19 is unstable and undetectable in Drosophila.Citation36 Furthermore, removal of Sce results in a drastic reduction of bulk H2Aub levels, suggesting that Sce is the main or even only E3 ligase that generates this modification in Drosophila.Citation6,Citation36
The constellation that removal of Sce abolishes H2A monoubiquitination but does not affect Psc protein levels has thus allowed analyzing the contribution of Sce and H2A monoubiquitination to the repression of PRC1 target genes and exploring whether and how Psc represses target genes independently of H2A monoubiquitination.
H2A Monoubiquitination Activity is Dispensable for Repression of a Large Fraction of PRC1-Regulated Genes
To study the role of H2A ubiquitination-dependent and -independent PRC1 repressor activities, Gutiérrez et al.Citation36 analyzed the expression of various PcG target genes in mutants that lack individual PRC1 subunits. The authors focused on genes that had been identified as being bound by Sce, Psc, Ph and Pc in genome-wide profiling studies in Drosophila tissue culture cellsCitation39 and in larvae.Citation36,Citation40 These studies revealed that PRC1-regulated genes fall into two distinct classes. Class I genes are derepressed in mutants lacking any of these four PRC1 subunits. Class II genes are only derepressed in mutants lacking the Psc/Su(z)2 and Polyhomeotic (Ph) proteins but remain stably repressed in the absence of the Sce and Polycomb (Pc) proteins. Repression of class II target genes therefore does not require Sce and H2A monoubiquitination but may depend on the chromatin compaction and remodeling inhibition activities of Psc-Su(z)2 and on the less well understood repressor function of Ph.Citation36 Two specific points should be emphasized in this context. First, the majority of the PRC1 target genes analyzed by Gutiérrez et al.Citation36 fall into class II. H2A ubiquitination-independent repression seems therefore to be a major mechanism by which PRC1 represses gene transcription. Second, the analyses reported by Gutiérrez et al.Citation36 were all performed in embryos and in imaginal disc tissues. It therefore remains possible that Sce and H2A monoubiquitination could still be required for repression of class II genes in other tissues or at particular developmental stages (e.g., in stem cells in adults). Nevertheless, the observation that Sce is targeted to a substantial fraction of PRC1 target genes that do not need it for their repression implies that Sce also monoubiquitinates H2A in the chromatin of genes that do not require this modification for repression.
Where is the H2Aub Modification Located in Drosophila Chromatin?
A major shortcoming in studying the role of H2A monoubiquitination in Polycomb repression has been the lack of an antibody that specifically recognizes this modification in Drosophila H2A. Earlier studies had found that a mouse monoclonal antibody called E6C5 specifically recognizes H2Aub in mammalian cells.Citation41 It is thought that this antibody selectively recognizes the branch point between H2A and ubiquitin. However, recent studies documented that this antibody lacks specificity for recognizing Drosophila H2Aub.Citation7 Specifically, the E6C5 antibody recognizes a multitude of protein bands in Drosophila nuclear extracts, and it recognizes recombinant Drosophila H2Aub and polyubiquitin chains with comparable efficiency (see Fig. S6 in ref. Citation7). One reason for this might be due to differences between mammalian and Drosophila H2A. The amino acid residues that flank the ubiquitinated lysine residue and form the C-terminus of H2A are different (). It is possible that these flanking residues form part of the epitope that is recognized by the E6C5 antibody and that the antibody therefore recognizes Drosophila H2Aub with lower affinity than human H2Aub. We therefore believe that earlier ChIP analyses that used the E6C5 antibody in DrosophilaCitation42 need to be interpreted with caution. Raising an antibody that recognizes Drosophila H2Aub with high specificity will be essential for being able to study the genomic localization of this modification by chromatin immunoprecipitation.
Steady-State Levels of H2Aub and H2Bub are Different in Embryos and Larvae
The lack of an antibody that specifically recognizes Drosophila H2Aub has limited the study of this modification in vivo to the examination of H2Aub bulk levels by western blot analyses with antibodies against unmodified H2A.Citation7,Citation36 These studies suggest that the fraction of H2A that is monoubiquitinated is substantially higher in larvae compared with embryos. Specifically, in acid extracted histones from wild-type Drosophila embryos, only about 1–2% of H2A is detected as being monoubiquitinated (see Fig. 2A in ref. Citation7). In contrast, in acid-extracted histones from larval imaginal disc tissues, H2Aub appears to comprise about 5–10% of total H2A (see Fig. 1D in ref. Citation36). Interestingly, the levels of the H2Bub show a comparable difference in these two developmental stages. In embryos, about 1–2% of total H2B appears as monoubiquitinated (see Fig. 2A in ref. Citation7), whereas in imaginal discs, about 5–10% of H2B is present as H2Bub.Citation43
The H2A Deubiquitinase PR-DUB is Essential for Repression of a Subset of Class I Polycomb Target Genes
The HOX, engrailed, no ocelli and elbow genes are categorized as class I genes because their repression requires Sce.Citation36 Repression of the HOX genes but not that of engrailed, no ocelli or elbow also requires PR-DUB.Citation7,Citation36 PR-DUB is thus apparently needed for repression of only a subset of class I target genes. Moreover, to date there is no evidence that PR-DUB would be required for repression of any of the class II target genes. Interestingly, genome-wide profiling studies found that PR-DUB nevertheless co-localizes with PRC1 not only at class I but also at many class II target genes.Citation7
PR-DUB is a major deubiquitinase for H2Aub in Drosophila (). Embryos that lack PR-DUB show a 10-fold increase in the bulk levels of H2Aub and die at the end of embryogenesis.Citation7 Like Sce, PR-DUB is required for repression of HOX genes both during embryogenesis and during larval development.Citation7,Citation44 Given that removal of the Sce E3 ligase that catalyzes H2Aub formation results in loss of HOX gene repression, it may seem paradoxical that removal of PR-DUB and the concomitant increase in H2Aub levels results in the same phenotype. In this context, it is important to note that the level of Sce protein itself is unaffected in embryos that lack PR-DUB.Citation7
A clue as to why H2A ubiquitination and deubiquitination are both required for HOX gene repression came from genetic studies that found a strong synergy between Sce and PR-DUB. This synergy is revealed in Sce homozygous embryos derived from heterozygous mothers, where maternally-deposited Sce protein persists during the early stages of embryogenesis and initially rescues repression of HOX genes. If such Sce mutant embryos also lack PR-DUB, repression of HOX genes is lost earlier in development and these embryos show the same phenotype as embryos that lack both maternal and zygotic Sce protein.Citation36 A straightforward interpretation of these findings is that H2A monoubiquitination by the maternally-deposited Sce protein becomes ineffective for HOX gene repression in embryos that lack PR-DUB. According to this view, PR-DUB would thus play a critical role in helping Sce to generate an H2Aub-modified repressed chromatin state at HOX genes.
How could this work? Earlier pulse-chase experiments in mammalian cells revealed that H2Aub is turned over rapidly and suggested that this modification has a half-life of about 90 min in HeLa cells.Citation45 Furthermore, treatment of HeLa cells with proteasome inhibitor, heat-shock or stress-response inducing agents causes a rapid decrease in the abundance of H2Aub and free ubiquitin.Citation46 These observations and the finding that steady-state levels of bulk H2Aub in PR-DUB mutant embryos are 10-fold higher than in wild-type embryos suggest that this modification is deposited at a substantial fraction of nucleosomes in the genome but is normally subject to dynamic turn-over in wild-type embryos. A possible consequence of the widespread H2Aub distribution in PR-DUB mutant embryos could be that ubiquitin or an H2Aub-binding protein becomes sequestered in the chromatin of PRC1-bound genes, including those genes that do not require Sce and H2Aub for repression. Two possible scenarios can be considered. In the first scenario it is the level of free ubiquitin that becomes limiting in PR-DUB mutants. Free ubiquitin would thus drop below a critical threshold and consequently monoubiquitination of H2A in the chromatin of HOX genes becomes ineffective and insufficient for repression. In the second scenario, the limiting factor is not ubiquitin itself but an H2Aub-binding repressor. The widespread hyper-ubiquitination of H2Aub in PR-DUB mutants would sequester this H2Aub-binding repressor at genes that do not require H2Aub for repression and thereby effectively deplete the repressor from HOX gene chromatin where it is needed for repression. The gist of both scenarios is that the critical function of PR-DUB is not to deubiquitinate H2Aub at HOX genes but rather in the chromatin of class II genes and perhaps also other regions of the genome. An alternative scenario would be that the critical function of PR-DUB indeed is the deubiquitination of H2Aub in HOX gene chromatin. Considering this view, one would have to postulate that HOX gene repression requires PR-DUB to confine H2A monoubiquitination to specific regions of their chromatin, or that H2Aub needs to be deposited and removed in a temporally regulated cycle.
Genetic Approaches for Assessing the Function of H2Aub in Target Gene Repression
If a catalytically dead version of a histone-modifying enzyme fails to rescue the phenotype of a mutant that is deficient for this enzyme, this provides strong evidence that it is indeed the histone-modifying activity of this enyzme that is critical for the repression mechanism. This type of structure/function experiment is, however, not straightforward in the case of Ring finger E3 ligases such as Sce or its mammalian orthologs Ring1A/B. Specifically, these E3 ligases are essential for the transfer of ubiquitin from the ubiquitin-conjugating E2 enzyme to the substrate lysine in H2A but they do not contain a catalytic reaction center that forms a covalent reaction intermediate during the transfer reaction. Rather, they function to dock the E2-ubiquitin conjugate in the appropriate orientation on the nucleosome substrate such that the ubiquitin moiety can be transferred to the appropriate lysine residue in H2A.
A Ring1B protein with an Ile53Ala substitution at the interface that binds the ubiquitin-conjugating E2 enzyme UbcH5c shows reduced activity for H2A monoubiquitination in vitro.Citation18,Citation47,Citation48 A genetic rescue assay using murine embryonic stem (ES) found that this Ring1BIle53Ala mutant protein was able to restore chromatin compaction and transcriptional repression at HOX gene loci, two processes that are defective in Ring1B-/- ES cells.Citation49 Specifically, Eskeland et al.Citation49 reported that in Ring1B-/- ES cells that still contain a wild-type Ring1A gene, the H2Aub levels are reduced to about 40% of the levels found in wild-type cells and that a transgene-encoded Ring1BIle53Ala protein is unable to restore wild-type levels of H2Aub in these cells. Based on these findings, the authors concluded that Ring1B compacts HOX gene chromatin and represses transcription independently of H2A ubiquitination.Citation49
Using a similar transgene rescue strategy in Drosophila, we found that a Sce protein carrying the equivalent Ile48Ala mutation (SceIle48Ala) fully maintains HOX gene silencing in Drosophila embryos and larvae (). However, unlike the wild-type Sce protein expressed from a control transgene, the SceIle48Ala protein had severely compromised capacity to rescue Sce null homozygotes into the pupal stages (). Because of the poor survival of these animals into larval and pupal stages, we have not been able to generate histone extracts to determine H2Aub levels (i.e., note that this analysis needs to be done in larvae rather than in embryos where maternally-deposited wild-type Sce protein might still contribute to H2A monoubiquitination). At present, it therefore remains unclear to what extent the Ile48Ala mutation compromises the E3 ligase activity of the SceIle48Ala protein in vivo. Inspection of the structure of the Ring1B:Bmi1 heterodimer in complex with the ubiquitin-conjugating E2 enzyme UbcH5c suggests that the Ile53Ala mutation in Ring1B alone is indeed unlikely to completely disrupt the interaction.Citation48 It could thus well be that, in vivo, the mutant protein retains sufficient ubiquitin ligase activity to maintain a level of H2Aub that still permits repression of class I target genes in Drosophila. Further investigations will be needed to conclusively resolve the question about the functional consequence of H2A ubiquitination for Polycomb repression. Possible strategies to do this include a more extensive, structure-guided mutagenesis of the E2-interaction surface of Sce and also use of histone geneticsCitation50 that will allow to analyze the phenotype of animals with a mutation of the H2A residue to which ubiquitin is conjugated by Sce.
Figure 2. The SceIle48Ala protein rescues HOX gene repression but not viability in Drosophila. (A) The SceIle48Ala protein represses the HOX gene Abd-B in embryos. Ventral views of stage 16 embryos, stained with antibody against the HOX protein Abd-B. Top: wildtype (wt) embryo; below: SceKO (ref. Citation34) homozygous embryos without a transgene (2nd from top), with an hsp70-Sce transgene (hs-Sce 3rd from top), or with an hsp70-SceIle48Ala transgene (hs-SceIle48Ala, 4th from top). In all cases, embryos were collected for 2 h and repeatedly heat-shocked (20 min at 37C) every 2 h, starting at 2–4 h of development. The arrowhead marks the anterior margin of the normal Abd-B expression domain in parasegment (ps) 10 in the central nervous system. In SceKO mutant embryos, Abd-B is misexpressed anterior to ps 10. Repression of Abd-B is rescued by the wild-type Sce protein supplied by the hs-Sce transgene and also by the SceIle48Ala protein supplied by the hs-SceIle48Ala transgene. In all cases, stocks with GFP-marked balancer chromosomes were used to identify SceKO/SceKO homozygous embryos. (B) The SceIle48Ala protein represses the HOX gene Ubx in imaginal disc cells. Wing imaginal discs from SceKO heterozygous larvae with clones for SceKO/SceKO homozygous cells that are marked by the absence of GFP. Discs were stained with an antibody against the HOX protein Ubx (top row) or with an antibody against the Sce protein (bottom row). The larvae carried no transgene (no TG, left column), or the hs-Sce (middle column), or hs-SceIle48Ala transgene (right column) as in (A). In all cases, clones were first induced 96 h prior to analysis and repeatedly heat-shocked for 1 h at 37C every 12 h, beginning at the time of clone induction. SceKO mutant clones show lack of Sce protein and strong misexpression of Ubx protein. Repression of Ubx is rescued by the wild-type Sce protein supplied by the hs-Sce transgene and also by the SceIle48Ala protein supplied by the hs-SceIle48Ala transgene. (C) The SceIle48Ala protein has impaired capacity for rescuing SceKO homozyotes into pupae. Embryos with the same genotype as in (A) were collected at 30°C and reared at 30°C until completion of embryogenesis. During postembryonic development, larvae were reared at 25°C and heat-shocked for 1 h at 37°C every 12 h. The fraction of embryos that reached the pupal stage was determined and is represented on the Y-axis. SceKO/ SceKO individuals all die at the end of embryogenesis (0 pupae from 403 embryos, asterisk). Of the SceKO/ SceKO embryos that carry the hs-Sce transgene, 16.98% were rescued to develop into pupae (107 pupae from 630 embryos). Of the wild-type embryos (wt) subjected to the same heat-shock treatment, a comparable fraction of 21.75% developed into pupae (261 pupae from 1200 embryos). In contrast, of the SceKO/ SceKO embryos that carry the hs-SceIle48Ala transgene, only 3.03% are rescued to develop into pupae (17 pupae from 561 embryos).
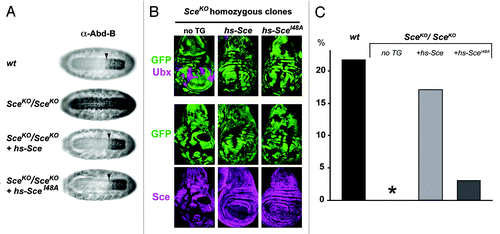
Acknowledgments
We thank Elena Conti and Reinhard Kalb for critical reading of the manuscript. This work was supported by funds from the Max-Planck Institute (MPI) of Biochemistry.
References
- Shao Z, Raible F, Mollaaghababa R, Guyon JR, Wu CT, Bender W, et al. Stabilization of chromatin structure by PRC1, a Polycomb complex. Cell 1999; 98:37 - 46; http://dx.doi.org/10.1016/S0092-8674(00)80604-2; PMID: 10412979
- Czermin B, Melfi R, McCabe D, Seitz V, Imhof A, Pirrotta V. Drosophila enhancer of Zeste/ESC complexes have a histone H3 methyltransferase activity that marks chromosomal Polycomb sites. Cell 2002; 111:185 - 96; http://dx.doi.org/10.1016/S0092-8674(02)00975-3; PMID: 12408863
- Müller J, Hart CM, Francis NJ, Vargas ML, Sengupta A, Wild B, et al. Histone methyltransferase activity of a Drosophila Polycomb group repressor complex. Cell 2002; 111:197 - 208; http://dx.doi.org/10.1016/S0092-8674(02)00976-5; PMID: 12408864
- Klymenko T, Papp B, Fischle W, Köcher T, Schelder M, Fritsch C, et al. A Polycomb group protein complex with sequence-specific DNA-binding and selective methyl-lysine-binding activities. Genes Dev 2006; 20:1110 - 22
- Nekrasov M, Klymenko T, Fraterman S, Papp B, Oktaba K, Köcher T, et al. Pcl-PRC2 is needed to generate high levels of H3-K27 trimethylation at Polycomb target genes. EMBO J 2007; 26:4078 - 88; http://dx.doi.org/10.1038/sj.emboj.7601837; PMID: 17762866
- Lagarou A, Mohd-Sarip A, Moshkin YM, Chalkley GE, Bezstarosti K, Demmers JAA, et al. dKDM2 couples histone H2A ubiquitylation to histone H3 demethylation during Polycomb group silencing. Genes Dev 2008; 22:2799 - 810
- Scheuermann JC, de Ayala Alonso AG, Oktaba K, Ly-Hartig N, McGinty RK, Fraterman S, et al. Histone H2A deubiquitinase activity of the Polycomb repressive complex PR-DUB. Nature 2010; 465:243 - 7; http://dx.doi.org/10.1038/nature08966; PMID: 20436459
- Cao R, Wang L, Wang H, Xia L, Erdjument-Bromage H, Tempst P, et al. Role of histone H3 lysine 27 methylation in Polycomb-group silencing. Science 2002; 298:1039 - 43; http://dx.doi.org/10.1126/science.1076997; PMID: 12351676
- Kuzmichev A, Nishioka K, Erdjument-Bromage H, Tempst P, Reinberg D.. Histone methyltransferase activity associated with a human multiprotein complex containing the Enhancer of Zeste protein. Genes Dev 2002; 16:2893 - 905; http://dx.doi.org/10.1101/gad.1035902
- Levine SS, Weiss A, Erdjument-Bromage H, Shao Z, Tempst P, Kingston RE. The core of the polycomb repressive complex is compositionally and functionally conserved in flies and humans. Mol Cell Biol 2002; 22:6070 - 8; http://dx.doi.org/10.1128/MCB.22.17.6070-6078.2002; PMID: 12167701
- Sarma K, Margueron R, Ivanov A, Pirrotta V, Reinberg D. Ezh2 requires PHF1 to efficiently catalyze H3 lysine 27 trimethylation in vivo. Mol Cell Biol 2008; 28:2718 - 31; http://dx.doi.org/10.1128/MCB.02017-07; PMID: 18285464
- Machida YJ, Machida Y, Vashisht AA, Wohlschlegel JA, Dutta A. The deubiquitinating enzyme BAP1 regulates cell growth via interaction with HCF-1. J Biol Chem 2009; 284:34179 - 88; http://dx.doi.org/10.1074/jbc.M109.046755; PMID: 19815555
- Sowa ME, Bennett EJ, Gygi SP, Harper JW. Defining the human deubiquitinating enzyme interaction landscape. Cell 2009; 138:389 - 403; http://dx.doi.org/10.1016/j.cell.2009.04.042; PMID: 19615732
- Yu H, Mashtalir N, Daou S, Hammond-Martel I, Ross J, Sui G, et al. The ubiquitin carboxyl hydrolase BAP1 forms a ternary complex with YY1 and HCF-1 and is a critical regulator of gene expression. Mol Cell Biol 2010; 30:5071 - 85; http://dx.doi.org/10.1128/MCB.00396-10; PMID: 20805357
- Gao Z, Zhang J, Bonasio R, Strino F, Sawai A, Parisi F, et al. PCGF homologs, CBX proteins, and RYBP define functionally distinct PRC1 family complexes. Mol Cell 2012; 45:344 - 56; http://dx.doi.org/10.1016/j.molcel.2012.01.002; PMID: 22325352
- Misaghi S, Ottosen S, Izrael-Tomasevic A, Arnott D, Lamkanfi M, Lee J, et al. Association of C-terminal ubiquitin hydrolase BRCA1-associated protein 1 with cell cycle regulator host cell factor 1. Mol Cell Biol 2009; 29:2181 - 92; http://dx.doi.org/10.1128/MCB.01517-08; PMID: 19188440
- Cao R, Zhang Y. SUZ12 is required for both the histone methyltransferase activity and the silencing function of the EED-EZH2 complex. Mol Cell 2004; 15:57 - 67; http://dx.doi.org/10.1016/j.molcel.2004.06.020; PMID: 15225548
- Buchwald G, van der Stoop P, Weichenrieder O, Perrakis A, van Lohuizen M, Sixma TK. Structure and E3-ligase activity of the Ring-Ring complex of polycomb proteins Bmi1 and Ring1b. EMBO J 2006; 25:2465 - 74; http://dx.doi.org/10.1038/sj.emboj.7601144; PMID: 16710298
- Li Z, Cao R, Wang M, Myers MP, Zhang Y, Xu R-M. Structure of a Bmi-1-Ring1B polycomb group ubiquitin ligase complex. J Biol Chem 2006; 281:20643 - 9; http://dx.doi.org/10.1074/jbc.M602461200; PMID: 16714294
- Nekrasov M, Wild B, Müller J. Nucleosome binding and histone methyltransferase activity of Drosophila PRC2. EMBO Rep 2005; 6:348 - 53; http://dx.doi.org/10.1038/sj.embor.7400376; PMID: 15776017
- Margueron R, Justin N, Ohno K, Sharpe ML, Son J, Drury WJ 3rd, et al. Role of the polycomb protein EED in the propagation of repressive histone marks. Nature 2009; 461:762 - 7; http://dx.doi.org/10.1038/nature08398; PMID: 19767730
- Schmitges FW, Prusty AB, Faty M, Stützer A, Lingaraju GM, Aiwazian J, et al. Histone methylation by PRC2 is inhibited by active chromatin marks. Mol Cell 2011; 42:330 - 41; http://dx.doi.org/10.1016/j.molcel.2011.03.025; PMID: 21549310
- Birve A, Sengupta AK, Beuchle D, Larsson J, Kennison JA, Rasmuson-Lestander A, et al. Su(z)12, a novel Drosophila Polycomb group gene that is conserved in vertebrates and plants. Development 2001; 128:3371 - 9; PMID: 11546753
- Ketel CS, Andersen EF, Vargas ML, Suh J, Strome S, Simon JA. Subunit contributions to histone methyltransferase activities of fly and worm polycomb group complexes. Mol Cell Biol 2005; 25:6857 - 68; http://dx.doi.org/10.1128/MCB.25.16.6857-6868.2005; PMID: 16055700
- Papp B, Müller J.. Histone trimethylation and the maintenance of transcriptional ON and OFF states by trxG and PcG proteins. Genes Dev 2006; 20:2041 - 54
- Ohno K, McCabe D, Czermin B, Imhof A, Pirrotta V. ESC, ESCL and their roles in Polycomb Group mechanisms. Mech Dev 2008; 125:527 - 41; http://dx.doi.org/10.1016/j.mod.2008.01.002; PMID: 18276122
- Anderson AE, Karandikar UC, Pepple KL, Chen Z, Bergmann A, Mardon G. The enhancer of trithorax and polycomb gene Caf1/p55 is essential for cell survival and patterning in Drosophila development. Development 2011; 138:1957 - 66; http://dx.doi.org/10.1242/dev.058461; PMID: 21490066
- Francis NJ, Saurin AJ, Shao Z, Kingston RE. Reconstitution of a functional core polycomb repressive complex. Mol Cell 2001; 8:545 - 56; http://dx.doi.org/10.1016/S1097-2765(01)00316-1; PMID: 11583617
- Lo SM, Ahuja NK, Francis NJ. Polycomb group protein Suppressor 2 of zeste is a functional homolog of Posterior Sex Combs. Mol Cell Biol 2009; 29:515 - 25; http://dx.doi.org/10.1128/MCB.01044-08; PMID: 18981224
- Lo SM, Francis NJ. Inhibition of chromatin remodeling by polycomb group protein posterior sex combs is mechanistically distinct from nucleosome binding. Biochemistry 2010; 49:9438 - 48; http://dx.doi.org/10.1021/bi100532a; PMID: 20873869
- Strübbe G, Popp C, Schmidt A, Pauli A, Ringrose L, Beisel C, et al. Polycomb purification by in vivo biotinylation tagging reveals cohesin and Trithorax group proteins as interaction partners. Proc Natl Acad Sci U S A 2011; 108:5572 - 7; http://dx.doi.org/10.1073/pnas.1007916108; PMID: 21415365
- Gorfinkiel N, Fanti L, Melgar T, García E, Pimpinelli S, Guerrero I, et al. The Drosophila Polycomb group gene Sex combs extra encodes the ortholog of mammalian Ring1 proteins. Mech Dev 2004; 121:449 - 62; http://dx.doi.org/10.1016/j.mod.2004.03.019; PMID: 15147763
- Francis NJ, Kingston RE, Woodcock CL. Chromatin compaction by a polycomb group protein complex. Science 2004; 306:1574 - 7; http://dx.doi.org/10.1126/science.1100576; PMID: 15567868
- Beh LY, Colwell LJ, Francis NJ. A core subunit of Polycomb repressive complex 1 is broadly conserved in function but not primary sequence. Proc Natl Acad Sci U S A 2012; 109:E1063 - 71; http://dx.doi.org/10.1073/pnas.1118678109; PMID: 22517748
- Martin EC, Adler PN. The Polycomb group gene Posterior Sex Combs encodes a chromosomal protein. Development 1993; 117:641 - 55; PMID: 7687213
- Gutiérrez L, Oktaba K, Scheuermann JC, Gambetta MC, Ly-Hartig N, Müller J. The role of the histone H2A ubiquitinase Sce in Polycomb repression. Development 2012; 139:117 - 27; http://dx.doi.org/10.1242/dev.074450; PMID: 22096074
- Breen TR, Duncan IM. Maternal expression of genes that regulate the bithorax complex of Drosophila melanogaster. Dev Biol 1986; 118:442 - 56; http://dx.doi.org/10.1016/0012-1606(86)90015-1; PMID: 3098596
- Fritsch C, Beuchle D, Müller J. Molecular and genetic analysis of the Polycomb group gene Sex combs extra/Ring in Drosophila. Mech Dev 2003; 120:949 - 54; http://dx.doi.org/10.1016/S0925-4773(03)00083-2; PMID: 12963114
- Schwartz YB, Kahn TG, Nix DA, Li X-Y, Bourgon R, Biggin M, et al. Genome-wide analysis of Polycomb targets in Drosophila melanogaster. Nat Genet 2006; 38:700 - 5; http://dx.doi.org/10.1038/ng1817; PMID: 16732288
- Gambetta MC, Oktaba K, Müller J. Essential role of the glycosyltransferase sxc/Ogt in polycomb repression. Science 2009; 325:93 - 6; http://dx.doi.org/10.1126/science.1169727; PMID: 19478141
- Vassilev AP, Rasmussen HH, Christensen EI, Nielsen S, Celis JE. The levels of ubiquitinated histone H2A are highly upregulated in transformed human cells: partial colocalization of uH2A clusters and PCNA/cyclin foci in a fraction of cells in S-phase. J Cell Sci 1995; 108:1205 - 15; PMID: 7622605
- Wang H, Wang L, Erdjument-Bromage H, Vidal M, Tempst P, Jones RS, et al. Role of histone H2A ubiquitination in Polycomb silencing. Nature 2004; 431:873 - 8; http://dx.doi.org/10.1038/nature02985; PMID: 15386022
- Scheuermann JC. Identification of PR-DUB - a Polycomb group complex with histone H2A deubiquitinase activity. EMBL. Heidelberg: Heidelberg University, 2011.
- Gaytán de Ayala Alonso A, Gutiérrez L, Fritsch C, Papp B, Beuchle D, Müller J. A genetic screen identifies novel polycomb group genes in Drosophila. Genetics 2007; 176:2099 - 108; http://dx.doi.org/10.1534/genetics.107.075739; PMID: 17717194
- Seale RL. Rapid turnover of the histone-ubiquitin conjugate, protein A24. Nucleic Acids Res 1981; 9:3151 - 8; http://dx.doi.org/10.1093/nar/9.13.3151; PMID: 6269073
- Mimnaugh EG, Chen HY, Davie JR, Celis JE, Neckers L. Rapid deubiquitination of nucleosomal histones in human tumor cells caused by proteasome inhibitors and stress response inducers: effects on replication, transcription, translation, and the cellular stress response. Biochemistry 1997; 36:14418 - 29; http://dx.doi.org/10.1021/bi970998j; PMID: 9398160
- Elderkin S, Maertens GN, Endoh M, Mallery DL, Morrice N, Koseki H, et al. A phosphorylated form of Mel-18 targets the Ring1B histone H2A ubiquitin ligase to chromatin. Mol Cell 2007; 28:107 - 20; http://dx.doi.org/10.1016/j.molcel.2007.08.009; PMID: 17936708
- Bentley ML, Corn JE, Dong KC, Phung Q, Cheung TK, Cochran AG. Recognition of UbcH5c and the nucleosome by the Bmi1/Ring1b ubiquitin ligase complex. EMBO J 2011; 30:3285 - 97; http://dx.doi.org/10.1038/emboj.2011.243; PMID: 21772249
- Eskeland R, Leeb M, Grimes GR, Kress C, Boyle S, Sproul D, et al. Ring1B compacts chromatin structure and represses gene expression independent of histone ubiquitination. Mol Cell 2010; 38:452 - 64; http://dx.doi.org/10.1016/j.molcel.2010.02.032; PMID: 20471950
- Günesdogan U, Jäckle H, Herzig A. A genetic system to assess in vivo the functions of histones and histone modifications in higher eukaryotes. EMBO Rep 2010; 11:772 - 6; http://dx.doi.org/10.1038/embor.2010.124; PMID: 20814422