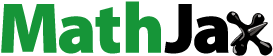
Abstract
Genetically modified (GM) crops is considered the fastest adopted crop technology in the history of modern agriculture. However, possible undesirable and unintended effects must be considered during the research steps toward development of a commercial product. In this report we evaluated effects of a common bean virus resistant line on arthropod populations, considered as non-target organisms. This GM bean line (named M1/4) was modified for resistance against Bean golden mosaic virus (BGMV) by expressing a mutated REP protein, which is essential for virus replication. Biosafety studies were performed for a period of three years under field conditions. The abundance of some species was significantly higher in specific treatments in a particular year, but not consistently different in other years. A regular pattern was not observed in the distribution of insects between genetically modified and conventional treatments. Data analyses showed that minor differences observed can be attributed to random variation and were not consistent enough to conclude that the treatments were different. Therefore the present study indicates that the relative abundance of species are similar in transgenic and non-transgenic fields.
Introduction
Commercial planting of genetically modified (GM) crops has been increasing worldwide over the past 18 y. The area being cultivated with transgenic crops increased 8% in 2011, and represented 36% of the global seed market.Citation1 With an accumulated cultivated area of over 1 billion hectares, during the period of 1996 to 2010, GM crops were considered one of the fastest adopted crop technology in the history of modern agriculture.Citation2 Over three billion people consume GM foods around the world and no negative effect on human health or environment has been proved to date.Citation3 However, possible effects on the maintenance and sustainability of biological diversity, albeit not well defined,Citation4 should be taken into consideration. To better address this issue the differences between GM and non-GM systems are analyzed on a case by case basis, and could be associated with the plant species, trait, or occasionally the nature of the change.
Several authorsCitation5,Citation6 have discussed the impact of insect-resistant GM crops on non-target arthropods, especially those expressing proteins from Bacillus thuringiensis (Bt) plants.Citation7 Among transgenic disease-resistant crops, only virus-resistant cultivars are currently commercially available.Citation8 Nevertheless, little research is available on the impact of virus-resistant GM crops on biodiversity. As this type of GM crop is not generated to act directly on disease vectors (mainly insects), the most studied environmental effects are gene flow, heterologous viral encapsidation and viral recombination.Citation8,Citation9 One example of the impact on insect populations by a virus-resistant GM crop was reported by Schubert et al. (2004), in a study which evaluated the effect of a GM potato event resistant to Potato virus Y on three species of aphids, colonizing potato plants.Citation10 They found no negative effects on the insect population that could be associated with the presence of the transgene. In the present study, we considered non-target organisms as any arthropod exposed to the GM plant in the field, even if the insect might reduce crop yield, including the virus vector, the whitefly Bemisia tabaci Genn.
Bean golden mosaic virus (BGMV) is one of the most devastating plant viruses, causing golden mosaic of common beans (Phaseolus vulgaris L.). There is no immunity or even high level of disease resistance in the germplasm evaluated. Therefore the management of BGMV has focused on controlling the insect vector, Bemisia tabaci. The virus belongs to the genus Begomovirus, family Geminiviridae. The virus replication associated protein (REP) has been modified to become a transdominant lethal to the homologous virus when expressed in tobacco protoplasts.Citation11 A genetically modified (GM) common bean line named Olathe M1/4 was obtained with a cassette containing the mutated BGMV rep gene and the bar gene by particle bombardment of embryos from cultivar Olathe Pinto.Citation12
This line expresses partial resistance to the homologous virus and also a good level of resistance to the herbicide glufosinate ammonium.Citation12 Biosafety studies were performed with this BGMV-resistant line focusing on possible effects of this GM plant on non-target arthropods as part of a set of biosafety evaluations to demonstrate the safety of the above named GM bean line, as required by the Brazilian Biosafety Committee regarding the environment. Here, we report the results of a three-year evaluation of the effects of Olathe M1/4 GM common bean on arthropod populations under field conditions.
Results
A total of 20 446 arthropod specimens from the classes Insecta and Aracnida were collected in the canopy of plants for both GM and non-GM plants. Some of the species found in lower frequencies were grouped by family (Cicadellidae, Chrysomelidae, Coccinelidae, Thripidae, and Pentatomidade, for which the prevalent species was Piezodorus guildinii). Order Lepidoptera included the families Crambidae (Omiodes sp.), Noctuidae (Spodoptera sp. and Pseudoplusia sp.), and Hesperiidae (Urbanus proteus). The most abundant insect species were Cerotoma arcuata, Bemisia tabaci, thrips species and the morpho-species from the order Acari (). Herbivores were the most frequent group, found in 21.9% of the samples, followed by predators at 8.6%. The number of individuals increased throughout the three years from 4045 individuals in 2004 to 5483 in 2005 and increased more than 2-fold to 10 918 in 2006. The number of species collected also increased in 2005 (28), including Pentatomidae (from the genera Euschistus, Edessa, Nezara and Piezodorus), some species of Lepidoptera (Spodoptera and Pseudoplusia), and certain morpho-species of Chrysomelidae (). In 2006, the number of species (20) was almost the same as in 2004 (18).
Table 1. Abundance of specimens (Genus/species), by order and family, sampled in the plant canopy of genetically modified (GM) and non-genetically modified common bean plants (NGM) by year (2004, 2005 and 2006) and respective trophic guilds
The ANOVA of the grouped data showed three significant differences between the two treatments out of the 27 pairs of data analyzed ().
Table 2. Mean number of the most frequent species sampled in plant canopy of genetically modified (GM) and non-genetically modified common bean plants (NGM) in the three-year experiment
The most important species identified by the Stepwise statistics procedure to be used subsequently in further analysis included B. tabaci (adults), C. arcuata, D. speciosa, E. kraemeri, and Pentatomidae ().
Table 3. Stepwise selection summary for the ordination procedure to select arthropod species that would be included in the canonical variate analysis for maximum discrimination between treatments
Discriminant analysis indicated some significant differences between treatments (GM and non-GM beans) regarding the abundance of the selected species (Wilks’ λ = 0.98; f = 3.52; df (num/den) = 5/794; P = 0.0037). Based on the canonical loading (total canonical structure) of the first canonical axis, the two species that most contributed to the divergence between the two main treatments were C. arcuata, which contributed positively, and E. kraemeri, which contributed negatively (). However, the mean number of these selected species pooled across sampling dates in a specific year, resulted in few dates in which the number of insects was significantly different between treatments (, , , , and ).
Table 4. Impact of the main treatments on arthropod assemblages in three years of experiments
Figure 1. Mean number of adults of Cerotoma arcuata sampled in plant canopy of genetically modified (GM) and non-genetically modified common bean plants (NGM), in 2 m of row, in eight sampling dates in 2004 (A), 2005 (B), and 2006 (C). Asterisk in the specific sample date indicates that treatments are significantly different (Tukey’s test of transformed data using ; P < 0.05).
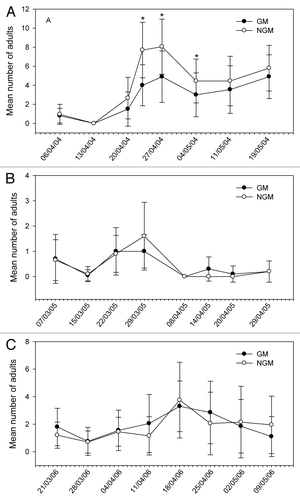
Figure 2. Mean number of adults of Bemisia tabacisampled in plant canopy of genetically modified (GM) and non-genetically modified common bean plants (NGM), in 2 m of row, in eight sampling dates in 2004 (A), 2005 (B), and 2006 (C). Asterisk in the specific sample date indicates that treatments are significantly different (Tukey’s test of transformed data using ; P < 0.05).
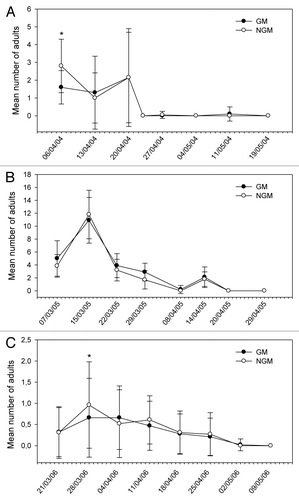
Figure 3. Mean number of nymphs and adults of Pentatomidae sampled in plant canopy of genetically modified (GM) and non-genetically modified common bean plants (NGM), in 2 m of row, in eight sampling dates in 2004 (A), 2005 (B), and 2006 (C). Asterisk in the specific sample date indicates that treatments are significantly different (Tukey’s test of transformed data using ; P < 0.05).
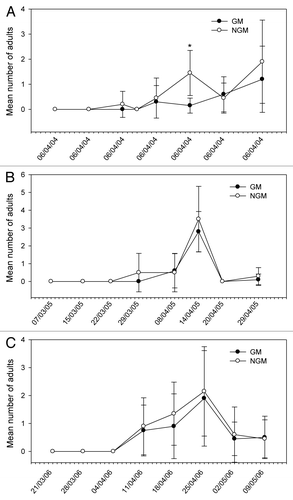
Figure 4. Mean number of nymphs and adults of Emposca kraemeri sampled in plant canopy of genetically modified (GM) and non-genetically modified common bean plants (NGM), in 2 m of row, in eight sampling dates in 2004 (A), 2005 (B), and 2006 (C). Asterisk in the specific sample date indicates that treatments are significantly different (Tukey’s test of transformed data using ; P < 0.05).
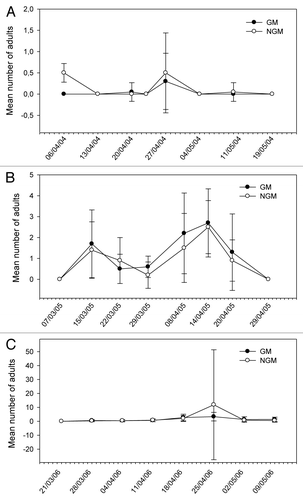
Figure 5. Mean number of adults of Diabrotica speciosa sampled in plant canopy of genetically modified (GM) and non-genetically modified common bean plants (NGM), in 2 m of row, in eight sampling dates in 2004 (A), 2005 (B), and 2006 (C). Asterisk in the specific sample date indicates that treatments are significantly different (Tukey’s test of transformed data using ; P < 0.05).
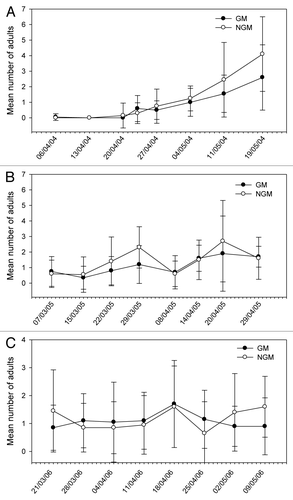
Considering that differences between treatments were observed only in some sampling dates, a factorial analysis was performed to verify the influence of common bean lines (GM and non-GM), experimental years (2004, 2005, and 2006), and sampling dates (eight sampling dates per year) in the mean number of the selected species. Common bean lines showed significant effects only on C. arcuata, D. speciosa, and Pentatomidae (P < 0.05). Highly significant effect of the variable “experimental year” was observed for all selected species (P < 0.0001), but it did not show interaction with the factor “common bean line,” except for C. arcuata (P < 0.0001). Sampling date also had a highly significant effect on all variables (P < 0.0001) and so did the interaction between year and sampling date. This would be expected, since sampling dates reflect plant age, development of the canopy and microenvironment. No significant interactions were observed among the three factors—common bean line x year x sampling date ().
Table 5. Summary of factorial analysis for Cerotoma arcuata, Diabrotica speciosa, Bemisia tabaci, Empoasca kraemeri, and Pentatomidae assemblages sampled in plant canopy of common bean lines (genetically modified and non-genetically modified) for three consecutive years (2004 to 2006) and through eight weekly sampling dates by year
Discussion
Environmental risk assessment of genetically modified plants is often focused on two main topics: (1) the risk of gene flow occurring and (2) potential effects on non-target organisms. As a virus-tolerant genetically modified event, the transgene of this common bean line was designed specifically against the Bean golden mosaic virus, and not against other viruses.Citation12 The mutated REP protein is involved in the virus replication complex and was expressed in leaves, stems and flowers (a weak signal) of the Olathe M1/4 plants, but not in seed tissues.Citation12 It should be pointed out that the transgenic bean was also expressing the PAT protein, which is regarded as non-toxic for arthropods.Citation13 As the target of the Olathe M1/4 line was the virus, the organisms associated with the plants were expected to not be affected. Some authorsCitation14 refer to non-target organisms as those that do not reduce crop yield (beneficial organisms) and were negatively affected by the product of the transgene itself.
The relative distribution of species appears to be similar in both GM and non-GM fields, as they were observed in both treatments. The class Insecta was represented by seven orders, 21 families, and about 29 morpho-species. Among these morpho-species, there were five species of predators, one parasitoid and one flower-visiting specimen. In the class Arachnida, spiders and phytophagous acari were collected. The predators Callida sp. and Geocoris sp. in 2004, and Zellus sp. and Chrysopidae in 2005 (), were exceptions, and were present in extremely low populations. A high frequency of capture (above 10%) was noticed for C. arcuata, Diabrotica speciosa, B. tabaci (nymphs, adults and eggs), thrips species, Cicadellidae, Empoasca kraemeri, the insects from the family Pentatomidae (especially Piezodorus guildinii), and the groups of spiders and acari. From these, the Pentatomidae and C. arcuata were significantly higher in non-GM plots only in 2004 (). In 2005, there were significant differences for the population of thrips (), which was higher on the GM plants. In further analysis using stepwise statistics, the species that most contributed to the variation observed were B. tabaci (adults), C. arcuata, D. speciosa, E. kraemeri, and Pentatomidae (), which were subsequently used in discriminant analysis. Although the number of B. tabaci and E. kraemeri specimens sampled was not significantly different between treatments in univariate test statistics (P < 0.4522 and P < 0.380, respectively), these two species were identified by the stepwise procedure, probably because in the presence of the other variables they helped to explain the variance of the treatments. On the other hand, although the total number of thrips was significantly different between treatments in 2005 (), this group was left out by the stepwise procedure. No significant difference between treatments was observed for the abundance of species that were not included in the discriminant analysis, such as natural enemies, for instance.
The abundance of some species was statistically higher in non-GM plants in some sample dates only in the first year, as for C. arcuata in three out of eight sampling dates and for B. tabaci and Pentatomidae group in one out of eight sampling dates of 2004 (–). In 2005, only Thripidae showed differences between treatments (; –). On the other hand, the abundance of E. kraemeri was higher in the GM treatment in one sampling date in the third year (), while the number of B. tabaci was higher in non-GM plants only in one sampling date in 2004 (). As the total number of some of these species did not differ between treatments in univariate analysis within years (), the differences observed in some sampling dates may have been random.
Changes in pest populations can also disturb ecosystem balance. Here, all arthropod species were found in both treatments and the species identified by the stepwise procedure were herbivores considered as common bean pests (C. arcuata; B. tabaci, E. kraemeri, D. speciosa, and those from the family Pentatomidae, especially P. guildinii). None of the natural enemies were identified as relevant species in the stepwise procedure to explain the variance between treatments.
The fluctuation of selected species varied through sampling dates within years and did not follow a distribution pattern (–). In a few sampling dates, the number of certain pest species was significantly lower in the GM than in non-GM plants. Although the introduced gene did not aim to reduce pest population levels, these results show that Olathe M1/4 GM plants were less visited by these pest species in a few sampling dates, especially C. arcuata (3 dates out of 24), B. tabaci (2 dates out of 24), and P. guildinii (1 date out of 24). These species are considered as common bean key pests in Brazil. The REP protein expressed in leaves and stems, which are the parts of the plant that these insects feed on, might have had no effect on these pest species, since the differences in some sampling dates appear to be random and they did not repeat routinely in time. If so, it does not seem to be a lethal effect, because insect mortality was not observed. In any case, this does not represent a loss of biodiversity, because in the absence of non-transgenic plants, these insect species would be able to feed on transgenic plants. To our knowledge, there is no report on a change in arthropod presence on plants infected by geminiviruses, which are obviously expressing the non-mutated REP protein version. On the other hand, a reduction in the number of insect pest species might be considered as a positive effect because it would allow broad-spectrum insecticide use to be reduced.Citation15 In Brazil, B. tabaci populations are still controlled by using agrochemicals, with a negative impact on biodiversity and ecosystem balance.
Although a lower number of some species were observed in GM plants, the total number and the diversity of arthropods sampled in the experiments rose through the experimental years. As no other crop was cultivated in the experimental field during the experiments, a possible allelochemical effect originating from the GM plants, which could reduce the number and diversity of arthropods in the area, was ruled out.
There are few studies on the effect of virus resistant/tolerant GM plants on arthropod assemblages or any other non-target organisms. This occurs because risk assessment studies on genetically modified plants usually aim to verify unintended effects on non-target populations and to provide information on the nature of the introduced gene productCitation16 and often this is not the case for virus-resistant GM plants. In this kind of GM plant, the gene product directly affects virus ability to replicate, disturbing it in almost all cases. In contrast, the gene product of Bt-expressing plants is a lethal protein to target arthropods, which is often expressed in all organs of the plant and might be as lethal to the target arthropod as to the non-target organisms. Therefore there are many studies on how Bt plants affect non-target organisms. Many authorsCitation17-Citation23 reported that no effect was observed on arthropod populations due to Bt plants while others,Citation24-Citation26 including direct use of conventional Bacillus thuringiensis,Citation24 detected negative effects which may have been due to prey-quality, as pointed out by Romeis et al.Citation27
Prendeville and PilsonCitation28 reported that virus-resistant GM cultivated squash plants affected pollinator behavior. These authors observed that honey bees visited female conventional flowers more than female transgenic flowers. They observed that conventional flowers were generally larger and with more nectar than transgenic flowers, but they did not attribute the difference in bee behavior to the floral traits because the insects did not visit male flowers as often as females, although male flowers were as large as female in conventional plants. In the present study, GM common bean plants showed no pleiotropic effect (plant height, site of first pod insertion, number of branches, internode length, foliar area, total number of flowers, pods and seeds),Citation12 even when they were backcrossed with commercial cultivars.Citation29
In the present study, differences in environmental conditions throughout the three years seemed to have greater impact on insect abundance and diversity than the GM line itself. The total number of specimens rose from the first year to the third year, probably due to agrochemicals applied on the experimental field for management purposes in the first year and at a lower rate in the second and third year ().As few differences were observed between transgenic and non-transgenic plots within years, environmental conditions seemed to have had the same impact on both treatments, within years. Priestley and BrownbridgeCitation30 studied the impact of some Bt transgenic silage corn lines on non-target soil arthropods and reported that transgenic plants had no negative effect on any of the organisms analyzed, but there was a significant year effect on the abundance and diversity of some taxa. Li et al.Citation31 studied the effect of Bt cotton continuously planted for many years on soil microorganisms in a three-year field experiment. These authors also reported that no significant difference in the abundance or diversity indexes could be attributable to long-term cultivation of transgenic cotton, but there was significant seasonal variation in the number of soil microorganisms collected in Bt cotton plots.
Table 6. Agrochemicals used in the field experiments
In our study, the abundance of some insect species varied through the years while some differences between treatments were observed in specific sampling dates. In general, insect species found in transgenic plots were also found in non-transgenic plots, even though in significantly different numbers, on a few occasions. Thus, the data analyses showed that minor differences observed can be attributed to random variation and were not consistent enough to conclude that the treatments were different. This finding is consistent with the mode of action of a plant anti-viral gene which would not have any effect on a non target arthropod.
Materials and Methods
Source of transgenic plant
Genetically modified common bean line Olathe M1/4 containing a mutated rep gene of Bean golden mosaic virus and bar gene was obtained by particle bombardment of embryos from cultivar Olathe Pinto.Citation12
Experimental design
Field experiments were performed at Embrapa Rice and Beans (Santo Antônio de Goiás, GO, Brazil; Lat. 16°28′00´´S, Long. 49°17′00´´W, Alt. 823 min), for three consecutive years, from 2004 to 2006. All experiments were performed under controlled field release, at the same experimental site, with permission from the National Biosafety Technical Commission (CTNBio). The experimental fields were not used for other crops during the period. The experiments were conducted using a complete randomized design, with ten plots (replications) per treatment (GM line Olathe M1/4 and the parental cultivar Olathe Pinto). The treatments were sowed on 03/24/2004, 02/23/2005, and 06/16/2006. Each plot had three three-meter-long rows in the first year and five five-meter-long rows in the second and third years. Rows were spaced 0.50 min apart, while plots were separated by one meter. Seeding densities were 10 plants/linear meter in the first year and 15 plants/linear meter in the others. Twenty rows of cultivar Olathe Pinto were also sown around the experiment, as a border, to contain undesirable gene flow. A corn border was sown in a swathe of ten meters surrounding the complete experiment, to further contain possible gene flow. The complete experiment was sown on the same day. Common beans were grown following regular agronomic practices, and irrigated by a conventional sprinkling irrigation system as necessary. Agrochemicals were used if strictly necessary, according to an established IPM program (), and included the common bean border rows.
Arthropod sampling
After plant emergence, sampling was performed weekly using the methodology developed by QuintelaCitation32 for Integrated Pest Management (IPM) of common bean with few adaptations. Samples were always collected at the same time of day, starting at 08:30 AM and ending by 10:00 AM, to avoid peak temperatures. Up to the third-leaf-stage, sampling was performed with visual searches for species on both adaxial and abaxial surfaces of the leaves over two meters of a randomly selected row per plot. After this plant stage, the insects were sampled using a ground cloth (1.0 min length by 0.5 min width) with two aluminum rods along the opposite sides of the cloth, in two randomized points per plot. The ground cloth was spread between two rows and the plants were shaken onto the sheet and the number of each taxon that fell on to it was registered. To sample the species of thrips, a white plastic plate (0.50 min length by 0.40 cm width) was used, above which plants were strongly shaken at two randomized points per plot, and then the number of insects that fell onto the plate was recorded. Adults of whitefly were sampled on ten random leaves per plot. The adults were sampled on both sides of each leaf on the top third of the plant canopy, which was turned down slowly to avoid dispersal of the insects. Additionally, ten leaves per plot were collected weekly to sample for eggs, nymphs and other arthropod developmental stages. The leaves were taken to the laboratory and surveyed using a stereoscopic microscope at 40× magnification.
Data analysis
The effect of the GM plants on the arthropod assemblage was studied by comparing the relative abundance of each specimen or group of morpho-species on the two treatments. Frequency of capture was determined by the formula: F (%) = number of samples with the species/total number of samples × 100. Only arthropods or group of arthropods showing frequency of capture above 10% were included in further analysis. Normality and homogeneity of variance were analyzed for the number of individuals using the HOVTEST WELCH option of GLM procedure of SAS.Citation33 Non-homogeneity counting values were transformed to , and the treatment means per year were compared using the Tukey test (P < 0.05). A discriminant analysis was performed to verify which species were more important in determining treatment differences. As there were some taxa in low number, only the taxa selected by the SASCitation33 STEPDISC procedure (PROC STEPDISC with STEPWISE selection) and with frequency of capture above 10% were included in the analysis. The data from the species selected were submitted to a canonical variate analysis, as described by Badji et al.Citation34 The effects of common bean line, year and sampling date were tested on data from the selected species using proc ANOVA.Citation32
Disclosure of Potential Conflicts of Interest
No potential conflicts of interest were disclosed.
Acknowledgments
We thank FINEP for partially supporting this work. We also thank José Francisco Arruda e Silva and Edmar Cardoso de Moura for their excellent technical assistance.
References
- Marshall A. Existing agbiotech traits continue global march. Nat Biotechnol 2012; 30:207; http://dx.doi.org/10.1038/nbt.2154; PMID: 22398607
- James C. Global status of commercialized Biotech/GM crops: 2010: ISAAA Brief 42-2010: executive summary. http://www.isaaa.org/resources/publications/briefs/42/executivesummary/default.asp Accessed 2012 Jan. 2.
- DeFrancesco L. How safe does transgenic food need to be?. Nat Biotechnol 2013; 31:794 - 802; http://dx.doi.org/10.1038/nbt.2686; PMID: 24022153
- Heink U, Bartz R. I. Kowarik I. How useful are the concepts of familiarity, biological integrity, and ecosystem health for evaluating damages by GM crops?. J Agric Environ Ethics 2012; 25:3 - 17; http://dx.doi.org/10.1007/s10806-010-9289-8
- Ferry N, Raemaekers RJM, Majerus MEN, Jouanin L, Port G, Gatehouse JA, Gatehouse AMR. Impact of oilseed rape expressing the insecticidal cysteine protease inhibitor oryzacystatin on the beneficial predator Harmonia axyridis (multicoloured Asian ladybeetle). Mol Ecol 2003; 12:493 - 504; http://dx.doi.org/10.1046/j.1365-294X.2003.01736.x; PMID: 12535099
- Lundgren JG, Wiedenmann RN. Coleopteran-specific Cry3Bb toxin from transgenic corn pollen does not affect the fitness of a nontarget species, Coleomegilla maculate DeGeer (Coleoptera: Coccinellidae). Environ Entomol 2002; 31:1213 - 8; http://dx.doi.org/10.1603/0046-225X-31.6.1213
- Romeis J, Bartsch D, Bigler F, Candolfi MP, Gielkens MMC, Hartley SE, Hellmich RL, Huesing JE, Jepson PC, Layton R, et al. Assessment of risk of insect-resistant transgenic crops to nontarget arthropods. Nat Biotechnol 2008; 26:203 - 8; http://dx.doi.org/10.1038/nbt1381; PMID: 18259178
- Collinge DB, Jørgensen HJL, Lund OS, Lyngkjaer MF. Engineering pathogen resistance in crop plants: current trends and future prospects. Annu Rev Phytopathol 2010; 48:269 - 91; http://dx.doi.org/10.1146/annurev-phyto-073009-114430; PMID: 20687833
- Thompson JR, Tepfer M. Assessment of the benefits and risks for engineered virus resistance. Adv Virus Res 2010; 76:33 - 56; http://dx.doi.org/10.1016/S0065-3527(10)76002-4; PMID: 20965071
- Schubert J, Matousek J, Mattern D. Pathogen-derived resistance in potato to Potato virus Y-aspects of stability and biosafety under field conditions. Virus Res 2004; 100:41 - 50; http://dx.doi.org/10.1016/j.virusres.2003.12.013; PMID: 15036834
- Hanson SF, Maxwell DP. Trans-dominant inhibition of geminiviral DNA replication by bean golden mosaic geminivirus rep gene mutants. Phytopathology 1999; 89:480 - 6; http://dx.doi.org/10.1094/PHYTO.1999.89.6.480; PMID: 18944720
- Faria JC, Albino MMC, Dias BBA, Cunha NB, Silva LM, Vianna GR, Aragão FJL. Partial resistance to Bean Golden Mosaic Virus in a transgenic common bean (Phaseolus vulgaris L.) line expressing a mutated rep gene. Plant Sci 2006; 171:565 - 71; http://dx.doi.org/10.1016/j.plantsci.2006.06.010
- Center for Environmental Risk Assessment, ILSI Research Foundation. A review of the environmental safety of the PAT protein. Environ Biosafety Res 2011; 10:73 - 101; http://dx.doi.org/10.1051/ebr/2012004; PMID: 22781085
- Pilson D, Prendeville HR. Ecological effects of transgenic crops and the escape of transgenes into wild populations. Annu Rev Ecol Evol Syst 2004; 35:149 - 74; http://dx.doi.org/10.1146/annurev.ecolsys.34.011802.132406
- Ammann K. Effects of biotechnology on biodiversity: herbicide-tolerant and insect-resistant GM crops. Trends Biotechnol 2005; 23:388 - 94; http://dx.doi.org/10.1016/j.tibtech.2005.06.008; PMID: 15979178
- Craig W, Tepfer M, Degrassi G, Ripandelli D. An overview of general features of risk assessments of genetically modified crops. Euphytica 2008; 164:853 - 80; http://dx.doi.org/10.1007/s10681-007-9643-8
- Sims SR. Bacillus thuringiensis var kurstaki [CryIAC] protein expressed in transgenic cotton: effects on beneficial and other non-target insects. Southwest Entomologist 1995; 20:493 - 500
- Wold SJ, Burkness EC, Hutchinson WD, Venette RC. In-field monitoring of beneficial insect populations in transgenic corn expressing a Bacillus thuringiensis toxin. J Entomol Sci 2001; 36:177 - 87
- Bhatti MA, Duan J, Head GP, Jiang C, Mckee MJ, Nickson TE, Pilcher CL, Pilcher CD. Field evaluation of the impact of corn rootworm (Coleoptera: Chrysomelidae) -protected Bt corn on ground-dwelling invertebrates. Environ Entomol 2005; a 34:1325 - 35; http://dx.doi.org/10.1603/0046-225X(2005)034[1325:FEOTIO]2.0.CO;2
- Bhatti MA, Duan J, Head GP, Jiang C, Mckee MJ, Nickson TE, Pilcher CL, Pilcher CD. Field evaluation of the impact of corn rootworm (Coleoptera: Chrysomelidae) -protected Bt corn on foliage-dwelling invertebrates. Environ Entomol 2005; b 34:1336 - 45; http://dx.doi.org/10.1603/0046-225X(2005)034[1336:FEOTIO]2.0.CO;2
- Daly T, Buntin GD. Effect of Bacillus thuringiensis transgenic corn for lepidopteran control on nontarget arthropods. Environ Entomol 2005; 34:1292 - 301; http://dx.doi.org/10.1603/0046-225X(2005)034[1292:EOBTTC]2.0.CO;2
- Pilcher CD, Rice ME, Obrycki JJ. Impact of transgenic Bacillus thuringiensis corn and crop phenology on five nontarget arthropods. Environ Entomol 2005; 34:1302 - 16; http://dx.doi.org/10.1603/0046-225X(2005)034[1302:IOTBTC]2.0.CO;2
- Arpaia S, Di Leo GM, Fiore MC, Schmidt JEU, Scardi M. Composition of arthropod species assemblages in Bt-expressing and near isogenic eggplants in experimental fields. Environ Entomol 2007; 36:213 - 27; http://dx.doi.org/10.1603/0046-225X(2007)36[213:COASAI]2.0.CO;2; PMID: 17349136
- Hilbeck A, Eckel C, Kennedy GG. Impact of Bacillus thuringiensis – insecticides on population dynamics and egg predation of the Colorado potato beetle in North Carolina potato plantings. BioControl 1998; a 43:65 - 75; http://dx.doi.org/10.1023/A:1009966404802
- Hilbeck A, Baumgartner M, Fried PM, Bigler F. Effects of transgenic Bacillus thuringiensis corn-fed prey on mortality and development time of immature Chrysoperla carnea (Neuroptera: Chrysopidae). Environ Entomol 1998; b 27:480 - 7
- Hilbeck A, Moar WJ, Pusztai-Carey M, Filippini A, Bigler F. Prey-mediated effects of Cry1Ab toxin and protoxin and Cry2A protoxin on the predator Chrysoperla carnea.. Entomol Exp Appl 1999; 91:305 - 16; http://dx.doi.org/10.1046/j.1570-7458.1999.00497.x
- Romeis J, Dutton A, Bigler F. Bacillus thuringiensis toxin (Cry1Ab) has no direct effect on larvae of the green lacewing Chrysoperla carnea (Stephens) (Neuroptera: Chrysopidae). J Insect Physiol 2004; 50:175 - 83; http://dx.doi.org/10.1016/j.jinsphys.2003.11.004; PMID: 15019519
- Prendeville HR, Pilson D. Transgenic virus resistance in cultivated squash affects pollinator behaviour. J Appl Ecol 2009; 46:1088 - 96; http://dx.doi.org/10.1111/j.1365-2664.2009.01698.x
- Pinheiro PV, Faria JC, Nogueira EOPL, Aragão FJL. Transgenes inheritance and genetic similarities of near isogenic lines of genetically modified common beans. Pesquisa Agropecu Bras 2009; 44:1168 - 76; http://dx.doi.org/10.1590/S0100-204X2009000900015
- Priestley AL, Brownbridge M. Field trials to evaluate effects of Bt-transgenic silage corn expressing the Cry1Ab insecticidal toxin on non-target soil arthropods in northern New England, USA. Transgenic Res 2009; 18:425 - 43; http://dx.doi.org/10.1007/s11248-008-9234-z; PMID: 19083116
- Li X, Liu B, Cui J, Liu D, Ding S, Gilna B, Luo J, Fang Z, Cao W, Han Z. No evidence of persistent effects of continuously planted transgenic insect-resistant cotton on soil microorganisms. Plant Soil 2011; 339:247 - 57; http://dx.doi.org/10.1007/s11104-010-0572-2
- Quintela ED. Manejo integrado de pragas do feijoeiro. 2001. http://ainfo.cnptia.embrapa.br/digital/bitstream/CNPAF/19418/1/circ_46.pdf Accessed 2013 Sept. 15.
- SAS Institute. Help and documentation – 9.1.3. Cary, NC: SAS Institute Inc., 2002-2004.
- Badji CA, Guedes RNC, Silva AA, Araújo RA. Impact of deltamethrin on arthropods in maize under conventional and no-tillage cultivation. Crop Prot 2004; 23:1031 - 9; http://dx.doi.org/10.1016/j.cropro.2004.03.003