Abstract
Models of Clostridium difficile infection (C. difficile) have been used extensively for Clostridium difficile (C. difficile) research. The hamster model of C. difficile infection has been most extensively employed for the study of C. difficile and this has been used in many different areas of research, including the induction of C. difficile, the testing of new treatments, population dynamics and characterization of virulence. Investigations using in vitro models for C. difficile introduced the concept of colonization resistance, evaluated the role of antibiotics in C. difficile development, explored population dynamics and have been useful in the evaluation of C. difficile treatments. Experiments using models have major advantages over clinical studies and have been indispensible in furthering C. difficile research. It is important for future study programs to carefully consider the approach to use and therefore be better placed to inform the design and interpretation of clinical studies.
C. difficile Infection
Clostridium difficile (C. difficile) was first described in 1935 by Hall and O’Toole,Citation1 but it was 40 years before it was identified as the primary cause of pseudomembranous colitis, and shown to be the main organism isolated from the feces of patients with diarrhea undergoing clindamycin treatment.Citation1-Citation4 These early studies and experimental animal model observations revealed C. difficile as a potential emerging pathogen capable of causing severe gastrointestinal disease in individuals undergoing antibiotic therapy. C. difficile infection (CDI) has increasingly become a problem in hospitals with outbreaks of infection which can be difficult to control.Citation5 CDI is a toxin-mediated intestinal disease, and extraintestinal manifestations are rare. C. difficile strains can produce two major toxins, termed A and B, which crucially mediate the intestinal inflammation and pathology characteristic of CDI. Some strains are non-toxigenic, i.e., do not produce either toxins A or B, while occasional strains produce toxin B but not toxin A. The latter appear capable of causing CDI. Strains that produce toxin A but not toxin B have not been convincingly described. C. difficile infection is thought to arise as a result of antimicrobial-mediated depletion of the normal gut flora allowing C. difficile proliferation and toxin production.Citation6 Clinical outcomes can range from asymptomatic colonization, through mild self-limiting diarrhea to more severe disease syndromes including abdominal pain, fever and leucocytosis. Fulminant or severe complicated CDI is characterized by inflammatory lesions and the formation of pseudomembranes in the colon. This can cause life threatening pseudomembranous colitis (PMC) which can result in toxic megacolon, bowel perforation sepsis shock and death.Citation5,Citation6 Those at greatest risk for C. difficile tend to be those who have received antibiotic therapy, particularly the hospitalized elderly, those with serious underlying disease and patients undergoing surgery.Citation6,Citation7 All antibiotics have been associated with C. difficile, but some carry a higher risk than others including clindamycin,cephalosporins and fluorquinolones,Citation7 while others (piperacillin-tazobactam, tigecycline) are rarely implicated in the disease. Symptomatic recurrences are a major problem in CDI patients:Citation8 either relapse due the original infecting strain, or re-infection with C. difficile spores.Citation8,Citation9
Despite the discovery of C. difficile as the etiological agent of PMC in the late 1970s, antimicrobial treatments for the disase have changed little in the intervening period. Antimicrobial treatments for CDI remain limited to oral metronidazole (400–500 mg three-times daily) or vancomycin (125 mg four-times daily).Citation10 Until recently, metronidazole was the preferred option for reasons of cost, and lower risk of vancomycin resistance selection. Early studies demonstrated little difference between metronidazole and vancomycin in terms of response or recurrence rates,Citation11,Citation12 although response time was faster with the latter.Citation13 More recent reports of poor metronidaozle efficacy, particularly for disease attributable to apparently hypervirulent C. difficile PCR ribotype 027 (NAP1/BI),Citation14 and reports of reduced metronidazole susceptibilityCitation15 have led to doubts over the efficacy of this drug. The need for new treatments for CDI, and the search for the mechanisms behind its pathogenesis mean that a number of experimental model approaches have been employed.
Models and C. difficile
Experimental animal models for Clostridium difficile have existed for over 30 years, with a number of studies using experimental clindamycin-induced enterocolitis in hamsters as a model of pseudomembranous colitis in humans.Citation16-Citation20 In vitro models have been also been used since the late 1970s and have developed in the intervening years from small test-tube fecal emulsions to multi-stage chemostat models. Experimental studies using models have major advantages over clinical studies. In animal models these include the availability of study subjects, standardization of disease severity, the ability to perform invasive tests, extensive tissue sampling and the possibility to test prophylactic and novel treatment strategies. A wide range of animal models of C. difficile have been developed and these have been indispensable in providing insight into disease pathophysiology, including population dynamics, colonization and environmental factors and potential treatment options.Citation16,Citation21-Citation25 In particular, early studies of C. difficile using a hamster model enabled the link to be made between antibiotic associated colitis and the C. difficile toxins.Citation20,Citation21,Citation26,Citation27 More recent work has substantiated the evidence in relation to the action of C. difficile major toxins, and further information on immune responses and associated pathology.Citation28-Citation30
Animal Models of Clostridium difficile Infection
Many different animal models have been used to study the mechanisms of CDI, including small animals such as hamsters, mice, rats, rabbits, hares, guinea pigs, prairie dogs and quails.Citation30,Citation32-Citation42 There have also been a number of limited descriptions of the use of larger animals including foals, piglets and monkeys.Citation43-Citation45 Zebrafish embryos have also recently been used to examine the action of C. difficile toxins.Citation46 The hamster model is the most well described having been used for the greatest variety of studies including testing of new potential antibacterials, the effects of C. difficile on the immune system, prophylactic treatments and population dynamics.Citation16,Citation30,Citation47-Citation50
Hamster Models
Hamster models, in particular Syrian hamsters, have been most extensively employed for the study of CDICitation2-Citation4,Citation16-Citation21,Citation26,Citation27,Citation29,Citation30,Citation32,Citation51. C. difficile in hamsters shares some of the recognized features of C. difficile isolated from humans, especially with respect to the susceptibility of the animal to infection following the administration of antimicrobial agents, but there are also key differences. In the hamster model, disease is induced by the administration of antibiotics which disrupt the normal gut flora, following infection with C. difficile, hamsters display many of the pathophysiological features seen in humans.Citation19 In addition to overall deterioration of the animal, there are changes in the appearance of the gastrointestinal tract in particular in the colon and cecum. Redness, inflammation, fluid accumulation and enlargement of the colon are usually seen, accompanied by a decrease in gut motility. If left untreated the disease is rapidly fatal in hamsters, and therefore, the endpoint of any experiment is the survival rate in days; actually, animal welfare legislation in some countries now means that animal euthanasia must occur before death caused by CDI. This rapidly and uniformly fatal disease pattern is not characteristic of human C. difficile, and a key drawback of the model is that hamsters do not typically develop diarrhea. They may occasionally develop “wet tail”, in which the hamster displays symptoms of watery diarrhea, lethargy, irritability and refuses food, but invariably, this leads to death.Citation49,Citation51 Thus, in the context of C. difficile treatment experiments, the hamster model is actually a prevention of death model.
The first hamster model was described by Small in 1968,Citation52 in which lincomycin hydrochloride was reported to cause fatal enterocolitis. Early studies with hamster models aimed to detect and characterize what was referred to as the “transmissible agent” in clindamycin-associated enterocolitis. A number of studies reported this transmissible agent to be a Clostridium species.Citation3,Citation4,Citation16,Citation53 Bartlett et al.Citation27 reported that clindamycin-associated colitis in hamsters was due to a clindamycin-resistant, toxin producing clostridial strain; however, they conceived that this may not be the only agent or toxin responsible. These early C. difficile models enabled elucidation of the etiological mechanism for pseudomembranous colitisCitation20 and then the identification of specific therapies. shows the main uses of hamster models, which have included toxin and strain characterization, tests for new treatment strategies, studies into the population dynamics and colonization capacity of C. difficile in the intestinal environment, investigations into virulence determinants, the effects of different diets and characterization of immune responses.
Table 1. Summary of animal C. difficile model studies
Colonization Studies in the Hamster Model
Colonization and maturation of the infant gut flora following birth have been well studied using the hamster model.Citation54-Citation57 It was hoped that the hamster model may explain the mechanism whereby C. difficile colonizes the intestinal tract of human infants in both the presence and absence of disease.Citation55 C. difficile colonizes around 50% of humans (depending on the extent of neonatal transmission) during the first few months of life,Citation58 but these infants remain asymptomatic despite large quantities of C. difficile toxin being present. Toxin production by C. difficile can be demonstrated in both healthy and symptomatic human infants and has been shown to be present in infant feces at a concentration similar to that found in the feces of adults with pseudomembranous colitis.Citation55 As the microflora within the infant intestine matures with infant age, C. difficile numbers decline, and by the age of two the gut flora resemble that of an adult without the presence of C. difficile.Citation59 A number of studies have used animal models to confirm the presence or absence of specific receptors for the C. difficile toxins. Work by Rolfe et al.Citation56 in hamster models showed that there was a specific receptor for toxin A in the adult and hamster brush border membrane, but they were unable to demonstrate a similar receptor for toxin B. They were also unable to demonstrate any differences in the binding of toxin A in the infant and adult hamster model. Eglow et al.Citation60 used a rabbit model and reported that newborn rabbit illeal brush border membranes do not possess specific binding sites for toxin A but noted that the number of receptors increased as the rabbit matured. In a further study by Rolfe et al.Citation61 it was confirmed that the receptors were present in the human infant intestine but that it was the presence of both unbound non-immunoglobulin and bound immunoglobulin fractions of human milk that inhibited the binding of toxin A to the purified receptor.
Role of C. difficile Toxins in the Hamster Model
Many papers describing the use of hamster models have investigated the action and role of C. difficile toxins.Citation29,Citation50,Citation62-Citation64 Initial studies to characterize C. difficile toxins were performed using hamster models, in which it was demonstrated that the role of C. difficile toxins in causing disease was similar in hamsters and humans. Taylor et al.Citation65 reported the isolation of two toxins from C. difficile with physical and biological differences using models of hamsters, rabbits and guinea pigs. These findings suggested that the second toxin identified (toxin B) may have an important role in the clinical and pathological symptoms ascribed to C. difficile.
There has been considerable debate regarding the relative importance of each toxin; it was originally believed that toxin A was more important than toxin B.Citation66-Citation69 These studies involved the administration of toxin A alone to hamsters which resulted in symptoms typical of C. difficile. In contrast, administration of toxin B did not elicit the same effect, unless intestinal damage was present or the toxin was co-administered with toxin A. This suggested there may be a synergistic effect, or that toxin B only had an effect following prior tissue damage by toxin A.
In 2009 Lyras et al.Citation29 and Carter et al.Citation64 provided evidence that toxin B was potentially the more important toxin and was essential for virulence. Lyras et al. used four independently derived toxin A or B mutants of C. difficile, which were characterized using a hamster model. The results demonstrated that toxin B was an essential virulence factor since disruption of the C. difficile B gene led to a significantly attenuated virulence phenotype. Those isolates which produced toxin B maintained a wild type phenotype, confirming the importance of toxin B in pathogenesis. Even the presence of toxin A at higher levels than toxin B was not lethal in the hamster model. Toxin A may still play an important part in the disease process, but appears from this study not to be an essential virulence determinant. This claim has major implications for future work on C. difficile. The authors suggested that it is important that studies are not solely performed on purified toxin and that the importance of the toxins in the context of the natural infection process is crucial to determining the role of toxins in disease.Citation29 Notably, however, a study by Kuehne et al.Citation70 reasserts the importance of both toxins A and B. In this study isogenic mutants of C. difficile producing either toxin A or B alone were shown to be able to cause fulminant disease in a hamster model of infection. Additionally, they also constructed a first ever double-mutant strain of C. diffiicile, in which both genes were inactivated; this completely attenuated bacterial virulence.Citation70 The conundrum surrounding the respective virulence of toxins A and B remains and may vary according to the host species. It should be noted that optimized treatment of human C. difficile using monoclonal anti-toxin antibodies may require the presence of high-affinity antibodies against both toxins.Citation71
Animal models are particularly useful in the study of immunological aspects of C. difficile and imuno-modifying treatment. However, as the following studies highlight, animal models may show variation in host response, and there may be considerable differences between different species. The hamster model has been used to study the efficacy of various immunization strategies including inactivated toxin, antibodies to recombinant toxin A and B, and surface layer proteins (SLP).Citation28,Citation72-Citation74 Kink and Williams described production of antibodies to recombinant toxin A and B proteins and their oral administration to clindamycin-treated hamsters (1 mg/100 g body weight), which were then exposed to C. difficile. They found that antibodies to both toxins A and B were required to protect against C. difficile. They also noted that hamsters treated with antitoxin, in contrast to vancomycin, did not suffer relapse and were refractory to reinfection.Citation28 Giannasca et al.Citation72 used a series of passive and active dosaging regimens, including routes of administration, to evaluate the efficacy of toxoid vaccine preparations in a clindmycin-induced (0.5 mg) C. difficile hamster model. They concluded that rectal administration, in conjunction with intramuscular vaccination conferred full protection against C. difficile, whereas other mucosal routes did not. Passive immunization was also successful, in a dose-dependant manner and the authors underlined the importance of circulating antibodies in protection against C. difficile.Citation72 This confirmed earlier work investigating a formalin-inactivated toxoid vaccine which also indicated that a combination of mucosal and parenteral admininistration routes conferred the greatest protection against disease.Citation75 However, with the exception of rechallenge experiments by Kink and Williams, which ran for up to 70 d, the time courses of these experiments was fairly short (14–20 d). This means that conclusions about the protection afforded by these treatments in the long-term cannot be drawn.
O’Brien et al. showed significantly prolonged survival in clindamycin-induced C. difficile model hamsters treated with anti-surface layer protein (SLP) antibodies compared with control hamsters (given rabbit anti-maltose binding protein, serum or left untreated) and the authors linked this to enhanced phagocytosis. However, despite prolonged survival (median 157 h) all hamsters in all groups succumbed to infection within 2–5 d post-C. difficile challenge, with no difference in time to first symptoms.Citation73 This group subsequently examined SLP as a component of a vaccine administered to clindamycin-treated hamsters according to several different immunization regimens. Hamsters were challenged with 105 cfu C. difficile 14 d after the final immunization dose. All hamsters died within 48 h of C. difficile exposure, regardless of immunization route, presence of adjuvant and serum SLP IgG titer. A non-challenge BALB/c mouse model showed stronger antibody responses. The authors reported considerable variation in responses among both hamsters and mice.Citation74
Induction Studies in the Hamster Model
Studies into the induction of C. difficile in hamsters have been performed by several groups.Citation17,Citation26,Citation48,Citation76-Citation79 Toshniwal et al. tested the effects of the orogastric administration of tetracyclines and observed that this caused diarrhea and death with evidence of hemorrhagic typhlitis.Citation17 They were able to culture tetracycline resistant C. difficile from the stools of the hamsters and reported that the lesions resembled those induced by clindamycin that have been attributed to C. difficile toxin.Citation26,Citation27,Citation80 Erbight et al.Citation78 tested the effects of 8 cephalosporins in a hamster model. The study concluded that poorly absorbed cephalosporins were effective in preventing clindamycin-induced cecitis in hamsters as their minimum inhibitory concentrations for C. difficile were higher than those of metronizadole and vancomycin. However they did not consider them to be more effective antibiotics for treating cases of C. difficile associated disease.Citation78 They also observed that cepaholosporins induced C. difficile in hamsters, as in humans. It is noteworthy that they did not describe the administration of C. difficile, and this was perhaps left to chance rather than a controlled administration. Likewise, Onderdonk et al. did not describe how C. difficile was administered in their comparative study of the effects of clindamycin and its metabolites in a hamster model of antibiotic associated colitis.Citation81 They reported no association between antimicrobial potency and AACD50 values, but it is possible that the likely uncontrolled C. difficile exposure could have influenced these results. The authors observed the reduction of de-N-methylclindamycin sulphoxide to the considerably more active de-N-methylclindamycin in hamsters. This had not previously been observed in rats and underscores some of the difficulties in comparing data between different animal models and crucially when extrapolating results to human C. difficile.
Larson and BorrielloCitation77 compared various antibiotics for their propensity to induce C. difficile in hamsters. Infection occurred after very small doses of ampicillin, clindamycin, flucloxacillin or cefuroxime were administered and little difference in the degree of susceptibility they induced was seen. This was measured by calculating 50% lethal doses in CFU for hamsters following antibiotic treatment administered at various intervals. Clindamycin administration induced a longer period of susceptibility to C. difficile. The authors suggested while the results could not be applied to humans, they provided further lines of investigation into how antibiotics may differ in terms of C. difficile risk. This was also the first study to attempt to control hamster exposure to C. difficile, which had previously largely been left to chance. By standardising the C. difficile strain and inoculum, and providing a sterile environment, the inherent problem of re-infection via contaminated environments was tackled. Larson and Welch drew on this work to examine colonization resistance in vivo and in vitro following clindamycin- or ampicilin-induced toxin production.Citation32 They demonstrated inhibition of C. difficile growth in hamsters and in hamster cecal contents in vitro by normal (non-antibiotic exposed) cecal contents. These inhibitory properties were lost upon filtering, freezing/thawing and dilution. The authors also reported longer inhibition in cecal contents of clindamycin- vs. ampicillin-treated hamsters, in agreement with the previous study. In a study demonstrating prevention of antibiotic-induced cecitis by administration of normal cecal homogenates, Wilson et al. used vancomycin (200 mg/kg) to successfully induce C. difficile in hamsters. Extrapolation from the drug elimination curve suggested that deaths from cecitis would have occurred when vancomcyin levels fell below the MIC of C. difficile, on days 6–10 after administration.Citation82 Similarly, in a recent comparative study of the propensities of oritavancin (50 mg/kg), clindamycin (100 mg/kg) and vancomycin (50 mg/kg) to induce C. difficile in the hamster modelCitation83 vancomcyin and clindamycin-treated hamsters died within 6 d of treatment, demonstrating the potential of vancomycin to induce C. difficile. Only oritavancin-treated hamsters survived until the experimental end-point (day 20).
New Potential Antibiotics and Therapies for Treatment of C. difficile Infection Using the Hamster Model
The identification of new potential therapies for C. difficile is a research priority, and many studies have reported on the use of the hamster model for this aim (). Notable studies include the following. The macrolide antibiotics tiacumicins B and C were tested in vivo in a hamster model of antibiotic associated colitis. Administration of tiacumicins at 0.2, 1 or 5 mg/kg hamster body weight protected all clindamycin-treated hamsters (100 mg of clindamycin per kg of body weight intraperitoneally) exposed to C. difficile,Citation84 while vancomycin was only effective at higher doses. More recently tiacumicin B, now known as fidaxomicin (OPT-80 or PAR-101),Citation85,Citation86 has shown good results in a phase III trial in comparison with vancomycin for the treatment of C. difficile infection. Recurrent C. difficile was significantly less frequent in fidaxomicin recipients (13.3% vs. 24%).Citation85
Table 2. Aspects investigated using mouse models
The efficacy of rifaximin a novel non absorbed antibiotic was tested in a hamster model.Citation87 Hamsters were given subcutaneous clindamycin (10 mg/kg), 24 h later challenged with C. difficile, and then given either vancomycin (50 mg/kg) or rifaximin (100, 50 or 25 mg/kg) for 5 d. Rifaximin was reported to be as effective as vancomycin in the prevention and treatment of colitis caused by the two strains tested, and no relapse occurred with this agent. Vancomycin was associated with significantly more recurrences than rifaximin in C. difficile due to strain VPI 10463 (75% vs. 0%, p < 0.01). Rifaximin has been used to treat C. difficile in humans although it is not licensed for this indication; there have been encouraging case reports notably in patients who have experienced multiple prior recurrences of C. difficile, although resistance emergence may be an Achilles heel.Citation87
Tolevamer is a novel toxin binding polymer and was investigated for the treatment of C. difficile in a hamster model.Citation88,Citation89 Studies involved challenging hamsters with C. difficile, giving clindamycin subcutaneously (10 mg/kg) 24 h later followed by tolevamer after a further 24 h. Tolevamer dosages of 500, 1000 and 1500 mg/kg/day led to survival rates of 90%, 70% and 70%, respectively. Tolevamer reduced the incidence of diarrhea and led to higher survival rates. The authors noted that there was no relapse of symptoms after discontinuation of dosing, compared with hamsters given metronidazole that died within 72 h of treatment cessation. The drug was also compared with an additional toxin binding compound cholestyramine; 80% of tolevamer treated hamsters survived compared with only 10% of those given cholestyramine.Citation88,Citation89 The tissue histology of the ceca of hamsters treated with tolevamer appeared normal, compared with saline treated controls. These results were consistent with the non-antibacterial activity of the compound. Despite the promising hamster data, the clinical development of tolevamer was halted in 2008 due to the failure to demonstrate non-inferiority in comparison with metronidazole or vancomycin in phase III studies.Citation90,Citation91
Rifalazil a new benzoxazinorifamycin with activity against Mycobacterium tuberculosis and Gram-positive bacteria was compared with vancomycin for the prevention and treatment of clindamycin-induced cecitis in a hamster model of C. difficile.Citation92,Citation93 Hamsters were injected subcutaneously with clindamycin phosphate (10 mg/kg), 24 h later challenged with C. difficile, and then either vancomycin (50 mg/kg) or rifalazil (20 mg/kg). No animals given vancomycin or rifalazil developed illness after 7 d. Within the ceca of rifalazil treated animals there was no demonstrable epithelial cell damage and significantly less edema and neutrophil infiltration when compared with vancomycin treated animals; hamsters relapsed following discontinuation of vancomycin but not rifalazil treatment. The authors suggested that once daily rifalazil may be effective for the treatment of C. difficile.Citation93
Freeman et al.Citation94 tested the efficiency of ramoplanin (a novel glycolipodepsipeptide) and vancomycin in a hamster model and in an in vitro gut model (see section on in vitro models). Hamsters were given 104 cfu C. difficile by mouth and housed in germ-free conditions with HEPA filtered air. Symptomatic disease in the hamster was initiated by administration of 100 mg/kg clindamycin after 24 h. Ramoplanin and vancomycin were administered after a further 24 h and the ceca analyzed between 1 and 10 d following the clindamycin challenge. C. difficile symptoms (watery feces) were present in 33% of both ramoplanin- and vancomycin-treated hamsters; control hamsters were uniformly symptomatic (wet tail, watery or hemorrhagic cecal contents). All animals in both treatment groups were asymptomatic by day 4 and thereafter. On day 4, C. difficile total counts and spores were quantifiable in 75 and 100% of vancomycin-treated hamsters, respectively, but C. difficile was undetectable in all ramoplanin-treated animals. The spore persistence results were consistent with those generated in an in vitro gut model of human C. difficile. Ramoplanin was suggested to be superior at killing/inhibiting spores and thus may be more effective at preventing C. difficile occurrence due to spore recrudescence. Ramoplanin has completed a phase II clinical study in which 25/29 patients with C. difficile respondedCitation95; it remains unclear whether the hamster and gut model data showing spore suppression will translate into superior clinical efficacy.
Nitazoxanide (NTZ) is a thiazolide derivative, was initially developed as an antiparasitic drug, but is active against a variety of enteric parasitic pathogens including protozoa in humans and animals.Citation96-Citation98 In vitro studies showed inhibition of C. difficile strains.Citation98 In vivo experiments were performed using a hamster model. Hamsters pre-treated with clindamycin but then only saline died within 60–70 h after challenge with 105 C. difficile; conversely, NTZ (15, 7.5 or 3 mg/100 g body weight), vancomycin (5 mg/100 g body weight) or metronizadole (15 mg/100 g body weight) prevented the rapid onset of disease. Most of the hamsters died within two weeks of stopping the treatment and it was unclear whether this was due to reinfection with an environmental strain of C. difficile, or possible relapse due to a failure to eradicate the existing infection. As a result of these experiments vancomycin was reported to be the most effective antibiotic, based on 3 out of 10 hamsters surviving, compared with none of those receiving MTZ.Citation25 These results are similar to those found in a study by Fekety et al.Citation48 comparing the effects of several other antibiotics to vancomycin and metronizadole. In this study similar contrasting efficiencies for vancomycin and metronizadole were reported, most likely as a result of vancomycin reaching higher concentrations in the gut than metronizadole.
Dong et al.Citation22 tested the lipopeptide antibiotic daptomycin (LY146032) in hamsters. Animals were administered 104 cfu C. difficile, then 5 d later they were given 1 mg clindamycin, followed by 5 d of treatment with either daptomycin or vancomycin (both 0.05 mg/day). The authors made no reference to the conditions under which the hamsters were kept. Administration of daptomycin delayed death in a hamster model and required a lower dose than that required for equivalent protection by vancomycin. The authors were unable to confirm a reason for the improved outcome, but suggested that it may be due to longer persistence of daptomycin in the gut or improved adherence to mucosal cells. Additionally, they suggested that there may be differential effects on intestinal flora, but substantive data are not available.
REP3123, a synthetic methionyl-tRNA synthtase inhibitor that inhibits toxin production and sporulation in vitro, was tested in a C. difficile hamster modelCitation99 After a subcutaneous dose of clindamycin (50 mg/kg), followed 24 h later by C. difficile (3.2 × 107cfu/ml), REP3123 or vancomycin (at dosages of 5 and 0.5 mg/kg bd) was administered for 5 d commencing after a further 24 h. At both dosages REP3123 was more effective than vancomycin in terms of hamster survival up to the end of the study (day 33), although a maximum of only 75% of animals survived.Citation99 Further study would be required to determine optimum dosage and whether these results could translate into effective treatment of human C. difficile.
Oritavancin is a lipoglycopeptide with good in vitro anti-C. difficile activity. Hamster and gut-model derived data both indicate anti-spore activity, and reduced risk of spore recrudescence/recurrence with oritavancin vs. vancomcyin. Marquis et al. described a comparative study of C. difficile induction by oritavancin (50 mg/kg) vancomycin (50 mg/kg) and clindamycin (100 mg/kg) in hamsters. All received 105 cfu C. difficile spores.Citation83 The study was controlled by including a vehicle-only (polyethylene glycol 400) group and hamsters given clindamycin (100 mg/kg) but no spores. The authors reported 100% survival of oritavancin-treated hamsters at the experimental endpoint compared with no survival by day 5 and 6 in vancomycin- and clindamycin-treated groups, respectively. Microbiological analysis of cecal contents found detectable levels of C. difficile toxin, spores and vegetative cells in only the clindamycin- (and spore inoculated) and vancomycin-treated hamsters. Investigations in a human gut model had previously indicated that oritavancin reduced spores to undetectable levels following clindamycin-induced germination and toxin production. In contrast, although total viable counts were reduced by vancomycin administration, spores remained.Citation100 The anti-spore activity of oritavancin is promising and further study of this compound continues.
Other Treatment Strategies Investigated Using the Hamster Model
The probiotic yeast Saccharomyces boulardii has been investigated as a potential exogenously administered agent for maintaining colonization resistance during antibiotic therapy.Citation101 S. boulardii decreased C. difficile associated cecitis and death in hamstersCitation101,Citation102 and antibiotic associated diarrhea in humans.Citation103 A hamster model of recurrent C. difficile was described by Elmer et al.Citation38 The authors suggested that S. boulardii administration may help prevent extensive overgrowth of C. difficile and could be used to reduce the high incidence of relapse after vancomycin treatment in humans. In a more recent clinical trial, S. boulardii was administered to patients in combination with standard antibacterials for treatment of C. difficile;Citation104 the authors concluded that this regimen was successful for recurrent C. difficile disease but had no effect on an initial C. difficile infection. Of some concern, examples of cross infection have been reported (i.e., from a S. boulardii treated patient) highlighting the potential virulence of this yeast in humans. Furthermore, S. boulardii isolates obtained from varied commercial sources were found to differ in virulence in a mouse model, suggesting a lack of uniformity of yeast strains in such preparations.Citation105
Diet alteration to modify intestinal flora has been investigated as a means of protecting against C. difficile. Frankel et al.Citation24 studied the role of a high fiber diet in the hamster model and found it delayed disease onset and prolonged survival from C. difficle ileocecitis. Increased intake of fiber by hamsters normalized the gut structure and improved absorption. Toxin positivity and stool liquidity were significantly reduced and survival times were improved by 34%. It is unclear if these findings are relevant to human C. difficile. A fiber rich diet may be beneficial for a patient at risk of C. difficile, although if this necessitated tube feeding, there may actually be an increased chance of infection.Citation106,Citation107 In a similar study, Blankenship-Paris et al.Citation33,Citation41 used a hamster model to investigate the effects of a high-fat diet, based on previous observations that hamsters fed a this type of diet were more susceptible to C. difficile enterocolitis. Hamsters were fed either a high fat or a control diet and then challenged with a toxigenic C. difficile strain. There was an increased rate of morbidity (80% vs. 11%, p ≤ 0.05) in hamsters fed the high fat diet. C. difficile was recovered from the ceca of affected hamsters and the presence of toxins A and B could be demonstrated. In vitro tests showed that cecal flora from both high-fat treated and control hamsters inhibited C. difficile growth, suggesting that impaired colonization resistance was not a factor in disease development in this study.Citation41
Naaber et al.Citation108 used a hamster model (and mouse model) to investigate bacterial translocation, intestinal microflora and the morphological changes within the intestinal mucosa as a result of C. difficile. The quantitative composition of luminal and mucosal microflora was evaluated as well as inflammatory changes of the mucosa and bacterial translocation into the blood, liver, spleen and mesenteric lymph nodes. They concluded that in mild C. difficile cases the extent of disturbance of intestinal microflora appeared to be more important in translocation than inflammatory activity in the mucosa. In fatal cases translocation was frequent with facultative species and C. difficile was present. The authors also investigated the protective effect of both probiotic (lactobacilli) and a prebiotic (xylitol) and reported that these were protective against C. difficile in these models.Citation108
Population Dynamics and Colonization Studies in the Hamster Model
Hamster models have also been used in studies on population dynamics and colonization. Wilson et al. compared the transit of C. difficile spores and vegetative cells to a 51Cr tracer in hamsters.Citation50 The majority of spores germinated within 1 h of inoculation (intragastric). Two independent forms of inhibition were seen: initial death of cells, which was unaffected by antimicrobial treatment, and a further inhibition of germination and proliferation, which was affected by antimicrobial treatment. Wilson et al. calculated dilution rates in hamsters and highlighted the similarity in dynamics between the hamster cecum and continuous culture systems. Wilson and SheagrenCitation109 studied the interplay between toxigenic and non toxigenic C. difficile strains. They induced colitis with clindamycin in a hamster model and found that administration of a non-toxigenic strain after 24 h was protective against a subsequent toxigenic strain administered 48 h later. Borriello and Barclay reported that prior colonization of clindamycin-treated hamsters (5 mg) with a non-toxigenic C. difficile strain provided protection against colonization by a toxigenic strain. Hamsters were housed individually and in a clean environment (autoclaved cages, food, water bottles and bedding). C. difficile inocula were administered orally and were derived from overnight cultures of 4 C. difficile strains of varying toxigenicity. C. difficile inocula were not standardized (range 3 × 106–3 × 109 cfu) and spores were not enumerated. All hamsters given toxigenic C. difficile alone developed disease and died within 48h. In contrast, 13 of 18 hamsters receiving both toxigenic and non-toxigenic C. difficile survived to day 25. The authors drew attention to the fact that colonization by the non-toxigenic C. difficile strain did not confer full protection over the longer term, but highlighted the potential use of non-toxigenic strains.Citation110 Szczesny et al.Citation111 studied the interactions between toxin deficient strains of C. difficile and a toxigenic strain. They observed that toxin A or B deficient strains did not cause disease in the hamster, but when hamsters were subsequently infected with a toxigenic strain, clinical disease and death resulted. However on macroscopic and microscopic observation, histopathological changes were less evident following re-infection with the toxigenic strains.
Sambol et al.Citation47 also examined the use of non-toxigenic C. difficile strains to protect against C. difficile in hamsters. Animals were given clindamycin to induce colitis and then exposed to a non-toxigenic C. difficile strain 48 h later. This study involved higher initial dosing of clindamycin (30 mg/kg), compared with 7.5 mg/kg in the study by Wilson and Sheagren and an additional 24 h before the administration of a non-toxigenic strain. Also, hamsters were housed individually and more comprehensive testing was performed; toxigenic strains were administered after 5 and 40 d. Merrigan et al.Citation112 examined the protective effect of colonization with non-toxigenic strains during daily ampicillin or ceftriaxone administration. Hamsters were housed individually in isolator cages and were given antibiotics, followed by oral administration of 1 × 106 non-toxigenic cfu C. difficile spores 3 h later. All animals were colonized by day 3 (determined by culture of feces), and correlated with survival after challenge with toxigenic C. difficile. Data showed that the relative antibiotic resistance of the administered non-toxigenic strain (i.e., to the C. difficile inducing agent) affected the likelihood of successful colonization. Such issues will need to be overcome if this approach is to be translated into a successful prophylactic strategy against human C. difficile in patients receiving antibiotic therapy.
Characterization of Relative C. difficile Virulence in the Hamster Model
Hamster models have been used to characterize virulence determinants. Borriello et al.Citation21 studied a number of different toxigenic strains of C. difficile in hamsters to determine differences in virulence and try to correlate this to production of toxins A and B. They concluded that the high virulence of C. difficile was determined by efficient disease-inducing colonization of the gut, and the ability to rapidly generate high levels of toxin A in vivo. They postulated a number of reasons for the difference in virulence of different strains, including ability of different strains to associate with the gut mucosa. Alternatively, there may be an affect on the rate of repair of the gut epithelium, and that in order to cause disease the rate of toxin production must be more than a certain minimum such that the rate of tissue damage exceeds that of tissue repair. Human data are unavailable to confirm or refute this theory.
Sambol et al.Citation51 compared the virulence in hamsters of five different toxigenic strains of known human epidemiological importance. They demonstrated pathogenicity differences between toxin variant strains and standard strains but no observable differences among standard strains. They concluded that human epidemic C. difficile strains predictably cause disease in the hamster model, but suggest that that there may be host factors affecting the strain virulence, such as pre-existing antibodies to toxins (as shown by Kyne et al.Citation113), but which are not present in the hamster model. This work was published prior to the emergence of C. difficile NAP1/BI/027 as strain associated with increased morbidity and mortality. Subsequent work by this group investigated historical and current epidemic BI (NAO1/BI/027) strains against toxinotype 0 isolates. The recent epidemic strain BI6 showed more rapid time to death from colonization, but there was little strain difference in time to death from challenge. The authors also noted high mortality with no prior signs of morbidity among hamsters infected with BI strains, consistent with clinical observations.Citation49
Goulding et al. investigated the profiles of infection and pathology of C. difficile strains 630 and B1 (as distinct from 027/BI/NAP1), highlighting the usefulness of the hamster model in terms of ability to study host reactions during the disease process.Citation114 Study hamsters were of similar weight, and the environment highly controlled throughout the experiment. In addition to sterile cages, food, water and bedding, hamsters were re-housed in a sterile environment following infection with C. difficile spores and every 24 h thereafter. Environmental contamination was monitored using hamsters treated with clindamycin alone, alongside infected animals. Detailed histological analysis and electron microscopy of hamster gut, alongside quantitation of C. difficile, showed clear differences in disease pathology and time course between the two C. difficile strains. The B1 strain caused more severe pathology and shorter time to death, and larger numbers of bacteria were present at the mucosal surface and within non-phagocytic cells; strain 630 was more often found in the crypt regions.
Mice and Rat Models
In a similar manner to the hamster model, mouse models have been used extensively to study the pathogenic process of C. difficile infection as well as toxin production (). Mice have also been used to determine the role of environmental conditions in modulating infection. Models have included the conventional mouse model, gnotobiotic mice, monoaxenic mice and the human micro biota-associated mouse model.Citation115 There has been considerable study of specific toxin-mediated and immunological events in tissue explants or ileal loops originating in mice, rats and rabbits. Some important studies relating to the elucidation of relative toxin A and B activity are considered here, but many studies are highly specific and beyond the scope of this review. Rat ileal loop models have provided useful insights into the actions of toxins. Characterization of the differing actions of C. difficile toxins A, B and an additional compound (which the authors called toxin C) were studied in the small intestine and colons of rats.Citation116 Fluid secretion in the small intestine was caused by all toxins, but only toxins A and C caused shedding of epithelial cells from the villi without visible damage to the crypt cells. In the colon, toxin A caused secretion and shedding of epithelial cells. The authors concluded that the differences in action of the various toxins may explain the differences in the broad spectrum of disease and intestinal disorders caused by C. difficile.Citation116
Savidge et al. transplanted human intestinal xenografts into immunodeficient mice to create a chimeric animal model which was used to examine the actions of C. difficile toxins on human intestinal tissue.Citation117 This study questioned the traditional view that toxin B acts as a cytotoxin and toxin A as an enterotoxin. They were able to show that toxin B, as well toxin A induced intestinal epithelial cell damage, increased mucosal permeability and caused an acute inflammatory response. They concluded that C. difficile toxin B is a potent inflammatory enterotoxin for the human intestine, and suggest that in future, therapeutic or vaccine strategies for C. difficile infection need to target both toxins. An explanation for the differences in responses to toxin B seen in earlier rodent models and the humanized mouse model is the absence of toxin B specific receptors in the rodent intestine. Riegler et al. 1995Citation69 found a strong correlation between the sensitivity of cells toward the action of the C. difficile toxins and the presence of toxin-specific receptors. It appears that the mechanisms employed by toxins A and B require specific receptors to which the toxins bind as an initial step in the intoxication process. While the detail of the receptors is not known, toxin A binds to receptors on the apical surface of enterocytes and toxin B binds to receptors on the basolateral surface.Citation118 Tissue damage that disrupts the intestinal mucosa must first occur to allow access to the basolateral receptors. This may explain why purified toxin B, which does not bind to the apical surface, did not elicit symptoms in the hamster model unless prior intestinal damage was present. If toxin A compromises the epithelial barrier at the apical surface, this may explain why sub-lethal concentrations of toxin A potentiate the activity of toxin B. This work suggested that if mutants of toxin A are as virulent as the wild type, then possibly another protein or compound produced by C. difficile may be responsible for priming the intestinal mucosa.Citation64
A rat ileal loop model was used to test the protective effect of a protease produced by the yeast S. boulardii for the prevention of C. difficile disease in humans.Citation119 The authors concluded that the protective effect was attributable to a yeast serine protease that hydrolized toxin A and inhibited binding of this toxin to its brush border glycoprotein receptor. This prevented the diarrhea and other intestinal effects of the toxin in the model. The relevance to the mechanism for possible prevention of C. difficile colonization in humans was not discussed. Further investigations into the influence of S. boulardii on fecal microbiota were made in a human-microbiota-associated mouse model.Citation120 Molecular analysis of the fecal flora using oligonucleotide probes demonstrated more rapid recovery from the deleterious effects of amoxicillin-clavulanate treatment to baseline levels in mice that had received S. boulardii.
A study by Corthier et al.Citation121 showed that it was possible to protect mice from inoculation with a toxigenic strain of C. difficile when they were previously inoculated with a strain of E. coli or Bifdiobacterium bifidum, although no mechanisms for this modulation were proposed. In a study by Wilson and FreterCitation122 the interactions between the entire fecal flora of hamsters and C. difficile (and E. coli) in gnotobiotic mice was investigated. The same interaction was also studied in a continuous–flow culture system. In each case the hamster flora suppressed the potential pathogens, the C. difficile population decreased, and the addition of the continuous culture contents decreased the size of the germfree mouse ceca back to normal size. It was suggested that an extract of the fecal flora contained a substance important for the colonization of the normal ceca flora. Results of a continuous culture model and the mouse mode concurred. Wilson and PeriniCitation123 did not use a mouse model but studied the effects of using the entire cecal flora from mice for elimination of C. difficile from the mouse cecum. They suggested that as yet unidentified commensal organisms competed more efficiently than C. difficile for the available nutrients, and that substances produced by indigenous flora may decrease the growth rate even when a nutritionally rich medium was available. Studies have shown that C. difficile loses viability when introduced into hamster cecal flora or human feces,Citation31,Citation123 suggesting nutrient depletion is not solely responsible for the effect. Boureau et al.Citation124 was able to isolate anaerobic strains from hamster flora which exhibited colonization resistance to C. difficile. These strains were unable to colonize the axenic mouse either alone or in combination, but were able to colonize a Bifidobacterium bifidium monoxenic mouse. They concluded that the contribution of enzymes from various strains were important for establishment of the colonization resistance effect.
A recent mouse model was described by Chen et al.Citation36 to overcome some of the problems associated with the current hamster models. They exposed C57Bl/6 mice to a mixture of antibiotics for 3 d. Clindamycin was administered 48 h later and mice were subsequently challenged with C. difficile of varying doses. They reported that the disease closely resembled human C. difficile, with typical histological features and would be valuable for testing of new treatment strategies and for future studies of pathogenesis. Disease in the mouse model varied in severity in accordance with the challenge dose. The mouse model offers potential benefits over hamsters as mouse specific reagents are more readily available and genetically modified animals are also more easily obtainable. Some more recent studies incorporating mouse models have extensively characterized C. difficile spores and the effect in the environment in relation to health care disinfection regimes.Citation125 Mice models were also used to investigate transmission of spores between immunocompetent and immunocompromised mice, the results of which may guide infection control strategies in the future. In this study Lawley et al.Citation126 showed that virulent C. difficile asymptomatically colonised the intestines of immunocompetent mice, and established a persistent carrier state. The authors demonstrated that high level C. difficile shedding following induction (“supershedder” state) promoted efficient transmission in contrast to the carrier state, which did not. Infected immunocompetent mice suffered mucosal inflammation, but infection of immunocompromised mice led to severe fatal disease, underlining the involvement of host factors in the C. difficile disease process. The authors concluded that the mouse models provided a useful tool for studying the transmission of spores in relation to C. difficile. In a further study by the same authors, a mouse model was used to characterize the nature of the C. difficile strain 630 spores.Citation127 They established the highly resistant nature of spores and also demonstrated the low infectious dose required to establish infection in a mouse model (≤ 7 spores per cm2).
Spore germination was also investigated in the mouse model by Giel et al.Citation128 Spores were able to germinate to a greater degree in antibiotic-treated mice, but this was attenuated by treatment with cholestyramine, which can bind bile salts. The authors suggested that results of this study, which also showed that populations of cecal bacteria from antibiotic-treated mice were less able to modify taurocholate, a spore germinant, were further evidence for the role of bile salts in in vivo germination of C. difficile. An aged mouse model has also recently been used to study disease progression following infection with a C. difficile NAP1/BI/027 strain.Citation129 The authors described the production of previously undocumented cecal and colonic cytokines, highlighting the role of the host response in disease development. This study provides a novel model for investigation of the host immune response and its relation to aging.
Investigations of the administration route and efficacy of C. difficile toxoid vaccines were performed in both hamsterCitation28,Citation72,Citation73 and BALB/c mouse models.Citation74 Ghose et al. also investigated transcutaneous immunization (TCI) of a toxoid A vaccine in Swiss Webster mice. As TCI is needle-free, it is a potentially attractive option for vaccine delivery. Good serum IgG levels, mucosal serum and stool anti-C. difficile toxin IgA levels and neutralizing antibody responses were seen following TCI with a toxoid A vaccine and C adjuvant.Citation130 Gardiner et al. vaccinated BALB/c mice with a DNA vaccine targeted to the receptor-binding region of toxin A and subjected them to intraperitoneal injection of toxin A over 2 weeks. The vaccine was immunogenic and protected mice against the effects of toxin challenge. The authors highlighted variation in immunogenicity on the basis of mouse strain and recommended further testing in other animal models.Citation131 Such observations highlight one of the drawbacks of the proliferation of new C. difficile animal models. Inter-model result variability raises uncertainly about which models are most predictive of human C. difficile.
Other Animal Models
Xia et al.Citation40 used a guinea pig model to study the effects of C. difficile toxin A to determine if stimulation of secretion and motility and the associated inflammatory response have a neurally mediated component. While a guinea pig model was described, the method involved removing segments of the small intestine, which were mounted into recording chambers where the effect of the toxin was measured.
Dabard et al.Citation37 showed that it was possible to reproduce disease experimentally in young axenic hares. They described the first proliferation of C. difficile disease in the digestive tract not induced by the ingestion of antibiotics; the significance of this observation remains uinclear. They concluded that C. difficile is the causal agent of neonatal diarrhea in conventional and gnotobiotic young hares, and that other clostridia enhanced its pathogenic effect. Additionally, they observed that C. difficile alone or associated with C. perfringens or C. tertium did not play any pathogenic role in young mice, rats or rabbits.
Triadafilopoulas et al.Citation132 used a rabbit ileal loop model and performed a number of experiments to study the effect of purified toxins A and B on intestinal secretion, epithelial permeability and morphology. After toxin A or B exposure intestinal permeability was measured by assessing the clearance of [3H] mannitol from the blood to the lumen. Toxin A (dose 5–100 mg/10cm illeal loop) caused a 3–5-fold increase in [3H] manitol permeability when compared with toxin B. Additionally more neutrophils were seen in the toxin A treated loops compared with the toxin B ones. Toxin A caused severe epithelial cell necrosis including destruction of villi and polymorphonuclear infiltration. By contrast, similar tests using toxin A on in vitro illeal explants revealed no effect on epithelial cell permeability, protein synthesis, release of alkaline phosphatase or morphology.
Interest in swine related C. difficile has increased markedly, noting the overlap with strains seen in humans. C. difficile has become the most commonly diagnosed cause of enteritis in neonatal pigs.Citation133-Citation135 In a study by Steele et al.,Citation43 germ-free piglets were consistently and extensively colonized after oral challenge with C. difficile PCR ribotpye 027. Piglets inoculated with a non-toxigenic C. difficile strain showed no signs of disease. By altering the dose and piglet age, it was possible to induce either acute and severe systemic infection or milder chronic disease. Gastrointestinal and systemic symptoms were seen along with characteristic mucosal lesions of the large bowel. Additionally, C. difficile toxins (as measured by a cytotoxicity assay) were detected in feces, body fluids and serum, along with increased serum interleukin 8 levels, in piglets with severe disease. The piglets infected with C. difficile appeared to mimic many of the key characteristics observed in humans, and thus this model could potentially be exploited to investigate why some strains are associated with more severe C. difficile.
Prairie dogs have been described as potentially useful models in studies of gallstone formation and billary tract motility.Citation136 As their bile composition and extrahepetic billary anatomy are similar to that in the human, they have been described as a potentially useful model for Clostridium difficile induced colitis.Citation42 In the study the sample size was small (6 test and 6 control animals), but the results showed that the dogs developed pseudomembranous cecitis following a single dose of antibiotic, and survived for up to 4 weeks.Citation42 The practical issues surrounding the use of large numbers of dogs appear to have precluded further use of this approach. There is limited information on the utility of foals as a model for C. difficile. Arroyo et al.Citation45 described colonization of the gastrointestinal tract by C. difficile spores and vegetative cells, subsequent production of toxin and onset of clinical signs. Despite fulfilling Koch’s postulates for C. difficile in foals, practical limitations have also limited further use of this model. In a study by Arnon et al.Citation44 infant rhesus monkeys were given C. difficile toxins A or B to investigate whether C. difficile causes a systemic illness, and sometimes death in infants, similar to that caused by Clostridium botulinum toxin. The animals showed no abnormalities for several hours but then developed lethargy, hypotonia, hypothermia, with subsequent breathing cessation. Post-mortem findings were insufficient to explain the cause of death, and no further C. difficile studies in monkeys have been reported.
Zebrafish embryos have been described as suitable models for investigation of damage to organs by C. difficile toxins. Zebrafish possess many of the major organs present in humans, and due to the transparency of the embryo, organ damage can easily be visualized and followed up by light microscopy (unlike in higher order animals). A study by Hamm et al.Citation46 found that the toxin B functioned as a potent cardiotoxin. These findings could be considered in the context of human disease, as patients with severe C. difficile may experience multi-organ failure, including impaired cardiac function.
Non-Animal Models
In vitro models of C. difficile infection
In vitro models of C. difficile have been in use since the late 1970s,Citation137 and have evolved into a practicable alternative to animal models in many respects. They offer the advantages of a greater control, greater numbers of replicates (in some cases), easier manipulation and elimination of the ethical issues surrounding animal models. In vitro models circumvent some of the practical and ethical issues inherent in animal models and also allow a high degree of experimental control. Investigations using in vitro models introduced the concept of “colonization resistance,” evaluated the role of antibiotics in C. difficile development, explored population dynamics and most recently have been useful in the evaluation of C. difficile treatments ().Citation31,Citation32,Citation94,Citation100,Citation123,Citation137,Citation138 However, the interaction of host-related factors such as secretory or immunological events in the disease process cannot be represented.
Table 3. Comparison of hamster experiments performed in testing new potential treatment
Fecal Emulsions
Borriello and Barclay devised an in vitro model to study C. difficile behavior,Citation31 following the success of fecal enemas in treatment of recurrent C. difficile, and the antagonism of C. difficile growth by fecal emulsions from healthy adults.Citation31,Citation139-Citation141 Borriello and Barclay investigated C. difficile growth in fecal emulsions from healthy subjects, patients receiving antimicrobial treatment but without diarrhea, patients not receiving antimicrobial treatment but with diarrhea, and patients with AAD and with proven C. difficile. C. difficile growth and cytotoxin production occurred in fecal emulsions from C. difficile patients, but less so in fecal emulsions derived from other patient groups (infants, children and geriatric patients); there was no growth in fecal emulsions from healthy individuals. This inhibition was removed from fecal emulsions by heating or filter sterilization. They attributed this to viable components within the fecal emulsion, known as “colonization resistance.” underlining the possible involvement of the human gut microbiota in preventing C. difficile.
The credibility of this model was scrutinized by comparing its predictive value against that of the hamster model.Citation77,Citation142 In these experiments, hamsters were treated with various antibiotics, and then challenged with C. difficile. A parallel set of antibiotic-treated hamsters were killed and their cecal contents used in the in vitro colonization model. There was a close correlation between in vivo and in vitro results. All the antibiotics tested (clindamycin, ampicillin, flucloxacillin and cefuroxime) rendered hamsters susceptible to small doses of C. difficile. However, the window of susceptibility varied greatly between antibiotics and was not necessarily dependant on the presence of detectable antibiotic. Larson and Welch investigated C. difficile toxin production in cecal suspensions from untreated and clindamycin-treated hamsters. They demonstrated high level toxin production in cecal contents of clindamycin-treated hamsters in vitro, but not in untreated hamster cecal contents. Toxin production was not seen in a 1:1 mixture of treated and untreated cecal contents.Citation32 Following reports of C. difficile due to an emerging C. difficile strain showing increased fluoroquinolone resistance, Adams et al. used the cecal contents of a female CF-1 mouse model to examine epidemic and non epidemic C. difficile strain growth and toxin in response to fluoroquinolone exposure.Citation143 The authors based the model on the earlier fecal emulsion model described by Borriello et al.Citation31 Levofloxacin, moxifloxacin, gatifloxacin, ciprofloxacin, ceftriaxone and clindamycin were administered subcutaneously in doses equivalent (in mg/g body weight) to that given to humans over a 24 h period. Cecal contents were harvested after animal sacrifice and challenged with 104 cfu/mL C. difficile prepared from an overnight culture. They reported that emergence of epidemic fluoroquinolone resistant C. difficile strains may be promoted by fluoroquinolones in this model. Pultz and DonskeyCitation144 showed that clindamycin, piperacillin-tazobactam and ceftriaxone promoted growth and toxin production by C. difficile strains in mouse cecal contents, while levofloxacin, cefepime and aztreonam did not. They were able to demonstrate detectable concentrations of the former three antimicrobials 3 d post-treatment, while the latter were undetectable by this time. These observations only partly support the colonization resistance theory in humans; for example, piperacillin-tazobactam (in contrast to clindamycin and ceftriaxone) has been shown to have a lower risk of C. difficile in humans,Citation145 despite causing significant disruption to fecal flora.Citation146 A similar study reported that tigecycline did not promote toxin production or colonization of mouse cecal contents, alone or in combination with clindamycin.Citation147 This is in line with previous results from an in vitro gut model reporting a lack of germination and toxin production following tigecycline dosing,Citation148 and other clinical evidence of a low C. difficile risk.Citation149 This group also used the same model to show that cecal contents from mice treated with parenteral ampicillin or piperacillin developed C. difficile overgrowth and toxin production following challenge with the organism, in contrast to cecal contents of mice given parenteral ampicillin or pipercillin with oral tazobactam-inactivated β-lactamase. This treatment strategy was relatively sparing of gut flora, but the authors noted that the reality of polypharmacy in many patients could diminish the efficacy of this approach in humans.Citation150
Batch culture systems have, to some extent, been replaced by continuous culture models which are more gut-reflective. However, Meader et al. recently used a 48 h batch fermentation model in which to evaluate the effects of bacteriophage treatment upon C. difficile.Citation151 The authors used fresh fecal slurries, diluted in growth media and inoculated with an overnight culture of C. difficile. A C. difficile growth control was compared with a remedial treatment (phage introduced 6 h into the experiment and also at 24 and 36 h) and a prophylactic treatment (phage introduced at 0, 6, 24 and 36 h). The authors reported a significant decrease in mean C. difficile total counts (p = < 0.0001); however, it should be noted that in real terms this difference was, in fact less than 1 log cfu/mL. Prophylactic phage administration resulted in a more marked decrease in recoverable C. difficile 151.
Continuous Culture Models
Fecal and cecal suspension/emulsion models are batch culture systems, whereas conditions within the gut are more analogous to continuous culture systems.Citation50 Batch culture bacterial growth dynamics differ significantly to those found in continuous culture. Simple studies by several investigators into the effects of antimicrobials upon C. difficile toxin production in batch (broth) culture have produced conflicting resultsCitation152-Citation156 and therefore researchers turned increasingly to more complex continuous culture systems to more accurately represent the conditions within the gut. Although Borriello and Barclay described their in vitro test tube fecal emulsion model of C. difficile in the mid 1980s, researchers were, in fact, already examining the behavior of C. difficile under continuous culture conditions as early as 1979.Citation137 Continuous culture systems not only allow the study of the organism in a more reflective environment but also enable controlled manipulation and monitoring of that environment over considerably longer time periods than in batch culture experiments. They have therefore been used to evaluate several possible factors in C. difficile development, including environmental stresses, antimicrobial agents and commensal microflora competition 32,94,100,122,123,137,157–165. Onderdonk et al. examined the effects of environmental stress on C. difficile toxin levels in continuous culture.Citation137 They showed that changes in Eh and temperature caused increased levels of C. difficile toxin during continuous culture, and also demonstrated increased toxin in response to sub-inhibitory concentrations of vancomycin or penicillin. Onderdonk et al. suggested that release of toxin by C. difficile was affected by changes in environmental conditions (temperature, Eh or sub-inhibitory antibiotics), and indicated the potential contribution of continuous culture systems to the field of study.
Hamster model studies had indicated that a stable, normal gut flora forms a natural barrier to C. difficile infection.Citation31,Citation109,Citation141 The few continuous culture studies of C. difficile therefore focused on further attempts to elucidate those components of the fecal/cecal flora responsible for colonization resistance.Citation122,Citation123,Citation166 Wilson et al. reported that hamster flora grown in continuous culture or in germfree mice suppressed C. difficile growth in germfree mice. Moreover, they also showed the ability of components of hamster cecal flora to suppress C. difficile in both murine and continuous flow culture experiments.Citation122 A further study by this group investigated the role of nutrient competition in C. difficile using the continuous culture model, and found that C. difficile growth was slowed in the absence of glucose, N-acetylglucosamine or N-acetylneuraminic acid. The authors suggested that competition for nutrients by the colonic microflora could suppress C. difficile growth. However, the study did not encompass any characterization of fecal flora components, and was performed using hamster rather than human-derived floras.Citation123 These issues were addressed to a certain extent by Yamamoto-Osaki et al.Citation165 who expanded on earlier workCitation122 that examined the effect of infant fecal flora on C. difficile growth. They compared C. difficile positive and negative feces for their ability to inhibit C. difficile growth and noted that C. difficile counts decreased in the presence of flora from C. difficile negative feces. Contrastingly, when C. difficile was re-introduced to flora from C. difficile positive feces, counts of the organism increased. The authors also suggested that inhibition of growth of C. difficile may be due to competition for amino acids by the normal flora. Amino acid analysis of the continuous flow cultures with intestinal strains (as opposed to whole microfloras) showed depletion of several amino acids, and replacement of these elicited C. difficile growth. However, it should also be noted that this system was not pH controlled and experienced considerable decreases in pH which were also associated with inhibition of C. difficile growth. In common with later researchers examining the diversity of fecal flora among human volunteersCitation166, they noted a greater diversity of bacterial species in C. difficile negative vs. positive feces.
Triple Stage Human Gut Model
A more recent series of studies has used a triple-stage chemostat model of the human gut to investigate the relationship between antibiotics and C. difficile in a series of experiments 94,100,157–164. The model consists of three anaerobically-maintained and pH-controlled vessels in a weir cascade series and top fed with growth media at a specific flow rate (). The system was first described by MacFarlane et al. in 1998 and was designed to reproduce the spatial, temporal, nutritional and physicochemical characteristics of the proximal to distal bowel. The model was validated against intestinal contents of sudden death victims.Citation167 Gut bacterial populations are introduced via a slurry comprising pooled feces from healthy volunteers, which are allowed to equilibrate over a 2 week period, in common with the earlier continuous culture systems of Wilson and Perini 122,123. This study series includes experiments running for 6–8 weeks, with daily monitoring of C. difficile vegetative cells, spores, toxin and gut flora components in all vessels, as well as determination of antimicrobial concentrations. Two main experimental designs have been used, one for evaluating potential predisposition to C. difficile, and the other to assess C. difficile treatment efficacy ().
Figure 1. Schematic representation of triple stage gut model experiments. (A) Approach to assessing potential predisposition to C. difficile. (B) Approach to assess C. difficile treatment efficacy
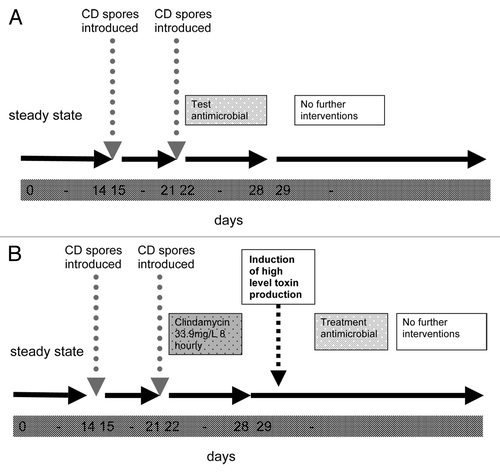
Previous studies had only examined single doses of antimicrobials and at concentrations derived from the MIC relative to test C. difficile strains. This is not necessarily reflective of the environment surrounding C. difficile in the gut. Contrastingly, in the triple-stage gut model experiments, antimicrobials are instilled into the model at fecal or biliary levels and according to a clinically reflective dosing regimen. The gut model has been used to examine antimicrobials for their propensity to induce C. difficileCitation146,Citation148,Citation157,Citation161 their efficacy as C. difficile treatments,Citation94,Citation100,Citation138,Citation158,Citation159,Citation162,Citation163 to examine emergence of resistance,Citation164 and relative C. difficile strain fitness.Citation160,Citation162
C. difficile Induction Studies in the Gut Model
Control experiment data showed that C. difficile spores remain in their quiescent state in the absence of antimicrobial pressure. Studies examining the propensity of antimicrobials associated with clinical C. difficile (cefotaxime, clindamycin, fluoroquinolones) demonstrated that drug exposure was followed by sustained C. difficile germination and high level toxin production.Citation94,Citation138,Citation158,Citation162,Citation164 Conversely, antimicrobial exposure to piperacillin-tazobactam, tigecyline or mecillinam did not precipitate C. difficile germination or high level toxin production;Citation146,Citation148,Citation157 these results are consistent with clinical data showing a lower risk of C. difficile with these antibiotics. Sampling for antimicrobial levels within an in vitro system is much simpler and can be performed more frequently than in animal models. Antimicrobial bioassays were therefore performed for many of these studies.Citation138,Citation146,Citation148,Citation157-Citation159,Citation162-Citation164 They showed that C. difficile remained in its quiescent spore form until antimicrobial concentrations fell below the MIC of the organism, germinating and producing toxin thereafter. This interesting observation extends similar results in both hamstersCitation78,Citation82 and in vitro continuous cultureCitation137 that suggest antimicrobial stimulation of C. difficile.
C. difficile Treatment Studies in the Gut Model
This model has also been used for the evaluation of existing and novel treatments for C. difficile. In these experiments clindamycin was used to elicit C. difficile germination and high level toxin production prior to treatment with the test compound, dosed according to a clinically reflective regimen. Metronidazole and vancomycin were both evaluated against epidemic C. difficile strains.Citation158,Citation162 Comparison with the results of a previous gut model study showed that vancomycin was more effective in reducing C. difficile PCR ribotype 027 numbers and cytotoxin titers than metronidazole.Citation158 Evaluation of metronidazole treatment of simulated C. difficile demonstrated unexpectedly low drug concentrations in distal vessels of the gut model; it was postulated that low drug concentrations may have been caused by fecal inactivation of metronidazole, possibly mediated by enterococci.Citation162
Novel treatments for C. difficile which have been evaluated in the gut model include ramoplanin, oritavancin, linezolid, tolevamer and other investigational compounds.Citation94,Citation100,Citation138,Citation159,Citation163 In the case of ramoplanin, the results of the in vitro gut model experiments were concordant with in vivo hamster experiments performed as part of the evaluation. Both ramoplanin and vancomycin treatment reduced cytotoxin production in the gut C. difficile model and resolved symptoms in the hamster model. Notably, however, vancomycin was associated with greater persistence of C. difficile spores in both the hamster and gut models. C. difficile spores were recovered significantly more often from the cecal contents of vancomyin-treated (n = 19/23) compared with ramoplanin-treated (n = 6/23) hamsters (p < 0.05).Citation94 The results indicate that ramoplanin is more effective than vancomycin at inhibiting spores and thus preventing spore recrudescence. The novel lipoglycopeptide oritavancin was also effective in treating clindamycin-induced C. difficile in a human gut model; the comparator, vancomycin, was associated with C. difficile recurrence (repeat germination and toxin production). Oritavancin demonstrated greater inhibition of spores, and again may prevent recrudescence of C. difficile spores.Citation100 Data from a recent study of oritavancin, vancomycin and clindamycin in hamsters also support these observations.Citation83
Tolevamer is a novel non-antimicrobial styrene derivative that binds to and neutralizes the toxic effects C. difficile toxins A and B in vitro. Early in vitro, hamster model and phase II clinical trial data were encouraging. However, evaluation of tolevamer as a treatment for simulated C. difficile in the gut model showed that neither the duration nor magnitude of cytotoxin activity was reduced by the agent.Citation138 These data supported Phase III clinical trial results in which tolevamer failed to meet its primary endpoint of non-inferiority to vancomycin.Citation91 An evaluation of linezolid for treatment of simulated C. difficile in the gut model concluded that linezolid may reduce toxin levels, but not viable counts and was associated with spore recrudescence in one model.Citation159 Examination of the efficacy of the novel antibiotic NVB302 in treatment of simulated C. difficile in the gut model demonstrated non-inferiority of NVB302 to vancomycin in terms of reducing cytotoxin levels and total C. difficile, with no evidence of spore recrudescence.Citation163
Rea et al. recently evaluated the bacteriocin thuricin C for its effects on C. difficile using a single vessel distal colon model. Their approach had several drawbacks in contrast to that of the triple stage model antimicrobial evaluations. Unlike previous continuous culture C. difficile models, there was no steady-state period during which fecal bacterial could equilibrate. Additionally, the short study duration (24 h) meant that it was not possible to draw clear conclusions concerning antimicrobial metabolism, effects on gut flora components or the potential for recurrence.Citation168
Indigenous Gut Microflora Changes
The data obtained from gut model experiments also allow the comparison of gut flora changes in response to antimicrobials. Neither gut flora components nor antimicrobial effects upon them had been extensively evaluated in previous in vitro models, despite the establishment of the colonization resistance theory. Interestingly, gross depletion of gut flora components (total facultative aerobes, total anaerobes, lactose fermenters, enterococci, clostridia, bacteroides spp, bifidobacteria and lactobacilli), for example as seen with piperacillin-tazobactam and tigecycline, did not lead to C. difficile germination.Citation146,Citation148 In contrast, cefotaxime instillation produced modest decreases in bifidobacteria and bacteroides populations but was nevertheless accompanied by C. difficile germination and high level toxin production.Citation161 Similarly, although clindamycin had a markedly deleterious effect upon gut flora, numbers invariably re-established to pre-drug levels before C. difficile germination and toxin production occurred.Citation94 This suggests that either those components responsible for colonization resistance were not assayed for, or that the role of the gut flora in moderating C. difficile is more complicated than previously thought.
C. difficile Population Dynamics and Resistance Development
C. difficile PCR ribotype 027 remained in a vegetative form and producing toxin for substantially longer than C. difficile PCR ribotype 001 in human gut model experiments. Such data are consistent with the presence of an 18 bp deletion in the putative negative regulator for toxin production in C. difficile PCR ribotype 027 and may explain the increased severity of symptoms associated with this C. difficile strain.Citation162 These observations part-contradicted and extended those of Warny et al. who reported higher peak median toxin production by NAP1/BI strains in vitro than median toxin production of a group of 12 other PFGE types.Citation169 Gut model continuous culture techniques showed that there was neither an increase in rate of toxin production by C. difficile PCR ribotype 027, nor in the titer of toxin present in the gut model at any given time; instead the duration of toxin production by C. difficile 027 was markedly longer than that of C. difficile PCR ribotype 001. These discrepancies highlight the drawbacks of using simple batch culture techniques to study C. difficile behavior.
Ciprofloxacin, levofloxacin and moxifloxacin all induced C. difficile germination and toxin production in the gut model.Citation164 Interestingly, fluoroquinolone-resistant isolates were detected during the experiments, implying the potential for in vivo selection. Moxifloxacin was also associated with early toxin production (pre-detectable germination and proliferation) in C. difficile PCR ribotype 027, suggesting the existence of strain-specific differences in response to fluoroquinolones. This may be particularly relevant in the light of controversies regarding the relative risk of C. difficile associated with fluoroquinolones. Reports of metronidazole failure in C. difficile cases,Citation170,Citation171 and the emergence of reduced susceptibility among C. difficile epidemic ribotypesCitation172,Citation173 prompted an investigation into the relative fitness of strains with differing metronidazole and clindamycin susceptibilities in the gut model.Citation160 Neither resistance to clindamycin nor reduced susceptibility to metronidazole elicited a fitness cost when no selective advantage for either strain was present, and there was no antagonism observed between strains. These studies highlight the value of using an in vitro gut model to evaluate potential resistance development and heterogeneous C. difficile populations.
Discussion
An animal model of C. difficile should ideally be standardized, produce reproducible results, and as far as possible resemble the etiology, pathophysiology and disease course seen in humans. The hamster model has been widely recognized as one of the most useful and predictive in vivo models of C. difficile. Hamster model studies initially focused on elucidating the pathogenesis of C. difficile and more recently exploring therapeutic and preventative strategies. Despite its recognition as a useful animal model, the hamster model is not fully standardized, has distinct pathophysiological differences compared with human disease, is problematic to establish, prone to contamination and suffers from reproducibility issues. As a result of these issues and ethical considerations surrounding animal models in general, the hamster model is only available in a few centers worldwide.
While hamsters are the most extensively used C. difficile animal models, particularly for the testing of novel therapeutic agents, there is a great deal of variation in the experimental methods employed. Such issues affect the reproducibility and interpretation of results. Many of the problems associated with hamster model experiments are also applicable to other small animal models. A key issue, exacerbated in hamsters given their exquisite susceptibility to C. difficile after antibiotic priming, is the potential for cross-contamination. This can occur via the local environment, food, water or by the animals themselves. In addition coprophagia may lead to further C. difficile exposure, compromising experimental validity. Animal environments have differed considerably between studies, with some hamsters housed individually and some in groups of up to five per cage.Citation22 In some cases HEPA filtered air, sterile food and water were used,Citation94 while in other reports these factors are not detailed. Douce and Goulding recommend individual housing in sterile cages with sterile food, water and bedding and transfer to a newly sterile environment every 24 h post-infection to minimize additional C. difficile exposure.Citation174
While vancomycin was used to induce C. difficile in early hamster model studies, clindamycin has become the inducing agent of choice. There has however been little standardization of either the dose or route of administration of either agent, both of which may potentially affect the timing and magnitude of C. difficile germination and toxin production. The dosing of clindamycin has varied 100-fold (1–100 mg/kg), and similarly in experiments to evaluate C. difficile treatments, marked differences in (apparently effective) dosages of the comparator antibiotic, which is usually vancomycin, also make inter-study comparison difficult. Although 50 mg/kg vancomycin administered orogastrically was used in the majority of studies, doses have varied between 0.05 mg/dayCitation22 to 200 mg/kg.Citation82 Vancomycin treatment regimens have ranged from single dose therapyCitation82 to daily administration over 5 d.Citation93,Citation94
Other potentially important confounding factors include qualitative and quantitative variations in C. difficile inocula. Most early studies did not deliberately expose hamsters to controlled amounts of C. difficile, instead relying on the exquisite susceptibility of the animal to C. difficile following antibiotic priming. Several studies did not determine relative populations of spore and vegetative cells; while others administered only vegetative populations, which may not survive the acid barrier of the stomach. C. difficile is thought to be ingested primarily as spores, and this has been considered in more recent animal model work, with researchers using controlled inocula of C. difficile spores.Citation47,Citation49,Citation114 Choice of C. difficile strain may also influence experimental outcome, since strains differ widely in their “virulence” properties, including toxigenicity, antimicrobial resistance, germination and sporulation capacities and colonization efficacy. Some studies stated whether known toxigenic and hypervirulent strains were administered,Citation39,Citation49 while others provided no information at all. Phenotypic properties can also affect the timing of C. difficile induction by clindamycin; clindamycin resistance in C. difficile strains has been associated with delayed disease onset in hamsters.Citation51 Such issues may be amplified according to the choice of model endpoint and duration of study. In hamster models the endpoint has invariably been animal death (or euthanasia), whereas some studies have included histological and/or microbiological analysis of gut mucosa and contents, while others did not. Duration of study is particularly relevant when evaluating treatment efficacy, and especially the likelihood of C. difficile recurrence; the hamster model has generally not been useful for measuring C. difficile recurrence, given the natural history of C. difficile in these animals.
The use of more complex in vitro models to examine the behavior of an organism, necessarily introduces more variables, and therefore it is difficult to compare experiments directly. Likewise, these systems have often evolved according to the advances in knowledge and technology made several decades. Such development may overcome many of the problems first encountered, but later models have often become more complex and expensive in the process. In vitro disease models also cannot mimic secretory or immunological events, which are important aspects of C. difficile disease. In vitro models of C. difficile have progressed from simple test-tube fecal emulsions to complex triple-stage chemostat models (). Batch fermentation and test-tube models carry the advantages of short experimental duration and high numbers of replicates. However, they poorly simulate the behavior of C. difficile or the gut microflora in vivo. Bacterial growth dynamics are significantly different under continuous vs. batch culture, and the human gut environment is more akin to the former. Moreover, continuous culture affords the researcher further flexibility and a longer experimental time-course, allowing a profile of C. difficile and gut microflora behaviors and responses to environmental changes to be determined. A number of authors have compared hamster and either gut modelCitation94 or alternative continuous flow culture systemsCitation122 and found results to be generally concordant.
Table 4. Comparison of in vitro models of C. difficile infection
With the exception of studies in the triple stage gut model, most feces based C. difficile experiments have been performed as stand-alone investigations. This inevitably means wide variations in total operating volume, duration of experiment, sources of fecal inoculum, fecal dilution factor and, importantly, the size and nature of the C. difficile inoculum. In addition, until later studies, the effects of antimicrobials on C. difficile was investigated using feces from antibiotic-treated patients or hamsters, and so were prone to variation in antimicrobial concentrations and thus difficult to compare. Continuous culture models have also differed in their volumes and retention times, all of which may influence the environment surrounding C. difficile and potentially its behavior. The series of experiments performed in the triple stage gut model have been well-standardized in terms of equipment, experimental protocol and microbiological and antimicrobial monitoring. The drawbacks of this approach are the labor-intensive nature of the model, additional expense and longer experimental duration.
Conclusions
It is clear that the choice of model may substantially affect the results obtained in C. difficile research. Hamster models have been considered the definitive option for C. difficile testing, especially for the evaluation of novel therapeutic agents. Experimental refinements in recent years have addressed some of the associated ethical and practical issues associated with animal testing, but standardization of methods remains a key issue. Recent advances in mouse models offer new opportunities and possibly improved reproducibility. However, the relevance of results obtained via animal models to human C. difficile remains a crucial issue. The development of standardized in vitro models additionally allows data comparison according to a range of variables, which in turn can provide confidence about the robustness of results. Appropriate model choice and standardization of experimental procedures are essential to generate clear and reproducible data. Animal and in vitro models of C. difficile both have pros and cons, and comprehensive study programs should ideally incorporate both approaches, taking care to understand key concordant and discrepant results. Such approaches can better inform the design and interpretation of clinical studies.
Future Directions
There appears to have been a reduction in use of animal models in C. difficile research over the past 30 years probably as a result of the ethical and practical drawbacks associated with animal testing. Crucial inter-species differences with respect to susceptibility to C. difficile and disease severity mean that extrapolation of results obtained from one animal model to another, and particularly to humans, may not be applicable. Such inter species variability is likely influenced by multiple factors, including presence of toxin receptors, and gut anatomy and flora.Citation175,Citation176 There has been only very limited use of larger laboratory animals (e.g., foals, piglets, monkeys) for the study of C. difficile, and hamsters and mice models remain the most practicable options. The use of germ-free, gnotobiotic mice and axenic mice indicates that these may be more reliable models for future studies.Citation36 The potential availability of specific genetic mouse breeds is both an advantage in terms of flexibility but also a disadvantage considering the desirables of standardization and reproducibility.
References
- Hall IC, O'Toole E. Intestinal flora in new-born infants; with a description of a new pathogenic anaerobe, Bacillus difficilis. Am J Dis Child 1935; 49:390 - 402
- Bartlett JG, Moon N, Chang TW, Taylor N, Onderdonk AB. Role of Clostridium difficile in antibiotic-associated pseudomembranous colitis. Gastroenterology 1978; 75:778 - 82; PMID: 700321
- George WL, Sutter VL, Goldstein EJ, Ludwig SL, Finegold SM. Aetiology of antimicrobial-agent-associated colitis. Lancet 1978; 1:802 - 3; http://dx.doi.org/10.1016/S0140-6736(78)93001-5; PMID: 85818
- Larson HE, Price AB, Honour P, Borriello SP. Clostridium difficile and the aetiology of pseudomembranous colitis. Lancet 1978; 1:1063 - 6; http://dx.doi.org/10.1016/S0140-6736(78)90912-1; PMID: 77366
- A report by the joint Department of Health and Public Health Laboratory Service working group. The Prevention and Management of Clostridium difficile infection. 1994.
- Rupnik M, Wilcox MH, Gerding DN. Clostridium difficile infection: new developments in epidemiology and pathogenesis. Nat Rev Microbiol 2009; 7:526 - 36; http://dx.doi.org/10.1038/nrmicro2164; PMID: 19528959
- Fashner J, Garcia M, Ribble L, Crowell K. Clinical inquiry: what risk factors contribute to C. difficile diarrhrea?. J Fam Pract 2011; 60:544 - 7
- van Nispen tot Pannerden CM, Verban A, Kuipers EJ. Recurrent CDI: What are the treatment options?. Drugs 2011; 71:853 - 68; http://dx.doi.org/10.2165/11591230-000000000-00000
- Wilcox MH, Fawley WN, Settle CD, Davidson A. Recurrence of symptoms in Clostridium difficile infection--relapse or reinfection?. J Hosp Infect 1998; 38:93 - 100; http://dx.doi.org/10.1016/S0195-6701(98)90062-7; PMID: 9522287
- Gerding DN, Muto CA, Owens RC Jr.. Treatment of Clostridium difficile infection. Clin Infect Dis 2008; 46:Suppl 1 S32 - 42; http://dx.doi.org/10.1086/521860; PMID: 18177219
- Teasley DG, Gerding DN, Olson MM, Peterson LR, Gebhard RL, Schwartz MJ, et al. Prospective randomised trial of metronidazole versus vancomycin for Clostridium-difficile-associated diarrhoea and colitis. Lancet 1983; 2:1043 - 6; http://dx.doi.org/10.1016/S0140-6736(83)91036-X; PMID: 6138597
- Wenisch C, Parschalk B, Hasenhündl M, Hirschl AM, Graninger W. Comparison of vancomycin, teicoplanin, metronidazole, and fusidic acid for the treatment of Clostridium difficile-associated diarrhea. Clin Infect Dis 1996; 22:813 - 8; http://dx.doi.org/10.1093/clinids/22.5.813; PMID: 8722937
- Wilcox MH, Howe R. Diarrhoea caused by Clostridium difficile: response time for treatment with metronidazole and vancomycin. J Antimicrob Chemother 1995; 36:673 - 9; http://dx.doi.org/10.1093/jac/36.4.673; PMID: 8591942
- Al-Nassir WN, Sethi AK, Nerandzic MM, Bobulsky GS, Jump RL, Donskey CJ. Comparison of clinical and microbiological response to treatment of Clostridium difficile-associated disease with metronidazole and vancomycin. Clin Infect Dis 2008; 47:56 - 62; http://dx.doi.org/10.1086/588293; PMID: 18491964
- Baines SD, O’Connor R, Freeman J, Fawley WN, Harmanus C, Mastrantonio P, et al. Emergence of reduced susceptibility to metronidazole in Clostridium difficile. J Antimicrob Chemother 2008; 62:1046 - 52; http://dx.doi.org/10.1093/jac/dkn313; PMID: 18693234
- Chang TW, Bartlett JG, Gorbach SL, Onderdonk AB. Clindamycin-induced enterocolitis in hamsters as a model of pseudomembranous colitis in patients. Infect Immun 1978; 20:526 - 9; PMID: 669810
- Toshniwal R, Silva J Jr., Fekety R, Kim KH. Studies on the epidemiology of colitis due to Clostridium difficile in hamsters. J Infect Dis 1981; 143:51 - 4; http://dx.doi.org/10.1093/infdis/143.1.51; PMID: 7217712
- Toshniwal R, Fekety R, Silva J Jr.. Etiology of tetracycline-associated pseudomembranous colitis in hamsters. Antimicrob Agents Chemother 1979; 16:167 - 70; PMID: 485127
- Fekety R, Silva J, Browne RA, Rifkin GD, Ebright JR. Clindamycin-induced colitis. Am J Clin Nutr 1979; 32:244 - 50; PMID: 760500
- Rifkin GD, Silva J Jr., Fekety R. Gastrointestinal and systemic toxicity of fecal extracts from hamsters with clindamycin-induced colitis. Gastroenterology 1978; 74:52 - 7; PMID: 336452
- Borriello SP, Ketley JM, Mitchell TJ, Barclay FE, Welch AR, Price AB, et al. Clostridium difficile--a spectrum of virulence and analysis of putative virulence determinants in the hamster model of antibiotic-associated colitis. J Med Microbiol 1987; 24:53 - 64; http://dx.doi.org/10.1099/00222615-24-1-53; PMID: 3612744
- Dong MY, Chang TW, Gorbach SL. Treatment of Clostridium difficile colitis in hamsters with a lipopeptide antibiotic, LY146032. Antimicrob Agents Chemother 1987; 31:1135 - 6; PMID: 2821892
- Elmer GW, Corthier G. Modulation of Clostridium difficile induced mortality as a function of the dose and the viability of the Saccharomyces boulardii used as a preventative agent in gnotobiotic mice. Can J Microbiol 1991; 37:315 - 7; http://dx.doi.org/10.1139/m91-049; PMID: 1913342
- Frankel WL, Choi DM, Zhang W, Roth JA, Don SH, Afonso JJ, et al. Soy fiber delays disease onset and prolongs survival in experimental Clostridium difficile ileocecitis. JPEN J Parenter Enteral Nutr 1994; 18:55 - 61; http://dx.doi.org/10.1177/014860719401800155; PMID: 8164305
- McVay CS, Rolfe RD. In vitro and in vivo activities of nitazoxanide against Clostridium difficile. Antimicrob Agents Chemother 2000; 44:2254 - 8; http://dx.doi.org/10.1128/AAC.44.9.2254-2258.2000; PMID: 10952564
- Bartlett JG, Chang TW, Moon N, Onderdonk AB. Antibiotic-induced lethal enterocolitis in hamsters: studies with eleven agents and evidence to support the pathogenic role of toxin-producing Clostridia. Am J Vet Res 1978; 39:1525 - 30; PMID: 697162
- Bartlett JG, Onderdonk AB, Cisneros RL, Kasper DL. Clindamycin-associated colitis due to a toxin-producing species of Clostridium in hamsters. J Infect Dis 1977; 136:701 - 5; http://dx.doi.org/10.1093/infdis/136.5.701; PMID: 915343
- Kink JA, Williams JA. Antibodies to recombinant Clostridium difficile toxins A and B are an effective treatment and prevent relapse of C. difficile-associated disease in a hamster model of infection. Infect Immun 1998; 66:2018 - 25; PMID: 9573084
- Lyras D, O’Connor JR, Howarth PM, Sambol SP, Carter GP, Phumoonna T, et al. Toxin B is essential for virulence of Clostridium difficile. Nature 2009; 458:1176 - 9; http://dx.doi.org/10.1038/nature07822; PMID: 19252482
- Alcantara C, Stenson WF, Steiner TS, Guerrant RL. Role of inducible cyclooxygenase and prostaglandins in Clostridium difficile toxin A-induced secretion and inflammation in an animal model. J Infect Dis 2001; 184:648 - 52; http://dx.doi.org/10.1086/322799; PMID: 11474431
- Borriello SP, Barclay FE. An in-vitro model of colonisation resistance to Clostridium difficile infection. J Med Microbiol 1986; 21:299 - 309; http://dx.doi.org/10.1099/00222615-21-4-299; PMID: 3723582
- Larson HE, Welch A. In-vitro and in-vivo characterisation of resistance to colonisation with Clostridium difficile. J Med Microbiol 1993; 38:103 - 8; http://dx.doi.org/10.1099/00222615-38-2-103; PMID: 8429534
- Blankenship-Paris TL, Walton BJ, Hayes YO, Chang J. Clostridium difficile infection in hamsters fed an atherogenic diet. Vet Pathol 1995; 32:269 - 73; http://dx.doi.org/10.1177/030098589503200308; PMID: 7604493
- Butel MJ, Roland N, Hibert A, Popot F, Favre A, Tessedre AC, et al. Clostridial pathogenicity in experimental necrotising enterocolitis in gnotobiotic quails and protective role of bifidobacteria. J Med Microbiol 1998; 47:391 - 9; http://dx.doi.org/10.1099/00222615-47-5-391; PMID: 9879939
- Castagliuolo I, Kelly CP, Qiu BS, Nikulasson ST, LaMont JT, Pothoulakis C. IL-11 inhibits Clostridium difficile toxin A enterotoxicity in rat ileum. Am J Physiol 1997; 273:G333 - 41; PMID: 9277411
- Chen X, Katchar K, Goldsmith JD, Nanthakumar N, Cheknis A, Gerding DN, et al. A mouse model of Clostridium difficile-associated disease. Gastroenterology 2008; 135:1984 - 92; http://dx.doi.org/10.1053/j.gastro.2008.09.002; PMID: 18848941
- Dabard J, Dubos F, Martinet L, Ducluzeau R. Experimental reproduction of neonatal diarrhea in young gnotobiotic hares simultaneously associated with Clostridium difficile and other Clostridium strains. Infect Immun 1979; 24:7 - 11; PMID: 222683
- Elmer GW, McFarland LV. Suppression by Saccharomyces boulardii of toxigenic Clostridium difficile overgrowth after vancomycin treatment in hamsters. Antimicrob Agents Chemother 1987; 31:129 - 31; PMID: 3566236
- Kokkotou E, Moss AC, Michos A, Espinoza D, Cloud JW, Mustafa N, et al. Comparative efficacies of rifaximin and vancomycin for treatment of Clostridium difficile-associated diarrhea and prevention of disease recurrence in hamsters. Antimicrob Agents Chemother 2008; 52:1121 - 6; http://dx.doi.org/10.1128/AAC.01143-07; PMID: 18195066
- Xia Y, Hu HZ, Liu S, Pothoulakis C, Wood JD. Clostridium difficile toxin A excites enteric neurones and suppresses sympathetic neurotransmission in the guinea pig. Gut 2000; 46:481 - 6; http://dx.doi.org/10.1136/gut.46.4.481; PMID: 10716676
- Blankenship-Paris TL, Chang J, Dalldorf FG, Gilligan PH. In vivo and in vitro studies of Clostridium difficile-induced disease in hamsters fed an atherogenic, high-fat diet. Lab Anim Sci 1995; 45:47 - 53; PMID: 7752614
- Muller EL, Pitt HA, George WL. Prairie dog model for antimicrobial agent-induced Clostridium difficile diarrhea. Infect Immun 1987; 55:198 - 200; PMID: 3793229
- Steele J, Feng H, Parry N, Tzipori S. Piglet models of acute or chronic Clostridium difficile illness. J Infect Dis 2010; 201:428 - 34; http://dx.doi.org/10.1086/649799; PMID: 20039803
- Arnon SS, Mills DC, Day PA, Henrickson RV, Sullivan NM, Wilkins TD. Rapid death of infant rhesus monkeys injected with Clostridium difficile toxins A and B: physiologic and pathologic basis. J Pediatr 1984; 104:34 - 40; http://dx.doi.org/10.1016/S0022-3476(84)80585-5; PMID: 6690674
- Arroyo LG, Weese JS, Staempfli HR. Experimental Clostridium difficile enterocolitis in foals. J Vet Intern Med 2004; 18:734 - 8; http://dx.doi.org/10.1111/j.1939-1676.2004.tb02613.x; PMID: 15515592
- Hamm EE, Voth DE, Ballard JD. Identification of Clostridium difficile toxin B cardiotoxicity using a zebrafish embryo model of intoxication. Proc Natl Acad Sci U S A 2006; 103:14176 - 81; http://dx.doi.org/10.1073/pnas.0604725103; PMID: 16966605
- Sambol SP, Merrigan MM, Tang JK, Johnson S, Gerding DN. Colonization for the prevention of Clostridium difficile disease in hamsters. J Infect Dis 2002; 186:1781 - 9; http://dx.doi.org/10.1086/345676; PMID: 12447764
- Fekety R, Silva J, Toshniwal R, Allo M, Armstrong J, Browne R, et al. Antibiotic-associated colitis: effects of antibiotics on Clostridium difficile and the disease in hamsters. Rev Infect Dis 1979; 1:386 - 97; http://dx.doi.org/10.1093/clinids/1.2.386; PMID: 549190
- Razaq N, Sambol S, Nagaro K, Zukowski W, Cheknis A, Johnson S, et al. Infection of hamsters with historical and epidemic BI types of Clostridium difficile. J Infect Dis 2007; 196:1813 - 9; http://dx.doi.org/10.1086/523106; PMID: 18190262
- Wilson KH, Sheagren JN, Freter R. Population dynamics of ingested Clostridium difficile in the gastrointestinal tract of the Syrian hamster. J Infect Dis 1985; 151:355 - 61; http://dx.doi.org/10.1093/infdis/151.2.355; PMID: 3968453
- Sambol SP, Tang JK, Merrigan MM, Johnson S, Gerding DN. Infection of hamsters with epidemiologically important strains of Clostridium difficile. J Infect Dis 2001; 183:1760 - 6; http://dx.doi.org/10.1086/320736; PMID: 11372028
- Small JD. Fatal enterocolitis in hamsters given lincomycin hydrochloride. Lab Anim Care 1968; 18:411 - 20; PMID: 4233847
- Marrie TJ, Faulkner RS, Badley BW, Hartlen MR, Comeau SA, Miller HR. Pseudomembranous colitis: isolation of two species of cytotoxic clostridia and successful treatment with vancomycin. Can Med Assoc J 1978; 119:1058 - 60; PMID: 743667
- Rolfe RD. Role of volatile fatty acids in colonization resistance to Clostridium difficile. Infect Immun 1984; 45:185 - 91; PMID: 6735467
- Rolfe RD, Iaconis JP. Clostridium difficile colonization of infant hamsters. In: Adams, DA, Hahn H, Opferkuch W (eds.). The Influence of Antibiotics on the Host-Parasite Relationship II. (Springer-Verlag: Berlin, 1985, pp. 220-227.
- Rolfe RD. Binding kinetics of Clostridium difficile toxins A and B to intestinal brush border membranes from infant and adult hamsters. Infect Immun 1991; 59:1223 - 30; PMID: 1900806
- Rolfe RD, Iaconis JP. Intestinal colonization of infant hamsters with Clostridium difficile. Infect Immun 1983; 42:480 - 6; PMID: 6642638
- Viscidi R, Willey S, Bartlett JG. Isolation rates and toxigenic potential of Clostridium difficile isolates from various patient populations. Gastroenterology 1981; 81:5 - 9; PMID: 7239125
- Holdeman LV, Good IJ, Moore WE. Human fecal flora: variation in bacterial composition within individuals and a possible effect of emotional stress. Appl Environ Microbiol 1976; 31:359 - 75; PMID: 938032
- Eglow R, Pothoulakis C, Itzkowitz S, Israel EJ, O’Keane CJ, Gong D, et al. Diminished Clostridium difficile toxin A sensitivity in newborn rabbit ileum is associated with decreased toxin A receptor. J Clin Invest 1992; 90:822 - 9; http://dx.doi.org/10.1172/JCI115957; PMID: 1325998
- Rolfe RD, Song W. Immunoglobulin and non-immunoglobulin components of human milk inhibit Clostridium difficile toxin A-receptor binding. J Med Microbiol 1995; 42:10 - 9; http://dx.doi.org/10.1099/00222615-42-1-10; PMID: 7739018
- Alworth L, Simmons J, Franklin C, Fish R. Clostridial typhlitis associated with topical antibiotic therapy in a Syrian hamster. Lab Anim 2009; 43:304 - 9; http://dx.doi.org/10.1258/la.2008.008072; PMID: 19116288
- Babcock GJ, Broering TJ, Hernandez HJ, Mandell RB, Donahue K, Boatright N, et al. Human monoclonal antibodies directed against toxins A and B prevent Clostridium difficile-induced mortality in hamsters. Infect Immun 2006; 74:6339 - 47; http://dx.doi.org/10.1128/IAI.00982-06; PMID: 16966409
- Carter GP, Rood JI, Lyras D. The role of toxin A and toxin B in Clostridium difficile-associated disease: Past and present perspectives. Gut Microbes 2010; 1:58 - 64; http://dx.doi.org/10.4161/gmic.1.1.10768; PMID: 20664812
- Taylor NS, Thorne GM, Bartlett JG. Comparison of two toxins produced by Clostridium difficile. Infect Immun 1981; 34:1036 - 43; PMID: 7333662
- Just I, Gerhard R. Large clostridial cytotoxins. Rev Physiol Biochem Pharmacol 2004; 152:23 - 47; http://dx.doi.org/10.1007/s10254-004-0033-5; PMID: 15449191
- Voth DE, Ballard JD. Clostridium difficile toxins: mechanism of action and role in disease. Clin Microbiol Rev 2005; 18:247 - 63; http://dx.doi.org/10.1128/CMR.18.2.247-263.2005; PMID: 15831824
- Lyerly DM, Saum KE, MacDonald DK, Wilkins TD. Effects of Clostridium difficile toxins given intragastrically to animals. Infect Immun 1985; 47:349 - 52; PMID: 3917975
- Riegler M, Sedivy R, Pothoulakis C, Hamilton G, Zacherl J, Bischof G, et al. Clostridium difficile toxin B is more potent than toxin A in damaging human colonic epithelium in vitro. J Clin Invest 1995; 95:2004 - 11; http://dx.doi.org/10.1172/JCI117885; PMID: 7738167
- Kuehne SA, Cartman ST, Heap JT, Kelly ML, Cockayne A, Minton NP. The role of toxin A and toxin B in Clostridium difficile infection. Nature 2010; 467:711 - 3; http://dx.doi.org/10.1038/nature09397; PMID: 20844489
- Lowy I, Molrine DC, Leav BA, Blair BM, Baxter R, Gerding DN, et al. Treatment with monoclonal antibodies against Clostridium difficile toxins. N Engl J Med 2010; 362:197 - 205; http://dx.doi.org/10.1056/NEJMoa0907635; PMID: 20089970
- Giannasca PJ, Zhang ZX, Lei WD, Boden JA, Giel MA, Monath TP, et al. Serum antitoxin antibodies mediate systemic and mucosal protection from Clostridium difficile disease in hamsters. Infect Immun 1999; 67:527 - 38; PMID: 9916055
- O’Brien JB, McCabe MS, Athie´-Morales V, McDonald GS, Ni´ Eidhin DB, Kelleher DP. Passive immunisation of hamsters against Clostridium difficile infection using antibodies to surface layer proteins. FEMS Microbiol Lett 2005; 246:199 - 205; http://dx.doi.org/10.1016/j.femsle.2005.04.005; PMID: 15899406
- Ni´ Eidhin DB, O’Brien JB, McCabe MS, Athie´-Morales V, Kelleher DP. Active immunization of hamsters against Clostridium difficile infection using surface-layer protein. FEMS Immunol Med Microbiol 2008; 52:207 - 18; http://dx.doi.org/10.1111/j.1574-695X.2007.00363.x; PMID: 18093141
- Torres JF, Lyerly DM, Hill JE, Monath TP. Evaluation of formalin-inactivated Clostridium difficile vaccines administered by parenteral and mucosal routes of immunization in hamsters. Infect Immun 1995; 63:4619 - 27; PMID: 7591115
- Bartlett JG, Gorbach SL. Pseudomembranous enterocolitis (antibiotic-related colitis). Adv Intern Med 1977; 22:455 - 76; PMID: 320848
- Larson HE, Borriello SP. Quantitative study of antibiotic-induced susceptibility to Clostridium difficile enterocecitis in hamsters. Antimicrob Agents Chemother 1990; 34:1348 - 53; PMID: 2386366
- Ebright JR, Fekety R, Silva J, Wilson KH. Evaluation of eight cephalosporins in hamster colitis model. Antimicrob Agents Chemother 1981; 19:980 - 6; PMID: 6973951
- Silva J, Fekety R, Werk C, Ebright J, Cudmore M, Batts D, et al. Inciting and etiologic agents of colitis. Rev Infect Dis 1984; 6:Suppl 1 S214 - 21; http://dx.doi.org/10.1093/clinids/6.Supplement_1.S214; PMID: 6718935
- Lusk RH, Fekety R, Silva J, Browne RA, Ringler DH, Abrams GD. Clindamycin-induced enterocolitis in hamsters. J Infect Dis 1978; 137:464 - 75; http://dx.doi.org/10.1093/infdis/137.4.464; PMID: 649990
- Onderdonk AB, Bartlett JG. The biological and clinical significance of Clostridium difficile. Crit Rev Clin Lab Sci 1981; 13:161 - 72; http://dx.doi.org/10.3109/10408368109106446; PMID: 7249664
- Wilson KH, Silva J, Fekety FR. Suppression of Clostridium difficile by normal hamster cecal flora and prevention of antibiotic-associated cecitis. Infect Immun 1981; 34:626 - 8; PMID: 7309245
- Marquis M, Higgins M-E. Sok Meng Mong, Lehoux D. Oritavancin pretreatment does not induce Clostridium difficile infection in an experimental hamster model. In Programs and Abstracts of the 50th Interscience Conference on Antimicrobial Agents and Chemotherapy, Boston. 2010. Abstract B-704. American Society for Microbiology, Washington, DC, 2010 p79. 2010.
- Swanson RN, Hardy DJ, Shipkowitz NL, Hanson CW, Ramer NC, Fernandes PB, et al. In vitro and in vivo evaluation of tiacumicins B and C against Clostridium difficile. Antimicrob Agents Chemother 1991; 35:1108 - 11; PMID: 1929250
- Louie TJ, Miller MA, Mullane KM, Weiss K, Lentnek A, Golan Y, et al, OPT-80-003 Clinical Study Group. Fidaxomicin versus vancomycin for Clostridium difficile infection. N Engl J Med 2011; 364:422 - 31; http://dx.doi.org/10.1056/NEJMoa0910812; PMID: 21288078
- Mullane KM, Miller MA, Weiss K, Lentnek A, Golan Y, Sears PS, et al. Efficacy of fidaxomicin versus vancomycin as therapy for Clostridium difficile infection in individuals taking concomitant antibiotics for other concurrent infections. Clin Infect Dis 2011; 53:440 - 7; http://dx.doi.org/10.1093/cid/cir404; PMID: 21844027
- Johnson S, Schriever C, Patel U, Patel T, Hecht DW, Gerding DN. Rifaximin Redux: treatment of recurrent Clostridium difficile infections with rifaximin immediately post-vancomycin treatment. Anaerobe 2009; 15:290 - 1; http://dx.doi.org/10.1016/j.anaerobe.2009.08.004; PMID: 19698797
- Barker RH Jr., Dagher R, Davidson DM, Marquis JK. Review article: tolevamer, a novel toxin-binding polymer: overview of preclinical pharmacology and physicochemical properties. Aliment Pharmacol Ther 2006; 24:1525 - 34; http://dx.doi.org/10.1111/j.1365-2036.2006.03157.x; PMID: 17206941
- Kurtz CB, Cannon EP, Brezzani A, Pitruzzello M, Dinardo C, Rinard E, et al. GT160-246, a toxin binding polymer for treatment of Clostridium difficile colitis. Antimicrob Agents Chemother 2001; 45:2340 - 7; http://dx.doi.org/10.1128/AAC.45.8.2340-2347.2001; PMID: 11451694
- Louie TJ, Peppe J, Watt CK, Johnson D, Mohammed R, Dow G, et al, Tolevamer Study Investigator Group. Tolevamer, a novel nonantibiotic polymer, compared with vancomycin in the treatment of mild to moderately severe Clostridium difficile-associated diarrhea. Clin Infect Dis 2006; 43:411 - 20; http://dx.doi.org/10.1086/506349; PMID: 16838228
- Bouza E, Dryden M, Mohammed R, Peppe J, Chasan-Taber S, Donovan J, et al. Results of a phase III clinical trial comparing tolevamer, vancomycin and metronizadole in patients with Clostridium difficile-associated diarrhorea. Eighteenth European Congress on Clinical Microbiology and Infectious Diseases. Barcelona, Spain. 0464. Clin Microbiol Infect 2008; 14:S103
- Rothstein DM, Shalish C, Murphy CK, Sternlicht A, Campbell LA. Development potential of rifalazil and other benzoxazinorifamycins. Expert Opin Investig Drugs 2006; 15:603 - 23; http://dx.doi.org/10.1517/13543784.15.6.603; PMID: 16732714
- Anton PM, O’Brien M, Kokkotou E, Eisenstein B, Michaelis A, Rothstein D, et al. Rifalazil treats and prevents relapse of clostridium difficile-associated diarrhea in hamsters. Antimicrob Agents Chemother 2004; 48:3975 - 9; http://dx.doi.org/10.1128/AAC.48.10.3975-3979.2004; PMID: 15388461
- Freeman J, Baines SD, Jabes D, Wilcox MH. Comparison of the efficacy of ramoplanin and vancomycin in both in vitro and in vivo models of clindamycin-induced Clostridium difficile infection. J Antimicrob Chemother 2005; 56:717 - 25; http://dx.doi.org/10.1093/jac/dki321; PMID: 16143709
- Gerding DN, Muto CA, Owens RC Jr. Treatment of Clostridium difficile infection. Clin Infect Dis 2008; 46:Suppl 1 S32 - 42; http://dx.doi.org/10.1086/521860; PMID: 18177219
- Rossignol JF, Kabil SM, El-Gohary Y, Younis AM. Nitazoxanide in the treatment of amoebiasis. Trans R Soc Trop Med Hyg 2007; 101:1025 - 31; http://dx.doi.org/10.1016/j.trstmh.2007.04.001; PMID: 17658567
- Rossignol JF, Kabil SM, Said M, Samir H, Younis AM. Effect of nitazoxanide in persistent diarrhea and enteritis associated with Blastocystis hominis. Clin Gastroenterol Hepatol 2005; 3:987 - 91; http://dx.doi.org/10.1016/S1542-3565(05)00427-1; PMID: 16234044
- Dubreuil L, Houcke I, Mouton Y, Rossignol JF. In vitro evaluation of activities of nitazoxanide and tizoxanide against anaerobes and aerobic organisms. Antimicrob Agents Chemother 1996; 40:2266 - 70; PMID: 8891127
- Ochsner UA, Bell SJ, O’Leary AL, Hoang T, Stone KC, Young CL, et al. Inhibitory effect of REP3123 on toxin and spore formation in Clostridium difficile, and in vivo efficacy in a hamster gastrointestinal infection model. J Antimicrob Chemother 2009; 63:964 - 71; http://dx.doi.org/10.1093/jac/dkp042; PMID: 19251726
- Baines SD, O’Connor R, Saxton K, Freeman J, Wilcox MH. Comparison of oritavancin versus vancomycin as treatments for clindamycin-induced Clostridium difficile PCR ribotype 027 infection in a human gut model. J Antimicrob Chemother 2008; 62:1078 - 85; http://dx.doi.org/10.1093/jac/dkn358; PMID: 18772161
- Massot J, Sanchez O, Couchy R, Astoin J, Parodi AL. Bacterio-pharmacological activity of Saccharomyces boulardii in clindamycin-induced colitis in the hamster. Arzneimittelforschung 1984; 34:794 - 7; PMID: 6541916
- Toothaker RD, Elmer GW. Prevention of clindamycin-induced mortality in hamsters by Saccharomyces boulardii. Antimicrob Agents Chemother 1984; 26:552 - 6; PMID: 6517545
- Tempe´ JD, Steidel AL, Blehaut H, Hasselmann M, Lutun P, Maurier F. [Prevention of diarrhea administering Saccharomyces boulardii during continuous enteral feeding]. Sem Hop 1983; 59:1409 - 12; PMID: 6306827
- McFarland LV, Surawicz CM, Greenberg RN, Fekety R, Elmer GW, Moyer KA, et al. A randomized placebo-controlled trial of Saccharomyces boulardii in combination with standard antibiotics for Clostridium difficile disease. JAMA 1994; 271:1913 - 8; http://dx.doi.org/10.1001/jama.1994.03510480037031; PMID: 8201735
- McCullough MJ, Clemons KV, McCusker JH, Stevens DA. Species identification and virulence attributes of Saccharomyces boulardii (nom. inval.). J Clin Microbiol 1998; 36:2613 - 7; PMID: 9705402
- Bliss DZ, Johnson S, Savik K, Clabots CR, Willard K, Gerding DN. Acquisition of Clostridium difficile and Clostridium difficile-associated diarrhea in hospitalized patients receiving tube feeding. Ann Intern Med 1998; 129:1012 - 9; PMID: 9867755
- O’Keefe SJ. Tube feeding, the microbiota, and Clostridium difficile infection. World J Gastroenterol 2010; 16:139 - 42; http://dx.doi.org/10.3748/wjg.v16.i2.139; PMID: 20066732
- Naaber P, Mikelsaar RH, Salminen S, Mikelsaar M. Bacterial translocation, intestinal microflora and morphological changes of intestinal mucosa in experimental models of Clostridium difficile infection. J Med Microbiol 1998; 47:591 - 8; http://dx.doi.org/10.1099/00222615-47-7-591; PMID: 9839563
- Wilson KH, Sheagren JN. Antagonism of toxigenic Clostridium difficile by nontoxigenic C. difficile. J Infect Dis 1983; 147:733 - 6; http://dx.doi.org/10.1093/infdis/147.4.733; PMID: 6842009
- Borriello SP, Barclay FE. Protection of hamsters against Clostridium difficile ileocaecitis by prior colonisation with non-pathogenic strains. J Med Microbiol 1985; 19:339 - 50; http://dx.doi.org/10.1099/00222615-19-3-339; PMID: 4009689
- Szczesny A, Martirosian G, Cohen S, Silva J Jr. Co-infection of hamsters with toxin A or toxin B-deficient Clostridium difficile strains. Pol J Microbiol 2005; 54:301 - 4; PMID: 16599301
- Merrigan MM, Sambol SP, Johnson S, Gerding DN. New approach to the management of Clostridium difficile infection: colonisation with non-toxigenic C. difficile during daily ampicillin or ceftriaxone administration. Int J Antimicrob Agents 2009; 33:Suppl 1 S46 - 50; http://dx.doi.org/10.1016/S0924-8579(09)70017-2; PMID: 19303570
- Kyne L, Warny M, Qamar A, Kelly CP. Asymptomatic carriage of Clostridium difficile and serum levels of IgG antibody against toxin A. N Engl J Med 2000; 342:390 - 7; http://dx.doi.org/10.1056/NEJM200002103420604; PMID: 10666429
- Goulding D, Thompson H, Emerson J, Fairweather NF, Dougan G, Douce GR. Distinctive profiles of infection and pathology in hamsters infected with Clostridium difficile strains 630 and B1. Infect Immun 2009; 77:5478 - 85; http://dx.doi.org/10.1128/IAI.00551-09; PMID: 19752031
- Collignon A. Methods for working with the mouse model. Methods Mol Biol 2010; 646:229 - 37; http://dx.doi.org/10.1007/978-1-60327-365-7_15; PMID: 20597013
- Torres J, Jennische E, Lange S, Lönnroth I. Enterotoxins from Clostridium difficile; diarrhoeogenic potency and morphological effects in the rat intestine. Gut 1990; 31:781 - 5; http://dx.doi.org/10.1136/gut.31.7.781; PMID: 2115017
- Savidge TC, Pan WH, Newman P, O’brien M, Anton PM, Pothoulakis C. Clostridium difficile toxin B is an inflammatory enterotoxin in human intestine. Gastroenterology 2003; 125:413 - 20; http://dx.doi.org/10.1016/S0016-5085(03)00902-8; PMID: 12891543
- Stubbe H, Berdoz J, Kraehenbuhl JP, Corthe´sy B. Polymeric IgA is superior to monomeric IgA and IgG carrying the same variable domain in preventing Clostridium difficile toxin A damaging of T84 monolayers. J Immunol 2000; 164:1952 - 60; PMID: 10657645
- Castagliuolo I, LaMont JT, Nikulasson ST, Pothoulakis C. Saccharomyces boulardii protease inhibits Clostridium difficile toxin A effects in the rat ileum. Infect Immun 1996; 64:5225 - 32; PMID: 8945570
- Barc MC, Charrin-Sarnel C, Rochet V, Bourlioux F, Sandre´ C, Boureau H, et al. Molecular analysis of the digestive microbiota in a gnotobiotic mouse model during antibiotic treatment: Influence of Saccharomyces boulardii. Anaerobe 2008; 14:229 - 33; http://dx.doi.org/10.1016/j.anaerobe.2008.04.003; PMID: 18511310
- Corthier G, Dubos F, Raibaud P. Modulation of cytotoxin production by Clostridium difficile in the intestinal tracts of gnotobiotic mice inoculated with various human intestinal bacteria. Appl Environ Microbiol 1985; 49:250 - 2; PMID: 3977313
- Wilson KH, Freter R. Interaction of Clostridium difficile and Escherichia coli with microfloras in continuous-flow cultures and gnotobiotic mice. Infect Immun 1986; 54:354 - 8; PMID: 3533778
- Wilson KH, Perini F. Role of competition for nutrients in suppression of Clostridium difficile by the colonic microflora. Infect Immun 1988; 56:2610 - 4; PMID: 3417352
- Boureau H, Guichet C, Romond M, Boulioux P. Anaerobic strains responsible for the colonisation resistance to Clostridium difficile:Importance of the first srain colonising the digestive tract of axenic mice. In: Borriello, SP (ed.). Clinical and Molecular aspects of Anaerobes. (Wrightson Biomedical Publishing Ltd., 1990, pp. 107-112.
- Lawley TD, Clare S, Deakin LJ, Goulding D, Yen JL, Raisen C, et al. Use of purified Clostridium difficile spores to facilitate evaluation of health care disinfection regimens. Appl Environ Microbiol 2010; 76:6895 - 900; http://dx.doi.org/10.1128/AEM.00718-10; PMID: 20802075
- Lawley TD, Clare S, Walker AW, Goulding D, Stabler RA, Croucher N, et al. Antibiotic treatment of clostridium difficile carrier mice triggers a supershedder state, spore-mediated transmission, and severe disease in immunocompromised hosts. Infect Immun 2009; 77:3661 - 9; http://dx.doi.org/10.1128/IAI.00558-09; PMID: 19564382
- Lawley TD, Croucher NJ, Yu L, Clare S, Sebaihia M, Goulding D, et al. Proteomic and genomic characterization of highly infectious Clostridium difficile 630 spores. J Bacteriol 2009; 191:5377 - 86; http://dx.doi.org/10.1128/JB.00597-09; PMID: 19542279
- Giel JL, Sorg JA, Sonenshein AL, Zhu J. Metabolism of bile salts in mice influences spore germination in Clostridium difficile. PLoS One 2010; 5:e8740; http://dx.doi.org/10.1371/journal.pone.0008740; PMID: 20090901
- Pawlowski SW, Calabrese G, Kolling GL, Platts-Mills J, Freire R, AlcantaraWarren C, et al. Murine model of Clostridium difficile infection with aged gnotobiotic C57BL/6 mice and a BI/NAP1 strain. J Infect Dis 2010; 202:1708 - 12; http://dx.doi.org/10.1086/657086; PMID: 20977342
- Ghose C, Kalsy A, Sheikh A, Rollenhagen J, John M, Young J, et al. Transcutaneous immunization with Clostridium difficile toxoid A induces systemic and mucosal immune responses and toxin A-neutralizing antibodies in mice. Infect Immun 2007; 75:2826 - 32; http://dx.doi.org/10.1128/IAI.00127-07; PMID: 17371854
- Gardiner DF, Rosenberg T, Zaharatos J, Franco D, Ho DD. A DNA vaccine targeting the receptor-binding domain of Clostridium difficile toxin A. Vaccine 2009; 27:3598 - 604; http://dx.doi.org/10.1016/j.vaccine.2009.03.058; PMID: 19464540
- Triadafilopoulos G, Pothoulakis C, O’Brien MJ, LaMont JT. Differential effects of Clostridium difficile toxins A and B on rabbit ileum. Gastroenterology 1987; 93:273 - 9; PMID: 3596162
- Yaeger MJ, Kinyon JM, Glenn Songer J. A prospective, case control study evaluating the association between Clostridium difficile toxins in the colon of neonatal swine and gross and microscopic lesions. J Vet Diagn Invest 2007; 19:52 - 9; http://dx.doi.org/10.1177/104063870701900108; PMID: 17459832
- Debast SB, van Leengoed LA, Goorhuis A, Harmanus C, Kuijper EJ, Bergwerff AA. Clostridium difficile PCR ribotype 078 toxinotype V found in diarrhoeal pigs identical to isolates from affected humans. Environ Microbiol 2009; 11:505 - 11; http://dx.doi.org/10.1111/j.1462-2920.2008.01790.x; PMID: 19196280
- Songer JG, Anderson MA. Clostridium difficile: an important pathogen of food animals. Anaerobe 2006; 12:1 - 4; http://dx.doi.org/10.1016/j.anaerobe.2005.09.001; PMID: 16701605
- Doty JE, Pitt HA, Kuchenbecker SL, DenBesten L. Impaired gallbladder emptying before gallstone formation in the prairie dog. Gastroenterology 1983; 85:168 - 74; PMID: 6303888
- Onderdonk AB, Lowe BR, Bartlett JG. Effect of environmental stress on Clostridium difficile toxin levels during continuous cultivation. Appl Environ Microbiol 1979; 38:637 - 41; PMID: 44176
- Baines SD, Freeman J, Wilcox MH. Tolevamer is not efficacious in the neutralization of cytotoxin in a human gut model of Clostridium difficile infection. Antimicrob Agents Chemother 2009; 53:2202 - 4; http://dx.doi.org/10.1128/AAC.01085-08; PMID: 19223641
- Bowden TA Jr., Mansberger AR Jr., Lykins LE. Pseudomembraneous enterocolitis: mechanism for restoring floral homeostasis. Am Surg 1981; 47:178 - 83; PMID: 7224366
- Rolfe RD, Helebian S, Finegold SM. Bacterial interference between Clostridium difficile and normal fecal flora. J Infect Dis 1981; 143:470 - 5; http://dx.doi.org/10.1093/infdis/143.3.470; PMID: 6785366
- Schwan A, Sjölin S, Trottestam U, Aronsson B. Relapsing Clostridium difficile enterocolitis cured by rectal infusion of normal faeces. Scand J Infect Dis 1984; 16:211 - 5; http://dx.doi.org/10.3109/00365548409087145; PMID: 6740251
- Borriello SP. 12th C. L. Oakley lecture. Pathogenesis of Clostridium difficile infection of the gut. J Med Microbiol 1990; 33:207 - 15; http://dx.doi.org/10.1099/00222615-33-4-207; PMID: 2258909
- Adams DA, Riggs MM, Donskey CJ. Effect of fluoroquinolone treatment on growth of and toxin production by epidemic and nonepidemic clostridium difficile strains in the cecal contents of mice. Antimicrob Agents Chemother 2007; 51:2674 - 8; http://dx.doi.org/10.1128/AAC.01582-06; PMID: 17562807
- Pultz NJ, Donskey CJ. Effect of antibiotic treatment on growth of and toxin production by Clostridium difficile in the cecal contents of mice. Antimicrob Agents Chemother 2005; 49:3529 - 32; http://dx.doi.org/10.1128/AAC.49.8.3529-3532.2005; PMID: 16048976
- Settle CD, Wilcox MH, Fawley WN, Corrado OJ, Hawkey PM. Prospective study of the risk of Clostridium difficile diarrhoea in elderly patients following treatment with cefotaxime or piperacillin-tazobactam. Aliment Pharmacol Ther 1998; 12:1217 - 23; http://dx.doi.org/10.1046/j.1365-2036.1998.00428.x; PMID: 9882029
- Baines SD, Freeman J, Wilcox MH. Effects of piperacillin/tazobactam on Clostridium difficile growth and toxin production in a human gut model. J Antimicrob Chemother 2005; 55:974 - 82; http://dx.doi.org/10.1093/jac/dki120; PMID: 15860551
- Jump RL, Li Y, Pultz MJ, Kypriotakis G, Donskey CJ. Tigecycline exhibits inhibitory activity against Clostridium difficile in the colon of mice and does not promote growth or toxin production. Antimicrob Agents Chemother 2011; 55:546 - 9; http://dx.doi.org/10.1128/AAC.00839-10; PMID: 21135181
- Baines SD, Saxton K, Freeman J, Wilcox MH. Tigecycline does not induce proliferation or cytotoxin production by epidemic Clostridium difficile strains in a human gut model. J Antimicrob Chemother 2006; 58:1062 - 5; http://dx.doi.org/10.1093/jac/dkl364; PMID: 17030519
- Wilcox MH. Evidence for low risk of Clostridium difficile infection associated with tigecycline. Clin Microbiol Infect 2007; 13:949 - 52; http://dx.doi.org/10.1111/j.1469-0691.2007.01792.x; PMID: 17697004
- Stiefel U, Nerandzic MM, Koski P, Donskey CJ. Orally administered beta-lactamase enzymes represent a novel strategy to prevent colonization by Clostridium difficile. J Antimicrob Chemother 2008; 62:1105 - 8; http://dx.doi.org/10.1093/jac/dkn298; PMID: 18693236
- Meader E, Mayer MJ, Gasson MJ, Steverding D, Carding SR, Narbad A. Bacteriophage treatment significantly reduces viable Clostridium difficile and prevents toxin production in an in vitro model system. Anaerobe 2010; 16:549 - 54; http://dx.doi.org/10.1016/j.anaerobe.2010.08.006; PMID: 20816997
- Nakamura S, Mikawa M, Tanabe N, Yamakawa K, Nishida S. Effect of clindamycin on cytotoxin production by Clostridium difficile. Microbiol Immunol 1982; 26:985 - 92; PMID: 7167065
- Honda T, Hernadez I, Katoh T, Miwatani T. Stimulation of enterotoxin production of Clostridium difficile ny antibiotics. Lancet 1983; 1:655; http://dx.doi.org/10.1016/S0140-6736(83)91832-9; PMID: 6131337
- Golledge CL, Carson CF, O’Neill GL, Bowman RA, Riley TV. Ciprofloxacin and Clostridium difficile-associated diarrhoea. J Antimicrob Chemother 1992; 30:141 - 7; http://dx.doi.org/10.1093/jac/30.2.141; PMID: 1399923
- Drummond LJ, Smith DG, Poxton IR. Effects of sub-MIC concentrations of antibiotics on growth of and toxin production by Clostridium difficile. J Med Microbiol 2003; 52:1033 - 8; http://dx.doi.org/10.1099/jmm.0.05387-0; PMID: 14614060
- Barc MC, Depitre C, Corthier G, Collignon A, Su WJ, Bourlioux P. Effects of antibiotics and other drugs on toxin production in Clostridium difficile in vitro and in vivo. Antimicrob Agents Chemother 1992; 36:1332 - 5; PMID: 1416834
- Baines SD, O’Connor R, Huscroft G, Saxton K, Freeman J, Wilcox MH. Mecillinam: a low-risk antimicrobial agent for induction of Clostridium difficile infection in an in vitro human gut model. J Antimicrob Chemother 2009; 63:838 - 9; http://dx.doi.org/10.1093/jac/dkp017; PMID: 19211576
- Baines SD, O’Connor R, Saxton K, Freeman J, Wilcox MH. Activity of vancomycin against epidemic Clostridium difficile strains in a human gut model. J Antimicrob Chemother 2009; 63:520 - 5; http://dx.doi.org/10.1093/jac/dkn502; PMID: 19112083
- Baines SD, Noel AR, Huscroft GS, Todhunter SL, O’Connor R, Hobbs JK, et al. Evaluation of linezolid for the treatment of Clostridium difficile infection caused by epidemic strains using an in vitro human gut model. J Antimicrob Chemother 2011; 66:1537 - 46; http://dx.doi.org/10.1093/jac/dkr155; PMID: 21504940
- Baines SD, Huscroft G, Freeman J, Todhunter SL, Wilcox MH. Co-infection of an in vitro human gut model of Clostridium difficile infection with two epidemic C. difficile strains. In Programs and Abstracts of the 50th Interscience Conference on Antimicrobial Agents and Chemotherapy, Boston. 2010. Abstract K-1303. American Society for Microbiology, Washington, DC.
- Freeman J, O’Neill FJ, Wilcox MH. Effects of cefotaxime and desacetylcefotaxime upon Clostridium difficile proliferation and toxin production in a triple-stage chemostat model of the human gut. J Antimicrob Chemother 2003; 52:96 - 102; http://dx.doi.org/10.1093/jac/dkg267; PMID: 12775682
- Freeman J, Baines SD, Saxton K, Wilcox MH. Effect of metronidazole on growth and toxin production by epidemic Clostridium difficile PCR ribotypes 001 and 027 in a human gut model. J Antimicrob Chemother 2007; 60:83 - 91; http://dx.doi.org/10.1093/jac/dkm113; PMID: 17483547
- Huscroft G, Baines SD, Todhunter SL, Freeman J, Wilcox M. NVB302 demonstrates non-inferiority to vancomycin in treatment of Clostridium difficile NAP1/027 in an in vitro human gut model. In Programs and Abstracts of the 50th Interscience Conference on Antimicrobial Agents and Chemotherapy, Boston. 2010. Abstract F-1607. American Society for Microbiology, Washington, DC.
- Saxton K, Baines SD, Freeman J, O’Connor R, Wilcox MH. Effects of exposure of Clostridium difficile PCR ribotypes 027 and 001 to fluoroquinolones in a human gut model. Antimicrob Agents Chemother 2009; 53:412 - 20; http://dx.doi.org/10.1128/AAC.00306-08; PMID: 18710908
- Yamamoto-Osaki T, Kamiya S, Sawamura S, Kai M, Ozawa A. Growth inhibition of Clostridium difficile by intestinal flora of infant faeces in continuous flow culture. J Med Microbiol 1994; 40:179 - 87; http://dx.doi.org/10.1099/00222615-40-3-179; PMID: 8114067
- Hopkins MJ, Macfarlane GT. Changes in predominant bacterial populations in human faeces with age and with Clostridium difficile infection. J Med Microbiol 2002; 51:448 - 54; PMID: 11990498
- Macfarlane GT, Macfarlane S, Gibson GR. Validation of a Three-Stage Compound Continuous Culture System for Investigating the Effect of Retention Time on the Ecology and Metabolism of Bacteria in the Human Colon. Microb Ecol 1998; 35:180 - 7; http://dx.doi.org/10.1007/s002489900072; PMID: 9541554
- Rea MC, Dobson A, O’Sullivan O, Crispie F, Fouhy F, Cotter PD, et al. Effect of broad- and narrow-spectrum antimicrobials on Clostridium difficile and microbial diversity in a model of the distal colon. Proc Natl Acad Sci U S A 2011; 108:Suppl 1 4639 - 44; http://dx.doi.org/10.1073/pnas.1001224107; PMID: 20616009
- Warny M, Pepin J, Fang A, Killgore G, Thompson A, Brazier J, et al. Toxin production by an emerging strain of Clostridium difficile associated with outbreaks of severe disease in North America and Europe. Lancet 2005; 366:1079 - 84; http://dx.doi.org/10.1016/S0140-6736(05)67420-X; PMID: 16182895
- Musher DM, Aslam S, Logan N, Nallacheru S, Bhaila I, Borchert F, et al. Relatively poor outcome after treatment of Clostridium difficile colitis with metronidazole. Clin Infect Dis 2005; 40:1586 - 90; http://dx.doi.org/10.1086/430311; PMID: 15889354
- Pepin J, Alary ME, Valiquette L, Raiche E, Ruel J, Fulop K, et al. Increasing risk of relapse after treatment of Clostridium difficile colitis in Quebec, Canada. Clin Infect Dis 2005; 40:1591 - 7; http://dx.doi.org/10.1086/430315; PMID: 15889355
- Freeman J, Fawley W, Hobbs JK, Else P, Verity P, Parnell P, et al. Decreasing metronizadole susceptibility in epidemic Clostridium difficile strains. In Programs and Abstracts of the 50th Interscience Conference on Antimicrobial Agents and Chemotherapy, San Fransisco. CA 2009. Abstract C2-1964. American Society for Microbiology, Washington, DC.
- Baines SD, O’Connor R, Freeman J, Fawley WN, Harmanus C, Mastrantonio P, et al. Emergence of reduced susceptibility to metronidazole in Clostridium difficile. J Antimicrob Chemother 2008; 62:1046 - 52; http://dx.doi.org/10.1093/jac/dkn313; PMID: 18693234
- Douce G, Goulding D. Refinement of the hamster model of Clostridium difficile disease. Methods Mol Biol 2010; 646:215 - 27; http://dx.doi.org/10.1007/978-1-60327-365-7_14; PMID: 20597012
- van Minnen LP, Blom M, Timmerman HM, Visser MR, Gooszen HG, Akkermans LM. The use of animal models to study bacterial translocation during acute pancreatitis. J Gastrointest Surg 2007; 11:682 - 9; http://dx.doi.org/10.1007/s11605-007-0088-0; PMID: 17468930
- Wells CL, Hess DJ, Erlandsen SL. Impact of the indigenous flora in animal models of shock and sepsis. Shock 2004; 22:562 - 8; http://dx.doi.org/10.1097/01.shk.0000145935.24344.2d; PMID: 15545829
- Geric B, Carman RJ, Rupnik M, Genheimer CW, Sambol SP, Lyerly DM, et al. Binary toxin-producing, large clostridial toxin-negative Clostridium difficile strains are enterotoxic but do not cause disease in hamsters. J Infect Dis 2006; 193:1143 - 50; http://dx.doi.org/10.1086/501368; PMID: 16544255
- Weinberg DS, Fernandes PB, Kao CC, Clark JM, Bonner DP, Sykes RB. Evaluation of aztreonam, cefoperazone, latamoxef and ceftazidime in the hamster colitis model. J Antimicrob Chemother 1986; 18:729 - 45; http://dx.doi.org/10.1093/jac/18.6.729; PMID: 3546243
- Rukhlina AA, Malkova LA, Shevniuk LA, Fomina TV, Bukhman VM, Koroleva VM, et al. [Eremomycin in the treatment of antibiotic-associated colitis in golden hamsters]. Antibiot Khimioter 1992; 37:20 - 2; PMID: 1514862
- Nord CE, Lindmark A, Persson I. Efficacy of daptomycin compared to teicoplanin in the treatment of Clostridium difficile colitis in hamsters. J Chemother 1989; 1:Suppl 209 - 10; PMID: 16312372
- Theodorou V, Chovet M, Eutamene H, Fargeau H, Dassaud M, Toulouse M, et al. Antidiarrhoeal properties of a novel sigma ligand (JO 2871) on toxigenic diarrhoea in mice: mechanisms of action. Gut 2002; 51:522 - 8; http://dx.doi.org/10.1136/gut.51.4.522; PMID: 12235074
- McVey DC, Liddle RA, Riggs-Sauthier J, Ekwuribe N, Vigna SR. Inhibition of Clostridium difficile toxin A-induced colitis in rats by APAZA. Dig Dis Sci 2005; 50:565 - 73; http://dx.doi.org/10.1007/s10620-005-2476-1; PMID: 15810644
- Chang TW, Dong MY, Gorbach SL. Effect of bismuth subsalicylate on Clostridium difficile colitis in hamsters. Rev Infect Dis 1990; 12:Suppl 1 S57 - 8; http://dx.doi.org/10.1093/clinids/12.Supplement_1.S57; PMID: 2305177
- Wilson KH, Sheagren JN, Freter R, Weatherbee L, Lyerly D. Gnotobiotic models for study of the microbial ecology of Clostridium difficile and Escherichia coli. J Infect Dis 1986; 153:547 - 51; http://dx.doi.org/10.1093/infdis/153.3.547; PMID: 3512730
- de Araújo Junqueira AF, Dias AA, Vale ML, Spilborghs GM, Bossa AS, Lima BB, et al. Adenosine deaminase inhibition prevents Clostridium difficile toxin A-induced enteritis in mice. Infect Immun 2011; 79:653 - 62; http://dx.doi.org/10.1128/IAI.01159-10; PMID: 21115723