Abstract
We found that immunostimulation of the intestinal immune system of neonatal mice by poly(I:C) injection decreased intestinal infection by the parasite Cryptosporidium parvum. We showed that the presence of dendritic cells and the cooperation of mutually dependent cytokines, such as IL-12p40, and type I and type II IFNs, were involved in the mechanism of protection induced by poly(I:C). This protection is dependent not only on TLR3-TRIF signaling, but also on the activation of the TLR5-MyD88 pathway by gut microbiota. These results raise the possibility that flagellated intestinal commensal bacteria may, in the presence of natural or synthetic agonists of TLR3, provide synergy between the TRIF and MyD88 signaling pathways, thereby favoring the development of mucosal defenses. In this addendum, we summarize these recent findings and discuss their implications for neonatal infections and immunomodulatory strategies.
Introduction
Both mouse and human neonates have a weaker immune response to infection than adults of the same species, resulting in a greater susceptibility to infections, particularly those caused by pathogens invading the mucosa of the respiratory and gastro-intestinal tracts. According to the World Health Organization, diarrhea accounts for 10.5% of the almost eight million deaths of children under the age of five years occurring annually. Various enteropathogenic microorganisms, including rotavirus, Cryptosporidium, Shigella, Listeria monocytogenes and Salmonella enterica affect neonates and infants more severely than older individuals.
Cryptosporidiosis, which is caused by the protozoan parasite Cryptosporidium, is a zoonotic disease affecting immunocompromised individuals and children under the age of five years. It is currently the second leading cause of diarrheal disease and death in infants and the leading cause of neonatal diarrhea in calves.Citation1 The entire development of Cryptosporidium takes place in intestinal epithelial cells in about four days. It begins with the ingestion of fully sporulated, environmentally resistant oocysts. This resistant form of the parasite is spherical or slightly ovoid, measuring 4.5 × 5.5 μm and contains four elongated sporozoites. During excystation in the upper small intestine, sporozoites released from oocysts penetrate the mucus layer and attach to enterocytes. During host cell invasion, sporozoites do not enter the cytoplasm but the adjacent epithelial membrane moves to encapsulate the sporozoite, providing a niche for parasite development called the parasitophorous vacuole. Asexual reproduction produces merozoites that infect new cells in which new parasitophorous vacuoles are produced. Later generations of merozoites undergo sexual differentiation that leads to formation of new oocysts with either a thick wall (80%) or a thin wall (20%), containing four sporozoites. The thick-walled oocysts are released into the lumen of the intestine, are excreted from the host in the feces, and are immediately infective, allowing the spread of infection to other susceptible hosts. In addition, Cryptosporidium is able to autoinfect the same host. Autoinfection occurs through the thin-walled oocysts, which excyst once they are separated from the epithelium, and the cycle starts again. Autoinfection and the recycling of type I meronts provide an explanation for persistent chronic infection (for a review, see ref. Citation2).
As in ruminants, susceptibility to Cryptosporidium parvum in mice is maximal during the first two weeks after birth, subsequently decreasing considerably during weaning. The age-dependent susceptibility to this intestinal infection is associated with incomplete colonization of the lamina propria by various types of immune cells, including dendritic cells (DCs), macrophages and T lymphocytes, and low basal levels of the type II interferon required to prevent parasite development in epithelial cells.Citation3-Citation5 We recently highlighted the importance of innate immunity for control of the acute phase of this infection in neonatal mice, with intestinal DCs, which are massively recruited during infection, playing a particularly crucial role.Citation5
During infection, immune responses are generally triggered by the recognition of microbial molecules by cellular sensors known as pattern recognition receptors (PRR), such as Toll-like receptors (TLRs), Nod-like receptors (NLRs) and helicases. In the intestine, these innate receptors are expressed on intestinal epithelial cells and on immune cells, such as mononuclear phagocytes. Activated PRRs induce individual intracellular signaling pathways leading to the release of antimicrobial peptides, the induction of phagocytosis, the secretion of cytokines and chemokines and the initiation of adaptive immunity. PRR ligands are also expressed by the commensal bacteria rapidly colonizing the intestinal mucosa of neonates shortly after birth. Indeed, birth leads to the gut epithelium coming into contact with microorganisms for the first time. Hosts and bacteria have thus developed strategies for maintaining 'friendly' relationships and controlling the signaling cascades occurring downstream from Toll-like receptors (TLRs).Citation6
We and others have shown that, in several mammalian species, including humans, mice, pigs, goats and sheep, the in vitro stimulation of neonatal cells with different TLR ligands induces a stronger inflammatory response than the stimulation of adult cells, with the production of large amounts of IL-12p40, IFN-γ, TNF-α, or IL-6.Citation7-Citation9 Various in vivo studies have demonstrated that neonatal mice display much stronger systemic or intestinal inflammatory responses than adult mice in response to stimulation with LPS and CpG, respectively.Citation10,Citation11 CpG administration has been shown to favor the recruitment of various immune cells to the intestinal lamina propria of neonates and to protect newborn mice against C. parvum infection.Citation11,Citation12 These data suggest that the induction of transient pro-inflammatory signals in the intestine of neonates by TLR stimulation can improve the capacity of the neonates to clear pathogens. However, such inflammation should remain moderate, to prevent excessive activation of the innate immune system, which might lead to the death of the neonate.Citation10
We recently reported an unexpected role of microbiota in the protection of neonatal mice against C. parvum following stimulation with the TLR ligand, polyinosinic:polycytidylic acid (poly(I:C)). In this addendum, we summarize these recent findings and discuss their implications and impact in the domain of neonatal infectious diseases.
Specific Features of the Neonatal Immune System
Neonates and young infants are protected by the maternal antibodies transmitted through the placenta during gestation, and via the colostrum and breast milk after birth. However, these maternal antibodies are not always sufficient for the efficient control of infections in neonates. The immune system of neonates and young infants is immature, resulting in a greater susceptibility to most infections and a weaker response to vaccination than in older individuals.
Many differences between the immune systems of neonates and adults have been described, most relating to the adaptive immune response following infection or immunisation. There are also specific epigenetic differences between the immune responses of adults and neonates. In neonates, immune responses are skewed toward a Th2 profile. This skewing has been attributed to the pattern of DNA methylation, with hypermethylation of the IFNγ promoter and hypomethylation at the Th2 cytokine locus, restricting the production of the IFN−γ (for a review, see ref. Citation13). Moreover, microRNAs are often more strongly expressed in neonates than in adults, limiting the inflammatory response Considerable attention was initially focused on T helper cells and the Th2-biased cellular response observed in human and mouse neonates, but there is now increasing evidence to suggest that other differences exist for the regulatory cells of neonates and adults. For example, B1a cells expressing CD5 account for more than 30% of splenic B cells in seven-day-old neonatal mice, vs. about 2% in adults. Following TLR9 signaling, CD5+ B cells control the IL-12-dependent Th1-priming capacity of neonatal DCs.Citation14 These regulatory B cells have been shown to downregulate innate inflammation and DC functions in neonatal mice, by producing large amounts of IL-10 in response to TLR stimulation (for a review, see ref. Citation15). Neonatal immature erythroid cells have also recently been reported to play an immunomodulatory role in CD4+ T-cell responses. The selective high abundance of these cells in the neonatal spleen in the first few days after birth has a major impact on the overall immune response.Citation15
Despite these specific features, neonates have been reported to mount fully functional immune responses in some circumstances. It may therefore be possible to develop treatment or vaccination strategies effective against infectious agents at this developmental stage. For example, we and others have shown that the administration of PRR ligands to neonates effectively strengthens the innate immune response and increases resistance to infection. CpG, which mimics bacterial DNA and binds TLR9, stimulates the innate immune system in neonates, mediating the rapid control of pathogens, as described in brain infection with Tacaribe arenavirus,Citation16 lung infection with respiratory syncytial virusCitation17 and intestinal infection with C. parvum.Citation12 In all these neonatal infection models, CpG administration enhances the IFN-γ response, compensating for the naturally low levels of this cytokine in the intestines of neonates.Citation18 Poly(I:C), a synthetic dsRNA analog that acts as a PRR ligand, has often been used to control mucosal viral diseases (HSV2, etc.) and, occasionally, to control enteric bacteria, such as Yersinia enterocolitica or Salmonella Typhimurium in adult mice.Citation19-Citation21 In a model of neonatal vaccination with tetanus toxoid (TT), a combination of poly(I:C) and retinoic acid was shown to enhance primary and secondary anti-TT-IgG responses and TT-specific IFN-γ responses.Citation22 We recently demonstrated, for the first time, that the administration of poly(I:C) as an immunostimulant efficiently controls an intestinal protozoan infection in mouse neonates.Citation23
The Role of Poly(I:C) in the Stimulation of Innate Immune Responses in Neonates
Poly(I:C) is a ligand of many pathogen recognition receptors, including TLR3, melanoma differentiation-associated protein 5 (MDA-5), retinoic acid-inducible gene 1 (RIG-1), and dsRNA-dependent protein kinase R (PKR). In adult mice, the in vivo injection of poly(I:C) stimulates the rapid production of IL-6, IL-10, IL-12 p40, MCP-1, TNFα, type I IFN, and IFN-γ, resulting in significant activation of DCs and NK cells. When co-injected with a protein antigen, poly(I:C) primes CD4+ T cells to produce Th1-type cytokines, stimulates antibody responses and promotes the cross-presentation of antigen to CD8+ T cells by DCs through TLR3 signaling.Citation24,Citation25
We investigated the ability of poly(I:C) to simulate the intestinal innate immune system of neonates. We observed that a single injection of poly(I:C) induced a rapid decrease in parasite load.Citation23 The protective mechanism was critically dependent on the TLR3 signaling molecule TRIF and the cytokines IL-12p40 and type I and II IFNs. Studies by McAllister and Zhou showed that the injection of poly(I:C) into adult mice induced a rapid remodelling of the small intestinal mucosa, with significant villus shortening.Citation26,Citation27 These two studies differed in terms of the conclusions drawn about the mechanisms by which poly(I:C) induced intestinal damage (roles of IL-15 and CD8αα intraepithelial lymphocytes), but both showed that TLR3 expression on intestinal epithelial cells was required. We observed no such intestinal damage, probably because the dose of poly(I:C) we injected was lower than that in the studies by McAllister and Zhou (by factors of 7 and 25, respectively). Moreover, during the first 10 d of life, TLR3 expression on intestinal epithelial cells was shown to be much weaker than that in adult mice.Citation28 DCs and all other radiosensitive hematopoietic cells express TLR3 and have been shown to respond to poly(I:C) through this receptor.Citation29 Based on these data, we suggested that, in neonates, the TLR3+ cells actively recruited during C. parvum infection contribute to the elimination of the parasite following poly(I:C) administration. CD11chi ClassII+CD103+ cells are the conventional DCs in the intestinal compartment. A CD8α+ DC subset was recently reported to express TLR3 selectively in the lamina propria of adult mice.Citation30 We detected both CD8α- and CD8α+ DC subset in the neonatal intestine, and both these subsets expressed TLR3.Citation23 This suggests that these two DC subsets may respond directly to poly(I:C) stimulation and participate in the protective mechanism.
We demonstrated, in a conditional mouse model of CD11c+ cell depletion, that the protection induced by poly(I:C) required the presence of these cells (). Following the depletion of these cells, the levels of IL-12p40 and of IFN-β induced by poly(I:C) decreased rapidly and significantly, suggesting that CD11c+ cells were the source of these cytokines. Experiments with neonatal mice lacking IL-12p40 and IFN-αR confirmed that the mechanism of protection was critically dependent on these cytokines.Citation23 Interestingly, it has recently been reported that most of the changes in DC gene expression in response to poly(I:C) are dependent on type I IFN signaling rather than TLR3 signaling.Citation31 Indeed, in an inflammatory environment containing type-I IFNs, direct signaling through TLR3 was not required for DC maturation.Citation31 This demonstrates the importance of this cytokine for amplifying the immune response and supports our data demonstrating the dependence of type I receptor signaling on IFN-γ and IL-12p40 production after the injection of poly(I:C). Furthermore, we found, in the same experimental conditions, that IFN-β and IFN-γ levels were also very low in IL-12p40−/− neonatal mice, suggesting a more complex interdependence between these three cytokines.Citation23
Figure 1. The protection of neonates against Cryptosporidium parvum induced by poly(I:C) required the presence of CD11c+ dendritic cells but not CD3+ cells or NCR1+ cells. Three-day-old neonatal mice were orally infected with C. parvum and received one intraperitoneal injection of poly(I:C) (4 µg/g) 5 d later. These graphs show the intestinal parasite load 24 h after poly(I:C) injection, in neonatal mice depleted of dendritic cells (CD11c-DTR mice) (A), in CD3e-deficient neonatal mice (B), and in neonatal mice depleted of NCR1+ cells (NCR1-DTR mice) (C) (Each point represents a single mouse; bars represent the median; NS = not significant; *P < 0.05).
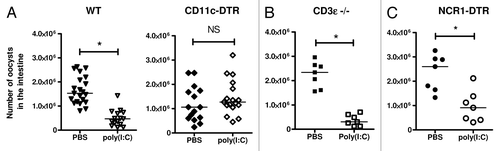
The development of C. parvum infection in intestinal epithelial cells is effectively controlled by both type I and type II IFNs.Citation32,Citation33 We observed that the injection of poly(I:C) into infected IFN-αR- and IFN-γ-deficient neonatal mice did not reduce the parasite load in the intestines.Citation23 Unlike type I IFNs, IFN-γ affects the invasion of enterocytes by C. parvum by decreasing the amount of cellular Fe2+ available.Citation32,Citation33 These different mechanisms of protection mediated by IFNs suggest synergy between these molecules in the control of parasite development. Type I and II IFNs bind to IFN-αR1-R2 and IFN-γR1-R2, respectively, but both induce the formation of Stat1-Stat1 homodimers, which are translocated to the nucleus, where they bind GAS (an IFN-γ-activated site) elements, thereby inducing gene transcription. However, only type I IFNs induce the formation of the Stat1-Stat2-IRF9 complex, which is translocated to the nucleus, where it binds to IFN-stimulated response elements (ISRE) to induce the expression of other genes. In our attempts to identify the IFN-γ-producing cells involved in protection, we were able to rule out conventional T cells, in studies performed in CD3ε-deficient neonatal mice (). Conventional NK (cNK) cells or ILC1 are another potential source of IFN-γ. However, McCartney et al. noted that mouse NK cells were not directly activated by poly(I:C)Citation29 but that both the type I and IL-12 produced by hematopoietic cells after poly(I:C) injection induced the production of IFN-γ by NK cells.Citation21 Moreover, the injection of poly(I:C) into IL15−/− neonatal mice, which lack IL15-dependent cNK cells, and neonatal mice with a transient depletion of NCR1+ cells, afforded protection against infection as effective as that achieved in wild-type neonatal mice (). This suggests that cNK cells (NCR1+, IL15-dependent), which are very rare in the neonatal intestine, are not involved in the protection induced by poly(I:C) at this age, despite their possible activation by type I IFN and IL-12.Citation5 NCR1 is also expressed in ILC subsets, but the fate of these cells in NCR1-DTR neonates after the administration of DT remains to be determined. It is, therefore, not yet possible to conclude that they play an active role in the mechanism of protection. We also recently demonstrated that, during C. parvum infection, the intestinal DCs of neonates are an additional source of IFN-γ.Citation5 We observed that the intestinal IFN-γ response was significantly weaker after poly(I:C) administration and the depletion of CD11c+ cells.Citation23 It therefore appears possible that an increase in IFN-γ expression by CD11c+ DCs expressing TLR3, in response to poly(I:C) stimulation, favors the rapid elimination of infected enterocytes.
Role of the Gut Flora in the Poly(I:C)-Induced Mechanism of Protection
While investigating the signaling pathways involved in the mechanism of protection mediated by poly(I:C), we unexpectedly observed that this protection was dependent not only on TRIF, but also on MyD88 pathway (). Some protozoan parasites exhibit TLR ligands such as profilin or GPI-anchored proteins, which target TLR11 and TLR2, respectively, both these receptors signaling via MyD88 (for a review see ref. Citation34). In a model of biliary cryptosporidiosis, an interaction between TLR2, TLR4 and Cryptosporidium was described, but the precise nature of the ligands was not identified.Citation35,Citation36 In the neonatal intestinal cryptosporidiosis model, we observed that an absence of TLR2 and TLR4 had no effect on parasite load in the intestine.Citation23 This raised questions about whether the microbiota in colonizing the neonatal intestine participated in the protection mechanism by triggering the MyD88 pathway. Indeed, the neonatal period is a critical window in development, during which the intestine undergoes a transition from complete sterility to a complex environment containing bacteria and dietary antigens. The microbiota colonizing the intestine have frequently been reported to be beneficial to the host in terms of both nutrition and immune system development. Moreover, intestinal bacteria also increase resistance to infection with pathogenic bacteria and provide protection against intestinal epithelial injury (for a review, see refCitation37). Baba et al. reported that a combination of Lactobacillus casei and TLR3 agonist synergistically increased IL-12p70 production by DCs in vitro.Citation38 We used neonatal mice with a reduced intestinal microflora to investigate the contribution of the gut flora to the protection of neonates against C. parvum infection after poly(I:C) administration. The microflora of these mice was limited by administering an antibiotic cocktail to the mother, via the drinking water. In this experimental design, poly(I:C) induced a significantly lower level of protection, and DC activation and IL-12p40 and IFN-β responses were also significantly weaker.Citation23
Figure 2. The protection of neonates against Cryptosporidium parvum induced by poly(I:C) required both TRIF and MyD88. Three-day-old neonatal mice were orally infected with C. parvum and received one intraperitoneal injection of poly(I:C) (4 µg/g) 5 d later. These graphs show the intestinal parasite load 24 h after poly(I:C) injection, in neonatal mice lacking TLR3, TRIF and MyD88 (*P < 0.05).
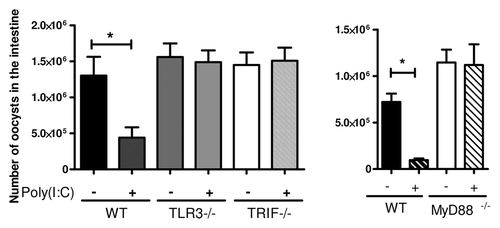
Among the TLR signaling through MyD88, we identified TLR5, that binds the bacterial flagellin, as a key receptor in the protection mediated by poly(I:C). This protein is not present in protozoans, but is produced by diverse gut commensals, including members of the phyla Firmicutes and Proteobacteria, but not by members of the Bacteroidetes.Citation39 TLR5 plays an important role in maintaining intestinal homeostasis and regulating host defenses against intestinal infection. In the intestine, TLR5 is expressed predominantly on mucosal DCs, but it is also present on intestinal epithelial cells on the basolateral side of the ileum, and on both the apical and basolateral sides of the colon.Citation40,Citation41 CD11c+CX3CR1+ CD11b+ macrophages also express TLR5, although Kinnebrew et al. have recently shown a preferential response of CD103+ DCs to TLR5 stimulation.Citation42 As reported by Fujimoto et al. for the intestine of adult mice, we found that TLR5 was expressed predominantly on the CD11c+CD103+CD8α- population in neonatal mice.Citation30 Mucosal infections favor the translocation of commensal bacteria and are generally associated with dysbiosis and/or an expansion of the population of commensal organisms with inflammatory potential (pathobionts), such as proteobacteria.Citation43,Citation44 We observed an increase in the intestinal permeability of C. parvum-infected neonates, suggesting that some flagellated commensal bacteria may be translocated and may stimulate CD8α- DCs via TLR5. In synergy with activation by poly (I:C), this leads to optimal IL-12 production after poly(I:C) stimulation, thus reducing the severity of the infection.Citation23
These results clearly demonstrate that co-operation between the TRIF and MyD88 signaling pathways enhances innate immune responses and resistance to C. parvum infection in neonates. During infection, several types of PRRs are activated simultaneously by the multiple PAMPs presented by pathogens, triggering an appropriate immune response. The simultaneous activation of several types of PRRs would be expected to have an effect different from that of the activation of a single PRR. NF-kB is a likely candidate for the main mediator of the synergistic effect of combined PRR stimulation. Indeed, this transcription factor is activated downstream from various PRRs and is a key regulator of the immune response controlling the expression of different cytokines (IL-6, IL-8, etc.), cytokine receptors (CCR2, IL2Ra, etc.), antimicrobial factors (lactoferrin, etc.) and anti-apoptotic proteins (Bcl2, etc.). Many studies have reported synergistic effects when members of two families of PRRs were activated simultaneously. For example, the combination of TLR5 and NOD1 stimulation potentiates intestinal and systemic levels of cytokine and antimicrobial peptide production in mice increasing resistance to Salmonella enterica serovar Typhimurium.Citation45 Moreover, TLR synergy, leading to an increase in the release of by DCs, has been reported to be most pronounced for a combination of MyD88-dependent TLR ligands and TRIF-dependent ligands.Citation46 For the induction of a protective immunity against Gram negative bacterial infection, Sotolongo et al. reported that macrophage TRIF signaling triggered by Yersinia enterocolitica LPS, and not just MyD88 signaling, is required.Citation20 Consistent with our results, the authors confirmed that, in addition to MyD88 signals, the simultaneous stimulation of the TRIF pathway by poly(I:C) was required for the effective control of intestinal Yersinia infection.Citation20
Strategies to Improve the Stability and Safety of TLR3 Ligands for Intestinal Targeting
The development of effective methods for protecting neonates against intestinal pathogens is challenging. We and others have clearly demonstrated that stimulation of the innate immune system is a promising strategy.Citation12,Citation16,Citation23 However, as mentioned above, the excessive stimulation of innate immunity can be highly damaging. Indeed, some studies have reported adverse effects of poly(I:C) due to the widespread expression of TLR3 and the recognition of poly(I:C) by other receptors, RIG-I and MDA-5.Citation27,Citation47 Moreover, poly(I:C) has been reported to increase the expression of class I MHC molecules by non-hematopoietic cells, favoring the presentation of self-antigens by these cells.Citation48 A recent review has described the “double-edged sword” effects of poly(I:C) and suggested the local delivery or the use of very low doses of poly(I:C) to minimize its toxicity.Citation48 In the neonatal cryptosporidiosis model, low doses of poly(I:C) were administered via the intraperitoneal route and were found to be sufficient for rapid parasite control. The oral route is generally preferred for the stimulation of a local (i.e., intestinal) response. We have shown that the oral administration of CpG to neonates affords protection against C. parvum, but this route of administration was unsuccessful for poly(I:C), probably due to the degradation of this molecule by RNases in the intestine.Citation23 Optimisation of the physicochemical properties of poly(I:C) has led to the generation of derivatives with greater stability or lower toxicity, such as polyI:CLC, polyI:C12U (Ampligen) and, more recently, RGC100.Citation49 Ampligen and RGC100 have the advantage of being exclusively TLR3 ligands, but their ability to stimulate intestinal responses following oral administration has yet to be investigated.
Many studies have also aimed to increase the stability of poly(I:C) and to decrease the inherent danger of associated systemic overstimulation, through the use of different formulations to facilitate locally restricted administration. For example, encapsulation in microspheres protects poly(I:C) from degradation by ribonucleases and increases the antigen-specific CTL response in the context of OVA immunisation.Citation48 Moreover, formulations of poly(I:C) with nanoparticles, such polysaccharide nanoparticles and triple-shell calcium phosphate nanoparticles, have been described as promising approaches for increasing the adjuvant properties of poly(I:C).Citation48 Whatever the strategy used to increase the efficacy of poly(I:C), scientists often consider DCs to be a key target and try to minimise the activation of non-hematopoietic cells. Recently, bacterial dsRNA of commensal but not pathogenic bacteria was described to be an effective TLR3 ligand that was beneficial as natural boosters of gut protective immunity by inducing type I IFN production by DCs.Citation50 Lactic acid bacteria resist digestion in the gut and have a spheroid or rod shape facilitating phagocytosis by DCs. These natural bacteria may, therefore, act as natural stimulators of TLR3 for the targeting of intestinal DCs, with properties similar to those of poly(I:C) but without the disadvantage of degradation following oral administration.
In summary, the stimulation of intestinal TLR3 with poly(I:C) activates intestinal DCs and protects neonates against enteric infection with the protozoan parasite C. parvum.Citation23 Co-operation between TLR3-TRIF activation by poly(I:C) and TLR5-MyD88 signals provided by the commensal flora is required for the induction of a protective immune response, with the production of IL-12p40, IFN-β and IFN-γ (). These data highlight the importance of the gut microbiota for the development of an adequate immune response and the key role of the TLR3 and TLR5 expressed by DCs of the lamina propria. Poly(I:C), if stabilized and included in vectors for intestinal delivery, could therefore potentially be used as an immunostimulant for controlling neonatal intestinal diseases, such as cryptosporidiosis.
Figure 3. The gut flora is required for the control of intestinal infection by poly(I:C) administration in neonates. After poly(I:C) administration, a rapid reduction in parasite burden was observed and proved to be dependent on TLR3/TRIF signaling and CD11c+ cells. Protection against C. parvum required additional signals provided by the gut flora through TLR5 and MyD88 signaling. This cooperation gave rise to higher levels of expression of type I IFN and IL12p40, and the production of IFNγ, a cytokine that plays a key role in the elimination of infected enterocytes.
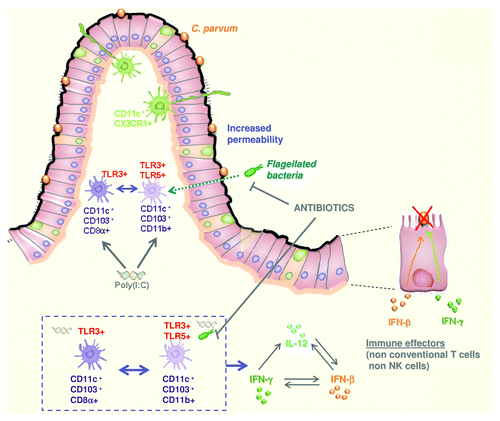
Disclosure of Potential Conflicts of Interest
No potential conflicts of interest were disclosed.
References
- Striepen B. Parasitic infections: Time to tackle cryptosporidiosis. Nature 2013; 503:189 - 91; http://dx.doi.org/10.1038/503189a; PMID: 24236315
- Bouzid M, Hunter PR, Chalmers RM, Tyler KM. Cryptosporidium pathogenicity and virulence. Clin Microbiol Rev 2013; 26:115 - 34; http://dx.doi.org/10.1128/CMR.00076-12; PMID: 23297262
- Lacroix-Lamandé S, Mancassola R, Naciri M, Laurent F. Role of gamma interferon in chemokine expression in the ileum of mice and in a murine intestinal epithelial cell line after Cryptosporidium parvum infection. Infect Immun 2002; 70:2090 - 9; http://dx.doi.org/10.1128/IAI.70.4.2090-2099.2002; PMID: 11895975
- Auray G, Lacroix-Lamandé S, Mancassola R, Dimier-Poisson I, Laurent F. Involvement of intestinal epithelial cells in dendritic cell recruitment during C. parvum infection. Microbes Infect 2007; 9:574 - 82; http://dx.doi.org/10.1016/j.micinf.2007.01.026; PMID: 17395519
- Lantier L, Lacroix-Lamandé S, Potiron L, Metton C, Drouet F, Guesdon W, Gnahoui-David A, Le Vern Y, Deriaud E, Fenis A, et al. Intestinal CD103+ dendritic cells are key players in the innate immune control of Cryptosporidium parvum infection in neonatal mice. PLoS Pathog 2013; 9:e1003801; http://dx.doi.org/10.1371/journal.ppat.1003801; PMID: 24367259
- Cerf-Bensussan N, Gaboriau-Routhiau V. The immune system and the gut microbiota: friends or foes?. Nat Rev Immunol 2010; 10:735 - 44; http://dx.doi.org/10.1038/nri2850; PMID: 20865020
- Ferret-Bernard S, Lacroix-Lamandé S, Remot A, Metton C, Bernardet N, Charley B, Drouet F, Laurent F. Mesenteric lymph node cells from neonates present a prominent IL-12 response to CpG oligodeoxynucleotide via an IL-15 feedback loop of amplification. Vet Res 2011; 42:19; http://dx.doi.org/10.1186/1297-9716-42-19; PMID: 21314903
- Tourais-Esteves I, Bernardet N, Lacroix-Lamandé S, Ferret-Bernard S, Laurent F. Neonatal goats display a stronger TH1-type cytokine response to TLR ligands than adults. Dev Comp Immunol 2008; 32:1231 - 41; http://dx.doi.org/10.1016/j.dci.2008.03.011; PMID: 18514862
- Auray G, Facci MR, van Kessel J, Buchanan R, Babiuk LA, Gerdts V. Porcine neonatal blood dendritic cells, but not monocytes, are more responsive to TLRs stimulation than their adult counterparts. PLoS One 2013; 8:e59629; http://dx.doi.org/10.1371/journal.pone.0059629; PMID: 23667422
- Zhao J, Kim KD, Yang X, Auh S, Fu YX, Tang H. Hyper innate responses in neonates lead to increased morbidity and mortality after infection. Proc Natl Acad Sci U S A 2008; 105:7528 - 33; http://dx.doi.org/10.1073/pnas.0800152105; PMID: 18490660
- Lacroix-Lamandé S, Rochereau N, Mancassola R, Barrier M, Clauzon A, Laurent F. Neonate intestinal immune response to CpG oligodeoxynucleotide stimulation. PLoS One 2009; 4:e8291; http://dx.doi.org/10.1371/journal.pone.0008291; PMID: 20011519
- Barrier M, Lacroix-Lamandé S, Mancassola R, Auray G, Bernardet N, Chaussé AM, Uematsu S, Akira S, Laurent F. Oral and intraperitoneal administration of phosphorothioate oligodeoxynucleotides leads to control of Cryptosporidium parvum infection in neonatal mice. J Infect Dis 2006; 193:1400 - 7; http://dx.doi.org/10.1086/503748; PMID: 16619188
- Adkins B. Neonatal immunology: responses to pathogenic microorganisms and epigenetics reveal an “immunodiverse” developmental state. Immunol Res 2013; 57:246 - 57; http://dx.doi.org/10.1007/s12026-013-8439-2; PMID: 24214026
- Sun CM, Deriaud E, Leclerc C, Lo-Man R. Upon TLR9 signaling, CD5+ B cells control the IL-12-dependent Th1-priming capacity of neonatal DCs. Immunity 2005; 22:467 - 77; http://dx.doi.org/10.1016/j.immuni.2005.02.008; PMID: 15845451
- Elahi S, Ertelt JM, Kinder JM, Jiang TT, Zhang X, Xin L, Chaturvedi V, Strong BS, Qualls JE, Steinbrecher KA, et al. Immunosuppressive CD71+ erythroid cells compromise neonatal host defence against infection. Nature 2013; 504:158 - 62; http://dx.doi.org/10.1038/nature12675; PMID: 24196717
- Pedras-Vasconcelos JA, Goucher D, Puig M, Tonelli LH, Wang V, Ito S, Verthelyi D. CpG oligodeoxynucleotides protect newborn mice from a lethal challenge with the neurotropic Tacaribe arenavirus. J Immunol 2006; 176:4940 - 9; http://dx.doi.org/10.4049/jimmunol.176.8.4940; PMID: 16585590
- Yamaguchi Y, Harker JA, Wang B, Openshaw PJ, Tregoning JS, Culley FJ. Preexposure to CpG protects against the delayed effects of neonatal respiratory syncytial virus infection. J Virol 2012; 86:10456 - 61; http://dx.doi.org/10.1128/JVI.01082-12; PMID: 22811525
- Rhee SJ, Walker WA, Cherayil BJ. Developmentally regulated intestinal expression of IFN-gamma and its target genes and the age-specific response to enteric Salmonella infection. J Immunol 2005; 175:1127 - 36; http://dx.doi.org/10.4049/jimmunol.175.2.1127; PMID: 16002714
- Thatte A, DeWitte-Orr SJ, Lichty B, Mossman KL, Ashkar AA. A critical role for IL-15 in TLR-mediated innate antiviral immunity against genital HSV-2 infection. Immunol Cell Biol 2011; 89:663 - 9; http://dx.doi.org/10.1038/icb.2011.7; PMID: 21339766
- Sotolongo J, España C, Echeverry A, Siefker D, Altman N, Zaias J, Santaolalla R, Ruiz J, Schesser K, Adkins B, et al. Host innate recognition of an intestinal bacterial pathogen induces TRIF-dependent protective immunity. J Exp Med 2011; 208:2705 - 16; http://dx.doi.org/10.1084/jem.20110547; PMID: 22124111
- Ashkar AA, Yao XD, Gill N, Sajic D, Patrick AJ, Rosenthal KL. Toll-like receptor (TLR)-3, but not TLR4, agonist protects against genital herpes infection in the absence of inflammation seen with CpG DNA. J Infect Dis 2004; 190:1841 - 9; http://dx.doi.org/10.1086/425079; PMID: 15499542
- Ma Y, Ross AC. The anti-tetanus immune response of neonatal mice is augmented by retinoic acid combined with polyriboinosinic:polyribocytidylic acid. Proc Natl Acad Sci U S A 2005; 102:13556 - 61; http://dx.doi.org/10.1073/pnas.0506438102; PMID: 16157890
- Lantier L, Drouet F, Guesdon W, Mancassola R, Metton C, Lo-Man R, Werts C, Laurent F, Lacroix-Lamandé S. Poly(I:C)-induced protection of neonatal mice against intestinal Cryptosporidium parvum infection requires an additional TLR5 signal provided by the gut flora. J Infect Dis 2014; 209:457 - 67; http://dx.doi.org/10.1093/infdis/jit432; PMID: 24014881
- Datta SK, Redecke V, Prilliman KR, Takabayashi K, Corr M, Tallant T, DiDonato J, Dziarski R, Akira S, Schoenberger SP, et al. A subset of Toll-like receptor ligands induces cross-presentation by bone marrow-derived dendritic cells. J Immunol 2003; 170:4102 - 10; http://dx.doi.org/10.4049/jimmunol.170.8.4102; PMID: 12682240
- Longhi MP, Trumpfheller C, Idoyaga J, Caskey M, Matos I, Kluger C, Salazar AM, Colonna M, Steinman RM. Dendritic cells require a systemic type I interferon response to mature and induce CD4+ Th1 immunity with poly IC as adjuvant. J Exp Med 2009; 206:1589 - 602; http://dx.doi.org/10.1084/jem.20090247; PMID: 19564349
- Zhou R, Wei H, Sun R, Tian Z. Recognition of double-stranded RNA by TLR3 induces severe small intestinal injury in mice. J Immunol 2007; 178:4548 - 56; http://dx.doi.org/10.4049/jimmunol.178.7.4548; PMID: 17372013
- McAllister CS, Lakhdari O, Pineton de Chambrun G, Gareau MG, Broquet A, Lee GH, Shenouda S, Eckmann L, Kagnoff MF. TLR3, TRIF, and caspase 8 determine double-stranded RNA-induced epithelial cell death and survival in vivo. J Immunol 2013; 190:418 - 27; http://dx.doi.org/10.4049/jimmunol.1202756; PMID: 23209324
- Pott J, Stockinger S, Torow N, Smoczek A, Lindner C, McInerney G, Bäckhed F, Baumann U, Pabst O, Bleich A, et al. Age-dependent TLR3 expression of the intestinal epithelium contributes to rotavirus susceptibility. PLoS Pathog 2012; 8:e1002670; http://dx.doi.org/10.1371/journal.ppat.1002670; PMID: 22570612
- McCartney S, Vermi W, Gilfillan S, Cella M, Murphy TL, Schreiber RD, Murphy KM, Colonna M. Distinct and complementary functions of MDA5 and TLR3 in poly(I:C)-mediated activation of mouse NK cells. J Exp Med 2009; 206:2967 - 76; http://dx.doi.org/10.1084/jem.20091181; PMID: 19995959
- Fujimoto K, Karuppuchamy T, Takemura N, Shimohigoshi M, Machida T, Haseda Y, Aoshi T, Ishii KJ, Akira S, Uematsu S. A new subset of CD103+CD8alpha+ dendritic cells in the small intestine expresses TLR3, TLR7, and TLR9 and induces Th1 response and CTL activity. J Immunol 2011; 186:6287 - 95; http://dx.doi.org/10.4049/jimmunol.1004036; PMID: 21525388
- Pantel A, Teixeira A, Haddad E, Wood EG, Steinman RM, Longhi MP. Direct type I IFN but not MDA5/TLR3 activation of dendritic cells is required for maturation and metabolic shift to glycolysis after poly IC stimulation. PLoS Biol 2014; 12:e1001759; http://dx.doi.org/10.1371/journal.pbio.1001759; PMID: 24409099
- Pollok RC, Farthing MJ, Bajaj-Elliott M, Sanderson IR, McDonald V. Interferon gamma induces enterocyte resistance against infection by the intracellular pathogen Cryptosporidium parvum. Gastroenterology 2001; 120:99 - 107; http://dx.doi.org/10.1053/gast.2001.20907; PMID: 11208718
- Barakat FM, McDonald V, Foster GR, Tovey MG, Korbel DS. Cryptosporidium parvum infection rapidly induces a protective innate immune response involving type I interferon. J Infect Dis 2009; 200:1548 - 55; http://dx.doi.org/10.1086/644601; PMID: 19821721
- Yarovinsky F. Innate immunity to Toxoplasma gondii infection. Nat Rev Immunol 2014; 14:109 - 21; http://dx.doi.org/10.1038/nri3598; PMID: 24457485
- O’Hara SP, Bogert PS, Trussoni CE, Chen X, LaRusso NF. TLR4 promotes Cryptosporidium parvum clearance in a mouse model of biliary cryptosporidiosis. J Parasitol 2011; 97:813 - 21; http://dx.doi.org/10.1645/GE-2703.1; PMID: 21506806
- Chen XM, O’Hara SP, Nelson JB, Splinter PL, Small AJ, Tietz PS, Limper AH, LaRusso NF. Multiple TLRs are expressed in human cholangiocytes and mediate host epithelial defense responses to Cryptosporidium parvum via activation of NF-kappaB. J Immunol 2005; 175:7447 - 56; http://dx.doi.org/10.4049/jimmunol.175.11.7447; PMID: 16301652
- Hooper LV, Littman DR, Macpherson AJ. Interactions between the microbiota and the immune system. Science 2012; 336:1268 - 73; http://dx.doi.org/10.1126/science.1223490; PMID: 22674334
- Baba N, Samson S, Bourdet-Sicard R, Rubio M, Sarfati M. Selected commensal-related bacteria and Toll-like receptor 3 agonist combinatorial codes synergistically induce interleukin-12 production by dendritic cells to trigger a T helper type 1 polarizing programme. Immunology 2009; 128:Suppl e523 - 31; http://dx.doi.org/10.1111/j.1365-2567.2008.03022.x; PMID: 19740313
- Lozupone CA, Stombaugh JI, Gordon JI, Jansson JK, Knight R. Diversity, stability and resilience of the human gut microbiota. Nature 2012; 489:220 - 30; http://dx.doi.org/10.1038/nature11550; PMID: 22972295
- Uematsu S, Jang MH, Chevrier N, Guo Z, Kumagai Y, Yamamoto M, Kato H, Sougawa N, Matsui H, Kuwata H, et al. Detection of pathogenic intestinal bacteria by Toll-like receptor 5 on intestinal CD11c+ lamina propria cells. Nat Immunol 2006; 7:868 - 74; http://dx.doi.org/10.1038/ni1362; PMID: 16829963
- Rumbo M, Nempont C, Kraehenbuhl JP, Sirard JC. Mucosal interplay among commensal and pathogenic bacteria: lessons from flagellin and Toll-like receptor 5. FEBS Lett 2006; 580:2976 - 84; http://dx.doi.org/10.1016/j.febslet.2006.04.036; PMID: 16650409
- Kinnebrew MA, Buffie CG, Diehl GE, Zenewicz LA, Leiner I, Hohl TM, Flavell RA, Littman DR, Pamer EG. Interleukin 23 production by intestinal CD103(+)CD11b(+) dendritic cells in response to bacterial flagellin enhances mucosal innate immune defense. Immunity 2012; 36:276 - 87; http://dx.doi.org/10.1016/j.immuni.2011.12.011; PMID: 22306017
- Stecher B, Robbiani R, Walker AW, Westendorf AM, Barthel M, Kremer M, Chaffron S, Macpherson AJ, Buer J, Parkhill J, et al. Salmonella enterica serovar typhimurium exploits inflammation to compete with the intestinal microbiota. PLoS Biol 2007; 5:2177 - 89; http://dx.doi.org/10.1371/journal.pbio.0050244; PMID: 17760501
- Lupp C, Robertson ML, Wickham ME, Sekirov I, Champion OL, Gaynor EC, Finlay BB. Host-mediated inflammation disrupts the intestinal microbiota and promotes the overgrowth of Enterobacteriaceae. Cell Host Microbe 2007; 2:204; http://dx.doi.org/10.1016/j.chom.2007.08.002; PMID: 18030708
- Tukhvatulin AI, Gitlin II, Shcheblyakov DV, Artemicheva NM, Burdelya LG, Shmarov MM, Naroditsky BS, Gudkov AV, Gintsburg AL, Logunov DY. Combined stimulation of Toll-like receptor 5 and NOD1 strongly potentiates activity of NF-κB, resulting in enhanced innate immune reactions and resistance to Salmonella enterica serovar Typhimurium infection. Infect Immun 2013; 81:3855 - 64; http://dx.doi.org/10.1128/IAI.00525-13; PMID: 23897616
- Krummen M, Balkow S, Shen L, Heinz S, Loquai C, Probst HC, Grabbe S. Release of IL-12 by dendritic cells activated by TLR ligation is dependent on MyD88 signaling, whereas TRIF signaling is indispensable for TLR synergy. J Leukoc Biol 2010; 88:189 - 99; PMID: 20360404
- Zhou R, Wei H, Sun R, Zhang J, Tian Z. NKG2D recognition mediates Toll-like receptor 3 signaling-induced breakdown of epithelial homeostasis in the small intestines of mice. Proc Natl Acad Sci U S A 2007; 104:7512 - 5; http://dx.doi.org/10.1073/pnas.0700822104; PMID: 17463084
- Hafner AM, Corthésy B, Merkle HP. Particulate formulations for the delivery of poly(I:C) as vaccine adjuvant. Adv Drug Deliv Rev 2013; 65:1386 - 99; http://dx.doi.org/10.1016/j.addr.2013.05.013; PMID: 23751781
- Naumann K, Wehner R, Schwarze A, Petzold C, Schmitz M, Rohayem J. Activation of dendritic cells by the novel Toll-like receptor 3 agonist RGC100. Clin Dev Immunol 2013; 2013:283649; http://dx.doi.org/10.1155/2013/283649; PMID: 24454470
- Kawashima T, Kosaka A, Yan H, Guo Z, Uchiyama R, Fukui R, Kaneko D, Kumagai Y, You DJ, Carreras J, et al. Double-stranded RNA of intestinal commensal but not pathogenic bacteria triggers production of protective interferon-β. Immunity 2013; 38:1187 - 97; http://dx.doi.org/10.1016/j.immuni.2013.02.024; PMID: 23791646