Abstract
We have previously shown that IsdB, a conserved protein expressed by Staphylococcus aureus, induces a robust antibody response which correlates with protection in a murine challenge model. Here we investigate the role of cellular immunity in IsdB mediated protection using lymphocyte deficient SCID mice. As opposed to WT CB-17 mice the CB-17 SCID mice were not protected against a lethal challenge of S. aureus after active and passive immunizations with IsdB. Adoptive transfer of in vitro isolated lymphocyte subsets revealed that reconstituting mice with IsdB specific CD3+ or CD4+ T-cells conferred antigen specific protection while CD8+ T-cells, CD19+ B-cells and plasma cells (CD138highB220intCD19lo) alone were not protective. A combination of CD3+ T-cells plus CD19+ B-cells conferred protection in CB-17 SCID mice, whereas bovine serum albumin (BSA) immune lymphocytes did not confer protection. Active immunization experiments indicated that IsdB immunized Jh mice (B-cell deficient) were protected against lethal challenge, while nude (T-cell deficient) mice were not. In vitro assays indicated that isolated IsdB specific splenocytes from immunized mice produced abundant IL-17A, much less IFN-γ and no detectable IL-4. IL-23 deficient mice were not protected from a lethal challenge by IsdB vaccination, pointing to a critical role for CD4+ Th17 in IsdB-mediated vaccination. Neutralizing IL-17A, but not IL-22 in vivo significantly increased mortality in IsdB immunized mice; whereas, neutralizing IFN-γ did not alter IsdB-mediated protection. These findings suggest that IL-17A producing Th17 cells play an essential role in IsdB vaccine-mediated defense against invasive S. aureus infection in mice.
Introduction
The need for a vaccine to prevent invasive disease caused by S. aureus has become an important public health concern, increasing in urgency over the last decades.Citation1-Citation3 Development of a vaccine often depends on gaining an understanding of the immune response to an organism, which can then be enhanced through rational vaccine design. Although extensively investigated, natural protective immunity to S. aureus is still poorly understood. Acute infection with S. aureus does not prevent re-infection.Citation1 Preclinical and clinical data indicate that immunization with intact whole bacteria induces high immune titers to staphylococcus, but does not confer protection from S. aureus disease.Citation1,Citation4 Clearance of S. aureus is historically thought to be dependent upon antibody (Ab) and complement mediated uptake and killing by phagocytes,Citation5-Citation10 with neutrophils being essential to resolution of disease.Citation11,Citation12 However it has been demonstrated that S. aureus can in fact survive within neutrophils, leading to exacerbated disease.Citation11,Citation13 Additionally, the S. aureus stimulated humoral immune response may not play a meaningful role in bacterial clearance in some models.Citation14-Citation17 While antibodies undoubtedly play some role in protection, they may not be determinative for vaccine protective efficacy because animals and humans already have sufficient baseline opsonins to allow for phagocytic uptake by neutrophils.
Recently, the importance of T-cells and T-cell cytokines in innate immunity to S. aureus has been the object of investigation.Citation14,Citation18-Citation20 T cells were observed to play a pivotal role in the prevention of S. aureus infection, as demonstrated in models of disseminated as well as local infection. These studies established a connection between T cells and neutrophils wherein IL-17A is important for recruiting neutrophils, enhancing chemotaxis, acting synergistically with TLR2 to enhance killing (non-antibody, i.e., pattern recognition), and priming neutrophils for bactericidal activity.Citation21,Citation22 In addition, Th17 immunity may be important for addressing S. aureus as an intracellular pathogenCitation23 and IL-17A induced Th1 helper response may be of particular importance in addressing such organisms.Citation24 Hence we wanted to define the role of T-cell immunity in response to our vaccine antigen.
IsdB is a ~72 kDa antigen expressed on the cell surface of S. aureus in iron limited environments.Citation25-Citation27 Its function is to capture and import heme iron from hemoglobin.Citation27 Due to the low iron environment of mammalian blood and tissue, IsdB is upregulated during pathogenesis in vivo.Citation28 IsdB is highly conserved among diverse S. aureus clinical isolates, both methicillin resistant and methicillin sensitive and humans, as well as mammals examined to date, have pre-existing antibody titers to IsdB,Citation29 although it is unknown whether these pre-existing titers offer protection. Additionally, Kuklin et al. demonstrated that immunization with IsdB formulated on amorphous aluminum hydroxyphosphate sulfate adjuvant (AAHSA) increased murine antibody titers by up to 20-fold, and non-human primate titers by 4-fold. Importantly, increased antibody titers correlated with enhanced survival in a murine disseminated challenge model.Citation30 Also, mAb to IsdB have in vitro opsonophagocytic (OP) activity, and efficacy in rodent challenge models.Citation28 Thus IsdB-specific mAb can confer protection after passive immunization, however it was of interest to determine what components of the adaptive immune system are important for protection mediated by active IsdB vaccination. To this end, a series of experiments were undertaken to dissect the immune response to IsdB in the murine lethal challenge model. Lymphocyte populations were evaluated for protective activity, and it was discovered that protection in this model is mediated by CD4+ T cells, and that IL-17A plays an important role in vaccine efficacy.
Results
Lymphocytes are essential for IsdB mediated protection in a murine sepsis model
IsdB was previously shown to elicit antibody responses which correlated with protection in a murine sepsis model. However, it is possible that the antigen also elicits an effector T-cell response which may contribute to enhanced survival. To investigate the role of cell mediated immune responses during IsdB induced protection, CB-17 (WT or SCID) mice were immunized with IsdB or BSA (negative control), via intramuscular injection to the thigh, as described in the Methods section. Mice were challenged with S. aureus and survival was monitored for 10 d.
Since survival in this disseminated infection model correlates with antibody titer,Citation30 it was expected that lymphocyte (and antibody) deficient SCID mice would be susceptible to challenge. As shown in , IsdB immunized CB-17 WT mice, with an intact lymphocyte population, showed significantly higher (p = 0.02) survival than IsdB immunized CB-17 SCID mice, or BSA vaccinated control mice (p = 0.02) (data not shown). It was expected that if the SCID mice were supplemented with IsdB specific mAb, they would be protected from challenge. Monoclonal antibody CS-D7 was used to passively immunize SCID mice. This monoclonal has been previously demonstrated to significantly enhance survival in the murine sepsis model.Citation31 Surprisingly, SCID mice treated with CS-D7 were not protected post challenge and showed similar reduced survival as animals treated with the isotype control (MK-24), or with animals uninjected with mAb (naïve mice) (). This experiment was performed using the Becker strainCitation28 as well as the SA025Citation31 strain as challenge inoculum, with equivalent results. Thus using either method of immunization, it was observed that an intact lymphocyte system was required for protection post IsdB immunization in the murine sepsis model of infection.
Figure 1. Survival of CB-17 (WT or SCID) mice immunized (active or passive) with IsdB. (A)WT or SCID mice (n = 10) were actively immunized i.m. with 20 µg of antigen on days 0, 7 and 21. On day 35, mice were challenged with S. aureus Becker (8.8 × 108 CFU) via the tail vein. Mice were monitored for survival for 10 d post challenge. Data were pooled from two independent experiments. Survival of IsdB immunized SCID mice vs. IsdB immunized WT mice, p = 0.02. Survival of IsdB immunized WT mice vs. control BSA immunized WT mice, p = 0.02 (data not shown). (B) Survival of CB-17 (SCID) mice passively immunized to IsdB and challenged via the tail vein. SCID mice (n = 10) were immunized i.p. with 400 µg of mAb (either IsdB specific CS-D7, or control MK24), or saline alone, 2 h prior to challenge with S. aureus SA025 (2 × 108 CFU) via the tail vein. Mice were monitored for survival for 10 d post challenge. Data were pooled from two independent experiments. Survival of IsdB immunized SCID mice vs. isotype immunized mice, p = 0.49. Error bars indicate the 95% CI.
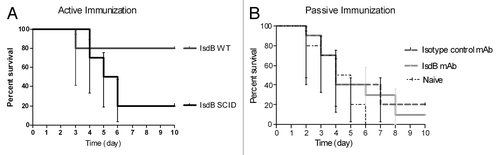
Identification of lymphocyte subset(s) critical in protection
Lymphocyte subset(s) were examined to determine which populations were critical in providing protection mediated by IsdB. Splenocytes harvested from IsdB or BSA immunized CB-17 WT mice were isolated into five major subsets: CD19+, CD138highB220intCD19lo, CD3+, CD4+, and CD8+, using MACS beads. These were adoptively transferred into CB-17 SCID mice via tail vein injection. Purity of the isolated populations was confirmed using flow cytometry (data not shown), and each population was > 95% homogeneous. Mice were challenged via the tail vein and monitored for survival for 10 d post challenge.
As shown in , CB-17 SCID mice reconstituted with a combination of CD19+ plus CD3+ lymphocytes (group #3) from animals immunized with IsdB were robustly protected, as compared with mice reconstituted with CD19+ plus CD3+ lymphocytes from BSA immunized animals (p < 0.01). Individual CD19+ (group #2) and CD3+ (group #4) lymphocytes were examined for protective activity. Surprisingly, reconstitution with IsdB immune CD19+ B lymphocytes alone did not generate significant protection (p = 0.54) vs. the control CD19+ cells. In case these lymphocytes were not producing sufficient antigen specific IgG, immune CD138highB220intCD19lo plasmacytes (group #1) were isolated and adoptively transferred. Contrary to our expectations, transfer of the IsdB immunized CD138highB220intCD19lo plasmacyte subset did not reconstitute statistically significant protection (p = 0.2) in the recipient mice. Thus neither IsdB immunized B cells (CD19+) nor plasmacytes (CD138highB220intCD19lo) were protective. However, adoptive transfer of CD3+ T cells (group #4) was found to be significantly protective (p < 0.01) over control. Two subsets of T cells were isolated and transferred, CD4+ (group #5) and CD8+ (group #6). No enhancement in survival was noted with the transfer of CD8+ T cells alone (p = 0.6), thus suggesting that the CD4+ T subset (p = 0.06) in the CD3+ T cell population was responsible for enhanced survival of recipient mice. The adoptive transfer of lymphocyte subsets from control (BSA) mice was unable to reconstitute protective immunity in any instance.
Table 1. Survival of CB-17 SCID mice after adoptive transfer of isolated lymphocyte populations and challenge via the tail vein with S. aureus*
CD4+ mediated protection was antigen specific
The enhanced survival observed in SCID mice reconstituted with IsdB specific CD4+ cells was investigated to determine if the response was antigen specific. Although BSA immune lymphocytes did not confer protection (), it was possible that IsdB immune lymphocytes could have induced a non-specific stimulation of the innate immune system leading to enhanced survival against S. aureus challenge. CD4+ lymphocytes from IsdB immune CB-17 mice were adoptively transferred into SCID mice. The mice were challenged with a lethal dose of S. aureus Becker isdB/harA deletion strain (KO), or the WT parental strain. A double deletion strain was used because harA has high homology with isdB, and therefore both genes need to be deleted to ensure complete removal of this antigen.
As observed in the adoptive transfer experiments above, SCID mice replete with IsdB immune CD4+ lymphocytes were significantly protected after challenge with the S. aureus Becker WT strain, when compared with SCID mice replete with BSA immune CD4+ lymphocytes, p < 0.003 (). However, when similar sets of mice were challenged with the S. aureus Becker KO strain, the IsdB immune SCID mice did not exhibit greater survival (p = 0.26) than the BSA immune SCID mice. Therefore, the protection mediated by IsdB immune CD4+ lymphocytes was antigen (IsdB) specific.
Figure 2. Survival of CB-17 SCID mice replenished with IsdB or BSA immunized CD4+ T cells. CD4+ T cells from CB-17 mice immunized with either IsdB, or BSA were adoptively transferred into SCID mice (n = 10) via the i.v. route, and the mice were challenged with either WT S. aureus Becker, or KO Becker (isdB/harA−/−)(LD80–90; 4.9 × 108–8.7 × 108) via the tail vein. Mice were monitored for survival for 10 d post challenge. Survival of mice challenged with WT S. aureus Becker, p = 0.003 for IsdB immunized SCID mice vs. BSA immunized SCID mice. Survival of mice challenged with KO S. aureus Becker, p = 0.26 for IsdB immunized SCID mice vs. BSA immunized SCID mice. Error bars indicate the 95% CI values.
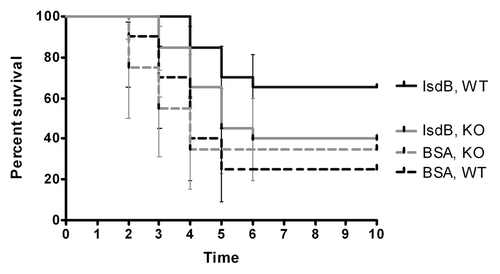
Lack of T cell immunity has fatal outcome while lack of B cell immunity does not
The inability of IsdB specific plasma and CD19+ B cells to confer protection in the CB-17 SCID adoptive transfer model prompted us to investigate the role of B and T cells in IsdB mediated protection, using an alternative strategy. Genetically altered immunoglobulin heavy chain deficient Jh (Balb/c background) and T cell deficient nude mice (Balb/c) were immunized with IsdB, or BSA using the same protocol as above. Mice were challenged with a lethal dose (LD80–90) of S. aureus Becker and survival was monitored for 10 d.
As shown in , IsdB immunized Jh (B cell deficient) mice were significantly protected after S. aureus challenge, compared with the BSA control Jh mice (p = 0.04) which were not protected. The control naive (unimmunized) Jh mice were also not protected. The IsdB immunized nude (T cell deficient) mice () were not protected after S. aureus challenge in comparison to the BSA immunized control nude mice (p = 0.88). These data were in agreement with our prior adoptive transfer experiments, indicating a critical role for T lymphocytes, but not B lymphocytes nor plasma cells, in mediating protection after immunization with IsdB, in the murine sepsis model. Titers to IsdB were measured for each group of mice. Jh mice had no IgG titer to IsdB () while nude mice had IsdB titers post immunization, although lower than the expected titer for WT mice ().
Figure 3. Survival of IsdB immunized B and T cell deficient mice challenged via the tail vein. IgH deficient Jh mice [(A) n = 10] or T-cell deficient nude mice [(B) n = 10] were actively immunized i.m. with IsdB or BSA and challenged with S. aureus Becker, (6.4 × 108 CFU, LD 80–90) via the tail vein, as described in the Methods section. Mice were monitored for survival for 10 d post challenge. IsdB immunized Jh mice survival compared with control BSA immunized Jh mice (p = 0.04). T-cell deficient nude mice immunized with IsdB survival compared with BSA immunized nude mice (p = 0.88). Jh (C) and nude (D) mice were bled prior to IsdB vaccination on day 0, and post vaccination on day 28, and titer of IsdB antibody response measured using an ELISA, as described in the Methods section.
![Figure 3. Survival of IsdB immunized B and T cell deficient mice challenged via the tail vein. IgH deficient Jh mice [(A) n = 10] or T-cell deficient nude mice [(B) n = 10] were actively immunized i.m. with IsdB or BSA and challenged with S. aureus Becker, (6.4 × 108 CFU, LD 80–90) via the tail vein, as described in the Methods section. Mice were monitored for survival for 10 d post challenge. IsdB immunized Jh mice survival compared with control BSA immunized Jh mice (p = 0.04). T-cell deficient nude mice immunized with IsdB survival compared with BSA immunized nude mice (p = 0.88). Jh (C) and nude (D) mice were bled prior to IsdB vaccination on day 0, and post vaccination on day 28, and titer of IsdB antibody response measured using an ELISA, as described in the Methods section.](/cms/asset/151e5414-a3bd-4e00-9fc5-9ea39d5ffdc2/khvi_a_10918946_f0003.gif)
IsdB Immunization primarily induced secretion of IL-17A but not IFN-g or IL-4
As observed in the adoptive transfer experiments, the CD4+ T cells were the cell population which conferred protection after challenge with S. aureus. To define the cytokine response induced by vaccination, spleens were harvested from IsdB and BSA immunized CB-17 WT mice two weeks post boost (day 28). The splenocytes were stimulated ex vivo for 20 h with IsdB peptide pools or IsdB intact protein (as described in the Methods section) and cytokine secretion by CD4+ T cells was measured by Elispot assay and ICS assay.
Intracellular cytokine staining (ICS) using intact IsdB protein revealed a significant increase in the frequency of IL-17A secreting CD4+ T cells post IsdB immunization ( and S1), as compared with BSA immunized control mice ( and S1). A minimal increase in IFNγ-secreting CD4+ T cells was also noted upon stimulation (). No response from IL4 secreting CD4+ T cells was observed. Additionally we also evaluated the secretion of IL-17A and IFN-g from immune splenocytes using IsdB peptide pools in an ELISpot assay (). It was observed that IsdB peptide pools 1 (a.a. 42–93) and 7 (a.a. 242–333), stimulated robust secretion of IL-17A from splenocytes (). Pools 1 (a.a.42–93), 10–11(a.a.402 to 457) stimulated IFN γ response, however, this was not as robust as the IL-17A response (). Alternatively, secretion of IL17A, IFN-γ and IL4 from splenocytes using IsdB peptide pools was also evaluated in an ICS assay. Similar to Elispot results, stimulation with pools 1, 6 and 7 resulted in robust IL-17A secretion from splenocytes (; Fig. S2) however we did not detect a robust IFN-γ response upon stimulation with the overlapping IsdB peptide pools using ICS technique (; Fig. S3).
Figure 4. ICS and ELISpot evaluation of IsdB immune splenocytes for cytokine secretion. Splenocytes were isolated from IsdB (A) or BSA (B) immune CB-17 mice and stimulated for 20 h in vitro with IsdB or BSA, or left unstimulated. IL17A, IFNγ and IL4 secreting CD4 T cells were identified by flow cytometry as described in the Methods section. Overlapping 15 mer peptide pools from IsdB were used to stimulate splenocytes from IsdB immune CB-17 mice. Splenocytes were subsequently analysis by ELISpot for the production of IL17A (C) or IFNγ (D) release. ICS was used to confirm ELISpot results by evaluating which IsdB peptide pools stimulated intracellular secretion of either IL17A (E) or IFNγ (F).
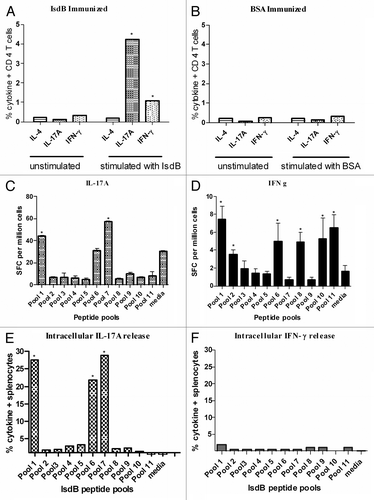
IsdB does not mediate protection in p19KO mice
Based upon the data above, it appeared that IsdB mediated immunity induced a robust Th17 (IL-17A), as opposed to a Th1 (IFN-γ), or Th2 (IL4) response in the CB-17 mice. It was therefore possible that stimulation of the Th17 response contributed to protection in mice in the sepsis model. To investigate this possibility, IL-23p19 KO mice (C57Bl/6 background) were utilized. Genetic deletion of the IL-23p19 subunit prevents production of the cytokine IL-23, and thus the formation of IL-17 and IL 22-secreting CD4+ T-cells.Citation21,Citation32 IL-23p19 KO mice, or the parental WT C57Bl/6 were immunized with either IsdB or BSA as previously described, and challenged with S. aureus.
As indicated in , IL-23p19 KO mice immunized with IsdB had a similar survival rate as mice immunized with BSA (p = 0.77). Although both IsdB and BSA immunized groups of mice had a higher survival rate than the naïve, unimmunized mice, there was no difference in survival between the two immunized groups.
Figure 5. Survival of p19KO mice immunized with IsdB or BSA and challenged via the tail vein. p19KO mice (n = 10) were immunized i.m. with 20 µg of antigen on days 0, 7 and 21. On day 35 mice were challenged with S. aureus Becker (8 × 108 CFU) via the tail vein. Mice were monitored for survival for 10 d post challenge. Survival of IsdB immunized p19KO mice vs. BSA immunized p19KO mice, p = 0.77. Error bars = 95% CI values.
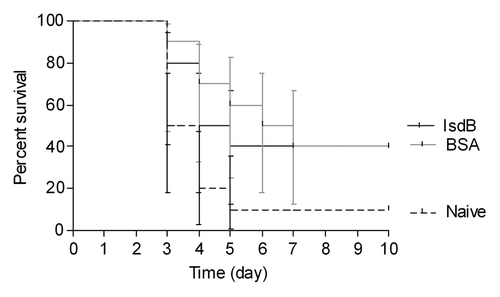
Protection in mice is mediated by IL-17A and not IFN-γ
Results from the IL-23p19 KO mouse strain supported the possibility of Th17 cells contributing to the IsdB vaccine-mediated protection of Balb/c mice in the sepsis model. If this were so, then IL-17A could be an important cytokine involved in, or mediating, the IsdB vaccine protection.Citation33,Citation34 Since IL23p19KO mice are also deficient in IL22 producing CD4T cells, the role of both IL17A and IL22 in protection was evaluated using neutralizing mAbs to each of these cytokines (; Fig. S4). IsdB immunized Balb/c were administered saline, or 400 µg of either IL-17A, IFN-γ, IL22 neutralizing mAb, or isotype control mAb per mouse, through the i.p. route. Two hours post administration of saline or mAb, mice were challenged through the tail vein and survival was monitored for 10 d.
Figure 6. Survival of Balb/c mice immunized with IsdB (A) or BSA (B) and challenged in the presence of neutralizing mAbs. Balb/c mice (n = 10) were immunized i.m. with 20 µg of antigen on days 0, 7 and 21. On day 35 mice were injected ip with 400 µg of neutralizing IL17A, IFN-γ or isotype control mAb, or an equal volume of saline. After 2 h, mice were then challenged with S. aureus Becker (7.8 × 108 CFU) via the tail vein. Mice were monitored for survival for 10 d post challenge. Data shown is from a single experiment repeated two times with equivalent results. (A) Survival of IsdB immunized mice injected with IL17A mAb vs. isotype control mAb, p = 0.004; survival of IsdB immunized mice injected with IFNγ mAb vs. isotype control mAb, p = 0.48. (B) Survival of BSA immunized mice injected with IL17A mAb vs. isotype control mAb, p = 0.17; survival of BSA immunized mice injected with IFN-γ mAb vs. isotype control mAb, p = 0.18. Error bars = 95% CI.
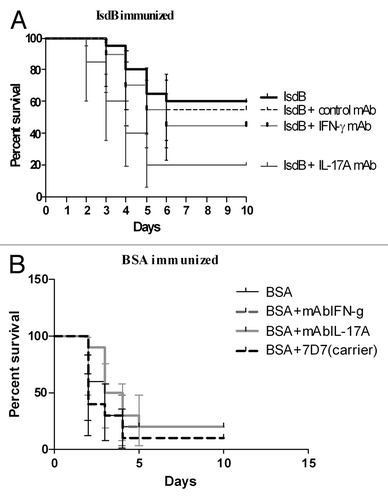
When IsdB immunized Balb/c mice were injected with saline (IsdB), or were injected with isotype control mAb, prior to S. aureus challenge, they had equivalent survival in this model (60% and 55% respectively) (). IsdB-immunized mice administered neutralizing antibody to IL-17A had a significant decrease in survival, when compared with IsdB-immunized mice administered isotype control mAb (p = 0.004). IsdB immunized mice administered IL-17A neutralizing mAb had the same survival rate as for negative control BSA immunized mice (20%). Administering IFN γ neutralizing mAb to IsdB immune mice resulted in a somewhat lower survival than for isotype control mAb injected mice, however, the difference was not significant (p = 0.48). BSA immune mice served as the negative control for this experiment. As shown in , there was no significant difference in survival in BSA immunized mice with saline nor any antibody injected (p = 0.05). Additionally, we found that IL22 does not play a major role in this model, as there was no significant drop in survival of IsdB immunized mice, challenged with a lethal S. aureus dose, comparing the IL22 neutralizing mAb to an isotype control mAb (p = 0.59, Fig. S4) This experiment indicates that there is a direct link between IsdB immunization, IL-17A production, and efficacy in the murine model of lethal sepsis.
Discussion
The humoral immune response to S. aureus is believed to be important for protection from infection, and as such, has been extensively investigated.Citation35-Citation39 However this response may not necessarily contribute to clearance of bacteria or reduction of disease.Citation15,Citation40 Evidence is mounting that antibody plays a limited role in the clearance of multiple bacterial pathogens. For example, the clearance of Streptococcus pneumoniae colonization may not depend on the humoral immune response,Citation41-Citation43 and antibodies are not important in the clearance of Helicobacter pylori in a murine challenge model.Citation44,Citation45 Investigation of human genetic linked disease has implicated a role for CD4+ Th17 cells in the natural clearance of S. aureus. Patients with hyperimmunoglobulin E syndrome (HIES), or Job syndrome, suffer from repeated S. aureus and C. albicans infections of the skin and mucosal surfaces.Citation46 Recently mutations in the signal transducer and activator of transcription 3 (STAT3) intracellular regulator were described in these patients, and the resulting defective STAT3 was linked to dramatically decreased or missing IL-17A-producing CD4+ cells (i.e., Th17 cells).Citation47-Citation49 A significant decrease in IL-17A was observed in these patients, which could account for the lack of protection against repeated S. aureus and C. albicans infections.Citation22 Th17 cells are thought to mediate protection from intracellular bacteria, extracellular bacteria and fungal infectionsCitation21,Citation32,Citation50,Citation51 particularly at mucosal surfaces.Citation22 IL-17A secreted by Th17 cells stimulates release of chemokines and granulocyte colony stimulating factors, thus recruiting neutrophils to sites of pathogen invasion.Citation50 Although IL-17A reduction was linked to the repeated S. aureus and C. albicans infections in Job syndrome patients, autoantibodies to IL-17A and IL-17F, as observed in autoimmune polyendocrine syndrome type I (APS-1) patients do not lead to S. aureus infections. These patients exhibit repeated C. albicans infections, but no unusual level of infections with other pathogens.Citation52 Perhaps the difference between the two syndromes resides with the intact Th17 activity in the APS-1 patients, which might provide some activation of mucosal cells and keratinocyte cationic peptide defenses and thus provide some residual immunity for pathogens like S. aureus at these sites. In any event, the connection between STAT3, Th17, IL-17A and S. aureus immunity is a complex and evolving story.Citation53
Mice models have been used to investigate the role of Th17 in natural immunity to S. aureus. In the absence of both IL-17A and IL-17F in a cytokine deletion murine strain (Il17a−/−IL17f−/−),Citation54 formation of spontaneous mucocutaneous abscesses was observed around the nose and mouth. These abscesses contained S. aureus, indicating the involvement of IL-17A/F cytokines in the natural control of S. aureus infections. However after active iv challenge with S. aureus, no difference was observed in survival, or kidney bacterial burden, between the Il17a−/−IL17f−/− strain and the wild type progenitor strain. Therefore, IL-17A/F were not critical immune modulators during disseminated S. aureus infection, but only for local mucocutaneous infection.Citation54 It is important to note that the mice in these experiments were un-immunized. The role of IL-17A in mucocutaneous protection from S. aureus was confirmed in work from a second group.Citation55 These investigators demonstrated that mice deficient in CD3+ γδT cells exhibited cutaneous lesions significantly larger than observed in wild type mice. Reconstituting the mice with a single dose of IL-17A could restore the impaired immunity of the deletion strain back to that of the wild type strain.
IsdB has significant vaccine activity when administered to mice, and IsdB specific antibodies generated by active immunization can confer protection.Citation28,Citation30,Citation56 In light of the expanding evidence supporting the importance of T cells in immunity to bacteria, we initiated experiments to explore the role of lymphocytes in IsdB mediated protection. To this end, lymphocyte populations were investigated to determine which population(s) conferred protection. In the absence of any lymphocytes (in SCID mice), no protection was observed after IsdB immunization, which was not unexpected, because these mice are defective for IgG production. However, protection could not be reconstituted by passive immunization with IsdB specific mAb in the absence of lymphocytes. The dependence of mAb mediated protection on having an intact lymphocyte system may be explained by the fact that Abs promote a more effective inflammatory response in the presence of T cells. Infact there are several other instances in which passive mAb efficacy requires an intact immune system.Citation57,Citation58 Thus, lymphocytes played a critical role in protection mediated by anti-IsdB mAb in our model. The identity of the critical population of lymphocytes was investigated through passive transfer of isolated lymphocyte sub-populations into SCID mice. The active cells were determined to be T cells (CD3+/CD4+), whereas B lineage cells (CD19+ or CD138hB22+/−CD19/-) provided little or no protection. This was an unexpected finding, and was confirmed through active vaccination and challenge of B-cell deficient, and T-cell deficient mice. B-cell deficient immunized mice were protected from challenge, whereas T-cell deficient mice were not. These data suggest that IgG and B-cells do not play a primary role in protection mediated by IsdB. We have previously shown that increasing antibody titers after vaccination with IsdB correlate with protection in a murine model of disseminated staph infection.Citation30 However a side by side evaluation of T-cell responses was not done in those experiments. In light of these data, it is likely that the rising antibody titers were a consequence of a robust CD4 T helper cell response. In fact, antibody titers have been used as surrogate markers of protectionCitation59 and shown to be elevated during polarized T helper responses.Citation60 Thus, IsdB vaccine efficacy in this model of disseminated infection was in fact mediated by IL-17A-secreting CD4 T lymphocytes. Additionally, protection mediated by T cells was antigen specific, as demonstrated in SCID mice passively immunized with IsdB specific CD4+ T cells and challenged with the S. aureus isdB-/harA- deletion strain. In that case, mice were not protected against challenge. These data confirm that T cell mediated protection was not due to non-specific immune stimulation stimulated by IsdB immune T cells. This experimental result is thus consistent with earlier findings in which Balb/c mice were actively vaccinated with IsdB and were not protected after challenge by the S. aureus isdB-/harA- deletion strain.Citation30
CD4+ T cells were identified as the effectors for IsdB mediated protection, in the adoptive transfer experiments. These cells were examined for the expression of cytokines to determine which T-helper population was likely mediating protection. Analysis of IsdB immune CD4+ cells revealed minimal or no increase in the frequencies of IFNγ (Th1) and IL-4 (Th2) secreting CD4 T cells upon stimulation with IsdB, whereas IL-17A (Th17) secreting cells made upto 4% of the CD4+ population. These results were confirmed using ELISpot and ICS analysis. To confirm that Th17 cells were important in IsdB mediated efficacy, mice deficient in IL-23 (IL-23p19 KO) were immunized and challenged with S. aureus. These mice cannot produce functional IL-23, which is critical in differentiation of Th17 cells.Citation32 Consequently, protection was not observed in this strain of mice. Additionally, anti-IL-17A mAb eliminated IsdB mediated protection in Balb/c mice (p = 004), whereas anti-INFγ mAb had much less effect (p = 0.48). Neither mAb affected survival in BSA immunized mice (p = 0.17 or p = 0.18 respectively). Since IL23p19KO mice are also deficient in the production of IL22, mice were also evaluated for survival post challenge by neutralizing only IL22, but not IL17A, in vivo. Here, upon challenge with S. aureus we found no difference in the survival of mice treated with anti IL22 mAb or not treated with the (IL 22) mAb (p = 0.59).
Taking the data in total, the protection using the IsdB vaccine in this disseminated lethal challenge model is mediated through a Th17 response, in which IL-17A plays an important role. Neither immune B cells nor immunoglobulin support efficacy. Other vaccine antigens that have been reported to enhance efficacy against lethal S. aureus challenge, in a disseminated murine model or kidney infection model, through Th17 stimulation; are the Candida albicans adhesin Als3pCitation14,Citation19 and S. aureus fibrinogen binding clumping factor A (Clf-A).Citation58 Als3p antigen protects mice from both C. albicans and S. aureus challenge in the absence of B lymphocytes.Citation14 IsdB differs from Als3p in that antibodies to IsdB, when passively administered, can provide protection from a lethal S. aureus challenge.Citation28,Citation30,Citation56 Data elucidating the underlying protective murine immune responses to these antigens may shed light on the necessary mechanism of adaptive immune response to induce protection to S. aureus in humans.Citation58,Citation61 There are several observations which imply that antibodies may not be sufficient for protection against S. aureus infection. For example, antibodies targeted to several S. aureus surface antigens have demonstrated preclinical efficacy, including polyclonal anti-capsule type 8 and type 5,Citation62-Citation64 polyclonal and monoclonal anti- ClfACitation65,Citation66 and Fab to an ABC transporter.Citation67 However, human clinical trials resulted in a disappointing lack of statistically significant efficacy.Citation68-Citation70 Taken together, the role of vaccine induced Th17 response should be evaluated as a biomarker for vaccine efficacy, along with antibody response, for future vaccine development. IsdB induces both humoral and cellular immune responses which may both contribute to efficacy.
Methods
Ethics statement
All animal work was performed in strict accordance with the recommendations in the Guide for care and use of Laboratory Animals of the National Institutes of Health. The protocol was approved by Institutional Animal Care and Use Committee (IACUC, APS# 10079975730248), Merck Research Labs, West Point, PA.
Bacterial strains: bacteria
Bacteria used in this investigation were as follows: S. aureus Becker-MSSA (obtained from Prof Chia Lee, University of Arkansas), S. aureus Becker isdB harA deletion mutant-MSSA, and SA025-MRSA (Merck clinical isolate).Citation31 Bacteria were grown on tryptic soy agar (TSA), or tryptic soy broth (TSB) overnight, pelleted and stored as frozen 15% glycerol stocks. For use in experiments, bacteria were thawed, pelleted, and resuspended in the appropriate buffer or medium. Bacterial CFU were quantitated by serial dilution and plating on TSA, with growth overnight at 37°C.
Murine strains
Mice were purchased from Taconic Farms Inc. CB-17 WT and CB-17 SCID mice were used for adoptive transfer studies. The CB-17 strain of mice is genetically similar to Balb/c except for the presence of immunoglobulin heavy chain, Igh-1b allele of the C57BL/Ka strain. These mice were chosen because of the availability of the SCID counterpart on the CB-17 background.Citation4 Additionally, immunoglobulin heavy chain deleted Jh (Balb/c) and nude (Balb/c) mice from Taconic farms were used for lethal challenges. C57BL/6 mice genetically deficient in IL-23p19 (B6.129-IL23p19tm1Dnax) also called IL-23p19 KO, were obtained from Merck Research Labs, Palo Alto, CA) All animals were housed in a specific-pathogen-free environment and were negative for pathogens in routine screening.
Murine lethal challenge model: Active and passive immunization and challenge
Active and passive immunization were used to evaluate IsdB-mediated protection in a disseminated lethal challenge model. The challenge model was previously described.Citation28 Briefly, mice were actively immunized three times with IsdB (20 µg per dose) formulated with amorphous aluminum hydroxyphosphate sulfate adjuvant (AAHSA) or bovine serum albumin (BSA, negative control) formulated with AAHSA. The doses were administered as two 50 µL intramuscular injections on days 0, 7 and 21. The mice were bled on day 28, and sera were screened for reactivity to IsdB by ELISA. On day 35 the mice were challenged with an LD80–90 dose of S. aureus (from TSA culture, 4.9 × 108 to 8.7 × 108 CFU/ mouse) by intravenous tail vein injection, and survival was monitored for 10 d, as most fatalities were encountered between 2 and 6 d post challenge. Unless otherwise noted, S. aureus strain Becker was used throughout for lethal challenges. In some experiments, 400 ug/mouse of a neutralizing rat anti-mouse IL-17A antibody (JL7.1D10, Merck Research Labs), a neutralizing rat anti-mouse IFN-γ antibody (XMG 1.2, Merck Research Labs), and mouse anti-human IL-22mAb (100% crossreactive with mouse IL-22, R&D Systems, Catalog # 782IL) or isotype control mAb (7D7, Merck Research Labs) were administered i.p. to immunized mice 2 h prior to iv bacterial challenge.Citation71 For passive IsdB immunization, CB-17 (WT and SCID) mice were injected with 400 µg of IsdB specific mAb (CS-D7) or irrelevant isotype matched control (MK-24) via the i.p. route, 2 h prior to lethal challenge with S. aureus SA025, as previously described.Citation31 Lethal challenge was done via tail vein using SA025 (TSA culture, 1.9 × 108 to 8.7 × 108 CFU) via tail vein injection. Survival was monitored for 10 d post challenge.
ELISA assays
To measure anti-IsdB titers an ELISA was performed as previously described.Citation30 IL-17A levels in the serum were determined using mouse IL-17A ELISA kit (Invitrogen, Catalog #KMC 3021) as per manufacturer's instructions (data not shown).
ELISpot assays
The gamma interferon (IFN-γ) and IL-17A spot ELISA (ELISpot) assays were performed using kits (Mabtech, Catalog # 3321–2A and 3521–2A respectively). Briefly, peptides spanning the entire length of IsdB were synthesized (JPT PeptideTech) and pooled such that each pool had a final concentration of 1mM. Peptides were synthesized as 15mers with 11 amino acid overlap and covered aa 1–645 of SACOL 1138 (IsdB). Splenocytes (2.5 × 105) were incubated with 10 µM peptide pools, for 18–20 h. Cells were suspended in 100 µl RPMI supplemented with 10% fetal bovine sera (Atlanta Biologicals), 10 mM HEPES, 2 mM glutamine, 100 U penicillin ml–1 and 100 µg streptomycin ml–1 (P/S) in duplicate wells. Spot-forming cells (SFCs) were detected with a biotin–avidin alkaline phosphatase conjugate, using an immunospot analyzer (Cellular Technology).
Lymphocyte isolation and adoptive transfers
Lymphocyte subsets, namely CD4+ T-cells, CD8+ T-cells, CD3+ T-cells, CD19+ B-cells, and CD138/B220/CD19+ plasma cells were purified from spleens of CB-17 mice using immuno magnetic selection (Miltenyi Biotec) as per the manufacturer's directions. Briefly, cells were labeled with a cocktail of cell surface markers except the marker for the population of interest. The labeled cell population was passed through a magnetic column and the negative fraction (unbound cells) was collected. A small sample from the eluted purified population was labeled with the CD marker of interest, and analyzed on a FACS Calibur flow cytometer (BD Biosciences) to assess the purity(data not shown). 107 isolated lymphocytes from IsdB immunized or BSA immunized mice were transferred into each recipient mouse via the tail vein. Lethal i.v. challenge with S. aureus was performed 2 h post transfer of lymphocyte subsets.
Cytokine analysis
The cytokine secretion analysis was done using intracellular cytokine staining kits (BD Biosciences, Catalog # 51-2041AK). Briefly, 1–2 × 106 lymphocytes were incubated with or without peptide pools (10 µg/mL) or with or without intact protein (IsdB, 25 μg/ml) for 2 h. Cells were treated with brefeldinA for an additional 4 h then washed, permeabilized and fixed using the cytofix/cytoperm reagent. PE-conjugated mouse IFN-γ, IL4 and IL-17A mAbs were used to measure cytokine secretion. PE-conjugated isotype control cocktail as well as purified blocking antibody cocktail were used to measure background. Additionally, in some instances cells were also stained with APC labeled anti-CD4 mAb (BD Biosciences, 553051) to determine the frequency of CD4+ cytokine+ cells. Forward- vs. side-scatter profiles were used to define the live splenocyte populations, and gates were set based on antibody isotype controls and cells that stained positive for the protein of interest. Samples were collected on a FACSCalibur flow cytometer (BD Biosciences), and analysis was performed using Treestar Flow Jo (Ashland) software.
Statistical methods
For comparison of survival in the murine lethal challenge experiments individual experiments were analyzed using the Prism software (Prism for Windows, version 5.01, GraphPad Software), and choosing the Log rank, Mantel Cox test statistical method for testing statistical significance. Probability (P) values < 0.05 were considered significant. In some cases, data were pooled from identical experiments for analysis.
Abbreviations: | ||
amorphous aluminum hydroxyphosphate sulfate adjuvant | = | AAHSA |
opsonophagocytosis | = | OP |
severe combined immunodeficiency | = | SCID |
bovine serum albumin | = | BSA |
intracellular cytokine staining | = | ICS |
Additional material
Download Zip (448.4 KB)Acknowledgments
The authors would like to thank Drs Jan ter Meulen and J. Donald Capra for fruitful discussions during the course of this work. We also wish to thank Dr Jon H Heinrichs for critical review of the manuscript.
References
- Lee JC. The prospects for developing a vaccine against Staphylococcus aureus. Trends Microbiol 1996; 4:162 - 6; http://dx.doi.org/10.1016/0966-842X(96)10021-4; PMID: 8728611
- Middleton JR. Staphylococcus aureus antigens and challenges in vaccine development. Expert Rev Vaccines 2008; 7:805 - 15; http://dx.doi.org/10.1586/14760584.7.6.805; PMID: 18665778
- Otto M. Targeted immunotherapy for staphylococcal infections : focus on anti-MSCRAMM antibodies. BioDrugs 2008; 22:27 - 36; http://dx.doi.org/10.2165/00063030-200822010-00003; PMID: 18215088
- Schaffer AC, Lee JC. Vaccination and passive immunisation against Staphylococcus aureus. Int J Antimicrob Agents 2008; 32:Suppl 1 S71 - 8; http://dx.doi.org/10.1016/j.ijantimicag.2008.06.009; PMID: 18757184
- Cunnion KM, Benjamin DK Jr., Hester CG, Frank MM. Role of complement receptors 1 and 2 (CD35 and CD21), C3, C4, and C5 in survival by mice of Staphylococcus aureus bacteremia. J Lab Clin Med 2004; 143:358 - 65; http://dx.doi.org/10.1016/j.lab.2004.03.005; PMID: 15192652
- Gregory SH, Sagnimeni AJ, Wing EJ. Bacteria in the bloodstream are trapped in the liver and killed by immigrating neutrophils. J Immunol 1996; 157:2514 - 20; PMID: 8805652
- Verdrengh M, Tarkowski A. Role of neutrophils in experimental septicemia and septic arthritis induced by Staphylococcus aureus. Infect Immun 1997; 65:2517 - 21; PMID: 9199413
- Peterson PK, Wilkinson BJ, Kim Y, Schmeling D, Douglas SD, Quie PG, et al. The key role of peptidoglycan in the opsonization of Staphylococcus aureus. J Clin Invest 1978; 61:597 - 609; http://dx.doi.org/10.1172/JCI108971; PMID: 641141
- Verbrugh HA, Peterson PK, Nguyen BYT, Sisson SP, Kim Y. Opsonization of encapsulated Staphylococcus aureus: the role of specific antibody and complement. J Immunol 1982; 129:1681 - 7; PMID: 7108223
- Leijh PCJ, van den Barselaar MT, Daha MR, van Furth R. Participation of immunoglobulins and complement components in the intracellular killing of Staphylococcus aureus and Escherichia coli by human granulocytes. Infect Immun 1981; 33:714 - 24; PMID: 7026443
- Anwar S, Prince LR, Foster SJ, Whyte MKB, Sabroe I. The rise and rise of Staphylococcus aureus: laughing in the face of granulocytes. Clin Exp Immunol 2009; 157:216 - 24; http://dx.doi.org/10.1111/j.1365-2249.2009.03950.x; PMID: 19604261
- Robertson CM, Perrone EE, McConnell KW, Dunne WM, Boody B, Brahmbhatt T, et al. Neutrophil depletion causes a fatal defect in murine pulmonary Staphylococcus aureus clearance. J Surg Res 2008; 150:278 - 85; http://dx.doi.org/10.1016/j.jss.2008.02.009; PMID: 18621398
- Voyich JM, Braughton KR, Sturdevant DE, Whitney AR, Saïd-Salim B, Porcella SF, et al. Insights into mechanisms used by Staphylococcus aureus to avoid destruction by human neutrophils. J Immunol 2005; 175:3907 - 19; PMID: 16148137
- Spellberg B, Ibrahim AS, Yeaman MR, Lin L, Fu Y, Avanesian V, et al. The antifungal vaccine derived from the recombinant N terminus of Als3p protects mice against the bacterium Staphylococcus aureus. Infect Immun 2008; 76:4574 - 80; http://dx.doi.org/10.1128/IAI.00700-08; PMID: 18644876
- Gjertsson I, Hultgren OH, Stenson M, Holmdahl R, Tarkowski A. Are B lymphocytes of importance in severe Staphylococcus aureus infections?. Infect Immun 2000; 68:2431 - 4; http://dx.doi.org/10.1128/IAI.68.5.2431-2434.2000; PMID: 10768927
- Gjertsson I, Nitschke L, Tarkowski A. The role of B cell CD22 expression in Staphylococcus aureus arthritis and sepsis. Microbes Infect 2004; 6:377 - 82; http://dx.doi.org/10.1016/j.micinf.2003.12.013; PMID: 15050965
- Zhao Y, Abdelnour A, Holmdahl R, Tarkowski A, Th International Congress of Immunology. The XID defect provides protection against Staphylococcus aureus-induced arthritis. J Immunol 1995; 155:2067 - 76; PMID: 7636257
- Sasaki S, Nishikawa S, Miura T, Mizuki M, Yamada K, Madarame H, et al. Interleukin-4 and interleukin-10 are involved in host resistance to Staphylococcus aureus infection through regulation of gamma interferon. Infect Immun 2000; 68:2424 - 30; http://dx.doi.org/10.1128/IAI.68.5.2424-2430.2000; PMID: 10768926
- Lin L, Ibrahim AS, Xu X, Farber JM, Avanesian V, Baquir B, et al. Th1-Th17 cells mediate protective adaptive immunity against Staphylococcus aureus and Candida albicans infection in mice. PLoS Pathog 2009; 5:e1000703; http://dx.doi.org/10.1371/journal.ppat.1000703; PMID: 20041174
- McLoughlin RM, Solinga RM, Rich J, Zaleski KJ, Cocchiaro JL, Risley A, et al. CD4+ T cells and CXC chemokines modulate the pathogenesis of Staphylococcus aureus wound infections. Proc Natl Acad Sci U S A 2006; 103:10408 - 13; http://dx.doi.org/10.1073/pnas.0508961103; PMID: 16801559
- Iwakura Y, Nakae S, Saijo S, Ishigame H. The roles of IL-17A in inflammatory immune responses and host defense against pathogens. Immunol Rev 2008; 226:57 - 79; http://dx.doi.org/10.1111/j.1600-065X.2008.00699.x; PMID: 19161416
- Minegishi Y, Saito M, Nagasawa M, Takada H, Hara T, Tsuchiya S, et al. Molecular explanation for the contradiction between systemic Th17 defect and localized bacterial infection in hyper-IgE syndrome. J Exp Med 2009; 206:1291 - 301; http://dx.doi.org/10.1084/jem.20082767; PMID: 19487419
- Tuchscherr L, Medina E, Hussain M, Völker W, Heitmann V, Niemann S, et al. Staphylococcus aureus phenotype switching: an effective bacterial strategy to escape host immune response and establish a chronic infection. EMBO Mol Med 2011; 3:129 - 41; http://dx.doi.org/10.1002/emmm.201000115; PMID: 21268281
- Lin Y, Ritchea S, Logar A, Slight S, Messmer M, Rangel-Moreno J, et al. Interleukin-17 is required for T helper 1 cell immunity and host resistance to the intracellular pathogen Francisella tularensis. Immunity 2009; 31:799 - 810; http://dx.doi.org/10.1016/j.immuni.2009.08.025; PMID: 19853481
- Roche FM, Massey R, Peacock SJ, Day NPJ, Visai L, Speziale P, et al. Characterization of novel LPXTG-containing proteins of Staphylococcus aureus identified from genome sequences. Microbiology 2003; 149:643 - 54; http://dx.doi.org/10.1099/mic.0.25996-0; PMID: 12634333
- Morrissey JA, Cockayne A, Hammacott J, Bishop K, Denman-Johnson A, Hill PJ, et al. Conservation, surface exposure, and in vivo expression of the Frp family of iron-regulated cell wall proteins in Staphylococcus aureus. Infect Immun 2002; 70:2399 - 407; http://dx.doi.org/10.1128/IAI.70.5.2399-2407.2002; PMID: 11953376
- Mazmanian SK, Skaar EP, Gaspar AH, Humayun M, Gornicki P, Jelenska J, et al. Passage of heme-iron across the envelope of Staphylococcus aureus. Science 2003; 299:906 - 9; http://dx.doi.org/10.1126/science.1081147; PMID: 12574635
- Brown M, Kowalski R, Zorman J, Wang XM, Towne V, Zhao QJ, et al. Selection and characterization of murine monoclonal antibodies to Staphylococcus aureus iron-regulated surface determinant B with functional activity in vitro and in vivo. Clin Vaccine Immunol 2009; 16:1095 - 104; http://dx.doi.org/10.1128/CVI.00085-09; PMID: 19553551
- Lowy FD. Staphylococcus aureus infections. N Engl J Med 1998; 339:520 - 32; http://dx.doi.org/10.1056/NEJM199808203390806; PMID: 9709046
- Kuklin NA, Clark DJ, Secore S, Cook J, Cope LD, McNeely T, et al. A novel Staphylococcus aureus vaccine: iron surface determinant B induces rapid antibody responses in rhesus macaques and specific increased survival in a murine S. aureus sepsis model. Infect Immun 2006; 74:2215 - 23; http://dx.doi.org/10.1128/IAI.74.4.2215-2223.2006; PMID: 16552052
- Ebert T, Smith S, Pancari G, Clark D, Hampton R, Secore S, et al. A fully human monoclonal antibody to Staphylococcus aureus iron regulated surface determinant B (IsdB) with functional activity in vitro and in vivo. Hum Antibodies 2010; 19:113 - 28; PMID: 21178283
- Wilson NJ, Boniface K, Chan JR, McKenzie BS, Blumenschein WM, Mattson JD, et al. Development, cytokine profile and function of human interleukin 17-producing helper T cells. Nat Immunol 2007; 8:950 - 7; http://dx.doi.org/10.1038/ni1497; PMID: 17676044
- Weaver CT, Hatton RD, Mangan PR, Harrington LE. IL-17 family cytokines and the expanding diversity of effector T cell lineages. Annu Rev Immunol 2007; 25:821 - 52; http://dx.doi.org/10.1146/annurev.immunol.25.022106.141557; PMID: 17201677
- Crome SQ, Wang AY, Levings MK. Translational mini-review series on Th17 cells: function and regulation of human T helper 17 cells in health and disease. Clin Exp Immunol 2010; 159:109 - 19; http://dx.doi.org/10.1111/j.1365-2249.2009.04037.x; PMID: 19912252
- Dryla A, Prustomersky S, Gelbmann D, Hanner M, Bettinger E, Kocsis B, et al. Comparison of antibody repertoires against Staphylococcus aureus in healthy individuals and in acutely infected patients. Clin Diagn Lab Immunol 2005; 12:387 - 98; PMID: 15753252
- Verkaik NJ, Lebon A, de Vogel CP, Hooijkaas H, Verbrugh HA, Jaddoe VWV, et al. Induction of antibodies by Staphylococcus aureus nasal colonization in young children. Clin Microbiol Infect 2010; 16:1312 - 7; http://dx.doi.org/10.1111/j.1469-0691.2009.03073.x; PMID: 19832714
- Verkaik NJ, Dauwalder O, Antri K, Boubekri I, de Vogel CP, Badiou C, et al. Immunogenicity of toxins during Staphylococcus aureus infection. Clin Infect Dis 2010; 50:61 - 8; http://dx.doi.org/10.1086/648673; PMID: 19947854
- Verkaik NJ, Boelens HA, de Vogel CP, Tavakol M, Bode LGM, Verbrugh HA, et al. Heterogeneity of the humoral immune response following Staphylococcus aureus bacteremia. Eur J Clin Microbiol Infect Dis 2010; 29:509 - 18; http://dx.doi.org/10.1007/s10096-010-0888-0; PMID: 20186449
- Colque-Navarro P, Palma M, Söderquist B, Flock JI, Möllby R. Antibody responses in patients with staphylococcal septicemia against two Staphylococcus aureus fibrinogen binding proteins: clumping factor and an extracellular fibrinogen binding protein. Clin Diagn Lab Immunol 2000; 7:14 - 20; PMID: 10618270
- Skurnik D, Merighi M, Grout M, Gadjeva M, Maira-Litran T, Ericsson M, et al. Animal and human antibodies to distinct Staphylococcus aureus antigens mutually neutralize opsonic killing and protection in mice. J Clin Invest 2010; 120:3220 - 33; http://dx.doi.org/10.1172/JCI42748; PMID: 20739753
- Malley R. Antibody and cell-mediated immunity to Streptococcus pneumoniae: implications for vaccine development. Journal of Molecular Medicine (Berlin) 2010; 88
- Zhang Z, Clarke TB, Weiser JN. Cellular effectors mediating Th17-dependent clearance of pneumococcal colonization in mice. J Clin Invest 2009; 119:1899 - 909; PMID: 19509469
- McCool TL, Weiser JN. Limited role of antibody in clearance of Streptococcus pneumoniae in a murine model of colonization. Infect Immun 2004; 72:5807 - 13; http://dx.doi.org/10.1128/IAI.72.10.5807-5813.2004; PMID: 15385481
- Flach CF, Östberg AK, Nilsson AT, Malefyt RdeW, Raghavan S. Proinflammatory cytokine gene expression in the stomach correlates with vaccine-induced protection against Helicobacter pylori infection in mice: an important role for interleukin-17 during the effector phase. Infect Immun 2011; 79:879 - 86; http://dx.doi.org/10.1128/IAI.00756-10; PMID: 21078851
- Ermak TH, Giannasca PJ, Nichols R, Myers GA, Nedrud J, Weltzin R, et al. Immunization of mice with urease vaccine affords protection against Helicobacter pylori infection in the absence of antibodies and is mediated by MHC class II-restricted responses. J Exp Med 1998; 188:2277 - 88; http://dx.doi.org/10.1084/jem.188.12.2277; PMID: 9858514
- Grimbacher B, Holland SM, Gallin JI, Greenberg F, Hill SC, Malech HL, et al. Hyper-IgE syndrome with recurrent infections--an autosomal dominant multisystem disorder. N Engl J Med 1999; 340:692 - 702; http://dx.doi.org/10.1056/NEJM199903043400904; PMID: 10053178
- Milner JD, Brenchley JM, Laurence A, Freeman AF, Hill BJ, Elias KM, et al. Impaired T(H)17 cell differentiation in subjects with autosomal dominant hyper-IgE syndrome. Nature 2008; 452:773 - 6; http://dx.doi.org/10.1038/nature06764; PMID: 18337720
- Ma CS, Chew GYJ, Simpson N, Priyadarshi A, Wong M, Grimbacher B, et al. Deficiency of Th17 cells in hyper IgE syndrome due to mutations in STAT3. J Exp Med 2008; 205:1551 - 7; http://dx.doi.org/10.1084/jem.20080218; PMID: 18591410
- Minegishi Y, Karasuyama H. Defects in Jak-STAT-mediated cytokine signals cause hyper-IgE syndrome: lessons from a primary immunodeficiency. Int Immunol 2009; 21:105 - 12; http://dx.doi.org/10.1093/intimm/dxn134; PMID: 19088064
- Fischer A. Human immunodeficiency: connecting STAT3, Th17 and human mucosal immunity. Immunol Cell Biol 2008; 86:549 - 51; http://dx.doi.org/10.1038/icb.2008.52; PMID: 18645579
- Peck A, Mellins ED. Precarious balance: Th17 cells in host defense. Infect Immun 2010; 78:32 - 8; http://dx.doi.org/10.1128/IAI.00929-09; PMID: 19901061
- Puel A, Döffinger R, Natividad A, Chrabieh M, Barcenas-Morales G, Picard C, et al. Autoantibodies against IL-17A, IL-17F, and IL-22 in patients with chronic mucocutaneous candidiasis and autoimmune polyendocrine syndrome type I. J Exp Med 2010; 207:291 - 7; http://dx.doi.org/10.1084/jem.20091983; PMID: 20123958
- Puel A, Cypowyj S, Bustamante J, Wright JF, Liu L, Lim HK, et al. Chronic mucocutaneous candidiasis in humans with inborn errors of interleukin-17 immunity. Science 2011; 332:65 - 8; http://dx.doi.org/10.1126/science.1200439; PMID: 21350122
- Ishigame H, Kakuta S, Nagai T, Kadoki M, Nambu A, Komiyama Y, et al. Differential roles of interleukin-17A and -17F in host defense against mucoepithelial bacterial infection and allergic responses. Immunity 2009; 30:108 - 19; http://dx.doi.org/10.1016/j.immuni.2008.11.009; PMID: 19144317
- Cho JS, Pietras EM, Garcia NC, Ramos RI, Farzam DM, Monroe HR, et al. IL-17 is essential for host defense against cutaneous Staphylococcus aureus infection in mice. J Clin Invest 2010; 120:1762 - 73; http://dx.doi.org/10.1172/JCI40891; PMID: 20364087
- Kim HK, DeDent A, Cheng AG, McAdow M, Bagnoli F, Missiakas DM, et al. IsdA and IsdB antibodies protect mice against Staphylococcus aureus abscess formation and lethal challenge. Vaccine 2010; 28:6382 - 92; http://dx.doi.org/10.1016/j.vaccine.2010.02.097; PMID: 20226248
- Markham RB, Pier GB, Schreiber JR. The role of cytophilic IgG3 antibody in T cell-mediated resistance to infection with the extracellular bacterium, Pseudomonas aeruginosa. J Immunol 1991; 146:316 - 20; PMID: 1898604
- Culkin SJ, Rhinehart-Jones T, Elkins KL. A novel role for B cells in early protective immunity to an intracellular pathogen, Francisella tularensis strain LVS. J Immunol 1997; 158:3277 - 84; PMID: 9120284
- Lin L, Ibrahim AS, Baquir B, Avanesian V, Fu Y, Spellberg B. Immunological surrogate marker of rAls3p-N vaccine-induced protection against Staphylococcus aureus. FEMS Immunol Med Microbiol 2009; 55:293 - 5; http://dx.doi.org/10.1111/j.1574-695X.2008.00531.x; PMID: 19159425
- Mosmann TR, Cherwinski H, Bond MW, Giedlin MA, Coffman RL. Two types of murine helper T cell clone. I. Definition according to profiles of lymphokine activities and secreted proteins. J Immunol 1986; 136:2348 - 57; PMID: 2419430
- Spellberg B, Daum R. A new view on development of a Staphylococcus aureus vaccine: insights from mice and men. Hum Vaccin 2010; 6:857 - 9; http://dx.doi.org/10.4161/hv.6.10.12469; PMID: 20930569
- Fattom AI, Sarwar J, Ortiz A, Naso R. A Staphylococcus aureus capsular polysaccharide (CP) vaccine and CP-specific antibodies protect mice against bacterial challenge. Infect Immun 1996; 64:1659 - 65; PMID: 8613375
- Fattom AI, Horwith G, Fuller S, Propst M, Naso R. Development of StaphVAX, a polysaccharide conjugate vaccine against S. aureus infection: from the lab bench to phase III clinical trials. Vaccine 2004; 22:880 - 7; http://dx.doi.org/10.1016/j.vaccine.2003.11.034; PMID: 15040941
- Cook J, Hepler R, Pancari G, Kuklin N, Fan HX, Wang XM, et al. Staphylococcus aureus capsule type 8 antibodies provide inconsistent efficacy in murine models of staphylococcal infection. Hum Vaccin 2009; 5:254 - 63; http://dx.doi.org/10.4161/hv.5.4.6765; PMID: 18787395
- Patti JM. A humanized monoclonal antibody targeting Staphylococcus aureus. Vaccine 2004; 22:Suppl 1 S39 - 43; http://dx.doi.org/10.1016/j.vaccine.2004.08.015; PMID: 15576200
- Vernachio JH, Bayer AS, Ames B, Bryant D, Prater BD, Syribeys PJ, et al. Human immunoglobulin G recognizing fibrinogen-binding surface proteins is protective against both Staphylococcus aureus and Staphylococcus epidermidis infections in vivo. Antimicrob Agents Chemother 2006; 50:511 - 8; http://dx.doi.org/10.1128/AAC.50.2.511-518.2006; PMID: 16436704
- Burnie JP, Matthews RC, Carter T, Beaulieu E, Donohoe M, Chapman C, et al. Identification of an immunodominant ABC transporter in methicillin-resistant Staphylococcus aureus infections. Infect Immun 2000; 68:3200 - 9; http://dx.doi.org/10.1128/IAI.68.6.3200-3209.2000; PMID: 10816464
- Rupp ME, Holley HP Jr., Lutz J, Dicpinigaitis PV, Woods CW, Levine DP, et al. Phase II, randomized, multicenter, double-blind, placebo-controlled trial of a polyclonal anti-Staphylococcus aureus capsular polysaccharide immune globulin in treatment of Staphylococcus aureus bacteremia. Antimicrob Agents Chemother 2007; 51:4249 - 54; http://dx.doi.org/10.1128/AAC.00570-07; PMID: 17893153
- DeJonge M, Burchfield D, Bloom B, Duenas M, Walker W, Polak M, et al. Clinical trial of safety and efficacy of INH-A21 for the prevention of nosocomial staphylococcal bloodstream infection in premature infants. J Pediatr 2007; 151:260 - 5, 265, e1; http://dx.doi.org/10.1016/j.jpeds.2007.04.060; PMID: 17719934
- Weems JJ Jr., Steinberg JP, Filler S, Baddley JW, Corey GR, Sampathkumar P, et al. Phase II, randomized, double-blind, multicenter study comparing the safety and pharmacokinetics of tefibazumab to placebo for treatment of Staphylococcus aureus bacteremia. Antimicrob Agents Chemother 2006; 50:2751 - 5; http://dx.doi.org/10.1128/AAC.00096-06; PMID: 16870768
- Chao CC, Chen SJ, Adamopoulos IE, Davis N, Hong K, Vu A, et al. Anti-IL-17A therapy protects against bone erosion in experimental models of rheumatoid arthritis. Autoimmunity 2011; 44:243 - 52; http://dx.doi.org/10.3109/08916934.2010.517815; PMID: 20925596