Abstract
Due to the strong relationship between the Human Papillomavirus (HPV) “high-risk” subtypes and cervical cancers, most HPV-related studies have been focusing on the “high-risk” HPV subtypes 16 and 18. However, it has been suggested that the “low-risk” subtypes of HPV, HPV6 and HPV11, are the major cause of recurrent respiratory papillomatosis and genital warts. In addition, HPV 6 and 11 are also associated with otolaryngologic malignancies, carcinoma of the lung, tonsil, larynx and low-grade cervical lesions. Therefore, development of HPV therapeutic vaccines targeting on subtypes 6 and 11 E6 or E7 are in great need. In this report, we describe two novel engineered DNA vaccines that encode HPV 6 and 11 consensus E6/E7 fusion proteins (p6E6E7 and p11E6E7) by utilizing a multi-phase strategy. Briefly, after generating consensus sequences, several modifications were performed to increase the expression of both constructs, including codon/RNA optimization, addition of a Kozak sequence and a highly efficient leader sequence. An endoproteolytic cleavage site was also introduced between E6 and E7 protein for proper protein folding and for better CTL processing. The expressions of both constructs were confirmed by western blot analysis and immunofluorescence assay. Vaccination with these DNA vaccines could elicit robust cellular immune responses. The epitope mapping assay was performed to further characterize the cellular immune responses induced by p6E6E7 and p11E6E7. The HPV 6 and 11 E6 or E7-specific immunodominant and subdominant epitopes were verified, respectively. The intracellular cytokine staining revealed that the magnitude of IFN-γ and TNF-α secretion in antigen-specific CD8+ cells was significantly enhanced, indicating that the immune responses elicited by p6E6E7 and p11E6E7 was heavily skewed toward driving CD8+ T cells. Such DNA immunogens are interesting candidates for further studies on HPV 6 and 11-associated diseases.
Introduction
Human Papillomavirus (HPV) has drawn a growing amount of scientific research and attention in light of the virus’s association with various endemic malignancies, including the causal relationship between HPV16, HPV18, and cervical cancer. Historically, HPV6 and HPV11 have been considered “low-risk” given the two strains’ more limited association with genital warts.Citation1,Citation2 However, recent research has begun to unravel the manifestation of low-risk strains of HPV in more aggressive forms of otolaryngologic malignancies.Citation3 Furthermore, various studies have revealed the presence of HPV6 and HPV11 in most incidences of recurrent respiratory papillomatosis.Citation3-Citation5 Moreover, additional studies have found that HPV6 and HPV11 are associated with more aggressive forms of cancer, including carcinomas of the lung, tonsil, and larynx.Citation4 Though only found in a small percentage of cervical cancer cases, HPV6 and HPV11 have been found in 2.6–5.2% of all cases of low-grade cervical lesions.Citation1 Thus, the growing clinical implications of HPV6 and HPV11 demands greater insight into ways to protect individuals from, not only historically notorious strains of HPV, but also those generally considered “lower risk,” including HPV6 and HPV11.
With over 100 subtypes now identified, much progress has been made in describing the etiology of the HPV virus. HPV is a double-stranded DNA virus composed of approximately 8,000 base pairs. Different genes within the HPV genome are broadly categorized into either “early” or “late” antigens, referred to using a nomenclature labeling genes with either an “E” denoting early or “L” denoting late expression during the viral life cycle. Various factors, such as conservation throughout the viral life cycle, similarities across subtypes, and role in capsid envelope structure, have supported successful HPV vaccine development to focus on the L1 protein.Citation6,Citation7 However, E6 and E7 are critical in the oncogenic transformation of infected cells. This discovery has become of special attention given that researchers have now determined approximately 15 subtypes of HPV to be either causative of or correlated with oncogenic proliferation of infected cells. E6 and E7 are regulated by E2, a gene that is generally lost through viral integration post-infection. In the absence of E2, E6 and E7 proteins inhibit p53 and retinoblastoma protein functionality, which eventually paves the way toward aggressive proliferation and oncogenic transformation of an infected host cell. The two oncogenes have been found to be highly conserved throughout HPV subtypes and avoid deletion throughout cellular transformation.Citation8 Having identified the critical role of E6 and E7, HPV vaccine research has increasingly aimed to exploit the abovementioned characteristics of the two oncogenes and make them the target of vaccine design.
The two licensed vaccines against HPV invoke L1-specific immune responses against up to 4 HPV strains. Numerous studies have confirmed and reiterated the vaccine’s ability to provide protection against infection for HPV naïve individuals. These same vaccines do not alter the course of prior infection, given the increasing rates of HPV infection and the steady amount of pre-existing global HPV burden, a prophylactic strategy alone will not solve the HPV pandemic. Acknowledging these shortcomings, researchers have explored other alternatives, including RNA-based vaccines, dendritic whole cell vaccines, and combinations of different vaccine platforms, targeting HPV oncogenes.Citation8-Citation11
Recent discoveries from such research have shed light on the growing promise of DNA-based vaccines as a possible therapeutic approach against HPV. DNA vaccines contain antigen-specific genes that are later expressed and presented to lymphocytes by antigen-presenting cells (APCs), such as dendritic cells. Due to their safety, ease of storage, and mass production capacity, DNA vaccines have received much attention in combating various diseases that are heavily endemic in the developing world. Numerous optimization, delivery and boost strategies have been developed and are currently being studied.Citation8,Citation12-Citation18 Recent work on DNA vaccines for HPV therapy have produced promising preliminary results which have now led to clinical trials targeting the HPV 16 or 18 subtypes.Citation12-Citation14,Citation19-Citation22 However, there have been no prior reports focusing on HPV subtypes 6+11.
This current study examines the immunogenicity of p6E6E7 and p11E6E7: plasmids encoding novel consensus oncogenes E6 and E7 of HPV6 and HPV11 subtypes. The two plasmids were designed by both codon and RNA optimization strategies and were delivered to groups of C57BL/6 mice using in vivo electroporation. We found that p6E6E7 and p11E6E7 proved to be highly immunogenic vaccines that mounted very robust HPV 6 or 11 E6 and E7-specific T-cell immune responses in C57BL/6 mice.
Results
Construction of HPV6 and 11 E6/E7 consensus-based fusion immunogens
The consensus sequence of HPV 6 E6 or E7 proteins was generated from 98 or 20 sequences, respectively, while the consensus sequence of HPV 11 E6 or E7 proteins was generated from 76 or 13 sequences, respectively. The multiple alignment procedure applied in the phylogenetic study included the application of Clustal X (version 2.0). As indicated in , there were about 0–2% of sequence divergence among the HPV strains belonging to the same type in their E6 and E7 proteins. However, the genetic distances could go up to 19.3% in the E6 protein and 16.3% in the E7 protein between HPV 6 and 11. Based on these results from the phylogenic analyses, we developed two type-specific E6/E7 consensus DNA vaccines.
Figure 1. Phylogenetic trees based on neighbor-joining evaluation of E6 and E7 alignments (A and B, respectively). Asterisks indicate location of consensus sequences on each tree. (C) Schematic design of p6E6E7 and p11E6E7 constructs.
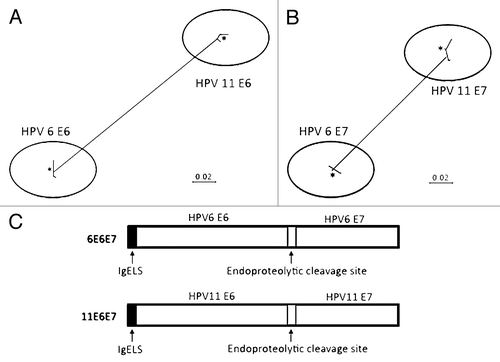
Several modifications were conducted after generating the consensus E6/E7 fusion sequence (). A highly efficient leader sequence was fused in frame upstream of the start codon to facilitate the expression. The codon and RNA optimization was also performed as described previously.Citation12,Citation13 An endoproteolytic cleavage site was introduced between E6 and E7 protein for proper protein folding and better CTL processing. Both synthetic engineered 6E6E7 gene and 11E6E7 genes were 840 bp in length. The sequence verified synthetic genes were subcloned into the pVAX expression vector at the BamHI and XhoI sites respectively for further study.
Confirmation of in vivo expression of p6E6E7 and p11E6E7
In order to test the in vivo expression of p6E6E7 and p11E6E7, rhabdomyosarcoma (RD) analysis cells were transfected with these plasmids and western blot analysis was performed. The results indicated that this construct expressed a 32 kDa E6/E7 protein (). The same procedure was performed using pVAX to serve as a negative control. The pVAX lane did not exhibit any notable or significant autoradiography bands.
Figure 2. In vivo expression of p6E6E7 and p11E6E7. Gene products were isolated from lysed transfected 293-T cells, run through SDS-PAGE gel, and detected using autoradiography. Both HPV 6 and HPV 11 E6/E7 proteins are approximately 32kDa each (A). Human rhabdomyosarcoma (RD) cells were also transfected with p6E6E7 and p11E6E7 and later fixed after immunofluorescence staining. FITC fluorescence confirms expression of p6E6E7 and p11E6E7 (B). DAPI fluorescence confirms nuclei localization consequent of Hoechst staining.
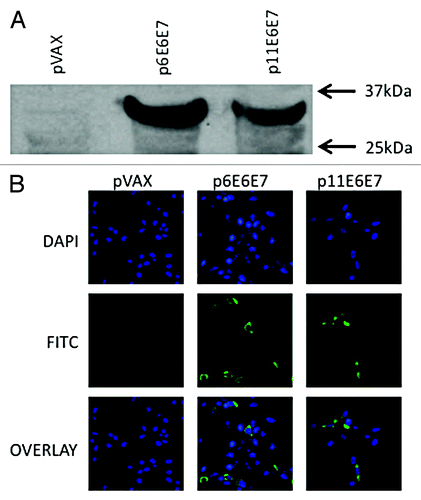
Indirect immunofluorescence assays were performed on transfected RD cells to reaffirm the findings from the above experiment. The resulting images confirmed specific expression of both p6E6E7 and p11E6E7, as indicated by the FITC-fluorescence observed under a confocal microscope (). Successful Hoechst staining localized cell nuclei, as indicated by DAPI-fluorescence. An additional group of RD cells was transfected with pVAX to serve as a negative control. The pVAX-transfected cells did not show any FITC-fluorescence – reinforcing the results described above. Furthermore, the genes expression was localized to the cytoplasm rather than the nucleus illustrating the effect of the addition of leader sequence.
Vaccination with p6E6E7 and p11E6E7 elicits strong HPV6 and 11 E6 and E7-specific T-cell responses in C57BL/6 mice
Given their sensitivity and ability to illustrate T-cell activity, IFN-γ ELISpot assays were used to determine the number of antigen-specific IFN-γ secreting cells in response to stimulation with either HPV 6 or 11 E6 and E7 peptides.
As shown in and 3B, the average number of SFU/106 splenocytes for mice vaccinated with p6E6E7 was 1442.8, while the average number of SFU/106 for mice immunized with p11E6E7 was 2845 against HPV6 E6/E7 and HPV11 E6/E7 peptides respectively, which were all significantly greater than the negative control group. Therefore, both p6E6E7 and p11E6E7 were effective in eliciting robust type-specific E6 and E7-specific immune response in mice.
Figure 3. IFN-γ ELISpot assays show induction of robust cell-mediated responses by p6E6E7 (A) and p11E6E7 (B) in C57BL/6 mice. Assays were performed using splenocytes isolated from mice in each respective group (5 mice per group) after three biweekly immunizations. Each immunization consisted of 20μg per construct. Mice in combo group received both p6E6E7 and p11E6E7 at 20μg per construct, for a total of 40μg DNA per immunization. DNA was administered via IM injection, followed by electroporation (* denotes p < 0.05).
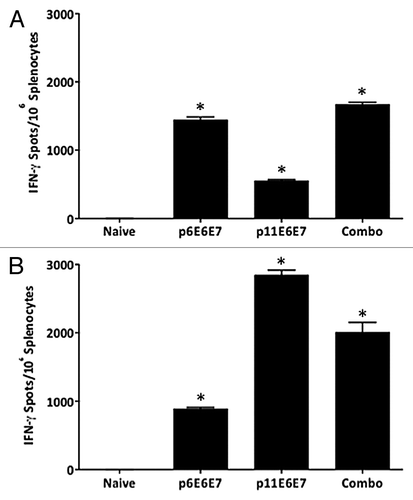
Interestingly, the cross-reactive cellular immune responses were also induced by vaccination with p6E6E7 or p11E6E7. The additive frequency of SFU/106 splenocytes in p11E6E7 immunized mice against HPV 6 E6/E7 peptides was 552.8 SFU/106 splenocytes, and the HPV 11 E6/E7-specific immune responses in p6E6E7 immunized mice was 888.3 SFU/106 splenocytes. As mentioned earlier, the E6 proteins of HPV 6 or 11 share about 80% identity, while the E7 proteins of HPV6 or 11 shared about 84% identity. Theoretically, there may be some shared immune epitopes between HPV 6 or 11 E6 and E7 antigens. The cross-reactivity observed from the IFN-γ ELISpot assay indicated that there were shared immune epitopes between HPV6 and 11 E6 and E7 antigens.
Splenocytes from mice that received both p6E6E7 and p11E6E7 (combo group) were also subjected to the above ELISpot assays in order to examine whether there was any immune interference when these two constructs were vaccinated together (). The combo group exhibited an average of 1670 SFU/106 splenocytes against HPV6 E6 and E7 peptides. The same group of splenocytes produced 2,010 SFU/106 splenocytes against HPV11 E6 and E7 peptides. The data suggest that concurrent vaccination with the two constructs can elicit a statistically significant E6 and E7-specific cellular response against HPV6 and HPV11 and that these responses do not interfere with each other.
Characterization of vaccine-induced E6 and E7-specific T-cell immune response by epitope mapping
To further characterize the above-mentioned response, epitope mapping assays were conducted to determine the immune dominant peptides within the E6/E7 consensus antigens ( and 4B). As discussed previously, each peptide used in this single-peptide analysis represented a partially overlapping fragment of the E6 and E7 antigens of HPV6 or HPV11. The mapping data indicated that peptide 7 (TAEIYSYAYKQLKVL) was the dominant epitope for the HPV6 E6 and E7 immunogens (). TAEIYSYAYKQLKVL contained 8, 9, 10-mer amino acid epitopes that are verified to be an H2-Kb restricted by the HLA-binding prediction software made available by NIH BIMAS. To further describe the HPV11 E6 and E7-specific T-cell immune response (), peptide analysis was also performed with overlapping fragments of HPV11 E6 and E7. Epitope mapping showed that the dominant epitopes for HPV 11 E6 and E7 antigens were peptides 7 (TAEIYAYAYKNLKVV) and 27 (HCYEQLEDSSEDEVD). As with peptide 7 in the HPV6 epitope mapping assay, the BIMAS HLA-binding prediction software confirmed TAEIYAYAYKNLKVV to be an H2-Kb restricted epitope. Another HLA-binding peptide database, the Immune Epitope Database and Analysis Resource provided by NIAID, confirmed that HCYEQLEDSSEDEVD is an H2-Kb restricted epitope. Another three E6 immune subdominant peptides; numbers 6 (FCKNALTTAEIYSYA), 9 (LFRGGYPYAACACCL), and 13 (YAGYATTVEEETKQD), were identified through this epitope mapping study.
Figure 4. Additional IFN-γ ELISpot assays performed using individual peptides to characterize dominant epitopes. Splenocytes isolated from vaccinated mice and negative control were stimulated with overlapping peptides that span the entire HPV 6 E6/E7 fusion protein (A) or HPV 11 E6/E7 fusion protein (B).
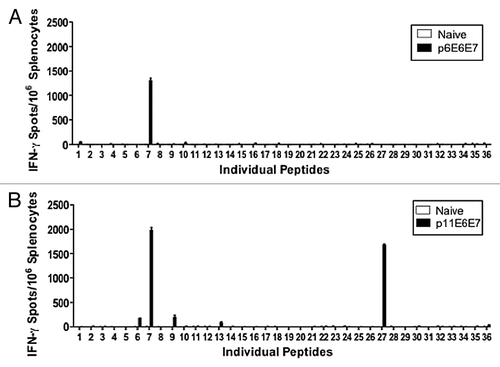
Vaccination with p6E6E7 and p11E6E7 elicits significantly increased production of IFN-γ, TNFα, and IL-2
In light of the high immune response portrayed by the IFN-γ ELISpot assays, intracellular cytokine staining assays were performed to provide a more holistic overview of the cellular response induced by p6E6E7 and p11E6E7. Per procedures outlined in the materials and methods section, cells were gated such that intracellular cytokine staining data can differentiate by CD4+ and CD8+ cell responses (). When stimulated with HPV6 E6 and E7-specific peptides, mice vaccinated with p6E6E7 exhibited averages of 0.163%, 0.003%, and 0.188% of total CD4+ cells producing IFN-γ, TNF-α, and IL-2, respectively (). The same group of mice had averages of 3.323%, 0.838%, and 1.172% of total CD8+ cells producing IFN-γ, TNF-α, and IL-2, respectively. The same intracellular cytokine data was collected using splenocytes from mice vaccinated with p11E6E7 after incubation with HPV11 E6 and E7 antigens (). Of all CD4+ cells in the p11E6E7 vaccinated mice, an average of 0.051% produced IFN-γ, 0.068% produced TNF-α, and 0.026% produced IL-2. Further, an average of 4.52%, 2.08%, and 0.21% of all CD8+ cells in p11E6E7 vaccinated mice produced IFN-γ, TNF-α, and IL-2, respectively. Based on the observation of the magnitude of cytokine production of CD4+ cells vs. CD8+ T-cells, one can conclude that the immune responses elicited by p6E6E7 and p11E6E7 is heavily skewed toward driving CD8+ T cells, which are associated with clearance of infected cells in HPV therapeutic animal models
Figure 5. Schematic diagram of gating strategy of T cells response to the HPV6- and HPV11-specific peptides.
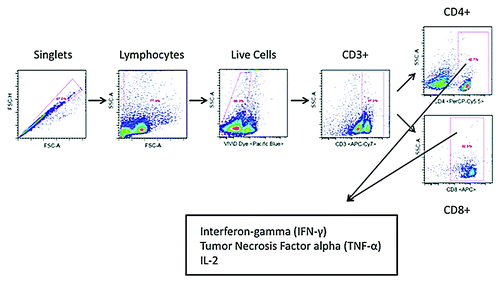
Figure 6. Cytokine production by HPV6 antigen-specific T-cells characterized by intracellular cytokine staining. Splenocytes isolated from mice vaccinated with p6E6E7 were stimulated with R10 growth medium, PMA, or consensus gene peptides for 4 h prior to surface marker and intracellular staining. Dot plots above show differences in background-subtracted percentages of either total CD4+ or CD8+ cells producing IFN-γ, IL-2, and TNF-α.
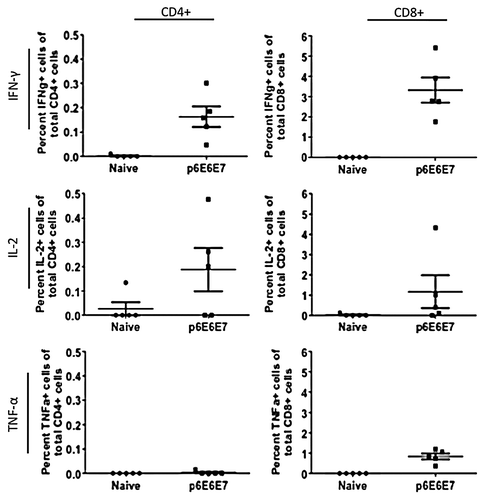
Figure 7. Cytokine production by HPV11 E6E7-specific T-cells quantified by intracellular cytokine staining. As with those cells used to measure HPV6 antigen-specific T-cell cytokine production, splenocytes from mice vaccinated with p11E6E7 were isolated and stimulated with R10 growth medium, PMA, or consensus gene peptides. The cells were subsequently processed for surface marker and intracellular staining. Dot plots above portray percentage of total Cd4+ or CD8+ cells producing IFN-γ, IL-2, and TNF-α. All values graphed have been background-subtracted.
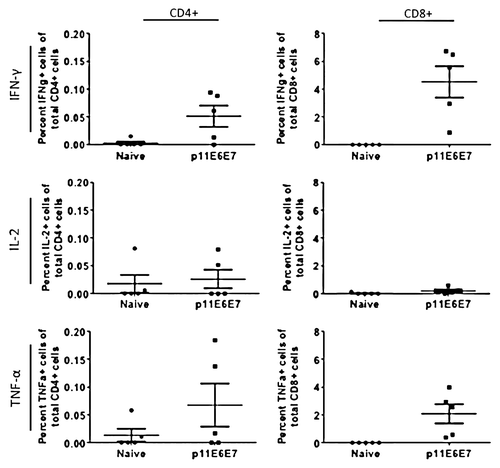
Discussion
This study is the first of its kind using a multi-phase strategy which includes consensus antigen design, codon and RNA optimization, addition of high efficient leader sequence and endoproteolytic cleavage site to develop more potent DNA vaccines against HPV6 and HPV11, and these two vaccines were delivered by electroporation to further improve their immunogenicity. There is much ongoing debate over the immunogenic efficacy of DNA vaccines. In response to such concerns, the two constructs, p6E6E7 and p11E6E7, were constructed using consensus gene design and implemented with optimization techniques that have shown promise in boosting the immunogenicity of DNA vaccines. The results we reported here further suggest and bolster the promising immunogenic potential DNA vaccines may attain when administered in conjunction with various design and delivery strategies, including in vivo electroporation.
This study has shown compelling evidence that DNA vaccines may be able to attain a level of immunogenicity found in experiments using other popular and traditional vaccine platforms. High levels of cellular responses measured in other similar E6 and E7-specific HPV DNA vaccine studies were associated with data suggestive of prophylactic and therapeutic anti-tumor efficacy. Most HPV-related research and disease challenge models focus on cervical cancer. Given that HPV6 and HPV11 are not as relevant to cervical cancer as other HPV subtypes, the therapeutic efficacy of p6E6E7 and p11E6E7 cannot be fully evaluated using conventional HPV disease challenge models. It would be insightful to determine the protective and therapeutic potential of p6E6E7 and p11E6E7 when appropriate disease challenge models become available. Thus, one can only infer the immunogenic efficacy of p6E6E7 and p11E6E7 by looking at the high levels of IFN-γ and cytokine production quantified by the ELISpot assays and intracellular cytokine staining. Nonetheless, such levels were found to be significantly robust in magnitude and show promise that the two constructs would elicit substantive levels of cellular immune responses. Because HPV-related malignancies are generally secondary to concurrent infection of multiple subtypes, there is a great need to look further into the feasibility of combining vaccines targeting different subtypes of the virus. Future studies examining T-cell dynamics and vaccine competition is warranted to further characterize the effects of concurrent vaccination of the two plasmids.
Intracellular cytokine staining showed that vaccination with p6E6E7 and p11E6E7 was able to elicit a significant percentage of IFN-γ, TNF-α, and IL-2 producing T-cells. Given its currently known function in the immune system, IFN-γ has historically been used as a metric of cellular immune responses. Some of these important roles include the ability to modulate and stimulate innate and adaptive immunity. Moreover, it is widely accepted that the principle producers of IFN-γ are T-cells, making IFN-γ production an acceptable mode of measuring the cellular immunogenicity of a given vaccine post-exposure to antigens. TNF-α is another cytokine that is involved in the regulation of the immune system and its known ability to induce apoptosis and regulate tumor proliferation makes it an important parameter to consider when characterizing post-vaccination immune responses. TNF-α production may be of further interest given the potential tumor proliferative properties of HPV6 and HPV11. IL-2 is another signaling molecule that has been observed to play a central role in the proliferation and differentiation of T-cells in the immune system. As a consequence, IL-2 is often examined in conjunction with other cytokines to gain further perspective on the magnitude and quality of a particular immune response. Given the above, the significant percentages of CD4+ and CD8+ cells producing IFN-γ, TNF-α, and IL-2 after vaccination with p6E6E7 suggests that the vaccine was successful in inducing a potent immune response. With the exception of IL-2 secreting CD4+ cells, the same trend is true for cells isolated from mice vaccinated with p11E6E7. Moreover, it is noteworthy to observe that CD8+ cells heavily drove the immune responses in vaccinated mice – a characteristic that is significant in evaluating the anti-tumor efficacy of the two plasmids.
Potential future studies may include various genetic adjuvants in hopes of further boosting the immune response post-vaccination driven by p6E6E7 and p11E6E7. Additional studies using p6E6E7 and p11E6E7 in conjunction with IL-28B/IFN-λ3 may be interesting.Citation23,Citation24 Prior studies examining the immunogenicity of novel DNA vaccines with E6/E7 consensus genes targeting HPV16 and HPV18 have shown much promise for future development into a vaccine for clinical use.Citation12,Citation13 Ideally, clinical trials in humans would be used as the ultimate test to evaluate the vaccines’ efficacy. However, various pre-clinical experiments using other models, including human MHC transgenic animals as well as non-human primates, may generate important data regarding the immune potency of these cassettes.
HPV related malignancies are generally associated with infection of multiple subtypes of the virus. As a result, it is difficult to delineate the clinical ramifications of HPV6 and HPV11 infection alone. Though historically known for their association with genital warts, HPV6 and HPV11 have now been associated with approximately 20% of low-grade squamous intraepithelial lesions that are now considered precursors to cervical cancer, including grade 2 and 3 cervical intraepithelial neoplasia and mild cervical dysplasia.Citation25 Increasing studies have revealed the association of the two subtypes with various forms of otolaryngologic diseases, including genital warts, recurrent respiratory papillomatosis, lung carcinoma, tonsillar carcinoma, laryngeal carcinoma, and other malignant transformations of otherwise benign neoplasms and dysplasia of the head and neck.Citation3-Citation5,Citation26,Citation27 Such etiological observations have brought HPV6 and HPV11 to greater significance in terms of clinical relevance and scientific research. The findings of this study shed light and future promise in the use of consensus sequence DNA vaccines against HPV6 and HPV11. The E6, E7 specificity of p6E6E7 and p11E6E7 addresses the aggressive oncogenic transformation of infected cells. Moreover, the ability of the two plasmids to elicit CD8+ lymphocyte-driven cellular responses is especially of interest given that efficacious anti-cancer therapies rely heavily on cytotoxic T-cell activity. Other investigators have shown a correlative relationship between the incidence of HPV11 infection and the aggressive transformation of benign papillomatosis.Citation3 Though current prophylactic vaccines provide prophylaxis against HPV6 and HPV11, the development of a vaccine capable of mounting robust cellular immune responses would provide a strategy against HPV diseases as well. Further study of these approaches is warranted.
Materials and Methods
HPV6 and HPV11 E6/E7 vaccine design
The HPV subtype 6 or 11 E6 and E7 gene sequences were collected from GeneBank, and the consensus E6 and E7 nucleotide sequences were obtained after performing multiple alignment. The consensus amino acid sequences were obtained by translating the consensus nucleotide sequences.
After obtaining HPV 6 and 11 consensus E6 and E7 sequences, condon optimization and RNA optimization was performed as previously described.Citation12,Citation13 The fusion genes encoding either HPV subtype 6 or 11 consensus E6/E7 fusion protein (6E6E7 or 11E6E7) were synthesized and sequence verified. The synthesized 6E6E7 or 11E6E7 was digested with BamHI and XhoI, cloned into the expression vector pVAX1 (Invitrogen, V260–20) under the control of the cytomegalovirus immediate-early promoter and these constructs were named as p6E6E7 or p11E6E7. The HA tag was introduced at the 3′end of the gene to facilitate analysis of expression.
In vivo expression of p6E6E7 and p11E6E7
293-T cells were cultured in 6-well plates and transfected with pVAX, p6E6E7, or p11E6E7 using FuGENE6 Transfection Reagent (Roche Applied Science, 11815091001). Two days after transfection, the cells were lysed using Modified RIPA cell lysis buffer and cell lysate was collected. The western blot analysis were performed with an anti-HA monoclonal antibody (Sigma-Aldrich, H3663) and visualized with horseradish peroxidase-conjugated goat anti-mouse IgG (GE Healthcare, NA931V) using an ECLTM western blot analysis system (GE Healthcare, RPN2132).
Indirect immunofluorescence assays were performed using human rhabdomyosarcoma cells (RD) to verify expression of p6E6E7 and p11E6E7. RD cells cultured in chamber slides were transfected with pVAX, p6E6E7, or p11E6E7 using Turbofectin 8.0 (Origene, TF81001). Afterwards, the cells were fixed with PFA and permeabilized with 0.1% Triton-X in PBS. The cells were subjected to 1–2 h incubations with primary and secondary antibodies in addition to washes with 0.15% Glycine and 0.5% BSA in PBS during each step. The primary and secondary antibodies used were monoclonal mouse anti-HA (Sigma-Aldrich, H3663) and FITC-conjugated anti-mouse IgG (Abcam, ab6785), respectively. Hoechst staining was also performed to identify cell nuclei. After all incubations were completed, the cells were mounted with Fluoromount-G (Southern Biotech, 0100–01). The samples were imaged using a confocal microscope (CDB Microscopy Core, University of Pennsylvania Cell and Developmental Biology).
Mice and treatment groups
Female C57BL/6 mice between 6 to 8 weeks old were used in this experiment. Mice were obtained from the Jackson Laboratory. The mice were housed and maintained by the University Laboratory Animal Resources at the University of Pennsylvania in observance with the policies of the National Institutes of Health and the University of Pennsylvania Institutional Animal Care and Use Committee (IACUC). The mice used in these experiments were separated into groups of five for immunization. Mice were immunized with p6E6E7, p11E6E7, or both constructs and pVAX group served as negative control.
DNA vaccination and electroporation
Each mouse received three doses of 20µg of each DNA plasmid at 14-d intervals. Mice in the group receiving both p6E6E7 and p11E6E7 together received 20µg of each plasmid for a total of 40µg of DNA per vaccination. The DNA constructs were administered via intramuscular injection of the right quadriceps muscle, followed by square-wave pulses generated by the CELLECTRA® constant current electroporation device (Inovio Pharmaceuticals). The device was configured to deliver two 0.1 Amp pulses of 52ms pulse width spaced apart by a 1 sec delay. Electroporation procedure was performed as described previously.Citation12,Citation13
IFN-γ ELISpot assay
Mice in both treatment and control groups were sacrificed 1 week after the third immunization. Spleens were harvested from each mouse and transferred to R10 media (RPMI-1640 medium supplemented with 10% fetal bovine serum and 1% antibiotics). Using a stomacher (Seward Laboratory Systems), the spleens were pulverized and subsequently transferred through a cell strainer. Erythrocytes were removed by adding ACK lysing buffer (Lonza, 10–548E). The splenocytes were isolated and resuspended in R10 media. High-protein IP 96-well Multiscreen™ plates (Millipore, S2EM004M99) were coated with monoclonal mouse IFN-γ Capture Antibody (R&D Systems, SEL485) and incubated overnight at 4°C. After three washes with 1 X PBS, the plates were blocked with 1% BSA and 5% sucrose in 1 x PBS for 2 h at ambient temperature. Isolated splenocytes in R10 medium were counted and added in triplicate wells at 2 x 105 cells per well. Two sets of peptides spanning the consensus E6/E7 sequence for HPV6 and HPV11 were synthesized by GenScript. The peptides contained 15 amino acid sequences, of which 8 residues overlapped with each sequential peptide. The peptides for HPV6 and 11 were each divided into two pools – one pool for E6 and another for E7 – at concentrations of 2µg/mL in DMSO. Wells reserved for positive and negative control received Concanavalin A (Sigma-Aldrich, C0412) and R10 culture medium in lieu of peptides, respectively. Plates were subsequently placed in a 5% CO2 atmosphere incubator. After incubation for 18–24 h at 37°C, the wells were washed with 1 x PBS. Biotinylated anti-mouse IFN-γ Detection Antibody (R&D Systems, SEL485) was added to each well and then incubated overnight at 4°C. The plates were subsequently washed and processed per a color development protocol provided by R&D Systems using Streptavidin-AP and BCIP/NBT Plus (R&D Systems, SEL002). The wells were air-dried overnight and spots inside wells were scanned and counted by an ELISpot plate reader system with ImmunoSpot®3 and ImmunoSpot®4 software (Cellular Technology Ltd.). Reported spot-forming cell counts were converted to represent spot-forming units per 1 x 106 splenocytes using arithmetic.
Epitope mapping
Epitope mapping studies were performed to determine dominant epitopes within peptide pools. The studies were performed similarly to the previously mentioned IFN-γ ELISpot assay. Instead of pools, individual peptides were used to stimulate the splenocytes.
Intracellular cytokine staining
Splenocytes from vaccinated and naive mouse groups were isolated and stimulated with peptides spanning the E6 and E7 regions of HPV6 and HPV11 for 4 h at 37°C in a 5% CO2 environment. Positive and negative controls were used in the assay by placing cells in phorbol 12-myristate 13-acetate (PMA) and R10 media, respectively. After incubation, the cells were first stained with ViViD Dye (LIVE/DEAD® Fixable Violet Dead Cell Stain kit; Invitrogen, L34955) to differentiate between live and dead cells, then all cells were stained with the following surface marker antibodies: APC-Cy7 Hamster anti-Mouse CD3e (BD Biosciences, 557596), PerCP-Cy5.5 Rat anti-Mouse CD4 (BD Biosciences, 550954), and APC Rat anti-Mouse CD8a (BD Biosciences, 553035). The cells were subsequently fixed using the Cytofix/Cytoperm kit (BD Biosciences, 554714). After fixation per manufacturer protocol, the cells were stained with the following intracellular marker antibodies: Alexa Fluor 700 Rat anti-Mouse IFN-γ (BD Biosciences, 557998), PE-Cy7 Rat anti-Mouse TNF-α (BD Biosciences, 557644), and PE Rat anti-Mouse IL-2 (BD Biosciences, 554428). After staining, the cells were fixed with 2% paraformaldehyde (Electron Microscopy Sciences, 15710). The prepared cells were acquired using an LSR II flow cytometer equipped with BD FACSDiva software (BD Biosciences). Acquired data was analyzed using the FlowJo software version 7.6.3 (Tree Star). CD4+ and CD8+ events were isolated using the following sequence of gates: singlet from FSC-A vs FSC-H, all splenocytes from FSC-A vs SSC-A, live cells from ViViD Dye (Pacific Blue) vs SSC-A, CD3+ cells from CD3 (APC-Cy7) vs SSC-A, and CD4+ or CD8+ from CD4 (PerCP-Cy5.5: positive – CD4+, negative – CD8+) vs SSC-A. The last two populations were gated against Alexa Fluor 700, PE-Cy7, and PE to observe changes in IL-2, IFN-γ, and TNF-α production, respectively.
Statistical Analysis
Student’s t-test was applied for comparison of the quantitative data. In this study, p < 0.05 has been considered statistically significant.
Abbreviations: | ||
HPV | = | human papillomavirus |
EP | = | in vivo electroporation |
ELISpot | = | enzyme-linked immunospot assay |
SFU | = | spot forming units |
IFN-γ | = | interferon γ |
IL-2 | = | interleukin 2 |
TNF-α | = | tumor necrosis factor α |
IL-28B/IFN-λ3 | = | interleukin 28B/interferon λ3 |
MHC | = | major histocompatibility complex |
kDa | = | kilodalton |
Disclosure of Potential Conflicts of Interest
DBW notes that he and his laboratory has several commercial relationships with companies in the area of vaccines. These include that he receives consulting fees, or received stock ownership, for Advisory Board/ Review Board Service,or received speaking support or research support from commercial entities including: Inovio, BMS, VGXI, Pfizer, Virxsys, J and J, Merck, Sanofi Pasteur, Althea, Novo Nordisk, SSI, Aldevron, Novartis, Incyte and possibly others. No writing assistance was utilized in the production of this manuscript.
References
- WHO/ICO HPV Information Centre. Human Papillomavirus and Related Cancers in World. Summary Report 2010. WHO. [ www. who. int/hpvcentre] Accessed on 8 July 2011.
- Muñoz N, Bosch FX, Castellsagué X, Díaz M, de Sanjose S, Hammouda D, et al. Against which human papillomavirus types shall we vaccinate and screen? The international perspective. Int J Cancer 2004; 111:278 - 85; http://dx.doi.org/10.1002/ijc.20244; PMID: 15197783
- Lin HW, Richmon JD, Emerick KS, de Venecia RK, Zeitels SM, Faquin WC, et al. Malignant transformation of a highly aggressive human papillomavirus type 11-associated recurrent respiratory papillomatosis. Am J Otolaryngol 2010; 31:291 - 6; http://dx.doi.org/10.1016/j.amjoto.2009.02.019; PMID: 20015762
- Bonagura VR, Hatam LJ, Rosenthal DW, de Voti JA, Lam F, Steinberg BM, et al. Recurrent respiratory papillomatosis: a complex defect in immune responsiveness to human papillomavirus-6 and -11. APMIS 2010; 118:455 - 70; http://dx.doi.org/10.1111/j.1600-0463.2010.02617.x; PMID: 20553528
- Donne AJ, Hampson L, Homer JJ, Hampson IN. The role of HPV type in Recurrent Respiratory Papillomatosis. Int J Pediatr Otorhinolaryngol 2010; 74:7 - 14; http://dx.doi.org/10.1016/j.ijporl.2009.09.004; PMID: 19800138
- Combita AL, Touzé A, Bousarghin L, Christensen ND, Coursaget P. Identification of two cross-neutralizing linear epitopes within the L1 major capsid protein of human papillomaviruses. J Virol 2002; 76:6480 - 6; http://dx.doi.org/10.1128/JVI.76.13.6480-6486.2002; PMID: 12050360
- Hildesheim A, Herrero R, Wacholder S, Rodriguez AC, Solomon D, Bratti MC, et al, Costa Rican HPV Vaccine Trial Group. Effect of human papillomavirus 16/18 L1 viruslike particle vaccine among young women with preexisting infection: a randomized trial. JAMA 2007; 298:743 - 53; http://dx.doi.org/10.1001/jama.298.7.743; PMID: 17699008
- Lin K, Roosinovich E, Ma B, Hung CF, Wu TC. Therapeutic HPV DNA vaccines. Immunol Res 2010; 47:86 - 112; http://dx.doi.org/10.1007/s12026-009-8141-6; PMID: 20066511
- Gissmann L. HPV vaccines: preclinical development. Arch Med Res 2009; 40:466 - 70; http://dx.doi.org/10.1016/j.arcmed.2009.07.002; PMID: 19853186
- Kanodia S, Da Silva DM, Kast WM. Recent advances in strategies for immunotherapy of human papillomavirus-induced lesions. Int J Cancer 2008; 122:247 - 59; http://dx.doi.org/10.1002/ijc.23252; PMID: 17973257
- Santin AD, Bellone S, Palmieri M, Zanolini A, Ravaggi A, Siegel ER, et al. Human papillomavirus type 16 and 18 E7-pulsed dendritic cell vaccination of stage IB or IIA cervical cancer patients: a phase I escalating-dose trial. J Virol 2008; 82:1968 - 79; http://dx.doi.org/10.1128/JVI.02343-07; PMID: 18057249
- Yan J, Harris K, Khan AS, Draghia-Akli R, Sewell D, Weiner DB. Cellular immunity induced by a novel HPV18 DNA vaccine encoding an E6/E7 fusion consensus protein in mice and rhesus macaques. Vaccine 2008; 26:5210 - 5; http://dx.doi.org/10.1016/j.vaccine.2008.03.069; PMID: 18455277
- Yan J, Reichenbach DK, Corbitt N, Hokey DA, Ramanathan MP, McKinney KA, et al. Induction of antitumor immunity in vivo following delivery of a novel HPV-16 DNA vaccine encoding an E6/E7 fusion antigen. Vaccine 2009; 27:431 - 40; http://dx.doi.org/10.1016/j.vaccine.2008.10.078; PMID: 19022315
- Kim D, Hoory T, Monie A, Ting JP, Hung CF, Wu TC. Enhancement of DNA vaccine potency through coadministration of CIITA DNA with DNA vaccines via gene gun. J Immunol 2008; 180:7019 - 27; PMID: 18453624
- Best SR, Peng S, Juang CM, Hung CF, Hannaman D, Saunders JR, et al. Administration of HPV DNA vaccine via electroporation elicits the strongest CD8+ T cell immune responses compared to intramuscular injection and intradermal gene gun delivery. Vaccine 2009; 27:5450 - 9; http://dx.doi.org/10.1016/j.vaccine.2009.07.005; PMID: 19622402
- Tseng CW, Trimble C, Zeng Q, Monie A, Alvarez RD, Huh WK, et al. Low-dose radiation enhances therapeutic HPV DNA vaccination in tumor-bearing hosts. Cancer Immunol Immunother 2009; 58:737 - 48; http://dx.doi.org/10.1007/s00262-008-0596-0; PMID: 18815785
- Seo SH, Jin HT, Park SH, Youn JI, Sung YC. Optimal induction of HPV DNA vaccine-induced CD8+ T cell responses and therapeutic antitumor effect by antigen engineering and electroporation. Vaccine 2009; 27:5906 - 12; http://dx.doi.org/10.1016/j.vaccine.2009.07.033; PMID: 19651174
- Ohlschläger P, Quetting M, Alvarez G, Dürst M, Gissmann L, Kaufmann AM. Enhancement of immunogenicity of a therapeutic cervical cancer DNA-based vaccine by co-application of sequence-optimized genetic adjuvants. Int J Cancer 2009; 125:189 - 98; http://dx.doi.org/10.1002/ijc.24333; PMID: 19358269
- Trimble CL, Peng S, Kos F, Gravitt P, Viscidi R, Sugar E, et al. A phase I trial of a human papillomavirus DNA vaccine for HPV16+ cervical intraepithelial neoplasia 2/3. Clin Cancer Res 2009; 15:361 - 7; http://dx.doi.org/10.1158/1078-0432.CCR-08-1725; PMID: 19118066
- Wu A, Zeng Q, Kang TH, Peng S, Roosinovich E, Pai SI, et al. Innovative DNA vaccine for human papillomavirus (HPV)-associated head and neck cancer. Gene Ther 2011; 18:304 - 12; http://dx.doi.org/10.1038/gt.2010.151; PMID: 20981112
- Peng S, Trimble C, Alvarez RD, Huh WK, Lin Z, Monie A, et al. Cluster intradermal DNA vaccination rapidly induces E7-specific CD8+ T-cell immune responses leading to therapeutic antitumor effects. Gene Ther 2008; 15:1156 - 66; http://dx.doi.org/10.1038/gt.2008.53; PMID: 18401437
- Huang CF, Monie A, Weng WH, Wu T. DNA vaccines for cervical cancer. Am J Transl Res 2010; 2:75 - 87; PMID: 20182584
- Morrow MP, Pankhong P, Laddy DJ, Schoenly KA, Yan J, Cisper N, et al. Comparative ability of IL-12 and IL-28B to regulate Treg populations and enhance adaptive cellular immunity. Blood 2009; 113:5868 - 77; http://dx.doi.org/10.1182/blood-2008-11-190520; PMID: 19304955
- Morrow MP, Yan J, Pankhong P, Shedlock DJ, Lewis MG, Talbott K, et al. IL-28B/IFN-lambda 3 drives granzyme B loading and significantly increases CTL killing activity in macaques. Mol Ther 2010; 18:1714 - 23; http://dx.doi.org/10.1038/mt.2010.118; PMID: 20571540
- Bonnez W. Papilloma Virus.In: Richman D, Whitley R, Hayden F, eds. Clinical Virology. 2nd ed. Washington DC: American Society for Microbiology Press, 2002:557-96.
- Peng S, Best SR, Hung CF, Loyo M, Lyford-Pike S, Flint PW, et al. Characterization of human papillomavirus type 11-specific immune responses in a preclinical model. Laryngoscope 2010; 120:504 - 10; http://dx.doi.org/10.1002/lary.20745; PMID: 20025042
- Jeong WJ, Park SW, Shin M, Lee YJ, Jeon YK, Jung YH, et al. Presence of HPV type 6 in dysplasia and carcinoma arising from recurrent respiratory papillomatosis. Head Neck 2009; 31:1095 - 101; http://dx.doi.org/10.1002/hed.20998; PMID: 19072996