Abstract
Rapid production of influenza vaccine antigen is an important challenge when a new pandemic occurs. Production of recombinant antigens in plants is a quick, cost effective and up scalable new strategy for influenza vaccine production. In this study, we have characterized a recombinant influenza haemagglutinin antigen (HAC1) that was derived from the 2009 pandemic H1N1 (pdmH1N1) virus and expressed in tobacco plants. Volunteers vaccinated with the 2009 pdmH1N1 oil-in-water adjuvanted vaccine provided serum and lymphocyte samples that were used to study the immunogenic properties of the HAC1 antigen in vitro. By 7 d post vaccination, the vaccine fulfilled the licensing criteria for antibody responses to the HA detected by haemagglutination inhibition and single radial hemolysis. By ELISA and ELISPOT analysis we showed that HAC1 was recognized by specific serum antibodies and antibody secreting cells, respectively. We conducted a kinetic analysis and found a peak of serum HAC1 specific antibody response between day 14 and 21 post vaccination by ELISA. We also detected elevated production of IL-2 and IFNγ and low frequencies of CD4+ T cells producing single or multiple Th1 cytokines after stimulating PBMCs (peripheral blood mononuclear cells) with the HAC1 antigen in vitro. This indicates that the antigen can interact with T cells, although confirming that an effective adjuvant would be required to improve the T-cell stimulation of plant based vaccines. We conclude that the tobacco derived recombinant HAC1 antigen is a promising vaccine candidate recognized by both B and T cells.
Introduction
The recent 2009 influenza pandemic clearly highlighted the limitations of the currently used egg-based influenza vaccine production system, in terms of producing enough vaccine antigen for the global market in a limited time window. Future strategies for pandemic vaccine manufacturing should therefore focus on increasing capacity and reducing cost and time required for antigen production. Several new technologies are under investigation and recombinant expression systems for vaccine antigen production are promising. Expression of antigen in tobacco plants is a rapid and up scalable system resulting in high concentration of antigen with a eukaryotic glycosylation pattern.Citation1-Citation3
Most pandemic influenza vaccine trials have concentrated on the highly pathogenic avian H5N1 viruses as these are continuing to cause zoonosis in humans. The 2009 pandemic was therefore an important reminder that pandemic viruses might well originate from other subtypes and zoonotic origins. Most H5 antigens elicit a poor immune response in man and need a strong immunological adjuvant and at least one booster dose to reach protective antibody titers equivalent to those required against seasonal influenza strains.Citation4-Citation7 The 2009 pandemic H1N1 (pdmH1N1) vaccine antigen was highly immunogenic, egg-based vaccines providing protection after a single dose in adults and children over ten years of age,Citation8-Citation13 hence providing a better opportunity to study the immunogenicity of next generation pandemic vaccines in man.
Antigens produced in tobacco plants have previously been shown to be immunogenic in animal models when combined with a suitable adjuvant.Citation3,Citation14-Citation17 The ability of these proteins to be recognized by the human immune system has however been poorly explored.
In 2009 we conducted a clinical trial vaccinating frontline healthcare workers with oil-in-water, (AS03) adjuvanted, low dose pdmH1N1 vaccine.Citation13 In the present study we have evaluated a recombinant haemagglutinin (HA) antigen (HAC1) derived from the 2009 pdmH1N1 virus (A/California/4/2009), produced in tobacco plants. We used serum and lymphocytes from the 2009 study to investigate the recognition of the HAC1 antigen by human pdmH1N1 specific antibodies and to investigate the ability of HAC1 to be recognized by B and T lymphocytes in vitro. As such this study is a first step in taking these new generation vaccines into human clinical trials.
Results
We have utilized in vitro immunological assays for evaluating a recombinant influenza HA protein (HAC1) as a potential human vaccine candidate. The HAC1 antigen contained amino acids 18–530 of the influenza surface glycoprotein, HA, derived from the pdmH1N1 virus A/California/4/2009 (H1N1). The antigen was expressed recombinantly in tobacco plants using plant virus vectors. Serum and lymphocytes from 23 individuals was used for the immunological assays. The individuals were healthcare workers participating in a clinical study evaluating the safety and immunogenicity of the oil-in-water adjuvanted low-dose split virus vaccine against the pdmH1N1 virus.Citation13
Study group demographics
The study group consisted of 20 women and three men, reflecting the female dominance among Norwegian healthcare workers (). The volunteers were between 25 and 64 y of age (mean age 50.2 y) when vaccinated between October 22nd and 27th 2009, two to three weeks prior to the peak in pdmH1N1 activity. Eight volunteers were concurrently immunized with the 2009 seasonal vaccine and 18 subjects had a history of previous seasonal influenza vaccination. Four volunteers had experienced influenza symptoms during the past 12 mo prior to vaccination, but none of the subjects had experienced clinical symptoms of pdmH1N1. Two volunteers reported taking an anti-influenza drug (Tamiflu) prophylactically during the 20 d post vaccination period. Two volunteers reported that they had underlying respiratory disease () and were thus in the high-risk group for influenza vaccination.
Table 1. Demographics of the enrolled volunteers
Vaccine-induced solicited adverse events
Vaccinees completed a diary card, registering any adverse events occurring during a 20 d post vaccination period and grading them in terms of severity. No serious adverse events were reported although the frequency of adverse events was high (). Twenty-two of the 23 vaccinees (95.6%) reported experiencing an adverse event of mild to moderate severity and no one reported any side reactions later than seven days after vaccination. Local side reactions were reported by 21 (91.3%) of the vaccinees, the most common being pain at the injection site experienced by 20 individuals (87.0%). Fourteen individuals (63.6%) experienced both systemic and local reactions after vaccination and only one individual (4.3%) reported only systemic reactions. The most common systemic reactions were headache and fatigue reported by 10 (43.5%) and 9 (39.1%) individuals, respectively. In summary the vaccine was well tolerated by the vaccinees, it had a good safety profile and no severe adverse reactions were observed.
Figure 1. Solicited adverse events after vaccination in the enrolled volunteers. The frequency of local and systemic adverse events for volunteers included in the study. Data are based on diary cards completed by the 23 subjects in the study. “Any” refers to the report of an adverse event on one or more days after vaccination.
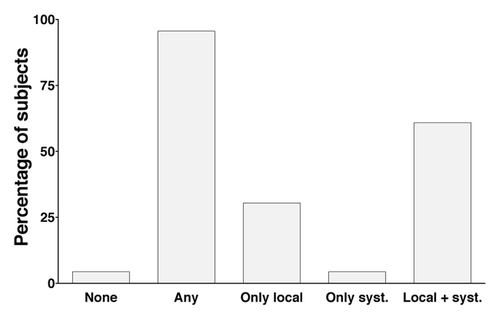
Vaccine immunogenicity
The Committee for Medicinal Products for Human Use (CHMP) has defined a set of criteria for evaluating seasonal and pandemic influenza vaccine efficacy, defining single radial hemolysis (SRH) areas of ≥ 25 mm2 and haemagglutination inhibition (HI) titers ≥ 40 as protective in humans. We investigated the level of surrogate protective antibodies to the vaccine antigen in the serum of our vaccinees according to these criteria. Three and two individuals in the study had pre-existing immunity to the vaccine antigen prior to vaccination, as measured by SRH and HI assays, respectively. This probably reflects that the volunteers were all frontline healthcare workers in the hospital. Already seven days post vaccination, protective antibody titers were observed in 14 and 12 of the 17 available serum samples by SRH and HI assays, respectively, and by 14 d post vaccination this had increased to 16 and 15, out of 16 subjects (). At seven days post vaccination the geometric mean area (GMA) for SRH was 35 mm2 and the geometric mean HI titer (GMT) was 86. At 14 d post vaccination GMA had increased to 57 mm2 and continued to increase to 66 mm2 on day 21. For HI, the GMT increased to 272 at 14 d and remained stable at 21 d (GMT 277). A Spearman correlation test showed a good correlation between the HI and SRH data for each volunteer (r = 0.7313 and p < 0.0001) at day 21 post vaccination.
Figure 2. Immunogenicity of the pdmH1N1 vaccine. (A) serum SRH titers for the enrolled volunteers pre-vaccination, and 7, 14, 21 d (d) post vaccination. (B) serum HI titers for the enrolled volunteers pre-vaccination, and 7, 14, 21 d (d) and 3, 6 and 12 mo (m) post vaccination. Each symbol in A and B represents one individual serum sample and lines indicate geometric mean area (GMA) or geometric mean titer (GMT) ± 95% confidence interval. The number of sera (n) included at each time point is indicated. (C) The reverse cumulative distribution curves for the HI antibody response. The HI data was standardized according to a conversion factor based on the GMT of the Candidate International Standard (09/194) in a collaborative study, as the Candidate International Standard has not been assigned International Units. Dotted lines indicate protective levels as defined by the Committee for Medicinal Products for Human Use (CHMP).
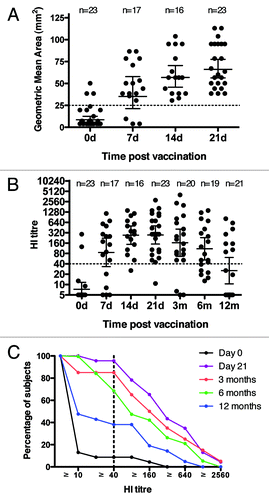
The longevity of the antibody response was measured by the HI assay. HI titers had fallen slightly at 3 mo post pdmH1N1 vaccination (GMT 164) and, for three individuals, titers were below the protective levels at three months. The HI titers continued to decrease by 6 mo (GMT = 109) and further by 12 mo (GMT = 25) and at these two last time points 68.4% and 38.0% of subjects still had protective HI titers (). Of the three subjects who were below protective HI antibody levels at three months, two were not sampled at 6 mo while one had a low but detectable HI titer (13) at 6 mo.
One subject did not reach a protective HI antibody titer after the first vaccination and was therefore given a booster dose of vaccine after the three-month blood sample. This person did, however, reach a protective SRH antibody titer both at 14 and 21 d post vaccination. This could reflect an increased sensitivity of the SRH assay in measuring low antibody responses.
Overall, the study group responded well to the vaccine as evaluated by the CHMP criteria.
The HAC1 protein was recognized by circulating IgG and IgA secreting B cells
The enzyme linked immunospot (ELISPOT) assay was used to investigate HAC1 recognition by specific antibody secreting B cells (ASCs) from 15 volunteers 7 d post vaccination and compared with the recognition of the vaccine antigen as a positive control. The number of ASCs producing each Ig class was enumerated per 100,000 peripheral blood mononuclear cells (PBMCs). We found that IgG (mean = 64.6) and IgA (mean = 52.7) but not IgM (mean = 1.2) secreting ASCs detected the HAC1 protein in vitro as shown in . In agreement with previous findings,Citation18 experiments on cells isolated from non-vaccinated individuals and on days 14 and 21 post vaccination showed very low numbers of HAC1 reactive ASCs per sample (mean numbers between 0 and 1, and no single measurement above 6 in 100,000 PBMCs) this was also similar to the results observed for the vaccine antigen (results not shown).
Figure 3. Human B-cells detecting HAC1. Human peripheral blood mononuclear cells (PBMCs) isolated 7 d post vaccination were analyzed by ELISPOT to identify antibody secreting cells (ASCs) recognizing the HAC1 antigen or the vaccine antigen. For each sample the number of B-cells secreting specific antibodies of the IgG, IgA and IgM classes were quantified in 100,000 PBMCs. Each symbol represents one individual volunteer. Lines indicate the mean ± standard error of the mean (SEM). n = 15
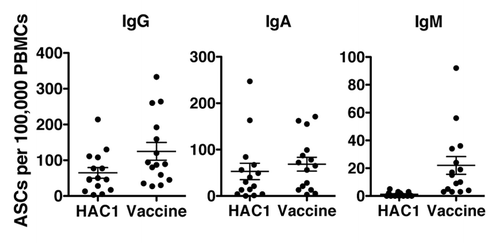
A non-parametric correlation test (Spearman's test) also showed significant correlation between numbers of IgA secreting ASCs detecting HAC1 and the positive control (r = 0.6869, p = 0.0047) while the correlation for IgG secreting ASCs was not significant (r = 0.4844, p = 0.0673).
The HAC1 protein was detected by serum antibodies
The kinetics of the serum antibodies recognizing HAC1 after vaccination was investigated by Enzyme linked immunosorbent assay (ELISA) (). The level of serum IgG antibodies interacting with HAC1 was investigated prior to vaccination and 7, 14 and 21 d and 3, 6 and 12 mo post vaccination. Prior to vaccination the antibody level was low (mean = 12.6 μg/ml), it increased on day 7 post vaccination (mean = 70.8 μg/ml) and reached its peak between 14 d (mean = 157.1 μg/ml) and 21 d (mean = 158.5 μg/ml) post vaccination. The mean response then gradually declined throughout the rest of the sampling period (3 mo = 70.9 μg/ml, 6 mo = 62.0 μg/ml, 12 mo = 44.8 μg/ml).
Figure 4. Human serum antibodies detecting HAC1. HAC1 detecting IgG antibodies were measured by ELISA on serum collected pre vaccination and at 7, 14 and 21 d (d) and 3, 6 and 12 mo (m) post vaccination. Each symbol represents one individual serum sample. Lines represent the mean ± standard error of the mean (SEM). ** and *** indicate concentrations significantly different from day 0 with p < 0.005 and p < 0.0005, respectively. The number of sera (n) included at each time point is indicated.
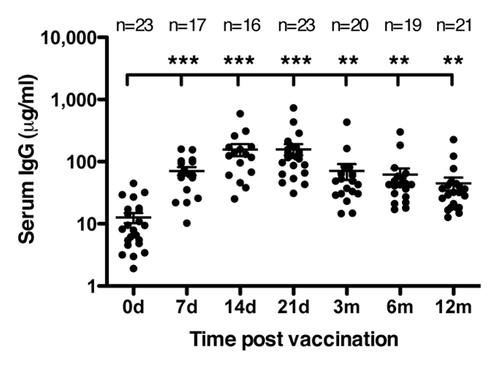
The increase in HAC1 detecting antibodies was statistically significant at all time points compared with pre vaccination concentrations. The peak response between day 14 and 21 reflected the rapid response as measured by HI and SRH () and clearly showed that the HAC1 protein was well recognized by human influenza specific serum antibodies. The ELISA results further appeared to follow the same trend as the HI results although there was no statistical significant correlation of the HI and ELISA results for each volunteer according to the Spearman't test.
The HAC1 protein induced low levels of cytokine secretion from PBMCs isolated 21 days post vaccination
The ability of HAC1 to induce cytokine secretion in pdmH1N1 specific human PBMCs in vitro was measured by multiplex cytokine assay of 10 different cytokines. PBMCs from 19 volunteers isolated 21 d post vaccination were stimulated in vitro with the HAC1 protein or vaccine antigen (positive control) or medium alone (negative control). The time post vaccination was chosen based on previous time studies of in vitro stimulation.Citation19 From it is evident that, although some of the human PBMC samples secreted high levels of cytokines after in vitro stimulation with the HAC1 protein, a number of samples did not respond to the HAC1 stimulation. By statistical analysis only IL-2 (p = 0.0243) and IFNγ (p = 0.0025) were significantly higher than the negative controls (). This indicates that although the protein seems to be well recognized by B-cells and their secreted antibodies, there are limitations as to the ability of the HAC1 protein alone to induce cytokine stimulation in human PBMCs in vitro. In comparison, the positive control elicited significantly higher cytokine secretion for the cytokines IL-2, IFNγ, IL-5, IL-10, IL-17, IL-13, GM-CSF and TNFα (p < 0.05) but not for IL-4 and IL-12. Even though HAC1 did not significantly stimulate the secretion of all these cytokines for the study group as a whole, for each cytokine there were nearly always a few individuals with elevated secretion indicating that HAC1 has the potential to also induce these cytokines but that further immunostimulatory agents might be needed when used as a vaccine. There was no significant correlation between the results obtained with HAC1 and the vaccine antigen for IL-2 and IFNγ, this could also be due to the poor stimulation by HAC1.
Figure 5. In vitro induction of cytokine secretion from human PBMCs by HAC1. Human PBMCs isolated 21 d post vaccination were stimulated in vitro with HAC1 or the vaccine antigen or left non-stimulated (Neg) for 3 d and the level of 10 different cytokines was measured in the cell supernatant by multiplex cytokine assay. Each symbol represents one individual volunteer. Bars represent the mean ± standard error of the mean (SEM). * and ** indicate significant difference with p < 0.05 and p < 0.005, respectively. n = 19.
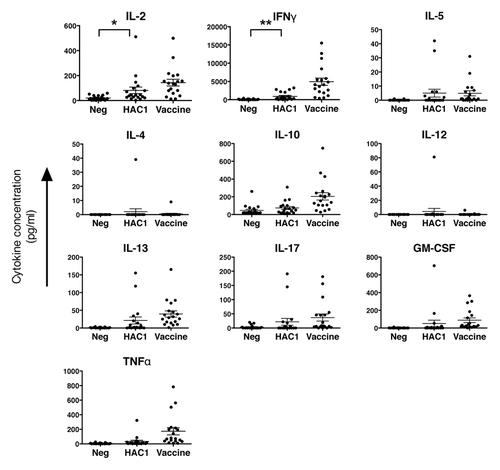
We examined if the same individuals were high expressers of each cytokine after stimulation. For HAC1 stimulation there did not seem to be any clear correlation as samples expressing high levels of one cytokine could express no or very low levels of another cytokine. On the other hand, samples expressing low levels of IFNγ generally also expressed very low or undetectable levels of the other cytokines investigated. For stimulation with the vaccine antigen, on the other hand, there seemed to be a good correlation, according to the spearman test, between the level of IFNγ expression and IL-10 (r = 0.7456, p = 0.0002), TNFα (r = 0.9140, p < 0.0001) and GM-CSF (r = 0.8416, p < 0.0001). The correlations after HAC1 stimulation may of course be less obvious due to no or low cytokine stimulation in many of the samples.
Multifunctional CD4+ T cells were stimulated by the HAC1 protein in vitro
We also investigated the ability of the HAC1 protein to stimulate intracellular cytokine production in CD4+ and CD8+ T cells in vitro. We used PBMCs isolated from 19 vaccinees at 21 d post vaccination. The time point post vaccination was chosen to allow for comparison with reports in the literature in which CD4+ T cells responses have also been measured at day 21.Citation20,Citation21 The samples were stimulated with HAC1, the vaccine antigen or medium alone and subsequently analyzed for CD4+ and CD8+ T cells expressing the cytokines IFNγ, IL-2 and TNFα by multiparameter flow cytometry. The number of CD4+ lymphocytes expressing each of these cytokines was significantly elevated after HAC1 stimulation compared with medium alone (IFNγ, p = 0.0012; IL-2, p < 0.0001; TNFα, p < 0.0001) (). We also found a significant correlation between the samples expressing elevated levels of each cytokine in response to HAC1 and the vaccine antigen, indicating that high responders recognized HAC1 (IFNγ, r = 0.7193, p = 0.0005; IL-2, r = 0.8811, p < 0.0001; TNFα, r = 0.6702, p = 0.0017). In contrast to CD4+ T cells, we did not see activation of CD8+ T cells in response to HAC1 or the vaccine antigen at this time point (data not shown). Previously published studies have shown that AS03 adjuvanted H5N1Citation20 and H1N1Citation21 vaccines induce an increase in the frequencies of influenza-specific CD4+ Th1 cells but not CD8+ T-cell responses.
Figure 6. HAC1 activation of multifunctional T cells. Human PBMCs isolated 21 d post vaccination were stimulated in vitro with HAC1 or the vaccine antigen or left non-stimulated (Neg) overnight. Cells were then fixed and analyzed for the presence of multifunctional T cells producing one or more of the cytokines TNFα, IL-2 and IFNγ by multi-parametric flow cytometry. (A) Total frequency of CD4+ cells producing each of the cytokines investigated. (B) Frequency of CD4+ cells producing only one, any two or all three of the cytokines investigated. *, ** and *** indicate significant difference with p < 0.05, p < 0.005 and p < 0.0005, respectively. Bars represent the mean ± standard error of the mean (SEM). n = 19.
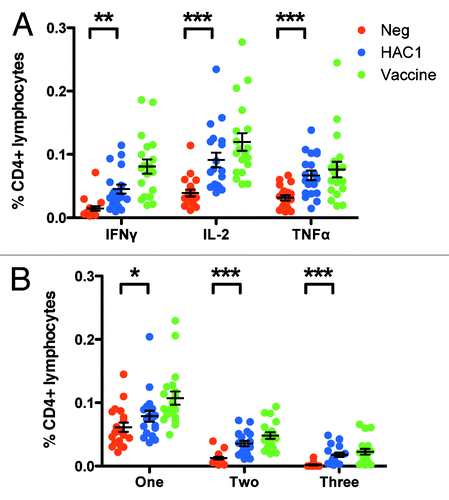
By this method we were also able to investigate the quality of the T-cell response as indicated by the frequency of multifunctional CD4+ Th1 cells, producing more than one cytokine simultaneously. This parameter has been associated with vaccine protectionCitation22-Citation24 and is shown to be an integral part of the response to influenza vaccination in murine models and in man.Citation25-Citation27 We measured the frequency of CD4+ cells producing one, any two or all three of the cytokines TNFα, IL-2 and IFNγ simultaneously. We found a significant increase both in single producing (p = 0.0136, mean increased from 0.061 to 0.079%), double producing (p < 0.0001, mean increased from 0.013 to 0.036%) and triple producing (p = 0.0004, mean increased from 0.002 to 0.018%) T cells after stimulation with the HAC1 protein (). There was also a good correlation in multifunctional cells stimulated by HAC1 and the vaccine antigen (single producers, r = 0.7368, p = 0.0003; double producers, r = 0.9298, p < 0.0001; triple producers, r = 0.7474, p = 0.0002).
Discussion
Expressing recombinant HA antigens in tobacco plants is a highly promising next generation vaccine production system, shown to be rapid, cost effective and up scalable.Citation1-Citation3
The HAC1 protein as well as haemagglutinin plant antigens derived from seasonal strains (H3N2) and three different H5N1 viruses have previously been tested as vaccine candidates in animal models showing good immune responses in mice and ferrets.Citation3,Citation14-Citation16 Medicago, Canada, have shown that plant produced influenza virus-like particles (VLPs) can induce protective immunity in mice and ferrets and show promising results in a phase one clinical trial.Citation28,Citation29 Together these studies demonstrate the potential for plants as biofactories for influenza antigens. The present study is the first to investigate the ability of human B and T cells to recognize recombinant influenza antigens produced in tobacco plants.
During the 2009 influenza pandemic we had access to a unique set of serum and lymphocyte samples, taken at sequential time points post vaccination, from vaccinees who elicited rapid and high protective antibody titers in response to the AS03 adjuvanted pdmH1N1 vaccine.Citation13 These samples provided a unique opportunity to test the interaction between the plant-produced pdmH1N1 specific HA antigen and specific human immune responses.
The HI and SRH titers of the individuals in the study-group reflected the high antibody titers and rapid protection reported for the larger studyCitation13 and were comparable to reports from other pdmH1N1 vaccines and other clinical trials using the AS03 adjuvant.Citation8-Citation12,Citation30,Citation31 The frequency and severity of the solicited adverse effects were also similar to other influenza vaccine trials using the AS03 adjuvant,Citation13,Citation30,Citation31 with transient, local pain at the injection site most commonly observed and no severe adverse events reported.
We found that the HAC1 antigen was well recognized by serum antibodies and antibody secreting cells from individuals in the study-group and that it stimulated cytokine secretion in lymphocytes from the same individuals, although at lower levels than the vaccine antigen. We also showed that HAC1 was able to induce secretion of IL-2 and IFNγ to statistically significant levels by in vitro stimulation of PBMCs from the vaccinated individuals. The in vitro stimulation of multifunctional Th1-cells from pdmH1N1 vaccinated individuals showed that the HAC1 protein was recognized and induced cytokine production. An important question for future studies is if plant produced antigens can induce the maturation of such polyfunctional T cells in man, as recent experiments conducted in mice point to this.Citation26
Importantly we saw a good correlation between the results obtained using the HAC1 antigen and those obtained using the vaccine antigen both in the ELISPOT assay and by intracellular cytokine staining, yet the results obtained for the vaccine antigen were always higher than those of HAC1. The X179a vaccine antigen was used as a homologous positive control commonly used for standard evaluation of the immune response after vaccination. It is important to note that the vaccine antigen is expected to induce a better response in our assays than the purified HA monomers of HAC1. In the ELISA and ELISPOT assays antibodies against the surface protein, neuraminidase (NA), will make a substantial contribution to the recognition of the whole virus vaccine antigen. In the T cell assays, trace viral RNA in the split virus vaccine antigen could induce innate mechanisms through activation of toll-like receptor 7 (TLR7)Citation32,Citation33 and together with recognition of NA and additional internal proteins such as the nucleoprotein (NP) and matrix 1 (M1), this could result in an increased T cell response. However, using the vaccine antigen as a positive control gives an indication of the immunological difference between the two antigens in a vaccination scenario, pointing to the need for an effective adjuvant for plant-based vaccines, especially for inducing a robust T-cell response. Oil-in-water formulated, squalene based compounds such as MF59, AF02 and AS03 are promising new proprietary adjuvants for intramuscular influenza vaccines. Triggering a local damage response from the muscle cells at the injection site, MF59 has been shown to activate macrophages and attract monocytes, resulting in the stimulation of dendrittic cell maturation.Citation34-Citation36 Other oil-in-water formulated adjuvants are likely to function in a similar manner and both AS03 and MF59 have been shown to induce CD4+ T cells after vaccination in humans.Citation37,Citation38 Both these adjuvants have shown good potential for enhancing systemic immune responses in clinical trials both for H5Citation39-Citation42 and pdmH1N1 vaccines.Citation12,Citation13 Another attractive adjuvant is the 3rd generation immunostimulating complex, Matrix M, that recently showed promising results in a phase one clinical trial directed against an avian influenza H5N1 virus performed by our lab.Citation43 The challenge in choosing a good adjuvant for a candidate vaccine today is, however, that all clinically approved adjuvants are proprietary, and hence not necessarily available for testing with a given antigen.
The field of developing targeted adjuvant technologies is still in its infancy, and a number of novel experimental adjuvants are under pre-clinical testing, some favoring alternative routes of administration. The bacterial second messenger, c-di-GMP, has shown a good immunostimulatory potential in particular as a mucosal adjuvant.Citation44,Citation45 We have previously found that intranasal administration of a plant produced H5 antigen together with c-di-GMP in a mouse model resulted in high antibody titers and good cytokine and T-cell responses,Citation26 further demonstrating the ability of plant-produced antigens to induce a robust response in combination with an adjuvant. The c-di-GMP adjuvant is not yet suitable for clinical studies as it has yet to be approved for use in humans but preclinical studies of this and other new generation adjuvants indicate the potential strength of new adjuvant technologies. In addition to the use of adjuvants, the vaccine response could also possibly be enhanced by combining the HAC1 protein with additional plant produced viral antigens such as antigens against NA and perhaps internal proteins such as nucleoprotein (NP) and matrix1 (M1) protein.
We conclude that the HAC1 protein has shown good vaccine potential as it was recognized by both human B- and T cells in vitro and should be combined with a safe and effective adjuvant for testing in clinical trials. Our results imply that the production of antigen in plants is a good strategy for future pandemic influenza vaccine production.
Materials and Methods
Study design
Inclusion of health care workers in the main study, which was a clinical study of pandemic H1N1 (pdmH1N1) split virus, AS03 adjuvanted, vaccine at Haukeland University Hospital, was performed as described in.Citation13 The study was approved by the Regional Committee for Medical Research Ethics, Western Norway (REKVest) and the Norwegian Medicines Agency and is registered in the European Clinical Trials Database (2009-016456-43), and National Institute for Health database Clinical trials.gov (NCT01003288). The exclusion criteria (a history of anaphylaxis or hypersensitivity to vaccines, an oral temperature > 38°C in the preceding 72 h, an acute respiratory infection up to 7 d prior to immunization or virologically confirmed pdmH1N1) were reviewed for each subject before administration of the vaccine and written informed consents were provided from all subjects prior to inclusion in the study. All volunteers were asked to fill in a diary card reporting any adverse effects, their seasonal influenza vaccination status and suspected or confirmed pdmH1N1 infection.
Serum and lymphocytes from 23 healthcare workers were included in the present study. Volunteers were included randomly based on time of vaccination and arrival of lymphocytes in the lab.
Vaccination was performed by mixing one vial of split virus vaccine antigen suspension (strain X179a) with one vial of AS03 emulsion according to the manufacturers instruction. The vaccine was administered intramuscularly (IM) by injecting 0.5 ml of vaccine into the deltoid muscle. Blood samples were collected prior to vaccination, 7, 14, 21 d and 3, 6 and 12 mo post vaccination.
All volunteers were sampled on days 0 and 21, but due to unavailability of some volunteers on certain sampling days and a variation in sampling based on differences in vaccination days, not all volunteers were included in all assays.
Samples were collected on the indicated days except for the day seven samples (one sample collected on day six) and the day 21 samples (one sample collected on day 20 and one on day 25). Three month samples were collected three to four months post vaccination, six month samples were collected six to seven months post vaccination (one sample at six months minus eight days) and 12 mo samples were collected at 12 mo +/− six weeks.
Sample preparation
Lymphocytes were isolated by centrifugation of blood collected in cell preparation tubes (CPT) according to manufacturer’s instructions. Plasma was removed and lymphocytes were washed twice in 15 ml PBS containing 5% fetal bovine serum (FBS). Cells were reconstituted in 1 ml/CPT of lymphocyte medium [RPMI 1640 containing 10% FBS and 1% penicillin, streptomycin and Amphotericin B (PSA)] and used directly in further assays.
Serum samples were prepared by centrifuging clotted blood at 1,500 × g for 10 min. All serum samples were aliquoted and stored at -80°C for subsequent use in the HI, SRH or ELISA assays.
Haemagglutination inhibition (HI) assay
The HI assay was performed as described in.Citation13 In brief, serial 2-fold dilutions of serum treated with four volumes of receptor destroying enzyme (RDE) were prepared from a 1/10 dilution in PBS and incubated with 8 haemagglutinating units of inactivated whole virus X179a [National Institute for Biological Standards and Control (NIBSC), UK] for one hour. Dilutions were further incubated with 0.7% (v/v) turkey erythrocytes for 30 min, and the individual HI titers were read as the reciprocal of the highest dilution at which 50% haemagglutination was inhibited. The geometric mean HI titer (GMT) was calculated for each subject and titers < 10 were assigned a value of 5 for calculation purposes.
Pre and post vaccination serum samples from each individual were tested simultaneously on up to three separate occasions. Positive control sera were included in all assays and consisted of a post infection ferret serum against X179a and a human post pdmH1N1 2009 infection plasma (09/194) prepared at NIBSC as a candidate International Standard for serological assays. The HI titers were standardised according to a conversion factor to allow comparison with other studies, as the Candidate International Standard has not been assigned International Units. This was calculated by dividing the Collaborative Study GMT by the laboratory GMT.
Single radial hemolysis (SRH)
SRH was based on a modified reference method standardized by Schild and colleagues.Citation46 Two different sets of SRH plates were prepared using turkey erythrocytes at final concentration 10%, to which were added 2,000 HAU/ml (Haemagglutinin Units/ml) inactivated whole virus X179a, provided by NIBSC/HPA UK. All samples were heat inactivated at 56°C for 30 min before the test, and 6 µl volumes were added to each well. Antibody titration was made in duplicate in two different assay sets. Diameters of hemolysis area for each sera tested were measured using a Transidyne Calibrating Viewer (Transidyne General Corporation). Both pre and post-vaccination sera were titrated simultaneously. A hemolysis area less than 4 mm2 was considered as seronegative, between 4 and 25 mm2 was considered positive but not protective, sera with areas of hemolysis equal to or higher than 25 mm2 were considered seroprotected, as defined in the CHMP guidelines. Undetectable SRH areas were assigned an area of 4 mm2. In each test a negative control sample and a positive control serum (sheep hyperimmune sera provided from NIBSC) were included.
Expression and preparation of recombinant antigen
HAC1 was expressed recombinantly in tobacco plants using plant virus vectors as described previously.Citation3,Citation14,Citation16 In brief amino acids 18–530 of the HA protein of the A/California/4/09 (H1N1) virus were cloned into a plant virus expression vector and transformed into Agrobacterium tumefaciens together with a helper plasmid. The transformed bacteria were introduced into 6-week-old Nicotiana benthamiana plants by vacuum infiltration. After seven days leaves were homogenized and extracts clarified by centrifugation, the protein was purified by immobilized metal affinity chromatography and anion exchange chromatography. The purity of the HAC1 protein was measured to > 90% by reverse-phase ultra-performance liquid chromatography (RP-UPLC).Citation3
Enzyme linked immunospot (ELISPOT) assay
The ELISPOT assay was conducted as previously describedCitation18 with the following modifications. Ninety-six well ELISPOT plates were coated with inactivated whole virus X179a (2 μg HA/ml) or recombinant HAC1 antigen (2 μg/ml) diluted in PBS overnight at 4°C. After blocking 100,000 PBMCs were added to duplicate wells and incubated at 37°C and 5% CO2 overnight. Secreted antibodies were detected with biotinylated goat anti-human class specific antibody [IgG (Sigma), IgA and IgM (both Southern Biotech)] for two hours at room temperature, followed by detection with extravidin peroxidase. Following development, the number of spots was counted using the ELISPOT reader (ImmunoscanTM) and the ImmunoSpot software (both from Cellular Technology, Ltd.).
Enzyme linked immunosorbent assay (ELISA)
The ELISA assay was performed as previously described.Citation47 In brief wells were coated with 100 µl (0.2 µg/well) recombinant HAC1 protein (or 0.1 µg goat anti-human IgG for the standard) at 4°C overnight. Wells were blocked with 150 µl PBS with 20% NCS at room temperature for one hour. Serum samples and human IgG standard were prepared in two-fold dilutions series and 100 µl was added to each well and incubated for two hours at room temperature. IgG was detected with a biotinylated goat anti-human IgG antibody (Sigma) for 1.5 h at room temperature and detected by extravidin-peroxydase.
Multiplex cytokine detection
We quantified the concentration of cytokines secreted in the supernatants from stimulated peripheral blood mononuclear cells (PBMCs) using Bio-plex cytokine assay (Biorad) according to the manufacturers instructions. In brief cells isolated on day 21 post vaccination were stimulated with 100 µl/well, 10 µg HA/ml of split virus X179a antigen or plant protein (HAC1) for 72 h before supernatants were harvested. A 10-plex kit (Biorad) was used to detect the cytokines IL-2, INF-γ, IL-4, IL-5, IL-10, IL-12, IL-13, GM-CSF, TNFα and IL-17 and plates were read with the Bio-Plex 200 reader using Bio-Plex manager 5.0 software (Biorad).
Intracellular cytokine staining and multiparameter flow cytometry
Fresh lymphocytes were incubated at 37°C and 5% CO2 overnight with 100 µl/well of HAC1 or split virus X179a antigen (10 µg HA/ml), 2 µg/ml anti-CD28 and CD49d antibodies (PharMingen) and GolgiStop (monensin) and GolgiPlug (10 µg/ml Brefeldin A) (BD Biosciences, USA) in lymphocyte medium before intracellular cytokine staining. Negative controls were incubated in lymphocyte medium alone (no antigen) and lymphocytes from pre vaccination samples incubated in medium with or without influenza antigen. Subsequently, cells were stained for CD3, CD4, CD8, IFN-γ, IL-2 and TNF-α (BD Biosciences) using the BD Cytofix/Cytoperm kit according to the manufacturer's instructions and as described in reference.Citation23 Finally, cells were re-suspended in PBS containing 5% FCS and live lymphocytes (ethidium monoazide negative) were acquired (300,000 cells per sample) using a BD FACSCanto flow cytometer. Data were analyzed by using FlowJo v8.8.6 software (Tree Star, USA), Pestle v1.6.2 and Spice 5.0 (Mario Roederer, Vaccine Research Centre, NIH) and polyfunctional T cells were identified as described in references.Citation23,Citation48 T cells were classified based on cytokine secretion as single producers (1 cytokine), double producers (2 cytokines) and polyfunctional triple producers (simultaneously secreting IFN-γ, IL-2 and TNF-α).
Statistics
Statistical analysis were performed using the GraphPad Prism 5.0c for Mac OS X. Significant changes were determined with a paired student t test (two-sided with 95% CI) except for the ELISA results where a non-paired t test was used (two-sided with 95% CI). All correlations were determined using the Spearman test (two-sided with 95% CI).
Abbreviations: | ||
ASC | = | antibody secreting cell |
CHMP | = | The Committee for Medicinal Products for Human Use |
ELISA | = | enzyme linked immunosorbent assay |
ELISPOT | = | enzyme linked immuno spot assay |
GMA | = | geometric mean area |
GM-CSF | = | granulocyte/macrophage colony stimulating factor |
GMT | = | geometric mean titre |
HA | = | haemagglutinin |
HI | = | haemagglutinin inhibition |
IFN | = | interferon |
IL | = | interleukin |
M1 | = | matrix 1 |
NA | = | neuraminidase |
NP | = | nucleoprotein |
PBMC | = | peripheral blood mononuclear cell |
pdmH1N1 | = | pandemic H1N1 |
SRH | = | single radial haemolysis |
TLR | = | toll-like receptor |
TNF | = | tumour necrosis factor |
Acknowledgments
The project was funded by The Norwegian Research Council program, GLOBVAC (185441) and intramurally by the Influenza Centre, University of Bergen, the Norwegian Directorate of Health and the Ministry of Health and Care Services. We thank Diane Major at the National Institute for Biological Standards and Control (NIBSC), UK, for supplying the whole virus X179a antigen and GlaxoSmithKline for supplying the split virus X179a antigen. We also thank the Bergen Clinical Vaccine Consortium for help with the clinical study and the staff at the Influenza Centre for help with laboratory analysis.
Disclosure of Potential Conflicts of Interest
No potential conflicts of interest were disclosed.
References
- Chichester JA, Yusibov V. Plants as alternative systems for production of vaccines. Hum Vaccin 2007; 3:146 - 8; http://dx.doi.org/10.4161/hv.3.4.4148; PMID: 17643065
- Chichester JA, Haaheim LR, Yusibov V. Using plant cells as influenza vaccine substrates. Expert Rev Vaccines 2009; 8:493 - 8; http://dx.doi.org/10.1586/erv.09.3; PMID: 19348564
- Shoji Y, Chichester JA, Jones M, Manceva SD, Damon E, Mett V, et al. Plant-based rapid production of recombinant subunit hemagglutinin vaccines targeting H1N1 and H5N1 influenza. Hum Vaccin 2011; 7:Suppl 41 - 50; http://dx.doi.org/10.4161/hv.7.0.14561; PMID: 21266846
- Lu X, Tumpey TM, Morken T, Zaki SR, Cox NJ, Katz JM. A mouse model for the evaluation of pathogenesis and immunity to influenza A (H5N1) viruses isolated from humans. J Virol 1999; 73:5903 - 11; PMID: 10364342
- Lipatov AS, Hoffmann E, Salomon R, Yen H-L, Webster RG. Cross-protectiveness and immunogenicity of influenza A/Duck/Singapore/3/97(H5) vaccines against infection with A/Vietnam/1203/04(H5N1) virus in ferrets. J Infect Dis 2006; 194:1040 - 3; http://dx.doi.org/10.1086/507709; PMID: 16991078
- Treanor JJ, Wilkinson BE, Masseoud F, Hu-Primmer J, Battaglia R, O’Brien D, et al. Safety and immunogenicity of a recombinant hemagglutinin vaccine for H5 influenza in humans. Vaccine 2001; 19:1732 - 7; http://dx.doi.org/10.1016/S0264-410X(00)00395-9; PMID: 11166898
- Nicholson KG, Colegate AE, Podda A, Stephenson I, Wood J, Ypma E, et al. Safety and antigenicity of non-adjuvanted and MF59-adjuvanted influenza A/Duck/Singapore/97 (H5N3) vaccine: a randomised trial of two potential vaccines against H5N1 influenza. Lancet 2001; 357:1937 - 43; http://dx.doi.org/10.1016/S0140-6736(00)05066-2; PMID: 11425416
- Zhu F-C, Wang H, Fang H-H, Yang JG, Lin XJ, Liang X-F, et al. A novel influenza A (H1N1) vaccine in various age groups. N Engl J Med 2009; 361:2414 - 23; http://dx.doi.org/10.1056/NEJMoa0908535; PMID: 19846844
- Liang X-F, Wang H-Q, Wang J-Z, Fang H-H, Wu J, Zhu F-C, et al. Safety and immunogenicity of 2009 pandemic influenza A H1N1 vaccines in China: a multicentre, double-blind, randomised, placebo-controlled trial. Lancet 2010; 375:56 - 66; http://dx.doi.org/10.1016/S0140-6736(09)62003-1; PMID: 20018364
- Greenberg ME, Lai MH, Hartel GF, Wichems CH, Gittleson C, Bennet J, et al. Response to a monovalent 2009 influenza A (H1N1) vaccine. N Engl J Med 2009; 361:2405 - 13; http://dx.doi.org/10.1056/NEJMoa0907413; PMID: 19745216
- Plennevaux E, Sheldon E, Blatter M, Reeves-Hoché M-K, Denis M. Immune response after a single vaccination against 2009 influenza A H1N1 in USA: a preliminary report of two randomised controlled phase 2 trials. Lancet 2010; 375:41 - 8; http://dx.doi.org/10.1016/S0140-6736(09)62026-2; PMID: 20018365
- Clark TW, Pareek M, Hoschler K, Dillon H, Nicholson KG, Groth N, et al. Trial of 2009 influenza A (H1N1) monovalent MF59-adjuvanted vaccine. N Engl J Med 2009; 361:2424 - 35; http://dx.doi.org/10.1056/NEJMoa0907650; PMID: 19745215
- Madhun AS, Akselsen PE, Sjursen H, Pedersen G, Svindland S, Nøstbakken JK, et al. An adjuvanted pandemic influenza H1N1 vaccine provides early and long term protection in health care workers. Vaccine 2010; 29:266 - 73; http://dx.doi.org/10.1016/j.vaccine.2010.10.038; PMID: 21034828
- Shoji Y, Chichester JA, Bi H, Musiychuk K, de la Rosa P, Goldschmidt L, et al. Plant-expressed HA as a seasonal influenza vaccine candidate. Vaccine 2008; 26:2930 - 4; http://dx.doi.org/10.1016/j.vaccine.2008.03.045; PMID: 18440103
- Shoji Y, Farrance CE, Bi H, Shamloul M, Green B, Manceva S, et al. Immunogenicity of hemagglutinin from A/Bar-headed Goose/Qinghai/1A/05 and A/Anhui/1/05 strains of H5N1 influenza viruses produced in Nicotiana benthamiana plants. Vaccine 2009; 27:3467 - 70; http://dx.doi.org/10.1016/j.vaccine.2009.01.051; PMID: 19200814
- Shoji Y, Bi H, Musiychuk K, Rhee A, Horsey A, Roy G, et al. Plant-derived hemagglutinin protects ferrets against challenge infection with the A/Indonesia/05/05 strain of avian influenza. Vaccine 2009; 27:1087 - 92; http://dx.doi.org/10.1016/j.vaccine.2008.11.108; PMID: 19100806
- Mett V, Musiychuk K, Bi H, Farrance CE, Horsey A, Ugulava N, et al. A plant-produced influenza subunit vaccine protects ferrets against virus challenge. Influenza Other Respi Viruses 2008; 2:33 - 40; http://dx.doi.org/10.1111/j.1750-2659.2008.00037.x; PMID: 19453491
- Cox RJ, Brokstad KA, Zuckerman MA, Wood JM, Haaheim LR, Oxford JS. An early humoral immune response in peripheral blood following parenteral inactivated influenza vaccination. Vaccine 1994; 12:993 - 9; http://dx.doi.org/10.1016/0264-410X(94)90334-4; PMID: 7975853
- Cox RJ, Madhun AS, Hauge S, Sjursen H, Major D, Kuhne M, et al. A phase I clinical trial of a PER.C6 cell grown influenza H7 virus vaccine. Vaccine 2009; 27:1889 - 97; http://dx.doi.org/10.1016/j.vaccine.2009.01.116; PMID: 19368768
- Moris P, van der Most R, Leroux-Roels I, Clement F, Dramé M, Hanon E, et al. H5N1 influenza vaccine formulated with AS03 A induces strong cross-reactive and polyfunctional CD4 T-cell responses. J Clin Immunol 2011; 31:443 - 54; http://dx.doi.org/10.1007/s10875-010-9490-6; PMID: 21174144
- Roman F, Clément F, Dewé W, Walravens K, Maes C, Willekens J, et al. Effect on cellular and humoral immune responses of the AS03 adjuvant system in an A/H1N1/2009 influenza virus vaccine administered to adults during two randomized controlled trials. Clin Vaccine Immunol 2011; 18:835 - 43; http://dx.doi.org/10.1128/CVI.00480-10; PMID: 21450978
- Seder RA, Darrah PA, Roederer M. T-cell quality in memory and protection: implications for vaccine design. Nat Rev Immunol 2008; 8:247 - 58; http://dx.doi.org/10.1038/nri2274; PMID: 18323851
- Darrah PA, Patel DT, De Luca PM, Lindsay RWB, Davey DF, Flynn BJ, et al. Multifunctional TH1 cells define a correlate of vaccine-mediated protection against Leishmania major. Nat Med 2007; 13:843 - 50; http://dx.doi.org/10.1038/nm1592; PMID: 17558415
- Forbes EK, Sander C, Ronan EO, McShane H, Hill AVS, Beverley PCL, et al. Multifunctional, high-level cytokine-producing Th1 cells in the lung, but not spleen, correlate with protection against Mycobacterium tuberculosis aerosol challenge in mice. J Immunol 2008; 181:4955 - 64; PMID: 18802099
- Madhun AS, Haaheim LR, Nilsen MV, Cox RJ. Intramuscular Matrix-M-adjuvanted virosomal H5N1 vaccine induces high frequencies of multifunctional Th1 CD4+ cells and strong antibody responses in mice. Vaccine 2009; 27:7367 - 76; http://dx.doi.org/10.1016/j.vaccine.2009.09.044; PMID: 19781678
- Madhun AS, Haaheim LR, Nøstbakken JK, Ebensen T, Chichester J, Yusibov V, et al. Intranasal c-di-GMP-adjuvanted plant-derived H5 influenza vaccine induces multifunctional Th1 CD4+ cells and strong mucosal and systemic antibody responses in mice. Vaccine 2011; 29:4973 - 82; http://dx.doi.org/10.1016/j.vaccine.2011.04.094; PMID: 21600260
- Pedersen G, Halstensen A, Sjursen H, Naess A, Kristoffersen EK, Cox RJ. Pandemic influenza vaccination elicits influenza-specific CD4+ Th1-cell responses in hypogammaglobulinaemic patients: four case reports. Scand J Immunol 2011; 74:210 - 8; http://dx.doi.org/10.1111/j.1365-3083.2011.02561.x; PMID: 21438900
- D’Aoust M-A, Lavoie P-O, Couture MM-J, Trépanier S, Guay J-M, Dargis M, et al. Influenza virus-like particles produced by transient expression in Nicotiana benthamiana induce a protective immune response against a lethal viral challenge in mice. Plant Biotechnol J 2008; 6:930 - 40; http://dx.doi.org/10.1111/j.1467-7652.2008.00384.x; PMID: 19076615
- Landry N, Ward BJ, Trépanier S, Montomoli E, Dargis M, Lapini G, et al. Preclinical and clinical development of plant-made virus-like particle vaccine against avian H5N1 influenza. PLoS One 2010; 5:e15559; http://dx.doi.org/10.1371/journal.pone.0015559; PMID: 21203523
- Chu DW-S, Hwang S-J, Lim FS, Oh HML, Thongcharoen P, Yang P-C, et al, H5N1 Flu Study Group for Hong Kong, Singapore, Taiwan and Thailand. Immunogenicity and tolerability of an AS03(A)-adjuvanted prepandemic influenza vaccine: a phase III study in a large population of Asian adults. Vaccine 2009; 27:7428 - 35; http://dx.doi.org/10.1016/j.vaccine.2009.07.102; PMID: 19683087
- Roman F, Vaman T, Gerlach B, Markendorf A, Gillard P, Devaster J-M. Immunogenicity and safety in adults of one dose of influenza A H1N1v 2009 vaccine formulated with and without AS03A-adjuvant: preliminary report of an observer-blind, randomised trial. Vaccine 2010; 28:1740 - 5; http://dx.doi.org/10.1016/j.vaccine.2009.12.014; PMID: 20034605
- Lund JM, Alexopoulou L, Sato A, Karow M, Adams NC, Gale NW, et al. Recognition of single-stranded RNA viruses by Toll-like receptor 7. Proc Natl Acad Sci U S A 2004; 101:5598 - 603; http://dx.doi.org/10.1073/pnas.0400937101; PMID: 15034168
- Diebold SS, Kaisho T, Hemmi H, Akira S, Reis e Sousa C. Innate antiviral responses by means of TLR7-mediated recognition of single-stranded RNA. Science 2004; 303:1529 - 31; http://dx.doi.org/10.1126/science.1093616; PMID: 14976261
- Dupuis M, Denis-Mize K, LaBarbara A, Peters W, Charo IF, McDonald DM, et al. Immunization with the adjuvant MF59 induces macrophage trafficking and apoptosis. Eur J Immunol 2001; 31:2910 - 8; http://dx.doi.org/10.1002/1521-4141(2001010)31:10<2910::AID-IMMU2910>3.0.CO;2-3; PMID: 11592066
- Seubert A, Monaci E, Pizza M, O’Hagan DT, Wack A. The adjuvants aluminum hydroxide and MF59 induce monocyte and granulocyte chemoattractants and enhance monocyte differentiation toward dendritic cells. J Immunol 2008; 180:5402 - 12; PMID: 18390722
- Mosca F, Tritto E, Muzzi A, Monaci E, Bagnoli F, Iavarone C, et al. Molecular and cellular signatures of human vaccine adjuvants. Proc Natl Acad Sci U S A 2008; 105:10501 - 6; http://dx.doi.org/10.1073/pnas.0804699105; PMID: 18650390
- Morel S, Didierlaurent A, Bourguignon P, Delhaye S, Baras B, Jacob V, et al. Adjuvant System AS03 containing α-tocopherol modulates innate immune response and leads to improved adaptive immunity. Vaccine 2011; 29:2461 - 73; http://dx.doi.org/10.1016/j.vaccine.2011.01.011; PMID: 21256188
- Galli G, Medini D, Borgogni E, Zedda L, Bardelli M, Malzone C, et al. Adjuvanted H5N1 vaccine induces early CD4+ T cell response that predicts long-term persistence of protective antibody levels. Proc Natl Acad Sci U S A 2009; 106:3877 - 82; http://dx.doi.org/10.1073/pnas.0813390106; PMID: 19237568
- Stephenson I, Bugarini R, Nicholson KG, Podda A, Wood JM, Zambon MC, et al. Cross-reactivity to highly pathogenic avian influenza H5N1 viruses after vaccination with nonadjuvanted and MF59-adjuvanted influenza A/Duck/Singapore/97 (H5N3) vaccine: a potential priming strategy. J Infect Dis 2005; 191:1210 - 5; http://dx.doi.org/10.1086/428948; PMID: 15776364
- Leroux-Roels I, Borkowski A, Vanwolleghem T, Dramé M, Clement F, Hons E, et al. Antigen sparing and cross-reactive immunity with an adjuvanted rH5N1 prototype pandemic influenza vaccine: a randomised controlled trial. Lancet 2007; 370:580 - 9; http://dx.doi.org/10.1016/S0140-6736(07)61297-5; PMID: 17707753
- Bernstein DI, Edwards KM, Dekker CL, Belshe R, Talbot HKB, Graham IL, et al. Effects of adjuvants on the safety and immunogenicity of an avian influenza H5N1 vaccine in adults. J Infect Dis 2008; 197:667 - 75; http://dx.doi.org/10.1086/527489; PMID: 18260764
- Levie K, Leroux-Roels I, Hoppenbrouwers K, Kervyn A-D, Vandermeulen C, Forgus S, et al. An adjuvanted, low-dose, pandemic influenza A (H5N1) vaccine candidate is safe, immunogenic, and induces cross-reactive immune responses in healthy adults. J Infect Dis 2008; 198:642 - 9; http://dx.doi.org/10.1086/590913; PMID: 18576945
- Cox RJ, Pedersen G, Madhun AS, Svindland S, Sævik M, Breakwell L, et al. Evaluation of a virosomal H5N1 vaccine formulated with Matrix M™ adjuvant in a phase I clinical trial. Vaccine 2011; 29:8049 - 59; http://dx.doi.org/10.1016/j.vaccine.2011.08.042; PMID: 21864624
- Karaolis DKR, Means TK, Yang D, Takahashi M, Yoshimura T, Muraille E, et al. Bacterial c-di-GMP is an immunostimulatory molecule. J Immunol 2007; 178:2171 - 81; PMID: 17277122
- Chen W, Kuolee R, Yan H. The potential of 3′,5′-cyclic diguanylic acid (c-di-GMP) as an effective vaccine adjuvant. Vaccine 2010; 28:3080 - 5; http://dx.doi.org/10.1016/j.vaccine.2010.02.081; PMID: 20197136
- Schild GC, Pereira MS, Chakraverty P. Single-radial-hemolysis: a new method for the assay of antibody to influenza haemagglutinin. Applications for diagnosis and seroepidemiologic surveillance of influenza. Bull World Health Organ 1975; 52:43 - 50; PMID: 1082381
- Brokstad KA, Cox RJ, Olofsson J, Jonsson R, Haaheim LR. Parenteral influenza vaccination induces a rapid systemic and local immune response. J Infect Dis 1995; 171:198 - 203; http://dx.doi.org/10.1093/infdis/171.1.198; PMID: 7798664
- Duvall MG, Precopio ML, Ambrozak DA, Jaye A, McMichael AJ, Whittle HC, et al. Polyfunctional T cell responses are a hallmark of HIV-2 infection. Eur J Immunol 2008; 38:350 - 63; http://dx.doi.org/10.1002/eji.200737768; PMID: 18200635