Abstract
The health burden of Chagas’ disease (resulting from Trypanosoma cruzi infection) in Latin America (estimated to outweigh that of malaria by 5-fold and affect 2–6 million people in Mexico alone) has motivated development of therapeutic vaccines to prevent infection progression to severe disease. Our economic model for a Chagas’ therapeutic vaccine in Mexico suggests that a vaccine would be highly cost-effective and in many cases economically dominant (providing both cost savings and health benefits) throughout a range of protection durations, severe adverse event risk, and dosing regimens and would be most likely to provide a positive return on investment if the vaccine prevented (rather than delayed) the onset of cardiomyopathy.
Introduction
The health burden of Chagas’ disease (American trypanosomiasis) in South and North America is substantial. Today an estimated 8–11 million people are believed to be infected with the etiologic agent, Trypanososma cruzi, an intracellular protozoan parasite of the heart and other organs.Citation1 The largest number of cases occur in areas of poverty in Latin America, especially in Bolivia, Mexico and Central America. Based on disability adjusted life years, the disease burden of Chagas’ disease may be five times greater than malaria,Citation2,Citation3 and based on this same metric, Chagas’ disease today ranks among the most important neglected tropical diseases in the Americas.Citation4
T. cruzi is most commonly transmitted through the feces of the infected triatomine vector. Acute infection, which is either asymptomatic or leads to swelling of the tissue surrounding the eye (Romana’s sign), fever, malaise, muscle pain, heptasplenomegaly, heart failure and pericardial effusion,Citation5 usually resolves within 6–8 weeks. Patients then enter the indeterminate disease phase, where most are asymptomatic, despite persistent infection and evidence of seropositivity on diagnostic assays that rely on IgG binding to parasite antigens (detected by ELISA, indirect immunofluorescence, or indirect hemagglutinin assays). Ultimately, approximately 30–40% of those infected progress to chronic disease, associated with either severe heart disease (typically cardiomyopathy) or megaviscera or both conditions. A case definition of Chagas’ disease is typically made based on an unequivocal positive serology for T. cruzi, either with or without signs and symptoms of heart disease and/or megaviscera, i.e., indeterminate or determinant disease, respectively. For example, in Mexico, an estimated 2 to 6 million people are currently infectedCitation6 with as many as 40% and 2% destined to develop cardiomyopathy and megaviscera, respectively, within 10–30 y following initial exposure and infection.Citation7 Chagas’ disease has also emerged in the US and Europe.Citation8-Citation10 This intense disease burden coupled with the globalization of Chagas’ disease has motivated the development of vaccines that prevent T. cruzi infection and/or therapeutic vaccines that inhibit progression of disease after infection.Citation11 Chagas’ disease manifestations can lead to substantial attendant economic burden (the estimated average annual patient cost in Mexico is $3,000–11,088, depending on the severity of disease and the level of medical careCitation12).
Although much attention has focused on the control of triatomine bugs, the insect vector that transmits T. cruzi via its feces to humans, Chagas' persistence and continuing spread suggests the need for additional interventions.Citation13 Drugs such as benznidazole and nifurtimox can prevent disease progression, with an efficacy of approximately 60% in children and recently infected adults,Citation14,Citation15 although this has been reported to decrease to 37% or less after chronic disease onset.Citation16 Unfortunately, currently available drugs have many drawbacks, including lengthy regimens, high treatment costs (est. drug cost: $59.54; est. total annual cost of acute disease: $591.80Citation17), risk of severe adverse events,Citation18 and limits on groups that are recommended to receive them.Citation7 Chagas’ disease has also emerged as an important disease in pregnancy, with high rates of vertical transmission and congenital infection,Citation19 which is problematic as both drugs are also contraindicated in pregnant women.
There are currently efforts to develop a therapeutic vaccine for Chagas’ disease. Our previous cost-effectiveness study focused on a preventive vaccine;Citation20 however, there remains a need to determine the potential economic value of a therapeutic vaccine to guide its development and potential implementation. Leading antigen candidates include Tc24 and TSA-1, which have been shown to reduce parasitemia and progression to cardiac disease in mice models.Citation11,Citation21-Citation23 However, other candidate molecules have also been put forward.Citation24 If successful, the vaccine is thought to function to either prevent or delay the onset of Chagasic cardiomyopathy and potentially megaviscera and could be used in place of or alongside currently available treatments. Although a therapeutic infectious disease vaccine has not been developed to date, regimens of such vaccines during development have been mostly multi-dose.Citation25,Citation26 An economic modeling study could help determine whether a vaccine would generate sufficient subsequent revenues to support its development, especially important since Chagas' disease tends to affect populations living in extreme poverty.Citation27 Additionally, such a study could help delineate the target product profile (TPP), i.e., the menu of ideal characteristics for the vaccine, while the vaccine is still early in development and can be more readily altered.Citation28 Therefore, we developed a stochastic economic Markov simulation model to help delineate a TPP and determine the potential return-on-investment (ROI) for developing a therapeutic Chagas’ vaccine.
Results
contains costs, probabilities, and disability weights used to parameterize the model. Baseline assumptions for both desired and minimally acceptable TPPs are outlined in . Sensitivity analyses varied vaccine dose regimen, compliance, risk of vaccine-induced cardiomyopathy (i.e., severe adverse events, SAEs), and duration of vaccine protection for each TPP; details are further described in the Methods.
Table 1. Table of inputs
Table 2. Desired and minimally acceptable target product profiles (tpps) baseline assumptions for desired and minimally acceptable vaccine targets
Cost-effectiveness analysis (CEA)
In our analyses, a Chagas’ therapeutic vaccine was highly cost-effective and frequently saved costs under a wide range of conditions evaluated. This was the case for both desired and minimally acceptable target vaccines with certain characteristics, as described below. In fact, even a vaccine meeting the minimally acceptable target criteria could be economically dominant (i.e., less costly and more effective) over not vaccinating.
Risk of vaccine-induced cardiomyopathy
Whether damage resulting from chronic Chagas’ disease is caused by an autoimmune response or parasite persistence is not well understood.Citation7 The possibility of an autoimmune mechanism raises concern that a vaccine could cause similar irreversible damage. Since this is an important concern regarding a therapeutic vaccine, we evaluated vaccination associated with several different risk possibilities, outlined in the Methods.
Severe adverse events (SAE) risk was the strongest driver of vaccine cost-effectiveness. delineates how the cost-effectiveness of a vaccine that meets the desired TPP varies with SAE risk, protection duration, and patient compliance with vaccination. shows the same for a vaccine that only meets the minimally acceptable TPP. For both and , the vaccine only affects (prevents or delays) the risk of cardiac outcomes and not megaviscera. Expanding the vaccine effects to prevent gastrointestinal outcomes did not significantly affect results and at most made the vaccine slightly more cost-effective. As and illustrate, the SAE risk threshold (below which vaccine becomes cost-effective) is much higher for a vaccine that can prevent rather than merely delay chronic disease onset. For example, a one-dose vaccine that could prevent chronic cardiac outcomes and provide lifetime protection (baseline desired TPP) could have an SAE risk of up to 12% and still remain cost-effective. By contrast, a two-dose vaccine delaying the onset of these outcomes to 10 y (minimally acceptable target) could have a SAE risk of no higher than 5.1% to remain cost-effective.
Table 3. Incremental cost-effectiveness ratio (ICER) for a two-dose vaccine meeting desired vaccine target criteria with no effect on megaviscera
Table 4. Incremental cost-effectiveness ratio (ICER) for a multi-dose vaccine meeting minimally acceptable vaccine target criteria with no effect on megaviscera
Dose regimen and compliance
Switching from a single to a multi-dose regimen, a more likely vaccine presentation, also resulted in considerably decreased vaccine cost-effectiveness, driven by two primary mechanisms: increasing the number of doses required raises the overall SAE risk, since each dose carries an SAE risk, and multi-dose regimens depend on patients being compliant in returning for subsequent doses. Increasing the immunization regimen from one dose to two lowered the SAE risk threshold considerably. For example, in most of our explored scenarios, a single-dose vaccine that either prevented or delayed the chronic disease outcome onset was highly cost-effective and often economically dominant (less costly and more effective) compared with not vaccinating (data not shown). By contrast, a multi-dose vaccine was cost-effective under a more limited spectrum of scenarios ( and ). Decreasing patient compliance considerably reduced the cost-effectiveness of a multi-dose vaccine. However, even with 100% compliance, each additional required dose resulted in a significant decrease in the cost-effectiveness of vaccination.
Duration of protection and delay of chronic outcomes
By comparison, the vaccine's duration of protection had less impact on the vaccine's cost-effectiveness, particularly for a vaccine meeting the desired TPP (). For a vaccine meeting the desired TPP and carrying a 3% SAE risk, decreasing the protection duration from lifetime to ten years resulted in the vaccine going from being cost-effective in 97% of simulated patients to 75% of simulated patients. In other words, the vaccine remained cost-effective in a majority of cases. A vaccine that delayed the onset of chronic cardiac outcomes (minimally acceptable vaccine target) was less cost-effective than a vaccine that prevented these outcomes (desired target); the duration of the delay in onset of chronic outcomes elicited by a vaccine had a moderate effect on the probability of the vaccine being cost-effective.
Cost per cardiomyopathy case averted
shows the cost per case of cardiomyopathy averted using a vaccine with the desired TPP, where negative costs indicate cost savings. A desired target vaccine under baseline model assumptions (no effect on megaviscera, one-dose, 3% risk of SAE, lifetime protection) could avert 319 cases of cardiomyopathy and 217 Chagas’ related deaths per 1,000 people vaccinated and save $3,005 per case of cardiomyopathy averted. If the vaccine were to additionally prevent megaviscera, vaccination often saved additional costs and averted more chronic outcomes (data not shown). While a one-dose vaccine was cost saving under a majority of conditions, continuously reducing compliance to a two-dose regimen likewise lowered the SAE risk threshold where cost savings would result. For scenarios where the vaccine only provided 10 y protection and SAE risk was 3% or greater, vaccination was often associated with higher costs and more chronic outcomes than not vaccinating. Vaccination with a minimally acceptable target vaccine frequently resulted in more cases, although it was often less costly at an SAE risk of 1% or less (data not shown).
Table 5. Cost per cardiomyopathy case averted (US$) using desired TPP vaccinea
Return on investment (ROI)
Our ROI analysis identified the roll-out strategies and vaccine criteria that would yield a positive return on investment in Mexico, assuming 6 million people were infected and eligible for vaccination. contains our results. If a desired target vaccine reached the market in 15 y after $100 million were spent on development, as long as ≥ 3.0% of the target population was vaccinated in the first year on the market, a substantially positive ROI would be realized. This threshold increased to 4.0% if development costs were $150 million, 5.5% if development costs were $200 million and 11.0% if $400 million were needed. If any of these targets were achieved, the additional return on investment costs would be $2–18 million; exceeding these targets would result in a more positive ROI. A vaccine with a $200 million vaccine development cost that took 20 y to reach the market would need to be administered to 7.0% of the target population to regain investment costs. Lower development costs of $150 million and $100 million only required 5.0% and 3.5% coverage, respectively, in order to generate a positive return on investment. A vaccine with a desired target TPP costing $100 million could take 30 y or longer to develop and reach the market, still regain initial costs ($9.8 million), and only require 4.5% coverage; similarly, a vaccine costing $150 million that took just as long to reach the market could provide a positive return on investment of $20.8 million by vaccinating 7.0% of the target population in the vaccine’s first year on the market. In contrast to a vaccine with the desired TPP, a vaccine with the minimally acceptable profile failed to provide a positive return on investment for any combination of time to market and coverage included in our analyses regardless of initial cost of development. As a single dose presentation may be unlikely, a two dose vaccine meeting the desired TPP requiring 15–30 y to develop could generate a positive return of $9.1–29.6 million during its first year on the market. In fact, such a vaccine would require coverage of as little as 3.0% if development costs were $100 million and 5.5% if development costs were $200 million during the first year to generate a positive return.
Table 6. Return on investment analyses for desired TPP vaccine
Discussion
Our results suggest that a therapeutic Chagas' vaccine would be even more cost-effective than a preventive vaccine.Citation11 Results from our previous study evaluating the cost-effectiveness of a preventative Chagas’ vaccine suggest that a vaccine would be economically dominant as long as the annual risk of infection is ≥ 5%, the vaccine (and its administration) cost ≤ $30, and vaccine efficacy ≥ 75%.Citation20 This finding is reasonable since a therapeutic vaccine would be administered to a more focused portion of the population with indeterminate and determinate status who are already at much higher risk of developing chronic (and therefore costly and disabling) disease. The true benefit of a licensed Chagas’ therapeutic vaccine may exceed these estimates if incomplete vaccine regimens offered partial protection. Additionally, from an economic standpoint, there will be a higher tolerance for vaccine-induced SAEs for a therapeutic vaccine vs. a preventive vaccine administered to an uninfected, healthier population.
Since the SAE risk is a major driver of the economic value of a therapeutic vaccine, delineating the tolerance for such risk can be essential in guiding the investment in and development of the vaccine. In terms of cost-effectiveness, a vaccine that prevents the onset of chronic disease manifestations would allow for a higher risk of SAEs (up to 12% if vaccine were to confer lifetime protection) than one that merely delays the onset (e.g., a 7% SAE risk would mean that a 2-dose vaccine that delayed the onset of cardiomyopathy for 10 y would have a < 20% chance of being cost-effective). With either target product profile explored, a vaccine would remain cost-effective with the baseline (3%) risk of vaccine-induced cardiomyopathy. Both preventative and therapeutic vaccine studies in mice have proven safe and have shown no signs of SAEs, suggesting that a risk < 3% for these outcomes may be feasible.Citation11 Although the risk of SAEs (as well as non-compliance with subsequent doses) favors single dose over multi-dose regimens, this will ultimately be determined by the vaccine formulation. Of course, there are additional ethical, societal, and other considerations taken into account when determining an “acceptable” level of SAE risk. These considerations would attenuate the thresholds estimated in this analysis; however, the degree to which this would occur would likely vary by location and risk of infection.
Our study also re-emphasizes the importance of limiting the number of doses required in a regimen, if possible. While each added dose may help achieve a higher level of protection, it also may increase the SAE risk (especially if each dose brings an independent risk of inducing an SAE) and the risk of patients not completing the entire regimen. The former consideration is essential for all vaccinees while the latter is particularly crucial for those with poor access to care.
Elucidating the potential ROI is important for any vaccine under development, but especially for one that targets a disease afflicting indigent populations. Although in the eyes of donor and non-government organizations achieving a positive ROI may not be a top priority, demonstrating the thresholds at which a positive return could be achieved may be informative. As our study demonstrates, the ROI for a Chagas’ therapeutic vaccine depends on the vaccine's characteristics. At vaccine development costs as high as $200 million, a vaccine meeting the desired TPP can have a positive ROI even if only very low coverage levels were attained. As our analysis examines the potential return after one year of vaccination, this ROI could ultimately far exceed $200 million. By contrast, a vaccine meeting the minimally acceptable TPP would have difficulty yielding a positive ROI.
Although a therapeutic vaccine may be more cost-effective than currently available drug treatment, a vaccine targeting an infected yet asymptomatic population presents many challenges. It is anticipated that the clinical development and testing of such a vaccine may require long time horizons to detect SAEs following completion of the vaccine series and possibly advancements in biomarkers for the detection of cardiac and other complications.Citation11 The success of a vaccine upon market release would largely depend on the screening strategy used to identify those infected with T. cruzi, but not yet showing signs of chronic disease. An inadequate strategy may result in underutilization of the vaccine and lead to economic loss. A therapeutic Chagas’ vaccine may also have a substantial market in developed countries, as Chagas’ is increasingly affecting those in countries not previously considered to be endemic, due to migration and geographic expansion of reported disease transmission.Citation29 For example, over 6 million documented immigrants from South and Central America and Mexico entered the US between 1981 and 2005, with 38,777–339,954 estimated to be infected with T. cruziCitation29 and approximately 11,633–101,986 progressing to chronic disease. Moreover, there is evidence of autochthonous transmission in Texas (and elsewhere in the US) among both canines and humans.Citation8 Additional estimates suggest that there could be as many as 18,811 Latin American immigrants infected with T. cruzi residing in Spain and as many as 1,067 infections in Australia.Citation29
Limitations
By definition, models are simplified representations of the real world and, therefore, cannot capture all potential factors, heterogeneity, and effects. For example, our model does not account for various co-morbidities (e.g., pre-existing cardiovascular disease) that may affect an individual's risk, age, and impact of developing chronic Chagas’ disease. The risk of vaccine induced SAEs may not scale linearly with the number of doses. Assumptions associated with our CEA analysis involving SAE risk, no vaccine protection being granted by incomplete vaccine regimens, and vaccination not attenuating the risk of CHF if an individual were to develop cardiomyopathy are likely very conservative; the benefit of a vaccine may therefore exceed these estimates. Additionally, not all scenarios explored may be achievable (e.g., lifetime protection against chronic disease). Due to uncertainty regarding number of doses and the unlikelihood of a single-dose product, total development costs for investment may be higher. Also if some product (bulk antigen) can be sold as a prophylactic vaccine, there would be more sales from the same capital investment. Since there is considerable variability in when an infected individual may develop chronic disease, our ROI analysis could not pinpoint the exact year in which the ROI may turn positive. While model inputs and assumptions came from an extensive literature review, data from these sources may not hold under all conditions.
Conclusion
A Chagas’ therapeutic vaccine meeting a variety of vaccine profiles may provide substantial health and economic benefits. Depending on the vaccine's characteristics, the vaccine could provide a positive ROI even if a relatively small portion of the target population eventually received the vaccine. The primary drivers of the vaccine's cost-effectiveness are the vaccine's ability to prevent (rather than merely delay) the onset of cardiac sequelae and risk of inducing SAEs. The number of doses in the regimen also considerably affects the cost-effectiveness of the vaccine. Our study demonstrates how the design of the vaccine will be crucial in its eventual economic impact and helps outline the thresholds for key vaccine characteristics.
Methods
Chagas’ disease model structure
This analysis focused on the use of a Chagas’ therapeutic vaccine in Mexico. This model extended our previously described Chagas’ disease modelCitation20 constructed in TreeAge Pro 2009 (TreeAge Software). shows the different possible mutually exclusive health states in this Markov model. Each simulated individual entered the model (and either received or did not receive an initial dose of vaccine) into the indeterminate health state (defined as a seropositive antibody test result without electrocardiographic changes). A draw from a normal distribution (mean: 37, standard deviation: 13) determined the age of an individual in this initial stage.Citation30 The cycle length for this model was one year.
Figure 1. Model structure. (A) Health states. Death from Chagas’ related or other causes could occur at any health state, see for mortality probabilities. aAll individuals entered the model in the Indeterminate health state (B) Transition probabilities for vaccinated individuals in the indeterminate health state. aAll individuals on the “Vaccinate” branch received the first dose of the vaccine. Receipt of subsequent doses was dependent upon compliance and the number of doses in a full vaccine course. bIncluded, but not limited to cardiomyopathy
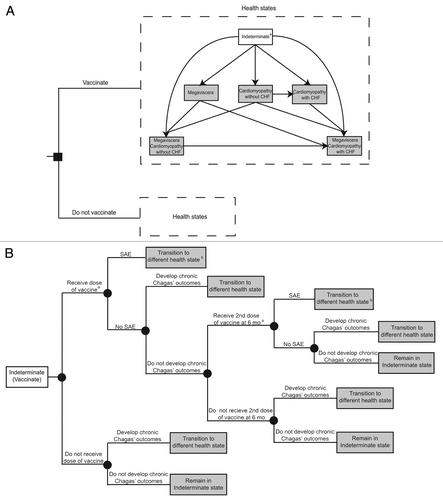
Each year the simulated patient had probabilities of staying in the same state or moving to another state until transitioning to the death state either from Chagas’ related (as determined by risk of death from Chagas’ complications) or from unrelated (as determined by the population's age stratified all-cause mortality) causes. At the beginning of each simulated patient's course through the model, a random draw from a uniform distribution (lower limit: 1 y, upper limit: 10 y) decided a patient’s number of years staying in the latent period, i.e., the number of years that the patient would remain in the indeterminate health state without vaccination before potentially progressing on to manifest clinical sequelae of chronic disease.Citation7 The state that a person was in during a particular year (one cycle) dictated the costs and fractions of a year lost to disability (if the patient manifested illness) that the patient accrued for that year. In other words, different chronic disease outcomes (i.e., cardiomyopathy without CHF, cardiomyopathy with CHF, megaviscera or two in combination) had probabilities of conferring various treatment-associated costs shown in (in 2011 US$); a 3% discount rate adjusted all subsequent costs and disability adjusted life-years (DALYs)Citation31 to 2011 values. Applying a half-cycle correction to costs and DALYs accounted for the fact that these events could occur throughout a given year. To remain conservative, those who developed both cardiac and gastrointestinal outcomes were only assigned the disability weight associated with the more severe disease manifestation.
Vaccination
Vaccination attenuated a patient's probability of progressing to clinical sequelae by 1-vaccine efficacy. To be conservative, no protection occurred until the completion of the entire course for multi-dose vaccine regimens (dose 1: month 0, dose 2: month 2, dose 3: year 2, dose 4: year 5). The baseline model scenarios considered vaccine profiles where a vaccine would (1) prevent (desired vaccine target) or (2) delay the onset of (minimally acceptable vaccine target) cardiomyopathy. Vaccine profiles are further outlined in . The desired target vaccine cost of $46 represents the minimum cost of treating chronic Chagas’ disease in ColombiaCitation32; the cost of $200 assumed for the minimally acceptable vaccine target is equal to one fifth of the maximum yearly cost of treatment reported from the same study.
Vaccination did not directly attenuate the probability of megaviscera, CHF or disease associated death in the baseline scenarios, however, we did evaluate a vaccine that additionally provided protection against megaviscera in our sensitivity analyses. Each vaccine dose had probabilities of inducing a severe adverse event (SAE), i.e., vaccine-induced cardiomyopathy (), since there remains the possibility that the clinical sequelae of Chagas’ disease are actually due to the host’s resulting immune response against the organism.Citation7 The occurrence of this SAE caused the affected individual to transition to have cardiomyopathy without a possibility of reverting back to the indeterminate state. As the risk of vaccine-induced cardiomyopathy has yet to be quantified, baseline SAE risk of 3% was chosen as it was approximately the midpoint of the range of reported nifurtimox and benznidazole associated risk of SAEs.Citation18,Citation33 Development of any clinical sequelae (either vaccine or disease-induced) meant immediate curtailment of the vaccine regimen. also lists the probabilities of treating minor side effects (with antihistamine and ibuprofen for uriticaria and carditis, respectively).Citation34 Each simulation run sent 1,000 paired hypothetical individuals through the model 1,000 times for a total of 1,000,000 individual outcomes. For each pair of individuals, one received vaccination and the other did not.
Cost-effectiveness analysis (CEA)
For each simulation, the equation below calculated the incremental cost-effectiveness ratio (ICER), expressed as the cost per disability-adjusted life year (DALY) averted, of vaccination over no vaccination:
Using standard World Health Organization (WHO) criteria, vaccination was highly cost-effective if the ICER ≤ $9,867 (1 × GDP/capita of Mexico), remained cost-effective if the ICER $9,868–29,602 (1–3 × GDP/capita of Mexico), and was no longer cost-effective if the ICER > $29,602 (3 × GDP/capita of Mexico).Citation35
Return on Investment (ROI)
Using Microsoft Excel (Microsoft), we developed a spreadsheet model that estimated the ROI for a Chagas’ therapeutic vaccine assuming a total net present value (NPV) vaccine development cost of $100 million, $150 million, $200 million and $400 million and determined the minimum target population coverage required during the vaccine’s first year on the market to achieve a positive ROI. Our analysis explored a range of target population coverage (range: 1–7%) and years to market (i.e., time from the beginning of clinical development through licensure until the vaccine reaches the market) scenarios (range: 15–30 y), assuming that a vaccine would not reach the market in < 15 y (due to the follow-up duration that may be necessary for vaccine trials). Each vaccinated individual accrued the NPV of cost savings from being vaccinated, derived from the aforementioned CEA. Multiplying this NPV of vaccinating an individual by the number of individuals vaccinated that year generated the cost impact of rolling out the vaccine in Mexico. All scenarios assumed 100% compliance and that vaccination had no effect on megaviscera.
Sensitivity analyses
Sensitivity analyses varied the following parameters for both desired and minimally acceptable vaccine target scenarios: compliance for full vaccine regimen (range: 25–100%), probability of severe adverse events (SAEs) or vaccine-induced cardiomyopathy (range: 0.1–7%), duration of protection (range: 10 y – lifetime) for the desired target and delay of onset of cardiomyopathy (range: 10–20 y) for the minimally acceptable vaccine target. All vaccinated individuals received the first dose of vaccine; compliance for a one-dose vaccine was therefore assumed to be 100%. In scenarios where the vaccine affected both the probability of cardiac and megaviscera outcomes, vaccine efficacy and duration of protection were assumed to be the same for both forms of disease. No booster dose was offered after protection was lost. The range of risk of SAEs represented the range of severe side effect rates seen in studies using benznidazole and nifurtimox.Citation18,Citation33
Acknowledgments
Supported by the Vaccine Modeling Initiative (VMI), funded by the Bill and Melinda Gates Foundation and the National Institute of General Medical Sciences Models of Infectious Disease Agent Study (MIDAS) through grant 5U54GM088491–02. The funders had no role in the study design, data collection and analysis, decision to publish, or preparation of the manuscript.
Disclosure of Potential Conflicts of Interest
No potential conflicts of interest were disclosed.
References
- Parasites- American Trypanosomiasis (also known as Chagas Disease). Atlanta: Centers for Disease Control and Prevention, 2010.
- Sustainable Development Department. The Millennium Development Goals in Latin America and the Caribbean: Progress, Priorities and IDB Support for Their Implementation. Washington, DC: Inter-American Development Bank, 2005.
- Annex Table 3 Burden of disease in DALYs by cause, sex and mortality stratum in WHO regions, estimates for 2002 In: The World Health Report 2004 -Changing History, ed. Geneva: World Health Organization, 2004.
- Hotez PJ, Bottazzi ME, Franco-Paredes C, Ault SK, Periago MR. The neglected tropical diseases of Latin America and the Caribbean: a review of disease burden and distribution and a roadmap for control and elimination. PLoS Negl Trop Dis 2008; 2:e300; http://dx.doi.org/10.1371/journal.pntd.0000300; PMID: 18820747
- Biolo A, Ribeiro AL, Clausell N. Chagas cardiomyopathy--where do we stand after a hundred years?. Prog Cardiovasc Dis 2010; 52:300 - 16; http://dx.doi.org/10.1016/j.pcad.2009.11.008; PMID: 20109600
- Cruz-Reyes A, Pickering-López JM. Chagas disease in Mexico: an analysis of geographical distribution during the past 76 years--a review. Mem Inst Oswaldo Cruz 2006; 101:345 - 54; http://dx.doi.org/10.1590/S0074-02762006000400001; PMID: 16951802
- Rassi A Jr., Rassi A, Marin-Neto JA. Chagas disease. Lancet 2010; 375:1388 - 402; http://dx.doi.org/10.1016/S0140-6736(10)60061-X; PMID: 20399979
- Bern C, Kjos S, Yabsley MJ, Montgomery SP. Trypanosoma cruzi and Chagas’ Disease in the United States. Clin Microbiol Rev 2011; 24:655 - 81; http://dx.doi.org/10.1128/CMR.00005-11; PMID: 21976603
- Hotez P. Neglected diseases amid wealth in the United States and Europe. Health Aff (Millwood) 2009; 28:1720 - 5; http://dx.doi.org/10.1377/hlthaff.28.6.1720; PMID: 19887412
- Hotez PJ. Neglected infections of poverty in the United States of America. PLoS Negl Trop Dis 2008; 2:e256; http://dx.doi.org/10.1371/journal.pntd.0000256; PMID: 18575621
- Quijano-Hernandez I, Dumonteil E. Advances and challenges towards a vaccine against Chagas disease. Hum Vaccin 2011; 7:1184 - 91; http://dx.doi.org/10.4161/hv.7.11.17016; PMID: 22048121
- Vallejo M, Montenegro P, Reyes PA. [How much does the medical treatment of chronic Chagas cardiopathy cost? Direct costs in a cardiology hospital]. Arch Cardiol Mex 2002; 72:129 - 37; PMID: 12148332
- Franco-Paredes C, Bottazzi ME, Hotez PJ. The unfinished public health agenda of chagas disease in the era of globalization. PLoS Negl Trop Dis 2009; 3:e470; http://dx.doi.org/10.1371/journal.pntd.0000470; PMID: 19582139
- Sosa Estani S, Segura EL, Ruiz AM, Velazquez E, Porcel BM, Yampotis C. Efficacy of chemotherapy with benznidazole in children in the indeterminate phase of Chagas’ disease. Am J Trop Med Hyg 1998; 59:526 - 9; PMID: 9790423
- de Andrade AL, Zicker F, de Oliveira RM, Almeida Silva S, Luquetti A, Travassos LR, et al. Randomised trial of efficacy of benznidazole in treatment of early Trypanosoma cruzi infection. Lancet 1996; 348:1407 - 13; http://dx.doi.org/10.1016/S0140-6736(96)04128-1; PMID: 8937280
- Fabbro DL, Streiger ML, Arias ED, Bizai ML, del Barco M, Amicone NA. Trypanocide treatment among adults with chronic Chagas disease living in Santa Fe city (Argentina), over a mean follow-up of 21 years: parasitological, serological and clinical evolution. Rev Soc Bras Med Trop 2007; 40:1 - 10; http://dx.doi.org/10.1590/S0037-86822007000100001; PMID: 17486245
- Basombrío MA, Schofield CJ, Rojas CL, del Rey EC. A cost-benefit analysis of Chagas disease control in north-western Argentina. Trans R Soc Trop Med Hyg 1998; 92:137 - 43; http://dx.doi.org/10.1016/S0035-9203(98)90720-9; PMID: 9764315
- Yun O, Lima MA, Ellman T, Chambi W, Castillo S, Flevaud L, et al. Feasibility, drug safety, and effectiveness of etiological treatment programs for Chagas disease in Honduras, Guatemala, and Bolivia: 10-year experience of Médecins Sans Frontières. PLoS Negl Trop Dis 2009; 3:e488; http://dx.doi.org/10.1371/journal.pntd.0000488; PMID: 19582142
- Buekens P, Almendares O, Carlier Y, Dumonteil E, Eberhard M, Gamboa-Leon R, et al. Mother-to-child transmission of Chagas’ disease in North America: why don’t we do more?. Matern Child Health J 2008; 12:283 - 6; http://dx.doi.org/10.1007/s10995-007-0246-8; PMID: 17602289
- Lee BY, Bacon KM, Connor DL, Willig AM, Bailey RR. The potential economic value of a Trypanosoma cruzi (Chagas disease) vaccine in Latin America. PLoS Negl Trop Dis 2010; 4:e916; http://dx.doi.org/10.1371/journal.pntd.0000916; PMID: 21179503
- Sanchez-Burgos G, Mezquita-Vega RG, Escobedo-Ortegon J, Ramirez-Sierra MJ, Arjona-Torres A, Ouaissi A, et al. Comparative evaluation of therapeutic DNA vaccines against Trypanosoma cruzi in mice. FEMS Immunol Med Microbiol 2007; 50:333 - 41; http://dx.doi.org/10.1111/j.1574-695X.2007.00251.x; PMID: 17521394
- Limon-Flores AY, Cervera-Cetina R, Tzec-Arjona JL, Ek-Macias L, Sánchez-Burgos G, Ramirez-Sierra MJ, et al. Effect of a combination DNA vaccine for the prevention and therapy of Trypanosoma cruzi infection in mice: role of CD4+ and CD8+ T cells. Vaccine 2010; 28:7414 - 9; http://dx.doi.org/10.1016/j.vaccine.2010.08.104; PMID: 20850536
- Hotez PJ, Dumonteil E, Heffernan MJ, Bottazzi ME. Innovation for the 'bottom 100 million': eliminating neglected tropical diseases in the Americas through mass drug administration and new vaccines for hookworm and Chagas disease. Adv Exp Med Biol 2012; In press
- Cazorla SI, Frank FM, Malchiodi EL. Vaccination approaches against Trypanosoma cruzi infection. Expert Rev Vaccines 2009; 8:921 - 35; http://dx.doi.org/10.1586/erv.09.45; PMID: 19538117
- Chongqing Jiachen Biotechnology Ltd. Therapeutic Hepatitis B Vaccine (Synthesized Peptide) in Treating Chronic Hepatitis B Patients. 2011.
- Rosenberg ES, Graham BS, Chan ES, Bosch RJ, Stocker V, Maenza J, et al, AIDS Clinical Trials Group A5187 Team. Safety and immunogenicity of therapeutic DNA vaccination in individuals treated with antiretroviral therapy during acute/early HIV-1 infection. PLoS One 2010; 5:e10555; http://dx.doi.org/10.1371/journal.pone.0010555; PMID: 20479938
- Berndt ER, Glennerster R, Kremer MR, Lee J, Levine R, Weizsäcker G, et al. Advance market commitments for vaccines against neglected diseases: estimating costs and effectiveness. Health Econ 2007; 16:491 - 511; http://dx.doi.org/10.1002/hec.1176; PMID: 17013993
- Lee BY, Burke DS. Constructing target product profiles (TPPs) to help vaccines overcome post-approval obstacles. Vaccine 2010; 28:2806 - 9; http://dx.doi.org/10.1016/j.vaccine.2009.09.047; PMID: 19782109
- Schmunis GA. Epidemiology of Chagas disease in non-endemic countries: the role of international migration. Mem Inst Oswaldo Cruz 2007; 102:Suppl 1 75 - 85; http://dx.doi.org/10.1590/S0074-02762007005000093; PMID: 17891282
- Espinosa R, Carrasco HA, Belandria F, Fuenmayor AM, Molina C, González R, et al. Life expectancy analysis in patients with Chagas’ disease: prognosis after one decade (1973-1983). Int J Cardiol 1985; 8:45 - 56; http://dx.doi.org/10.1016/0167-5273(85)90262-1; PMID: 3997291
- Gold M, Siegel J, Russell L, Weinstein M. Cost-Effectiveness in Health and Medicine. New York, 1996.
- Castillo-Riquelme M, Guhl F, Turriago B, Pinto N, Rosas F, Martínez MF, et al. The costs of preventing and treating chagas disease in Colombia. PLoS Negl Trop Dis 2008; 2:e336; http://dx.doi.org/10.1371/journal.pntd.0000336; PMID: 19015725
- Jackson Y, Alirol E, Getaz L, Wolff H, Combescure C, Chappuis F. Tolerance and safety of nifurtimox in patients with chronic chagas disease. Clin Infect Dis 2010; 51:e69 - 75; http://dx.doi.org/10.1086/656917; PMID: 20932171
- Frye JE, ed. International Drug Price Indicator Guide. Arlington: Mangement Sciences for Health, Inc., 2009.
- The World Bank. GDP per capita (current US$). 2009.
- Wilson LS, Strosberg AM, Barrio K. Cost-effectiveness of Chagas disease interventions in latin america and the Caribbean: Markov models. Am J Trop Med Hyg 2005; 73:901 - 10; PMID: 16282301
- Pinotti HW, Habr-Gama A, Cecconello I, Felix VN, Zilberstein B. The surgical treatment of megaesophagus and megacolon. Dig Dis 1993; 11:206 - 15; http://dx.doi.org/10.1159/000171413; PMID: 8222303
- Rassi A Jr., Rassi SG, Rassi A. Sudden death in Chagas’ disease. Arq Bras Cardiol 2001; 76:75 - 96; PMID: 11175486
- World Health Organization. Global Burden of Disease 2004 Update: Disability Weights for Diseases and Conditions. Geneva, 2004.