Abstract
A famous milestone in the vaccine field has been the first successful vaccination against smallpox, in 1798, by Edward Jenner. Using the vaccinia cowpox virus, Jenner was able to protect vaccinees from variola or smallpox. The Modified Virus Ankara (MVA) poxvirus strain has been one of the vaccines subsequently developed to prevent smallpox infection and was selected by the US government in their Biodefense strategy. Progress in molecular biology and immunology associated with MVA infection has led to the development of MVA as vaccine platform, both in the field of preventive and therapeutic vaccines. This later class of therapeutics has witnessed growing interest that has translated into an increasing number of vaccine candidates reaching the clinics. Among those, MVA-based therapeutic vaccines have addressed four major chronic infections including viral hepatitis, AIDS, human papillomavirus-linked pathologies and tuberculosis. Clinical trials encompass phase 1 and 2 and have started to show significant results and promises.
Progress in molecular biology and immunology associated with MVA infection has led to the development of MVA as vaccine platform, both in the field of preventive and therapeutic vaccines. This later class of therapeutics has witnessed growing interest that has translated into an increasing number of vaccine candidates reaching the clinics. Among those, MVA-based therapeutic vaccines have addressed four major chronic infections including viral hepatitis, AIDS, human papillomavirus-linked pathologies and tuberculosis. Clinical trials encompass phase 1 and 2 and have started to show significant results and promises.
Introduction
Therapeutic vaccination is a field still in its infancy when compared with the two words from which it originates: prophylactic vaccination and immune-therapeutics (). Therapeutic vaccines combine features from both of these worlds while also displaying specific ones. Enrollment of the host’s immune system (directly or indirectly) to prevent or fight the established disease is the common pillar in the mechanism of action (MOA) of these three classes of products. While efficacy of prophylactic vaccines is typically based on induction of neutralizing antibodies, that targeted by therapeutic vaccines is expected to rely principally on induction of cellular immunity. Immunotherapeutics such as human monoclonal antibodies have long been considered as “passive” vis-a-vis the host‘s immune system but recent investigations have also demonstrated the implication of active immunological mechanisms as an important contribution to their overall MOA. In case of monoclonal antibodies for example, these include induction of a cytotoxic activity mediated by APCs including macrophages, natural killer cells and implication of CD4+-T lymphocytes (e.g., increase of tumor-associated antigen specific CD4+-T cell responses).Citation1,Citation2
Figure 1: Positioning of therapeutic vaccines: at the interface between two worlds (Capgemini LifeSciences Team Analysis 2006, with permission).
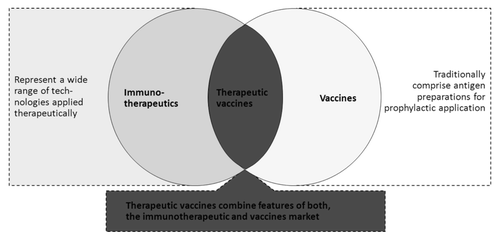
Earlier therapeutic vaccines have used technologies well established in the field of prophylactic vaccination, including the standard adjuvanted recombinant proteins or peptides. The field is nowadays evaluating a whole array of platforms, including vector-based (DNA, virus or bacteria-derived vectors), whole cell-based and dendritic cell-based platforms (). Only a handful of therapeutic vaccines have today reached the market. Those rely on two different technologies: they are either based on dendritic cells (DC) or on adjuvanted recombinant antigens. The Provenge® vaccine aims at treating prostate cancer and is a DC-based vaccine (DCs are loaded with PAP/GM-CSF before re-infusion in the patient).Citation3 It is the first FDA approved therapeutic cancer vaccine and has been on the US market since 2010. CVac™ a vaccine developed by Prima BioMed Ltd to treat ovarian cancer, is also a dendritic-cell based vaccine and is available in Dubai Healthcare City. It consists of the adjuvant mannan attached to the mucin 1 tumor cell surface protein. The product is known as MFP or mannan fusion protein. The treatment is patient-specific and delivered via autologous dendritic cell therapy. Two vaccines are marketed for the treatment of renal cell carcinoma: the Oncophage® vaccine that is based on adjuvanted heat-shock protein, and the CreaVax-RCC® vaccine based again on DCs. The first one is marketed in the Russian Federation, the second in South Korea. Therapeutic vaccines have the potential to address therapeutic areas where unmet medical needs are significant, impacting deeply these markets. They propose paradigm shifts in healthcare treatment, both in oncology and infectious diseases, investigating targets such as breast, colorectal, lung, prostate, pancreatic cancers and chronic infectious diseases such as hepatitis B and C and papillomavirus-associated pathologies.Citation4-Citation7 But even if therapeutic vaccines are one of the ways to approach many of those diseases, future therapeutic breakthroughs will most likely come from the combined use of therapeutic vaccines and standards-of-care, so called “combination approaches”.
We review here some key features of the poxvirus MVA together with recent clinical trials conducted with MVA-based therapeutic vaccines in the field of chronic infectious diseases.
Some Major Features of MVA
Genome organization and virus replication
Produced from Chorioallantoid Vaccinia virus Ankara (CVA), a Turkish smallpox vaccine strain, MVA became defective for replication in human cells after more than 570 passages in primary chicken embryo fibroblast cells (CEFs).Citation8,Citation9 That defect arises from the loss of nearly 30Kb of virus genome compared with the parental CVA, as well as from the emergence of multiple deletions and mutations.Citation10 The lost genes encoded mainly for virulence factors which impacted negatively on host’s immune response to infection. This attenuation obtained by Mayr et al.Citation8 was a key step that allowed for an extended use of MVA in human clinical applications.
Member of the DNA double stranded viruses, MVA encodes tens of proteins required for virus replication. Unlike other DNA viruses, it replicates in the cytoplasm of cells. This specific feature makes it “independent” from the host genome preventing viral genome integration in the cell’s DNA. Poxvirus gene expression is typically controlled by its own promoter and transcriptional machinery. Therefore genes do not contain introns and mRNAs are not spliced.Citation11 Viral replication and gene expression are tightly regulated leading to a complex viral assembly characterized by the emergence of different forms of viral particles. In vitro studies have shown that after virus entry, early genes are transcribed and lead to viral DNA replication in a “replication factory”. Intermediate and late genes are then expressed and viral DNA is incorporated into immature virions which then become, infectious intracellular mature particles.Citation12 This process is well described in vitro but remains yet to be documented in vivo, following administration in a host. It has been shown that MVA life-cycle stops before or during this step in non-permissive cells, whereas other poxviruses can in such context continue their maturation. Thus, in human cells, MVA initiates a replication but is unable to productively grow and generate mature infectious particles.Citation13,Citation14 This scenario differs widely from that taking place in cells capable to support full replication of the virus such as BHK-21, CEFs and other avian cells.Citation15,Citation16
One interesting feature of MVA is its genome plasticity which allows large amounts of foreign DNA (at least 25 kb) to be incorporated without loss of infectivity while still retaining high levels of gene expression. Processes leading to the generation of recombinant MVA vectors are well established.Citation17 They require a genomic insertion by homologous recombination between the viral genome and the DNA from the shuttle plasmid that carries the immunogenic sequences of interest encoded under Vaccinia Virus (VV) specific promoters. Deletion sites within the MVA genome or the gene loci encoding for VV proteins such as those encoding for thymidine kinase or hemagglutinin, are today the classically used sites for the insertion of recombinant gene sequences.Citation18
Antigen presentation.
An adaptive immune response relies on antigen presentation by professional antigen-presenting cells (APC) such as dendritic cells (DC). This presentation of viral antigen, through the class I-restricted major histocompatibility complex, can derive from intracellular or extracellular sources whether peptides are generated and presented following infection of the APC itself or from cross-presentation during which APC acquires the antigen produced by other infected cells.Citation19
MVA infects cells through a passive membrane fusion and therefore possess a wide tropism infecting a large panel of cells, among which antigen presenting cells.Citation20 Published data have indicated that MVA has a pronounced tropism for monocytes, macrophages, but can also infect some DC and B cells, all of which are capable of acting as professional APCs.Citation21 MVA infection of DC inhibits their phagocytic and migratory activity and induces DC apoptosis.Citation22 This rapid induction of death by apoptosis seems to apply also to other cell types. Indeed, initially described in HeLa cells, it has been subsequently described by Brandler et al. for different cell types.Citation23 These authors and others showed that a recombinant MVA expressing HIV Gag, Pol, Nef (MVA-HIV) induces, following in vitro infection, apoptotic cell death with morphological changes noticeable at 6 h post-exposure.Citation23,Citation24 It was shown-in in vitro cultures, that this time period allows for activation of cytotoxic T cell clones by DC although this timing may not be sufficient to prime T-cell responses in vivo. Further studies are required to clarify this point.
A number of studies have addressed the issue of mechanism associated with antigen presentation following MVA infection. Tested in a mouse model, MVA-infected DCs did not prime T-cell responses, whereas injection of MHC-deficient MVA-infected cells led to specific T-cell priming.Citation25 This study and others strongly suggest that cross-presentation is likely the primary mechanism of action responsible for the immunogenicity achieved following MVA vaccination.Citation26,Citation27 Furthermore, MVA is known to trigger early migration of leukocytes at the site of infection.Citation28 DCs are expected to be recruited at the inoculation site, to sample MVA-infected dying cells (e.g., fibroblasts and adipocytes) and following maturation to migrate to lymph nodes and activate antigen-specific T cells.Citation29,Citation30 Much less is known about the sensitivity of primary connective, epithelial or muscle cells to MVA infection. These cells could be some of the first cells, along with DCs, targeted by the vector following mucosal intramuscular or subcutaneous vaccination. Finally, an element becoming increasingly important for an efficient cross-presentation is the stability of the expressed immunogen. Gasteiger et al., proposed that an immunogen produced by MVA as long-lived mature protein is the best substrate for efficient TCD8 priming.Citation25
Innate immune response
In response to a viral infection, mammalian cells are able to produce inflammatory cytokines and interferon to resolve this infection. This production is mediated through virus recognition by Toll Like Receptors (TLR). Among host defenses, early pathogen recognition process is critical for alerting the host to the presence of foreign and dangerous elements, and to start an innate immune response followed by an efficient adaptive immune response.
is summarizing the principal experimental approaches that have been pursued to decipher the relationship between MVA and the host’s innate immune system. Many studies have demonstrated that VVs are mainly recognized by the TLR-2 pathway through cytoplasmic MyD88 and induce a MIP-2 production. But TLR independent recognition was also observed in macrophages and DCs leading to type I interferon secretion.Citation31,Citation32 The main difference between VV and MVA is the absence of VV immunomodulatory genes in MVA which leads to an MVA specific and complex innate immune response. MVA genome deletion has suppressed genes expressing homologous TNF, type I interferon and IFN-γ receptors, which are responsible for some host immune response abrogation. However, MVA retains an IL-1β receptor homolog, leading to a reduced virulence and to a prolonged viral persistence.
Table 1: Principal experimental approaches used to study the interplay between MVA and the host’s innate immune system
Among the different sensings of innate immunity by MVA, involvement of different TLRs was documented (mainly TLR-2 to TLR-6) together with the cytosolic sensor MDA-5 and the NALP3 inflammasome. Complexity of cell signaling by MVA is growing as mechanisms are unraveled. Micro-array analysis have been implemented to demonstrate that infection of DCs by MVA induces expression of genes associated with innate immunity such as IFN-β, TNF-α, RIG-1, MDA-5 and many TLRs.Citation24 Specific studies have addressed more in details the implication of TLRs and have shown, for example, that the absence of TLR-2, TLR-6 and MyD88 in bone marrow macrophages, obtained from mice deficient in these molecules, leads to the extinction of MIP-2 expression but not of the type I interferons. These observations suggest that separate pathways exist for cytokine secretion. The role of TLR-7 has also been studied using murine bone marrow cells treated with chloroquine (an endosome acidification inhibitor). Chloroquine treatment leads to inhibition of the type I interferon response suggesting that TLR-7, the only other compatible MyD88 dependent endosomal TLR remaining, was involved.Citation33,Citation34 These studies have also shown that in addition to interferons, MVA-infected DCs secrete cytokines and chemokines, including RANTES, MIP-1α, IL-6, and TNF. Following infection of macrophages different pathways seem to be activated, including those involving NFκb, ERK1/2, JNK, IRF3, IRF7 and STAT1.Citation28 Another study has documented that the up-regulation of CD40 on infected DCs partially depends on TLR-9 sensing; however T cell expression of CD69, IFN-α and IL-6 was unaffected by the absence of TLR-9, which may be redundant.Citation35
In parallel, TLR independent type I responses have also been demonstrated after infection of human THP-1 cells resulting in the up-regulation of the cytoplasmic RNA sensors MDA-5, RIG-1 as well as IPS-1.Citation33,Citation36 These results indicate that MDA-5 is important in sensing viral RNA derived from MVA transcription.
Finally, MVA has been shown to activate the inflammasome. The inflammasome, a molecular cytosolic complex, assembles after an infection and leads to caspase-1 activation, and to the cleavage and the release of active forms of inflammatory mediators like IL-1β, IL-18 and immune potentiating danger associated molecular patterns. For example, infection by MVA induces cooperation between TLR-2 and the NLRP3 inflammasome leading to IL-1β production and caspase-1 activation.Citation33,Citation37 A recent study by Hornung et al., has also shown that murine macrophages loose cell viability after infection with vaccinia virus and that this event requires the DNA inflammasome protein AIM-2.Citation38 A more recent study suggests the same involvement of AIM-2 on MVA intracellular sensing.Citation39
Overall, because of its attractive properties, the poxvirus MVA has become one important platform in the development of therapeutic vaccines. Highly stable recombinants that have the capacity to express large open-reading frames can be generated. Appropriate innate “danger signals” directed toward the adaptative immune system can be generated as MVA mimics to some extend viral infection, resulting in a particular strong induction of cellular-based immunity. This replication-defective virus provides major features of a killed virus vaccine with the immunogenicity of a live virus vaccine.
MVA-based clinical efforts in chronic infectious diseases
There are currently four major infectious agents targeted by MVA-based therapeutic vaccines: human immunodeficiency virus (HIV), hepatitis C virus (HCV), human papillomavirus (HPV) and Mycobacterium tuberculosis (Mtb). Current clinical trials include phase I and phase IIa and IIb (). MVA-vectors have been used for more than 40 years for vaccination or for gene therapy without any major adverse effect (for a review see refs. Citation40 and Citation41). MVA has proven to be safely tolerated including in patients with HIV infection. Typical safety profile is similar across the different MVA-based vaccines and consists in mild to moderate injection site reactions and flu-like symptoms. The local moderate reactogenicity doesn’t seem to differ significantly between the different MVA based therapeutic vaccines, and seems also identical in patients and in healthy subjects. Earlier studies have been conducted in immuno-compromized patients including HIV infected patients receiving HAART.Citation42,Citation43 This good safety is possibly related to the fact that the local induced infection is self-limited by the absence of new infectious viral particles production. In all recent and ongoing trials described thereafter, no serious adverse events (SAE) have been reported when MVA vaccines are administered alone in chronically infected patients. A few cases of SAE were reported recently in the ongoing TG4040-based trial HCVac (see below section on Hepatitis Vaccines). In this trial, four patients who received a combination of PegIFN/ribavirin and TG4040 developed either severe peripheral thrombocytopenia and aplastic anemia, conditions that were never observed when the MVA vaccine was administered as stand-alone in HCV patients. All four patients recovered following treatment and analysis are under investigation to clarify potential mechanism of action. A common allele, HLA-DRB1*04, known to be associated with development of such events were found shared between the three patients with thrombocytopenia (JM Limacher personal communication and reported during the EASL conference, Barcelona, April 2012).
Table 2 : Current MVA-based clinical trials in infectious diseases
HPV vaccines.
Carcinoma of the uterine cervix, or cervical cancer (CxCa) is the second most frequent cause of death by neoplasia in women worldwide. Half a million new cases worldwide each year and nearly 300 000 and 30,000 deaths, respectively, are estimated yearly worldwide and in European countries.Citation44,Citation45 The two recently approved prophylactic vaccines, both targeting the prevalent genotypes HPV 16 and 18 found in cervical cancer ( Cervarix) and also HPV 6 and 11 for one of them (Gardasil), are expected to greatly help in reducing CxCa morbidity and mortality.Citation46 Yet, control of HPV infection will take years and the need remains particularly acute in developing countries. Different stages of the HPV-induced cervical neoplastic diseases can be targeted by therapeutic vaccination. A first indication of therapeutic vaccination could be to provide infected women suffering from high-grade intra-epithelial neoplasia (CIN2/3) with an alternative to the current surgical-based standard-of-care that is the Loop Electrical Excision Procedure (LEEP). There are basically two programs that are using MVA as central vaccine platform in the development of therapeutic HPV vaccines targeting neoplasia.
The first program includes three studies reported by the same group on the use of a MVA expressing the BPV 1 E2 protein (MVA-E2). The basic concept of this vaccine relies on the property of the E2 protein to promote cell arrest and apoptosis in HeLa cellsCitation47 and not so much on the immunogenic properties of E2. These studies report trials based on the direct injection of the vaccine into pathological sites (lesions). In two trials,Citation7,Citation48 MVA-E2 was administered directly in the cervix of women with CIN 1, 2 or 3 (multiple doses at weekly intervals). Evolution of lesions was followed weekly by colposcopy and cytology analysis. In the vaccinated group, two partial remissions were observed out of the 36 vaccinees, while 34 of them showed complete remission. Viral DNA was nonetheless cleared only in 50% of women. All vaccinees developed antibody responses to the MVA-E2 and generated a cytotoxic response against papillomavirus-transformed cells. Another trial targeted only women with high-grade CIN lesions.Citation49 Complete remission of lesions was seen in 20 out of the 34 vaccinees. As in the previous study, antibodies against the vector and E2 were detected in all cases as well as cytotoxic responses against HPV-transformed cells. Of note, the antibody response correlated with the regression of lesions and the extent of response was correlated with the size of initial lesions, i.e., small lesions were completely eliminated after treatment. Administration of the MVA-E2 did not generate major adverse effects. Finally, a third trial was conducted in men with intraurethral flat condiloma and showed very similar immunogenicity and efficacy and no adverse events were observed.Citation50 Overall, unfortunately, no placebo control was included in two of the three conducted studies. This clearly poses a problem with respect to the high rate of spontaneous resolution observed in CIN situations (> 20‒25%, up to 50%). Of note, the size of the lesions was evaluated by colposcopy. No description of E2-specific T cells was provided in any of the studies and because no control empty MVA vaccine was used in these studies, it remains difficult to formally conclude on the role played by the vaccine-expressed E2 protein.
The biotech company Transgene has developed a HPV-therapeutic vaccine using a MVA expressing the E6 and E7 proteins from HPV 16 (TG4001). Both viral proteins were selected as they are expected to be expressed in late stages of the infection, in particular in CIN 2 and CIN 3 carrying women. This vaccine given subcutaneously aims at inducing strong cellular immune responses susceptible to recognize infected cells and to clear them. Earlier clinical trials were reported showing overall safety of the vaccine and those lead to the conduct of a Phase 2a trial in 21 women with HPV 16-related CIN 2/3.Citation51 Subjects received three weekly subcutaneous injections of TG4001. Regression of the CIN 2/3 lesions and clearance of the HPV 16 infection were monitored by cytology, colposcopy and HPV DNA/messenger RNA (mRNA) detection. Ten patients (48%) were evaluated as clinical responders at month 6. Nine patients experienced an improvement of their HPV 16 infection, by mRNA+/- eradication. HPV 16 mRNA clearance was associated with CIN 2/3 cytologic and colposcopic regression in 7/10 patients. At month 12, 7/8 patients without conization reported neither suspicion of CIN 2/3 relapse nor HPV 16 re-infection. The remaining patient was lost to follow-up. These data were considered very encouraging in particular those reporting on mRNA clearance. Unfortunately, no immunological analysis was reported in this trial and correlation between induction, type and robustness of HPV-specific immune responses induced by TG4001 and viral efficacy has yet to be analysed. A larger clinical trial (phase 2b) has then been launched to confirm these results (NCT01022346). The trial included 206 patients with CIN 2/3 related to high risk HPV, and patients were randomized 2 to 1 against placebo. Primary end-point was histological resolution at 6 months defined as a complete disappearance of CIN; histological response (no CIN or reduction to CIN1), cytological as well as virological evolution over time were part of the secondary endpoints. The vaccine, RG3484 (or RO5217790)/TG4001, was given subcutaneously three times at days 1-8-15. Headline results of this trial made public in May 2012 (Transgene press release) showed a significant improvement of both resolution: (25% in vaccinees vs. 10% in the placebo group), overall response rate (36% vs. 21%) and DNA-based viral clearance (37% vs. 14%) 6 months after vaccination. Although this level of response remains below expectations to provide for an alternative to surgery in CIN2/3, the proof of concept of therapeutic vaccination is sufficient to consider this vector in combination with chemotherapy in more advanced settings such as cervical cancers where medical need is much higher. Another positioning of the TG4001/RO5217790 may be in the treatment of the 30 to 40% head and neck tumors related to a HPV infection.Citation52
In contrast to preventive vaccination, implementation of HPV therapeutic vaccination to treat CIN diseases will remain a challenge and may be of limited utility in most developing countries as they do not carry effective screening programs to identify the lesions
HCV vaccines.
Roughly 170 to 200 million people are infected worldwide by hepatitis C virus (HCV) with another 3 million people newly infected each year.Citation53 HCV infection has become the most common cause of chronic liver diseases in developed countries where it has even become the major cause of cirrhosis and liver cancer and therefore first indication of liver transplantation. Major progress has been achieved in the treatment of chronically infected subjects, with a cure rate in treated patients ranging from 35-55% with a combination regimen of pegIFN-α and ribavirin to up to 70% and more in genotype 1 patients when combining this regimen with first generation of anti-proteases.Citation54,Citation55 HCV treatment is moving to more complex drug combinations as second generations of anti-proteases and anti-polymerases as well as other small molecules are being developed.Citation56 It is becoming increasingly clear that the field is moving towards interferon-free regimens, yet, it remains to be proven that complete suppression of interferon will not decrease the chances of cure, in particular for hard-to-treat patients. Development of HCV therapeutic vaccines falls within the expanding field of immunotherapeutic strategies aimed at exploiting mechanisms of action different than those typically enrolled with the newly developed small agents.Citation6 A HCV therapeutic vaccine capable of increasing cure rate in hard-to-treat patients, reducing duration of treatment without adding to the toxic burden would be a major contribution. One correlate of protection has emerged from human but also chimpanzee studies: HCV patients displaying an early functional and maintained Th1-oriented CD4+ T lymphocytes-mediated response, typically associated with the mounting of matured and multifunctional effector CD8+ lymphocytes, exert a more efficient control of viremia and are more prone to evolve toward recovery.Citation57 Multiple studies have by now established a correlation between control of viremia and/or disease and the mounting of T-cell responses targeting non-structural proteins, in particular the non-structural protein 3 (NS3).Citation58
Two MVA-based HCV therapeutic vaccines are currently being developed. One referred to as MVA-NSmut, developed by the company Okairos is currently being administered to healthy volunteers as well as HCV chronic patients, either as stand-alone or in a prime-boost regimen with an adenovirus counterpart (chimpanzee adenovirus type 3) (NCT01296451). This MVA expresses all of HCV non-structural (NS) proteins. This first trial aims to assess safety of the vaccine combination, secondary end-points include measures of vaccine immunogenicity and potential impact on viral load. The MVA-NSmut is administered at a dose of 2 x 108 pfu as the booster vaccine. No data is yet available from this trial.
Transgene has developed an original MVA expressing three of HCV NS proteins (NS3, NS4 and NS5B) and has shown in pre-clinical models that this vaccine induces robust, long-lasting, poly-functional T-cells capable of exerting effector functions after migrating to the liver.Citation59 A phase I clinical trial in treatment-naïve patients with chronic hepatitis C has been performed with the vaccine, so called TG4040 (NCT00529321).Citation60 Three vaccine doses were tested in this trial (106, 107 and 108 pfu). They were administered weekly at one week interval with a booster injection performed 6 months later in the highest dose group. TG4040 was shown to be well tolerated (primary end-point of the trial) and to induce HCV-specific cellular immune responses observed in 5 out of 15 of the vaccinees. The number of subjects in the trial was too low and vaccine doses too diverse (three doses tested) to conclude on patients’ profile or patients’ specifics that will be associated with the mounting of detectable T-cell responses. A larger trial will be required to properly address this issue. In addition, a transient viral load decrease was observed, ranging from 0.5 to 1.24 log10 in 8 patients consistently around 37 days post-initial vaccination i.e., at the expected peak of immune response. Interestingly, this trial pointed to the superiority of the two lower vaccine doses rather than the higher one and opened the road to the conduct of a phase 2 clinical trial in treatment-naïve patients this time in association with standard-of-care (referred to as “SOC”). The HCVac trial (NCT01055821), including 153 patients, aims at testing the value of TG4040 in association with the most widely used therapy based on pegIFN-α and ribavirin. Two hypotheses were tested: a “lead-in” vaccination period prior to initiation of SOC versus a “lead-in” SOC treatment period prior to initiation of vaccination. A third arm represents the control group receiving the SOC alone. This trial has met its primary end-point which was improvement of the so called cEVR (complete Early-Viral Response) indicative of patients who have a non-detectable viremia 12 weeks following initiation of SOC). Indeed, the number of patients reaching cEVR in the “lead-in” vaccine arm was basically doubled compared with that observed in the SOC arm.Citation61 This is the first HCV therapeutic vaccine capable to demonstrate a potent early antiviral effect when associated with therapy, reaching a cEVR level of efficacy close to what has been reported with the first generation of anti-proteases combined with SOC.Citation54,Citation55 This study also showed the importance of the sequence and schedule of administration of the therapeutic vaccine when given in association with SOC. The expected MOA of this vaccine is represented in . Following subcutaneous administration of TG4040, antigen presentation and priming of T-cells is foreseen to happen in lymphoid organs (not in the liver where most of these events are likely to happen during natural infection by HCV as massive expression of viral antigens is found in hepatocytes). Primed T-cells but also likely other cells from the innate immune system (see first section of this review), are expected to migrate from lymphoid organs to the liver and exert their effector functions, including cytolytic and/or non-cytolytic activities, and to contribute to the control and/or clearance of infected hepatocytes. Such functions were demonstrated to some extent in an alternative murine pre-clinical model used to validate TG4040 activity.Citation59
Figure 3: Postulated Mechanisms of Action of TG4040. Following subcutaneous administration of TG4040, antigen presentation and priming of T-cells is foreseen to happen in lymphoid organs. Primed T-cells but also likely other cells from the innate immune system are expected to migrate to the liver and exert their effector functions, including cytolytic and/or non-cytolytic activities, and contribute to the control and/or clearance of infected hepatocytes
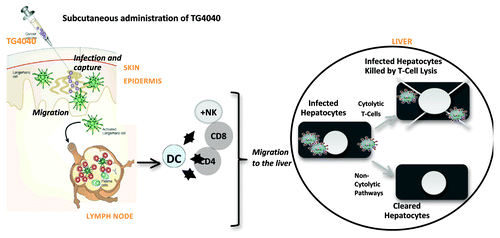
Finally, it is interesting to mention another MVA-based trial in the hepatitis field although not targeting HCV but chronic hepatitis B. The trial has been completed many years ago but was only recently published. This trial reports a prime-boost approach combining a DNA and a MVA vaccine expressing the hepatitis B surface antigen, in association in some experimental arms with lamivudine.Citation62 This assay was complex, displaying heterogeneity at different levels (e.g., different patients were consecutively enrolled, including both HBeAg+ , HBeAg- carriers, with different history of infection and from different geographical areas). A total of 72 patients were enrolled and while safety of the vaccine combination was established (overall, only mild local or systemic adverse events were observed, in particular no ALT or AST elevations were detected), no effect of the vaccine administration could be demonstrated in any of the cohorts. Curiously, IFN-γ producing cells detected by ELISPOT assay were made by antigen non-specific CD16+ cells. This disappointing result may reflect a wrong choice of immunogen, the wrong selection of patients in the trial, alternatively the fact that MVA is not the appropriate platform to develop a HBV therapeutic vaccine.
HIV vaccines.
More than 40 million people are infected worldwide with HIV, with an estimated 15,000- 20,000 new infections every day (UNAIDS). Despite more than 15 years of research, an effective vaccine against HIV and acquired immunodeficiency syndrome (AIDS) has still not been developed. Immunologists have gathered considerable evidence that cellular immune responses can effectively control HIV-1 replication during acute and chronic infections thereby possibly protecting individuals from infection and preventing the spread of HIV. Ideally, a vaccine should induce responses specific to immunologically conserved regions in order to be truly effective. HIV-MVA vaccines are largely developed for prophylactic application while only a handful of the candidates are for therapeutic use. Three NIH reported trials are listed.
The NCT00390078 trial (Completed).
This trial is a single-blind, controlled safety and immunogenicity study of recombinant MVA to treat HIV Infection. The MVA-mBN32 vaccine is an epitope-based vaccine encompassing conserved CTL and HTL epitopes of HIV-1 and thus has been designed to elicit a polyfunctional immune response with a targeted focus to conserved epitopes. In this study the safety, tolerability and immunogenicity of the recombinant MVA-mBN32 vs. the control vector MVA-BN® was examined in 30 HIV-infected subjects. Data have not yet been reported but future reports are expected to describe analysis of CD4+ T helper cells and CD8+ CTL responses to these epitopes spanning different viral antigens.
The NCT01378156 trial (ongoing)
This trial is a safety and immunogenicity study of a Plasmid DNA Prime and MVA Boost Vaccine in HIV-1 infected adults on ART (Anti-Retroviral Therapy). Both vaccines express Gag, Pol, and Env. More precisely, GV-TH-01 is an open label Phase 1 study of 10 HIV-1 infected adults with suppressed viremia who started ART within 18 months of a negative HIV antibody test. This original study includes 3 phases. In the first phase, patients are vaccinated with the pGA2/JS7 DNA and MVA vaccine following a prime/boost regimen at weeks 1 and 9 (DNA vaccine) and weeks 17 and 25 (MVA vaccine). The second phase of the study is a treatment interruption phase, whereby ART is interrupted for a 12 week period approximately 8 weeks following the last vaccination. The third phase occurs after the 12 week treatment interruption phase and is called the treatment reinstitution phase, because subjects reinstitute ART and are followed for an additional 24 weeks. This unique trial should be very informative as it is looking into fine interplay between standard-of-care and vaccination. In addition, it combines two vaccines (DNA and MVA) in a regimen (prime-boost) mainly described so far in preventive vaccination. The vaccine was well tolerated with only mild local adverse effects. Results from a previous phase I showed that HIV-specific T-cell responses were regimen-dependent.Citation63 T-cell responses were primarily generated against Gag and Env and were shown to be directly correlated with the number of MVA boosts.
The NCT00189930 (completed) is aiming to evaluate the immunogenicity and safety of two doses of MVA-Nef vs. MVA-BN in HIV-1 infected patients. Primary outcome includes the measure of T-cell response against MVA-BN and the Nef antigen assessed by intracellular cytokine staining assay (ICS). Secondary end-points include occurrence, intensity and relationship of adverse events occurring at any time during the study. Again, data have not yet been published.
An earlier trial by Greenough et al, using one of the most complex HIV therapeutic vaccine reports a study in young adults infected with HIV.Citation64 Patients were all under ART and received a MVA vaccine expressing Env, Gag, Tat, Rev and Nef-RT. They also received a booster vaccination with a counterpart fowlpox vaccine. In this study, it was possible to detect clear increased frequencies of CD4+ and CD8+ specific responses in blood of vaccines yet those did not translate into an effect neither on the plasma HIV—specific antibody response—nor on their neutralizing activity.
Interestingly, HIV-MVA vaccines seem to evolve toward a greater complexity i.e., including a larger array of immunogens such as those being tested in recent development by Bavarian Nordic (MVA-BN-HIV covering eight HIV antigens described above). Clearly today, only poxvirus-based platforms have the capacity to cover such large number of antigens. Overall, MVA-based HIV therapeutic vaccines have not yet delivered convincing data.
TB vaccines.
Tuberculosis (TB) remains a major cause of morbidity and mortality worldwide. One third of the world population is expected to be infected by TB (so called “latent tuberculosis”), each year, about 9 million new cases of TB are detected and 2 million will die from consequences of active infection.Citation65 Tuberculosis infection is far from being controlled for different reasons: poor patient compliance with the prescribed standard-of-care in areas with limited resources and full access to health care is increasingly leading to emergence of drug resistant strains (including multi-drug and extra-drug resistant strains ( MDR and XDR) , exacerbation of TB epidemics linked to that of HIV, poor performance of BCG vaccination which is moderately protective against disseminated TB in children, but ineffective in protecting against the prevalent adult pulmonary TB. Two concepts are emerging in the field of TB vaccine development: that of trying to vaccinate “latently” infected subjects in order to prevent “reactivation” of the bacteria, and that of using a vaccine as an adjunct to anti-tuberculosis chemotherapy in particular for the treatment of MDR/XDR strains (with the goal to shorten duration of treatment, increase cure rate) . Development in these areas can be assimilated to development of “therapeutic vaccines” in as much as these TB vaccines will be applied to the already infected host.Citation66 While the second positioning is clearly yet in pre-clinical stage of development and has shown a wide range of results (from encouraging to totally disappointing),Citation67-Citation69 the first positioning has already been tested to some extent in the clinic with a MVA-based vaccine that has shown protection in a variety of pre-clinical models.Citation70
The first phase 1 study using this vaccine, a MVA expressing antigen 85A (MVA85A), was performed in healthy volunteers. The vaccine was found to induce high levels of antigen-specific IFN-γ-secreting T cells when used alone in bacillus Calmette-Guerin (BCG)-naïve healthy volunteers. When administered in volunteers who had been vaccinated 0.5‒38 years previously with BCG, higher levels of antigen-specific IFN-γ-secreting T cells were induced. In a longer follow-up, (24 weeks after vaccination) these levels were 5‒30 times greater than those observed in vaccines who were administered a single BCG vaccination. This first clinical study indicated that boosting vaccinations with MVA85A could offer an efficient way for enhancing and prolonging anti-mycobacterial immunity in tuberculosis-endemic areas.Citation71 Since this trial, MVA85A has been used in more than 15 trials () and is currently being tested in extended Phase 2 clinical trials in Africa.Citation72 Its safety has been first demonstrated in latently infected (LTBI) subjects from the UK. In these subjects, induction of potent antigen-specific IFN-γ and Il-2 producing T-cells has been shown following a single intra-dermal injection of the MVA85A (5 × 107 pfu) but also in healthy adults, infants and LTBI subjects in endemic populations from The Gambia and South Africa.Citation73,Citation74 An interesting feature of this vaccine is its apparent capacity at overall inducing poly-functional T- cells expressing a variety of cytokines including IFN-γ , TNF-α, Il-2 and Il-17,Citation75 a profile that has been reported to be important the control of chronic infection. Although not in a therapeutic setting, it is worth mentioning that the largest trial in the TB field ever carried out since the development of BCG is currently being conducted with the MVA85A in Gambian infants. This trial aims at evaluating safety and efficacy of the MVA85A in a BCG-booster setting. The purpose of the study was to assess whether MVA85A can stimulate immune responses against the tuberculosis bacteria in infants and whether it could feasibly be given at the same time as other childhood vaccines as part of the EPI (Expanded Program of Vaccination). The randomised trial involves 214 healthy four-month-old infants, who had already received BCG at birth. Children were given the EPI alone, MVA85A alone or MVA85A in conjunction with the EPI.Citation76 Overall, MVA85A was deemed to be safe and well tolerated and induced a strong immune response. Importantly, the responses to the standard EPI vaccines were not affected by giving MVA85A at the same time; however, the immune response to MVA85A was lower in infants who received it in conjunction with EPI vaccines than in infants who received the new vaccine alone. It is not clear whether the immune response that is generated in conjunction with EPI will be sufficient to confer protection against Mtb. An efficacy trial of a higher dose of the vaccine in South African BCG-vaccinated infants is being conducted and results are expected to be available by end of 2012.
Figure 4: Clinical trials (target population and time lines) performed with the MVA85A. (With permission from McShane; 2011).
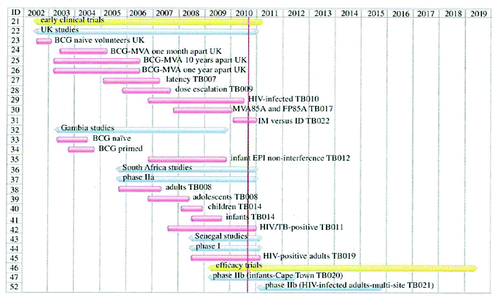
Although MVA85A has yet to be tested as an adjunct to standard-of-care in MDR/XDR subjects, the complex development of this vaccine illustrates well the close interplay between a prophylactic and a therapeutic development and how this interplay can enrich each respective development. The recent demonstration that the Oka/Merck VZV vaccine, originally developed for prophylaxis in young children, was capable to reduce incidence and burden of illness linked to VZV reactivation in elderlyCitation77 has in fact paved the ground for use of therapeutic vaccination in “latently” infected subjects.
Conclusion
Treatment of chronic infectious diseases remains a challenge for a majority of infections either because curative treatments do not exist (e.g., HIV/AIDS) or are seldom efficient (e.g., HBV infection) or remain toxic (e.g., IFN-α tolerance in HCV treatment). The field is clearly moving towards increasingly complex drug combinations including better tolerated combinations. The most efficient drug regimens today combine small molecules displaying complementary mechanism of action (e.g., anti-polymerases and anti-proteases such as witnessed in the HCV therapeutic field).Citation54,Citation55 We have illustrated in this review how a novel class of therapeutics, so called therapeutic vaccines based on the poxvirus MVA platform, is becoming an active player in paradigm shifts witnessed in healthcare treatment of chronic diseases. While the MOA underlying clinical efficacy so far reported with therapeutic vaccines in general and MVA-based ones in particular, are not yet fully characterized, it is expected that contribution of both innate and adaptative responses is key. The next rounds of clinical trials performed in the frame of “combination regimens” with standard-of-care and reaching now phase 2b and 3 will be critical for a full appreciation of the old but yet full of resources vaccine platform that is MVA.
Abbreviations: | ||
AIDS | = | acquired immunodeficiency syndrome |
ALT | = | alanine aminotransferase |
APC | = | antigen presenting cells |
ART | = | anti-retroviral therapy |
AST | = | aspartate aminotransferase |
BCG | = | bacillus calmette-guerin |
BPV | = | bovine papillomavirus |
BHK-21 | = | baby hamster kidney (fibroblasts) |
CEF | = | chicken embryo fibroblast cells |
CVA | = | Chorioallantoid vaccinia virus ankara |
cEVR | = | complete early-viral response |
CxCA | = | cervical cancer |
DC | = | dendritic cells |
DNA | = | deoxyribonucleic acid |
EPI | = | expanded program of immunization |
HCV | = | hepatitis C virus |
HBV | = | hepatitis B virus |
HIV | = | human immunodeficiency virus |
HPV | = | human papillomavirus |
MDR | = | multi-drug resistance |
MOA | = | mechanism of action |
Mtb | = | mycobacterium tuberculosis |
MV | = | mature virions |
MVA | = | modified virus ankara |
NS | = | non-structural |
PAP | = | prostatic acid phosphatase |
RPA | = | RNase protection assay |
SAE | = | serious adverse events |
SOC | = | standard-of-care |
TB | = | tuberculosis |
THP | = | human acute monocytic leukemia cell line |
TLR | = | toll like receptor |
TNF | = | tumor necrosis factor |
VV | = | vaccinia virus |
XDR | = | extra-drug resistance |
WT | = | wild type |
Acknowledgements
Our warm thanks to, Nathalie Silvestre, Karola Rittner, Perrine Martin and Sophie Jallat for critical reading of the manuscript, Teobaldo Benites for his priceless help in bibliography search and Jean Yves Bonnefoy and Philippe Archinard for their constant support.
References
- Abès R, Gélizé E, Fridman WH, Teillaud JL. Long-lasting antitumor protection by anti-CD20 antibody through cellular immune response. Blood 2010; 116:926 - 34; http://dx.doi.org/10.1182/blood-2009-10-248609; PMID: 20439625
- Taylor C, Hershman D, Shah N, Suciu-Foca N, Petrylak DP, Taub R, et al. Augmented HER-2 specific immunity during treatment with trastuzumab and chemotherapy. Clin Cancer Res 2007; 13:5133 - 43; http://dx.doi.org/10.1158/1078-0432.CCR-07-0507; PMID: 17785568
- Kantoff PW, Higano CS, Shore ND, Berger ER, Small EJ, Penson DF, et al, IMPACT Study Investigators. Sipuleucel-T immunotherapy for castration-resistant prostate cancer. N Engl J Med 2010; 363:411 - 22; http://dx.doi.org/10.1056/NEJMoa1001294; PMID: 20818862
- Acres B, Bonnefoy JY. Clinical development of MVA-based therapeutic cancer vaccines. Expert Rev Vaccines 2008; 7:889 - 93; http://dx.doi.org/10.1586/14760584.7.7.889; PMID: 18767940
- Michel ML, Deng Q, Mancini-Bourgine M. Therapeutic vaccines and immune-based therapies for the treatment of chronic hepatitis B: perspectives and challenges. J Hepatol 2011; 54:1286 - 96; http://dx.doi.org/10.1016/j.jhep.2010.12.031; PMID: 21238516
- Inchauspe G, Bach G, Martin P, Bonnefoy JY. Vaccination against hepatitis B and C: towards therapeutic application. Int Rev Immunol 2009; 28:7 - 19; http://dx.doi.org/10.1080/08830180802488436; PMID: 19241251
- Cid-Arregui A. Therapeutic vaccines against human papillomavirus and cervical cancer. Open Virol J 2009; 3:67 - 83; http://dx.doi.org/10.2174/1874357900903010067; PMID: 19915722
- Mayr A, Stickl H, Müller HK, Danner K, Singer H. [The smallpox vaccination strain MVA: marker, genetic structure, experience gained with the parenteral vaccination and behavior in organisms with a debilitated defence mechanism (author’s transl)]. Zentralbl Bakteriol B 1978; 167:375 - 90; PMID: 219640
- Sutter G, Staib C. Vaccinia vectors as candidate vaccines: the development of modified vaccinia virus Ankara for antigen delivery. Curr Drug Targets Infect Disord 2003; 3:263 - 71; http://dx.doi.org/10.2174/1568005033481123; PMID: 14529359
- Antoine G, Scheiflinger F, Dorner F, Falkner FG. The complete genomic sequence of the modified vaccinia Ankara strain: comparison with other orthopoxviruses. Virology 1998; 244:365 - 96; http://dx.doi.org/10.1006/viro.1998.9123; PMID: 9601507
- Tolonen N, Doglio L, Schleich S, Krijnse Locker J. Vaccinia virus DNA replication occurs in endoplasmic reticulum-enclosed cytoplasmic mini-nuclei. Mol Biol Cell 2001; 12:2031 - 46; PMID: 11452001
- Moss B. Poxvirus entry and membrane fusion. Virology 2006; 344:48 - 54; http://dx.doi.org/10.1016/j.virol.2005.09.037; PMID: 16364735
- Gallego-Gómez JC, Risco C, Rodríguez D, Cabezas P, Guerra S, Carrascosa JL, et al. Differences in virus-induced cell morphology and in virus maturation between MVA and other strains (WR, Ankara, and NYCBH) of vaccinia virus in infected human cells. J Virol 2003; 77:10606 - 22; http://dx.doi.org/10.1128/JVI.77.19.10606-10622.2003; PMID: 12970445
- Sancho MC, Schleich S, Griffiths G, Krijnse-Locker J. The block in assembly of modified vaccinia virus Ankara in HeLa cells reveals new insights into vaccinia virus morphogenesis. J Virol 2002; 76:8318 - 34; http://dx.doi.org/10.1128/JVI.76.16.8318-8334.2002; PMID: 12134037
- Drexler I, Heller K, Wahren B, Erfle V, Sutter G. Highly attenuated modified vaccinia virus Ankara replicates in baby hamster kidney cells, a potential host for virus propagation, but not in various human transformed and primary cells. J Gen Virol 1998; 79:347 - 52; PMID: 9472619
- Sutter G, Moss B. Nonreplicating vaccinia vector efficiently expresses recombinant genes. Proc Natl Acad Sci U S A 1992; 89:10847 - 51; http://dx.doi.org/10.1073/pnas.89.22.10847; PMID: 1438287
- Pastoret PP, Vanderplasschen A. Poxviruses as vaccine vectors. Comp Immunol Microbiol Infect Dis 2003; 26:343 - 55; http://dx.doi.org/10.1016/S0147-9571(03)00019-5; PMID: 12818621
- Staib C, Drexler I, Sutter G. Construction and isolation of recombinant MVA. Methods Mol Biol 2004; 269:77 - 100; PMID: 15114009
- Albert ML, Sauter B, Bhardwaj N. Dendritic cells acquire antigen from apoptotic cells and induce class I-restricted CTLs. Nature 1998; 392:86 - 9; http://dx.doi.org/10.1038/32183; PMID: 9510252
- Kastenmuller W, Drexler I, Ludwig H, Erfle V, Peschel C, Bernhard H, et al. Infection of human dendritic cells with recombinant vaccinia virus MVA reveals general persistence of viral early transcription but distinct maturation-dependent cytopathogenicity. Virology 2006; 350:276 - 88; http://dx.doi.org/10.1016/j.virol.2006.02.039; PMID: 16595141
- Sánchez-Puig JM, Sánchez L, Roy G, Blasco R. Susceptibility of different leukocyte cell types to Vaccinia virus infection. Virol J 2004; 1:10; http://dx.doi.org/10.1186/1743-422X-1-10; PMID: 15555076
- Liu L, Chavan R, Feinberg MB. Dendritic cells are preferentially targeted among hematolymphocytes by Modified Vaccinia Virus Ankara and play a key role in the induction of virus-specific T cell responses in vivo. BMC Immunol 2008; 9:15; http://dx.doi.org/10.1186/1471-2172-9-15; PMID: 18412969
- Brandler S, Lepelley A, Desdouits M, Guivel-Benhassine F, Ceccaldi PE, Lévy Y, et al. Preclinical studies of a modified vaccinia virus Ankara-based HIV candidate vaccine: antigen presentation and antiviral effect. J Virol 2010; 84:5314 - 28; http://dx.doi.org/10.1128/JVI.02329-09; PMID: 20219934
- Guerra S, Nájera JL, González JM, López-Fernández LA, Climent N, Gatell JM, et al. Distinct gene expression profiling after infection of immature human monocyte-derived dendritic cells by the attenuated poxvirus vectors MVA and NYVAC. J Virol 2007; 81:8707 - 21; http://dx.doi.org/10.1128/JVI.00444-07; PMID: 17537851
- Gasteiger G, Kastenmuller W, Ljapoci R, Sutter G, Drexler I. Cross-priming of cytotoxic T cells dictates antigen requisites for modified vaccinia virus Ankara vector vaccines. J Virol 2007; 81:11925 - 36; http://dx.doi.org/10.1128/JVI.00903-07; PMID: 17699574
- Climent N, Guerra S, García F, Rovira C, Miralles L, Gómez CE, et al. Dendritic cells exposed to MVA-based HIV-1 vaccine induce highly functional HIV-1-specific CD8(+) T cell responses in HIV-1-infected individuals. PLoS One 2011; 6:e19644; http://dx.doi.org/10.1371/journal.pone.0019644; PMID: 21625608
- Flechsig C, Suezer Y, Kapp M, Tan SM, Löffler J, Sutter G, et al. Uptake of antigens from modified vaccinia Ankara virus-infected leukocytes enhances the immunostimulatory capacity of dendritic cells. Cytotherapy 2011; 13:739 - 52; http://dx.doi.org/10.3109/14653249.2010.549123; PMID: 21250864
- Lehmann MH, Kastenmuller W, Kandemir JD, Brandt F, Suezer Y, Sutter G. Modified vaccinia virus ankara triggers chemotaxis of monocytes and early respiratory immigration of leukocytes by induction of CCL2 expression. J Virol 2009; 83:2540 - 52; http://dx.doi.org/10.1128/JVI.01884-08; PMID: 19129447
- Greiner S, Humrich JY, Thuman P, Sauter B, Schuler G, Jenne L. The highly attenuated vaccinia virus strain modified virus Ankara induces apoptosis in melanoma cells and allows bystander dendritic cells to generate a potent anti-tumoral immunity. Clin Exp Immunol 2006; 146:344 - 53; http://dx.doi.org/10.1111/j.1365-2249.2006.03177.x; PMID: 17034588
- Pascutti MF, Rodríguez AM, Falivene J, Giavedoni L, Drexler I, Gherardi MM. Interplay between modified vaccinia virus Ankara and dendritic cells: phenotypic and functional maturation of bystander dendritic cells. J Virol 2011; 85:5532 - 45; http://dx.doi.org/10.1128/JVI.02267-10; PMID: 21411535
- Barbalat R, Lau L, Locksley RM, Barton GM. Toll-like receptor 2 on inflammatory monocytes induces type I interferon in response to viral but not bacterial ligands. Nat Immunol 2009; 10:1200 - 7; http://dx.doi.org/10.1038/ni.1792; PMID: 19801985
- Zhu J, Martinez J, Huang X, Yang Y. Innate immunity against vaccinia virus is mediated by TLR2 and requires TLR-independent production of IFN-beta. Blood 2007; 109:619 - 25; http://dx.doi.org/10.1182/blood-2006-06-027136; PMID: 16973959
- Delaloye J, Roger T, Steiner-Tardivel QG, Le Roy D, Knaup Reymond M, Akira S, et al. Innate immune sensing of modified vaccinia virus Ankara (MVA) is mediated by TLR2-TLR6, MDA-5 and the NALP3 inflammasome. PLoS Pathog 2009; 5:e1000480; http://dx.doi.org/10.1371/journal.ppat.1000480; PMID: 19543380
- Waibler Z, Anzaghe M, Ludwig H, Akira S, Weiss S, Sutter G, et al. Modified vaccinia virus Ankara induces Toll-like receptor-independent type I interferon responses. J Virol 2007; 81:12102 - 10; http://dx.doi.org/10.1128/JVI.01190-07; PMID: 17855554
- Samuelsson C, Hausmann J, Lauterbach H, Schmidt M, Akira S, Wagner H, et al. Survival of lethal poxvirus infection in mice depends on TLR9, and therapeutic vaccination provides protection. J Clin Invest 2008; 118:1776 - 84; http://dx.doi.org/10.1172/JCI33940; PMID: 18398511
- Myskiw C, Arsenio J, Booy EP, Hammett C, Deschambault Y, Gibson SB, et al. RNA species generated in vaccinia virus infected cells activate cell type-specific MDA5 or RIG-I dependent interferon gene transcription and PKR dependent apoptosis. Virology 2011; 413:183 - 93; http://dx.doi.org/10.1016/j.virol.2011.01.034; PMID: 21354589
- Blanchard TJ, Alcami A, Andrea P, Smith GL. Modified vaccinia virus Ankara undergoes limited replication in human cells and lacks several immunomodulatory proteins: implications for use as a human vaccine. J Gen Virol 1998; 79:1159 - 67; PMID: 9603331
- Hornung V, Ablasser A, Charrel-Dennis M, Bauernfeind F, Horvath G, Caffrey DR, et al. AIM2 recognizes cytosolic dsDNA and forms a caspase-1-activating inflammasome with ASC. Nature 2009; 458:514 - 8; http://dx.doi.org/10.1038/nature07725; PMID: 19158675
- Wenzel M, Wunderlich M, Besch R, Poeck H, Willms S, Schwantes A, et al. Cytosolic DNA triggers mitochondrial apoptosis via DNA damage signaling proteins independently of AIM2 and RNA polymerase III. J Immunol 2012; 188:394 - 403; http://dx.doi.org/10.4049/jimmunol.1100523; PMID: 22140256
- Verheust C, Goossens M, Pauwels K, Breyer D. Biosafety aspects of modified vaccinia virus Ankara (MVA)-based vectors used for gene therapy or vaccination. Vaccine 2012; 30:2623 - 32; http://dx.doi.org/10.1016/j.vaccine.2012.02.016; PMID: 22342706
- Esteban M.. Attenuated poxvirus vectors MVA and NYVAC as promising vaccine candidates against HIV/AIDS. 2009; 5:867 - 71
- Cosma A, Nagaraj R, Bühler S, Hinkula J, Busch DH, Sutter G, et al. Therapeutic vaccination with MVA-HIV-1 nef elicits Nef-specific T-helper cell responses in chronically HIV-1 infected individuals. Vaccine 2003; 22:21 - 9; http://dx.doi.org/10.1016/S0264-410X(03)00538-3; PMID: 14604567
- Harrer E, Bäuerle M, Ferstl B, Chaplin P, Petzold B, Mateo L, et al. Therapeutic vaccination of HIV-1-infected patients on HAART with a recombinant HIV-1 nef-expressing MVA: safety, immunogenicity and influence on viral load during treatment interruption. Antivir Ther 2005; 10:285 - 300; PMID: 15865223
- Smith JS, Lindsay L, Hoots B, Keys J, Franceschi S, Winer R, et al. Human papillomavirus type distribution in invasive cervical cancer and high-grade cervical lesions: a meta-analysis update. Int J Cancer 2007; 121:621 - 32; http://dx.doi.org/10.1002/ijc.22527; PMID: 17405118
- http://globocan.iarc.fr/.
- Cid-Arregui A. Prophylactic HPV vaccines. Open Vaccine J 2012; In press
- Dowhanick JJ, McBride AA, Howley PM. Suppression of cellular proliferation by the papillomavirus E2 protein. J Virol 1995; 69:7791 - 9; PMID: 7494290
- Corona Gutierrez CM, Tinoco A, Navarro T, Contreras ML, Cortes RR, Calzado P, et al. Therapeutic vaccination with MVA E2 can eliminate precancerous lesions (CIN 1, CIN 2, and CIN 3) associated with infection by oncogenic human papillomavirus. Hum Gene Ther 2004; 15:421 - 31; http://dx.doi.org/10.1089/10430340460745757; PMID: 15144573
- García-Hernández E, González-Sánchez JL, Andrade-Manzano A, Contreras ML, Padilla S, Guzmán CC, et al. Regression of papilloma high-grade lesions (CIN 2 and CIN 3) is stimulated by therapeutic vaccination with MVA E2 recombinant vaccine. Cancer Gene Ther 2006; 13:592 - 7; http://dx.doi.org/10.1038/sj.cgt.7700937; PMID: 16456551
- Albarran Y, Carvajal A, de la Garza A, Cruz Quiroz BJ, Vazquez Zea E, Díaz Estrada I, Mendez Fuentez E, et al. MVA E2 recombinant vaccine in the treatment of human papillomavirus infection in men presenting intraurethral flat condyloma: a phase I/II study. BioDrugs 2007; 21:47 - 59; http://dx.doi.org/10.2165/00063030-200721010-00006; PMID: 17263589
- Brun JL, Dalstein V, Leveque J, Mathevet P, Raulic P, Baldauf JJ, et al. Regression of high-grade cervical intraepithelial neoplasia with TG4001 targeted immunotherapy. Am J Obstet Gynecol 2011; 204:169 - , e1-8; http://dx.doi.org/10.1016/j.ajog.2010.09.020; PMID: 21284968
- Psyrri A, Boutati E, Karageorgopoulou S. Human papillomavirus in head and neck cancers: biology, prognosis, hope of treatment, and vaccines. Anticancer Drugs 2011; 22:586 - 90; http://dx.doi.org/10.1097/CAD.0b013e328344ec44; PMID: 21403517
- Shepard CW, Finelli L, Alter MJ. Global epidemiology of hepatitis C virus infection. Lancet Infect Dis 2005; 5:558 - 67; http://dx.doi.org/10.1016/S1473-3099(05)70216-4; PMID: 16122679
- Poordad F, McCone J Jr., Bacon BR, Bruno S, Manns MP, Sulkowski MS, et al, SPRINT-2 Investigators. Boceprevir for untreated chronic HCV genotype 1 infection. N Engl J Med 2011; 364:1195 - 206; http://dx.doi.org/10.1056/NEJMoa1010494; PMID: 21449783
- Jacobson IM, McHutchison JG, Dusheiko G, Di Bisceglie AM, Reddy KR, Bzowej NH, et al, ADVANCE Study Team. Telaprevir for previously untreated chronic hepatitis C virus infection. N Engl J Med 2011; 364:2405 - 16; http://dx.doi.org/10.1056/NEJMoa1012912; PMID: 21696307
- Welsch C, Jesudian A, Zeuzem S, Jacobson I. New direct-acting antiviral agents for the treatment of hepatitis C virus infection and perspectives. Gut 2012; 61:Suppl 1 i36 - 46; http://dx.doi.org/10.1136/gutjnl-2012-302144; PMID: 22504918
- Thimme R, Oldach D, Chang KM, Steiger C, Ray SC, Chisari FV. Determinants of viral clearance and persistence during acute hepatitis C virus infection. J Exp Med 2001; 194:1395 - 406; http://dx.doi.org/10.1084/jem.194.10.1395; PMID: 11714747
- Wertheimer AM, Miner C, Lewinsohn DM, Sasaki AW, Kaufman E, Rosen HR. Novel CD4+ and CD8+ T-cell determinants within the NS3 protein in subjects with spontaneously resolved HCV infection. Hepatology 2003; 37:577 - 89; http://dx.doi.org/10.1053/jhep.2003.50115; PMID: 12601356
- Fournillier A, Gerossier E, Evlashev A, Schmitt D, Simon B, Chatel L, et al. An accelerated vaccine schedule with a poly-antigenic hepatitis C virus MVA-based candidate vaccine induces potent, long lasting and in vivo cross-reactive T cell responses. Vaccine 2007; 25:7339 - 53; http://dx.doi.org/10.1016/j.vaccine.2007.08.020; PMID: 17875349
- Habersetzer F, Honnet G, Bain C, Maynard-Muet M, Leroy V, Zarski JP, et al. A poxvirus vaccine is safe, induces T-cell responses, and decreases viral load in patients with chronic hepatitis C. Gastroenterology 2011; 141:890 - 9 e1-4
- Wedemeyer H, Janczewska KE, Mazur W, Stanciu C, Habersetzer F, Carreno V. HCVac study: antiviral activity of TG4040 therapeutic vaccination in genotype- 1 chronic HCV patients. 62nd Ann Meeting Am Ass Study Liver Dis (AASLD) 2011; poster 1336.
- Cavenaugh JS, Awi D, Mendy M, Hill AV, Whittle H, McConkey SJ. Partially randomized, non-blinded trial of DNA and MVA therapeutic vaccines based on hepatitis B virus surface protein for chronic HBV infection. PLoS One 2011; 6:e14626; http://dx.doi.org/10.1371/journal.pone.0014626; PMID: 21347224
- Goepfert PA, Elizaga ML, Sato A, Qin L, Cardinali M, Hay CM, et al, National Institute of Allergy and Infectious Diseases HIV Vaccine Trials Network. Phase 1 safety and immunogenicity testing of DNA and recombinant modified vaccinia Ankara vaccines expressing HIV-1 virus-like particles. J Infect Dis 2011; 203:610 - 9; http://dx.doi.org/10.1093/infdis/jiq105; PMID: 21282192
- Greenough TC, Cunningham CK, Muresan P, McManus M, Persaud D, Fenton T, et al, Pediatric AIDS Clinical Trials Group P1059 Team. Safety and immunogenicity of recombinant poxvirus HIV-1 vaccines in young adults on highly active antiretroviral therapy. Vaccine 2008; 26:6883 - 93; http://dx.doi.org/10.1016/j.vaccine.2008.09.084; PMID: 18940219
- Glaziou P, Floyd K, Raviglione M. Global burden and epidemiology of tuberculosis. [vii.] Clin Chest Med 2009; 30:621 - 36, vii; http://dx.doi.org/10.1016/j.ccm.2009.08.017; PMID: 19925958
- Rook GA, Lowrie DB, Hernàndez-Pando R. Immunotherapeutics for tuberculosis in experimental animals: is there a common pathway activated by effective protocols?. J Infect Dis 2007; 196:191 - 8; http://dx.doi.org/10.1086/518937; PMID: 17570105
- Lowrie DB, Tascon RE, Bonato VL, Lima VM, Faccioli LH, Stavropoulos E, et al. Therapy of tuberculosis in mice by DNA vaccination. Nature 1999; 400:269 - 71; http://dx.doi.org/10.1038/22326; PMID: 10421369
- Cardona PJ, Amat I, Gordillo S, Arcos V, Guirado E, Díaz J, et al. Immunotherapy with fragmented Mycobacterium tuberculosis cells increases the effectiveness of chemotherapy against a chronical infection in a murine model of tuberculosis. Vaccine 2005; 23:1393 - 8; http://dx.doi.org/10.1016/j.vaccine.2004.09.008; PMID: 15661388
- Mwinga A, Nunn A, Ngwira B, Chintu C, Warndorff D, Fine P, et al, LUSKAR collaboration. Mycobacterium vaccae (SRL172) immunotherapy as an adjunct to standard antituberculosis treatment in HIV-infected adults with pulmonary tuberculosis: a randomised placebo-controlled trial. Lancet 2002; 360:1050 - 5; http://dx.doi.org/10.1016/S0140-6736(02)11141-X; PMID: 12383985
- Verreck FA, Vervenne RA, Kondova I, van Kralingen KW, Remarque EJ, Braskamp G, et al. MVA.85A boosting of BCG and an attenuated, phoP deficient M. tuberculosis vaccine both show protective efficacy against tuberculosis in rhesus macaques. PLoS One 2009; 4:e5264; http://dx.doi.org/10.1371/journal.pone.0005264; PMID: 19367339
- McShane H, Pathan AA, Sander CR, Keating SM, Gilbert SC, Huygen K, et al. Recombinant modified vaccinia virus Ankara expressing antigen 85A boosts BCG-primed and naturally acquired antimycobacterial immunity in humans. Nat Med 2004; 10:1240 - 4; http://dx.doi.org/10.1038/nm1128; PMID: 15502839
- McShane H. Tuberculosis vaccines: beyond bacille Calmette-Guerin. Philos Trans R Soc Lond B Biol Sci 2011; 366:2782 - 9; http://dx.doi.org/10.1098/rstb.2011.0097; PMID: 21893541
- Sander CR, Pathan AA, Beveridge NE, Poulton I, Minassian A, Alder N, et al. Safety and immunogenicity of a new tuberculosis vaccine, MVA85A, in Mycobacterium tuberculosis-infected individuals. Am J Respir Crit Care Med 2009; 179:724 - 33; http://dx.doi.org/10.1164/rccm.200809-1486OC; PMID: 19151191
- Hawkridge T, Scriba TJ, Gelderbloem S, Smit E, Tameris M, Moyo S, et al. Safety and immunogenicity of a new tuberculosis vaccine, MVA85A, in healthy adults in South Africa. J Infect Dis 2008; 198:544 - 52; http://dx.doi.org/10.1086/590185; PMID: 18582195
- Scriba TJ, Tameris M, Mansoor N, Smit E, van der Merwe L, Isaacs F, et al. Modified vaccinia Ankara-expressing Ag85A, a novel tuberculosis vaccine, is safe in adolescents and children, and induces polyfunctional CD4+ T cells. Eur J Immunol 2010; 40:279 - 90; http://dx.doi.org/10.1002/eji.200939754; PMID: 20017188
- Ota MO, Odutola AA, Owiafe PK, Donkor S, Owolabi OA, Brittain NJ, et al. Immunogenicity of the tuberculosis vaccine MVA85A is reduced by coadministration with EPI vaccines in a randomized controlled trial in Gambian infants. Sci Transl Med 2011; 3:88ra56; http://dx.doi.org/10.1126/scitranslmed.3002461; PMID: 21697532
- Oxman MN, Levin MJ, Johnson GR, Schmader KE, Straus SE, Gelb LD, et al, Shingles Prevention Study Group. A vaccine to prevent herpes zoster and postherpetic neuralgia in older adults. N Engl J Med 2005; 352:2271 - 84; http://dx.doi.org/10.1056/NEJMoa051016; PMID: 15930418