Abstract
We evaluated the immunogenicity and protective efficacy of DNA vaccines expressing the codon-optimized envelope glycoprotein genes of Zaire ebolavirus, Sudan ebolavirus, and Marburg marburgvirus (Musoke and Ravn). Intramuscular or intradermal delivery of the vaccines in BALB/c mice was performed using the TriGrid™ electroporation device. Mice that received DNA vaccines against the individual viruses developed robust glycoprotein-specific antibody titers as determined by ELISA and survived lethal viral challenge with no display of clinical signs of infection. Survival curve analysis revealed there was a statistically significant increase in survival compared to the control groups for both the Ebola and Ravn virus challenges. These data suggest that further analysis of the immune responses generated in the mice and additional protection studies in nonhuman primates are warranted.
Introduction
Filoviruses are non-segmented, negative-sense, enveloped RNA viruses classified in the family Filoviridae, which contains two defined genera; Marburgvirus and Ebolavirus. In a new taxonomic classification scheme, three serologically distinct genera have been identified in the Filoviridae family; Marburgvirus, Ebolavirus, and Cuevavirus.Citation1 The Marburgvirus genus includes a single viral species, Marburg marburgvirus and two defined viruses; Marburg virus (MARV) and Ravn virus (RAVV). The Ebolavirus genus includes five distinct viruses: Ebolavirus (EBOV, formerly ZEBOV or Zaire virus); Sudan virus (SUDV); Bundibugyo virus (BDBV); Tai Forest virus (TAFV, formerly Cote d’Ivoire virus); and Reston virus (RESTV). All but RESTV are pathogenic for humans. Currently, there are no licensed filovirus vaccines or therapeutics for human use, although numerous approaches, including DNA vaccines, are being investigated. In previous studies, DNA vaccines containing the glycoprotein gene (GP) of either MARV or RAVV provided complete protection from homologous virus challenge to guinea pigs and the MARV GP vaccine protected four of six vaccinated nonhuman primates (NHP) from lethal challenge.Citation2 These results suggested that if it was possible to improve either the delivery method and/or the vaccine constructs, the DNA vaccine platform might be an effective means of protecting against filovirus infections.
To advance our DNA vaccine constructs, we optimized the genes to reflect codons most commonly used by Homo sapiens and to remove elements that are known to compromise expression. This approach has been shown many times by us and others to greatly improve expression and in turn, immunogenicity of DNA vaccines.Citation3-Citation8 To improve delivery, we switched from gene gun to intramuscular electroporation, which has been shown to be a very effective means of introducing DNA vaccines to small and large animals.Citation4,Citation5 Electroporation also allows for the delivery of larger quantities of DNA in a single dosing than did gene gun, which is especially important for multiagent vaccines, as will be required for protection against the antigenically diverse marburgviruses and ebolaviruses that can cause disease in humans. Here, we report the results of studies in mice in which we evaluate the immunogenicity of individual and multiagent codon-optimized filovirus GP DNA vaccines, delivered by the Ichor Medical System TriGrid™ (TDS) intramuscular electroporation (IM) and intradermal (ID) devices.
DNA Vaccines
DNA vaccines expressing the codon-optimized GP genes of EBOV (EBOVCO), SUDV (SUDVCO), MARV (MARVCO) and RAVV (RAVVCO) were synthesized by Geneart and cloned into the pWRG7077 eukaryotic expression vector downstream of the cytomegalovirus immediate-early promoter. Research grade plasmids were manufactured by Aldevron.
Immunogenicity study design
Female BALB/c mice (6 to 8 weeks old) were vaccinated three times at 3-week intervals, with 20 µg/20µl, 5 µg/20 µl and 1 µg/20 µl of DNA diluted in calcium-and magnesium-free phosphate buffered saline (Life Technologies). Groups of 10 mice were either vaccinated IM or ID using the TDS as described previously.Citation4,Citation9 Briefly, mice were anesthetized by IM injection of a diluted acepromazine-ketamine-xylanzine mixture in one tibialis anterior muscle with 20 µl of DNA solution using a 3/10 cm3 U-100 insulin syringe (Becton Dickinson) inserted into the center of a TriGrid electrode array with 2.5 mm electrode spacing. Injection of DNA was followed immediately by electrical stimulation at an amplitude of 250 V/cm, and the total duration was 40 ms over a 400 ms interval. Blood was collected from mice at days 0, 14, 21, 37, 42 and 56 by retro-orbital blood collection under anesthesia.
Immunological assays
Total IgG anti-EBOV, anti-SUDV, anti-MARV and anti-RAVV endpoint antibody titers were determined for isolated serum samples by standard enzyme-linked immunosorbent assay (ELISA) using sucrose-purified, gamma-irradiated, whole-virion EBOV, SUDV, MARV and RAVV antigen. Each test serum was serially diluted twofold starting at 1:100 and incubated with 250 ng to 500 ng of antigen per well in 96-well plates. A heavy chain-specific goat anti-mouse secondary antibody conjugated to horseradish peroxidase (HRP) (Sigma-Aldrich) and TMB 2 Peroxidase substrate (KPL) were used in conjunction with a SpectraMax M2e microplate reader (Molecular Devices) at an optical density of 450 nm to calculate endpoint titers with Softmax Pro v5 software (Molecular Devices).
Challenge study design
Additional groups of female BALB/c mice (6 to 8 weeks) were vaccinated with 5 µg/20 µl of the individual DNA vaccines or with a mixture of all four DNA vaccines (20 µg/20 µl total DNA) by IM delivery as described above. Mice received two or three vaccinations 3 weeks apart. Blood was collected from each mouse by retro-orbital–blood collection under anesthesia before dosing on all vaccination days and 3 weeks following last vaccination (Days 0, 21, 42, 63). All sera wasscreened by ELISA as described above.
Mouse-adapted filoviruses
Mouse-adapted EBOV (maEBOV) and RAVV (maRAVV) were prepared as previously described.Citation10,Citation11 Mice underwent intraperitoneal (IP) challenge with 1000 PFU of maEBOV or maRAVV (~1000 LD50) 4 weeks after the last vaccination.
Statistical analysis
Kaplan Meier survival curve analysis using a log rank test was performed to determine p value significance of vaccinated groups surviving lethal challenge compared to the control group using GraphPad Prism v5.03 for Windows (GraphPad Software). Further, log10 transformations were applied to inactivated, whole-virus, end-point ELISA titers using Softmax Pro v5.4.1 (Molecular Devices, LLC).
Results
Flow cytometry analysis revealed that EBOVCO produced more GP protein than the non-optimized plasmid (). For MARVCO, there was no significant quantitative difference in protein expression as compared to the non-codon optimized plasmid using this detection method (data not shown). In the immunogenicity study, total IgG antibodies, as detected by ELISA, were highest in the groups of mice vaccinated by the IM route after the third dose (day 56) for EBOVCO (). For RAVVCO, the mean log10 titers peaked 3 weeks after the second vaccination (day 42) by the IM route (). Further, for RAVVCO the mean log10 titers were almost identical on day 42 between the three groups indicating that vaccination with 1 µg of DNA was just as efficient as the 20 µg dose. For EBOVCO, there was no statistically significant difference among titers for the 20 µg, 5 µg and 1 µg dose groups at Day 56.
Figure 1. Standard curve of EBOV GP expression in COS cells as determined in the flow cytometry assay. Cells were prepared, washed, stained, fixed, and analyzed as described previously.Citation12 Specifically, a EBOV-GP1 specific monoclonal antibody (6E3) was diluted to 1:100 and used as the primary antibody.Citation13 The secondary antibody was a AlexaFluor488-labeled goat anti-mouse antibody (Invitrogen) diluted to 1:400. EBOVCO had higher overall GP expression than the non-optimized construct (EBOVWT).
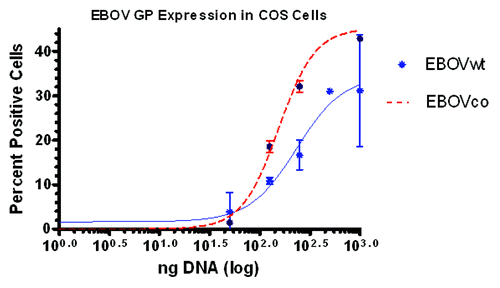
Figure 2. Antibody responses of vaccinated mice measured by ELISA. Female BALB/c mice (n = 10 per group) were vaccinated three times at a 3-week interval with either 20 µg, 5 µg or 1 µg of EBOVCO (A) or RAVVCO (B) using IM or ID electroporation delivery. Serum samples were collected on days 0, 14, 21, 37, 42, 56 and screened for total IgG on the specified blood collection days and the log10 mean titers were determined as indicated by the bar graph. The standard error of the mean (SEM) is also shown.
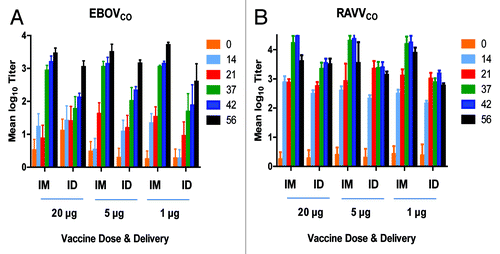
In a separate study, we tested the ability of the vaccines to protect mice from homologous challenge or cross-challenge after two or three vaccinations with the individual plasmids or a mixture of all four plasmids. Mice receiving two or three vaccinations with the individual EBOV plasmid or the combination vaccine were completely protected from maEBOV challenge (). As expected, the majority of mice receiving the vaccine against the serologically distinct SUDV or with control plasmid did not survive challenge with maEBOV (). Mice receiving the vaccines against either MARVCO, RAVVCO or with the combination vaccines were protected from challenge with maRAVV and showed no visible signs of disease at any time after the challenge. Although only half of the controls succumbed or were humanely euthanized, all of them showed visible signs of disease, which included ruffled fur, lethargy, and dehydration (). Kaplan Meier survival curve analysis, using a log rank test, revealed statistically significant survival in both the EBOV (P < 0.0001) and MARV (P < 0.0018) challenge groups for vaccinated versus control mice even though 100% mortality was not achieved in the control groups.
Figure 3. Survival of vaccinated mice after challenge with ma EBOV. Female BALB/c (n = 10 per group) were vaccinated at 3-week intervals with either two (2×) or three (3×) administrations of 5 µg of pWRG7077 (empty vector), EBOVCO DNA, SUDVCO DNA or a combination of all four DNA vaccines. Four weeks after the final dose, animals were challenged with 103 PFU of maEBOV by the IP route. Kaplan-Meier survival analysis is shown indicating the percentage of surviving mice for 28 days postchallenge.
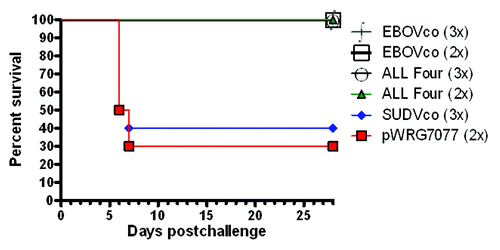
Figure 4. Survival of vaccinated mice after challenge with ma RAVV. Female BALB/c (n = 10 per group) were vaccinated twice at a 3-week interval with 5 µg of pWRG7077 (empty vector), RAVVCO DNA, MARVCO DNA or a combination of all four DNA vaccines. Four weeks after the final dose, animals were challenged with 103 PFU of maRAVV by the IP route. Kaplan-Meier survival analysis is shown indicating the percentage of surviving mice for 28 days postchallenge.
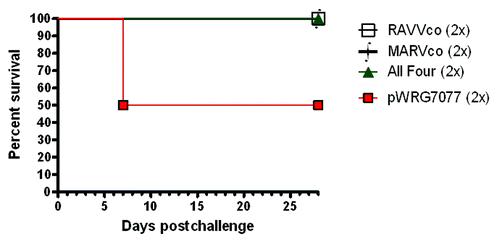
Discussion
In this study, we evaluated the ability of codon-optimized DNA vaccines against the GP of EBOV, SUDV, MARV or RAVV delivered by electroporation, individually or as a mixture, to protect mice from lethal filovirus challenge. Further, we also tested two different routes of vaccine delivery (IM vs. ID) and various DNA doses to optimize protection and elicit the most robust antibody responses. The results from our study indicate that both EP methods elicited strong GP-specific antibody responses with low DNA doses and few vaccinations. Further, Kaplan Meier survival curve analysis revealed that the protective efficacy of the filovirus DNA vaccines in mice was statistically significant for both the maEBOV and maRAVV challenges. These findings indicate IM EP delivery of individual and combined codon-optimized filovirus DNA vaccines represents a promising strategy for eliciting protection against filovirus challenge. Further studies to assess the cell mediated immune responses, which are also known to be important for protection from filoviruses, and studies to further evaluate the vaccines in NHP are planned.
Acknowledgments
The authors thank Joshua Moore for performing the sample collection and blinded animal health assessments, Michelle Richards for technical help with vaccinations and Angela Silva our grants and contracts specialist. This study was supported by funding from NIAID Grant 1U01AI082069-01.
Disclaimer
Research was conducted under an IACUC approved protocol in compliance with the Animal Welfare Act and other Federal statutes and regulations relating to animals and experiments involving animals and adheres to principles stated in the “Guide for the Care and Use of Laboratory Animals,” National Research Council, 1996. The facility where this research was conducted is fully accredited by the Association for Assessment and Accreditation of Laboratory Animal Care International.
Note
Opinions, interpretations, conclusions, and recommendations are those of the author and are not necessarily endorsed by the US Army.
References
- Kuhn JH, Becker S, Ebihara H, Geisbert TW, Johnson KM, Kawaoka Y, et al. Proposal for a revised taxonomy of the family Filoviridae: classification, names of taxa and viruses, and virus abbreviations. Arch Virol 2010; 155:2083 - 103; http://dx.doi.org/10.1007/s00705-010-0814-x; PMID: 21046175
- Riemenschneider J, Garrison A, Geisbert J, Jahrling P, Hevey M, Negley D, et al. Comparison of individual and combination DNA vaccines for B. anthracis, Ebola virus, Marburg virus and Venezuelan equine encephalitis virus. Vaccine 2003; 21:4071 - 80; http://dx.doi.org/10.1016/S0264-410X(03)00362-1; PMID: 12922144
- Cid-Arregui A, Juárez V, zur Hausen H. A synthetic E7 gene of human papillomavirus type 16 that yields enhanced expression of the protein in mammalian cells and is useful for DNA immunization studies. J Virol 2003; 77:4928 - 37; http://dx.doi.org/10.1128/JVI.77.8.4928-4937.2003; PMID: 12663798
- Dupuy LC, Richards MJ, Ellefsen B, Chau L, Luxembourg A, Hannaman D, et al. A DNA vaccine for venezuelan equine encephalitis virus delivered by intramuscular electroporation elicits high levels of neutralizing antibodies in multiple animal models and provides protective immunity to mice and nonhuman primates. Clin Vaccine Immunol 2011; 18:707 - 16; http://dx.doi.org/10.1128/CVI.00030-11; PMID: 21450977
- Kutzler MA, Weiner DB. DNA vaccines: ready for prime time?. Nat Rev Genet 2008; 9:776 - 88; http://dx.doi.org/10.1038/nrg2432; PMID: 18781156
- Ternette N, Tippler B, Uberla K, Grunwald T. Immunogenicity and efficacy of codon optimized DNA vaccines encoding the F-protein of respiratory syncytial virus. Vaccine 2007; 25:7271 - 9; http://dx.doi.org/10.1016/j.vaccine.2007.07.025; PMID: 17825960
- Deml L, Bojak A, Steck S, Graf M, Wild J, Schirmbeck R, et al. Multiple effects of codon usage optimization on expression and immunogenicity of DNA candidate vaccines encoding the human immunodeficiency virus type 1 Gag protein. J Virol 2001; 75:10991 - 1001; http://dx.doi.org/10.1128/JVI.75.22.10991-11001.2001; PMID: 11602739
- Geisbert TW, Bailey M, Geisbert JB, Asiedu C, Roederer M, Grazia-Pau M, et al. Vector choice determines immunogenicity and potency of genetic vaccines against Angola Marburg virus in nonhuman primates. J Virol 2010; 84:10386 - 94; http://dx.doi.org/10.1128/JVI.00594-10; PMID: 20660192
- Luxembourg A, Hannaman D, Wills K, Bernard R, Tennant BC, Menne S, et al. Immunogenicity in mice and rabbits of DNA vaccines expressing woodchuck hepatitis virus antigens. Vaccine 2008; 26:4025 - 33; http://dx.doi.org/10.1016/j.vaccine.2008.05.021; PMID: 18556096
- Bray M, Davis K, Geisbert T, Schmaljohn C, Huggins J. A mouse model for evaluation of prophylaxis and therapy of Ebola hemorrhagic fever. J Infect Dis 1998; 178:651 - 61; http://dx.doi.org/10.1086/515386; PMID: 9728532
- Warfield KL, Bradfute SB, Wells J, Lofts L, Cooper MT, Alves DA, et al. Development and characterization of a mouse model for Marburg hemorrhagic fever. J Virol 2009; 83:6404 - 15; http://dx.doi.org/10.1128/JVI.00126-09; PMID: 19369350
- Badger CV, Richardson JD, Dasilva RL, Richards MJ, Josleyn MD, Dupuy LC, et al. Development and application of a flow cytometric potency assay for DNA vaccines. Vaccine 2011; 29:6728 - 35; http://dx.doi.org/10.1016/j.vaccine.2010.12.053; PMID: 21219978
- Wilson JA, Hevey M, Bakken R, Guest S, Bray M, Schmaljohn AL, et al. Epitopes involved in antibody-mediated protection from Ebola virus. Science 2000; 287:1664 - 6; http://dx.doi.org/10.1126/science.287.5458.1664; PMID: 10698744