Abstract
Pertussis toxin in its detoxified form is a major component of all current acellular pertussis vaccines. Here we report the membrane translocation and internalization activities of pertussis toxin and various pertussis toxoids using Chinese hamster ovary cells and confocal microscopy based on indirect immunofluorescence labeling. Chemically detoxified pertussis toxoids were able to translocate/internalize into cells at the concentration about 1,000 times higher than the native toxin. Pertussis toxoids detoxified with different procedures (glutaraldehyde, glutaraldehyde plus formaldehyde, hydrogen peroxide or genetic mutation) showed differences in fluorescence intensity under the same condition, indicating toxoids from different detoxification methods may have different translocation/internalization activities on cells.
Whooping cough caused by infection with Bordetella pertussis is a serious disease especially for infants and young children. Two types of pertussis vaccines, namely whole-cell (WCV) and acellular pertussis vaccines (ACV) are recommended by WHO for immunisation campaigns worldwide for the prevention of the pertussis disease.Citation1 Pertussis toxin in its detoxified form, is an essential protective antigen for both whole-cell and acellular pertussis vaccines.
Pertussis toxin (PTx) has the A-B type molecular structure typical of many other bacterial toxins, with an enzyme active A-protomer (monomer), the S-1 subunit, and a binding B-oligomer consisting of subunits S-2 through to S-5.Citation2-Citation5 Intact B-oligomer is required for binding of the holotoxin to receptor sites on the cell surface and enables entry of the A-protomer into the cells.Citation6-Citation8 The toxin A-protomer catalyzes the ADP-ribosylation of eukaryote GTP-binding regulatory proteins, prevents hormonal inhibition of adenylate cyclase and results in an increase in intracellular levels of cAMP.Citation9,Citation10 Therefore, PTx is capable of initiating two types of cellular response. One results from the lectin like action of the B-oligomer moiety, while the other reflects the ADP ribosylation, and hence the abolition of the signal transduction by guanine-nucleotide binding proteins (G-proteins).Citation6,Citation9
Since the native PTx is considered to be too toxic as a vaccine component, various methods have been widely used by vaccine manufacturers to detoxify the native toxin. These methods include genetic inactivation of the PTx A-protomer by double amino acids substitution (Arg9 → Lys and Glu129 → Gly) in the S-l subunit,Citation11-Citation13 and chemical treatments of the toxin with either formaldehyde, glutaraldehyde, tetranitromethane, hydrogen peroxide or with a combination of formaldehyde and glutaraldehyde.Citation14-Citation16 Different detoxification procedures using different reagents and conditions have been shown to result in different amino acid side-chain modifications and changes in conformational and epitope binding patterns for the resulting pertussis toxoids.Citation17-Citation23 The precise nature and location of the effects of different toxoiding reactions on the PTx molecule have not yet been defined. Thus, the detoxified pertussis toxin (PTd) presented in pertussis vaccines from different manufacturers could be modified at different sites of the A-subunit, B-oligomer or both.
Monitoring chemically detoxified PTds for residual toxicity and reversion to toxicity is an essential part of the safety evaluation of ACVs and is required by regulatory authorities. The in vivo histamine sensitization test (HIST) is the official pharmacopoeial safety test used for detecting residual PTx in such products.Citation24-Citation27 The HIST is a lethal test and the precise mechanism is unclear. It is difficult to standardise. Therefore, there is an urgent need for a replacement of the HIST. An in vitro assay system for measuring the B-oligomer carbohydrate binding activity in combination with the enzyme-HPLC coupled assay (E-HPLC), which measures the A-protomer activity has been developed as potential alternative to the in vivo HIST.Citation28-Citation35 However, this assay system does not address the toxin/toxoids membrane translocation/internalization activity. Furthermore, there is still a question of whether the translocation/internalization activity of the toxin are completely or partially destroyed by various chemical treatments. Therefore, further understanding and demonstration of the PTx and toxoid translocation into cells would lead to better development of in vitro alternative assays which should lead to improvement of safety test for ACVs. Here we report a study using confocal microscopy based on indirect immunofluorescence labeling method to investigate the translocation/internalization activity of PTx/PTd on the Chinese hamster ovary (CHO) cells. The PTx used in this study was a freeze-dried reference preparation [National Institute for Biological Standards and Control (NIBSC), UK, code 90/518]. Four different pertussis toxoids with different detoxification approaches were used in this study including a genetic mutant pertussis toxin (PTd-I) from Dr. R. Rappuoli and Dr. N.Hug, Chiron, Siena, Italy; PTd-II detoxified with formaldehyde and glutaraldehyde (a gift from GlaxoSmithKline Biologicals); PTd-III detoxified with Glutaraldehyde (a gift from Sanofi Pasteur); PTd-IV detoxified with hydrogen peroxide (a gift from SSI). All other chemicals and reagents used in the study were of analytical grade purchased from Life Technologies, Sigma-Aldrich or VWR-BD. Since PTd-III and PTd-IV were provided in aluminum hydroxide absorbed form, a desorption procedure was performed by treatment with a desorption buffer (6 mM EDTA disodium salt dissolved in 0.5 M di-sodium hydrogen orthophosphate) according to the methods described in reference Citation35. The antigen concentrations in these preparations after the desorption procedure were measured at 275 nm (Lambda 800 UV/Vis spectrometer, Perkin Elmer) and calculated against a bovine serum albumin standard. CHO cells K1 strain (ICN) were cultured at 37þC, 5% CO2 for 3 d in complete RPMI 1640 medium containing 10% fetal bovine serum (Life Technologies,) and 1% antibiotic solution (100 × solution containing 10,000 U penicillin, 10,000 μg streptomycin/ml). Coverslips (VWR) were placed in 24 wells plate, coated with 0.2% gelatine and washed with PBS twice in advance. The cultured cells were trypsinized and diluted to a concentration of 6 × 104 cells/ml with complete medium. Cell suspension at 1 ml/well was incubated at 37°C, 5% CO2 for 30–40 h. Subsequently, cells were treated with PTx/or PTd at indicated concentration in serum-free medium for 12 h. After the incubation, medium was removed and cells were washed in PBS (without Ca2+ and Mg2+) before being fixed with 4% paraformaldehyde (pH 7.4) for 20 min at room temperature. Cells were washed with PBS containing 0.15% glycine and permeabilized in 0.2% (v/v) TritonX-100 in PBS for 15 min at room temperature. Subsequently cells were blocked with 5% BSA in PBS (1 h) at room temperature and incubated with anti-PTx guinea pig serum (NIBSC, at 1:1,000) overnight at 4°C in a humid environment. The secondary antibody: Alexa Fluor 488 goat anti-guinea pig IgG (Life Technologies), was then added to the cells and incubated (1 h). After washing, cells were further incubated with Alexa Fluor 594 phalloidin (1:120) (Life Technologies) and 0.1 μg/ml DAPI (4’,6-diamidino-2-phenylindole, Life Technologies) to stain cellular F-actin and nucleus, respectively. Between each incubation step except after the blocking step, the cells were washed thoroughly with PBS. Coverslips were mounted onto microscope slides (VWR) using mounting medium (Dako) and sealed with clear nail polish. Fluorescence was visualized using a Leica SP2 confocal microscope (Leica Microsystems, GmbH). Appropriate fluorescence excitation and emission wavelengths were adopted and applied consistently for all samples according to the three fluorescent labels employed. Image stacks of each sample were collected for individual emission/detection channels and a composite image formed from data from multi-channels. Cells treated under identical condition, but without incubation with PTx or PTd were used as negative control in all experiments.
The progress of toxin translocation was investigated by treatment of CHO cells with PTx at 200 ng/ml for 2 h, 12 h or 48 h. After 2 h treatment, green fluorescence of the secondary antibody was located in the surrounding area and cytosol of cells (). At 12 h, fluorescence intensity was almost entirely located inside the cells within the cytosolic space (). It is interesting to note that by 48 h, the green fluorescence could no longer be observed which might indicate that at this time point, the toxin had been degraded in the cells (data not shown). The confocal microscopy results showed direct evidence that PTx was able to internalize into CHO cells. These results also indicated that the translocation/internalization of PTx already occurred at 2 h treatment time and most toxin molecules were internalized to the cytosol of cells by 12 h. According to this observation, 12 h of toxin incubation time was chosen for the subsequent experiments.
Figure 1. Confocal images of CHO cells treated with 200 ng/ml of PTx for different incubation periods. (A) negative control; (B) 2 h incubation; (C) 12 h incubation, to exhibit the effect of incubation time on toxin translocation. Green fluorescence represents PTx, blue fluorescence represents nucleus, and red fluorescence represents F-actin of cytoskeleton. For figures (B and C), the images are shown (for clarity) without blue fluorescence. Scale bar = 20 μm
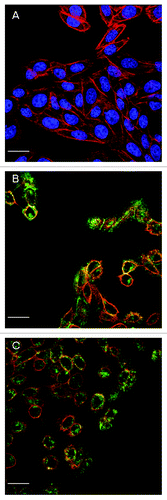
In order to investigate the effect of different concentrations of PTx on translocation, CHO cells were treated with PTx at 200 ng, 100 ng, 50 ng and 20 ng/ml for 12 h. Toxin dose dependent response was observed with increasing green fluorescence of the anti-PTx antibody from 50 ng to 200 ng/ml (−D). At 20 ng/ml toxin, the green fluorescence intensity was at the limits of detection (data not shown).
Figure 2.Confocal images of CHO cells treated with different PTx concentrations for 12 h. (A) Negative control; (B) PTx at 50 ng/ml; (C) PTx at 100 ng/ml; D: PTx at 200 ng/ml, to exhibit the effect of PTx concentration on toxin translocation [PTx at 20 ng/ml (data not shown)]. Green fluorescence represents PTx, blue fluorescence represents nucleus, and red fluorescence represents F-actin of cytoskeleton. For clarity, each plate is presented showing only blue/ green fluorescent channels with an insert showing red/ blue/ green fluorescence. Scale bar = 20 μm
![Figure 2.Confocal images of CHO cells treated with different PTx concentrations for 12 h. (A) Negative control; (B) PTx at 50 ng/ml; (C) PTx at 100 ng/ml; D: PTx at 200 ng/ml, to exhibit the effect of PTx concentration on toxin translocation [PTx at 20 ng/ml (data not shown)]. Green fluorescence represents PTx, blue fluorescence represents nucleus, and red fluorescence represents F-actin of cytoskeleton. For clarity, each plate is presented showing only blue/ green fluorescent channels with an insert showing red/ blue/ green fluorescence. Scale bar = 20 μm](/cms/asset/00096f8f-53df-4400-8244-ed404284fd35/khvi_a_10922795_f0002.gif)
To study the translocation/internalisation activity of various PTds, cells treated under identical condition without the antigen PTx/or PTd, were used as the negative control () and cells treated with PTx at 200 ng/ml were used as the positive control (). The genetically inactivated PTd (PTd-I) was able to translocate into the cells, but with less efficiency () in comparison to the PTx at the same concentration (). This indicated that although no modification in the B-oligomer of the PTx molecule by genetic inactivation of the S1 subunit was intended, formaldehyde treatment, even at a very low concentrations [~0.5%(w/v)], used to stabilize the antigen molecule, could have resulted in reduction in its translocation/internalization activity.Citation21 As predicted, much higher concentrations of chemically detoxified PTds (II to IV) was required to demonstrate translocation/internalization activity and green fluorescence were only observed in cells treated with PTds at 200 μg/ml (1,000 times of the PTx concentration). These treatments also showed lower green fluorescence intensity when compared with PTx treated cells. Each of the 3 toxoids at the concentrations tested were able to translocate into cells (-), but there were differences in observed fluorescent intensities between the three types of toxoids with different chemical detoxification methods. Comparison of the fluorescence intensity of the PTd detoxified by glutaraldehyde alone () and with that detoxified by glutaraldehyde plus formaldehyde (), the latter showed lower green fluorescence intensity in cells and this implied less translocation/internalization activity with this type of PTd.
Figure 3. Comparison of PTx and different PTds inactivated with different approaches in translocation to CHO cells shown by the fluorescence intensity of target protein in confocal images. CHO cells were treated with PTx or PTd for 12 h simultaneously. (A) Negative control; (B) Positive control, PTx (200 ng/ml); (C) PTd-I (200 ng/ml); (D) PTd-II (200 μg/ml); (E) PTd-III (200 μg/ml); (F) PTd-IV (200 μg/ml). Green fluorescence is of target protein, blue fluorescence is of nucleus, and red fluorescence representing F-actin of cytoskeleton. For clarity, each plate is presented showing only blue/ green fluorescent channels with an insert showing red/ blue/ green fluorescence. Scale bar = 20 μm.
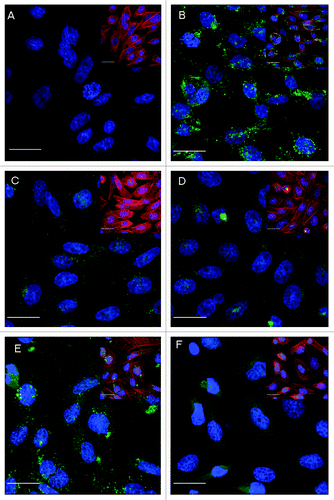
Residual PTx is considered as a major contributor to the reactogenicity of pertussis vaccines. To our knowledge, so far no confocal microscopic study on the internalization/translocation activities of PTds from different detoxification methods has been reported. Here we investigated the internalization properties of various PTds in CHO cells using confocal microscopy based on an indirect immunofluorescence labeling. We demonstrated that chemically detoxified PTds, like the native toxin, were able to translocate/internalize into cells. However, the concentration of PTd required to show the internalization effect was more than 1,000 times higher than PTx. This indicates that the various chemical detoxification methods are able to destroy most of, but not completely, the translocation/internalization domains/sites of the toxin molecular. This is supported by previous finding that a PTd showed approximately 850 times lower carbohydrate binding activity than PTx.Citation34 Although the chemical detoxification procedures used for PTx in most acellular pertussis vaccines could modify both the B- and the A-subunits (direct evidence of changes in the A-subunit structure remains elusive), the resulted PTds still retain some residual functional ADP ribosyltransferase and carbohydrate binding activities.Citation35 Since the clinical safe level of PTx has not been defined, it is important to continue to control the detoxification process and monitor its residual toxicity. For the genetically detoxified PTd (g-PTd), due to no modification in the B-oligomer, the translocation/internalization activity in this type of PTd is much higher than the chemically detoxified PTds. However, although it is able to translocate into target cells at low concentration as native toxin, since the enzymatic activity is destroyed by mutation, it should not exhibit any toxic activity. Furthermore, since this type of PTd retains most of the PTx molecular integrity and its ability to bind to receptors and to translocate into cells, this may explain the observation that although the g-PTd vaccine contains lower concentration of the antigen, it still shows higher immune responses compared with vaccines containing higher concentration of chemically detoxified PTds in human.Citation36
The precise nature and location of the effects of different toxoiding reactions on the PTx molecule have not been defined and detoxification procedures with different chemicals could result in different amino acid side-chain modifications and changes in conformational and epitope binding patterns for the resulting PTds.Citation17-Citation23 Although the guinea pig polyclonal antibody used for detecting target molecules translocated inside the cells in the present study was shown to be able to bind to PTx and all different types of toxoids in previous in- house studies (data not shown), its specific avidity to each of the toxoids has not been studied and this may deserve further investigation. Nevertheless, in the present study, the three chemically detoxified PTds showed differences in their translocation/internalization activities. Since different types of products are usually manufactured by a variety of detoxification conditions which resulted in varying degrees of detoxification and differences in bioactivities between products, our results supported the conclusion that the levels of residual PTx enzymatic activity, the binding activity and the translocation/or internalisation activities of these vaccine types are product specific.Citation35
PTx in its detoxified form is the most important component in ACVs. The monitoring of the level of residual PTx activity in ACVs is required by regulatory authorities.Citation24-Citation27 The molecular action of PTx involves binding of the toxin to its receptors via the B-oligomer, membrane translocation of the S1-subunit and intracellular expression of the ADP-ribosyltransferase activity catalyzed by the S1-subunit.Citation2-Citation5 The results from this study are consistent with our previous finding of the carbohydrate binding activities of PTx and various acellular pertussis vaccine products where PTd was found to have much lower carbohydrate binding activity than PTxCitation34 and different carbohydrate binding activities were found for various acellular pertussis vaccine products, e.g., a product made from PTd detoxified by glutaraldehyde plus formaldehyde (PTd-II) showed much lower carbohydrate binding activity than a product made from PTd detoxified by glutaraldehyde alone (PTd-III).Citation35,Citation37 Taken together with the confocal microscopic evidence and previous results on binding activities, indicate that a PTd with higher carbohydrate binding activityCitation35 could result in higher translocation/internalization activity in cells. The carbohydrate binding as well as membrane translocation and enzymatic activities, together play key role for PTx toxicity.
In conclusion, a confocal microscopic method based on indirect immunofluorescence has been developed to assess the translocation/internalization activities of PTx and various PTds on CHO-cells. PTds with different detoxification procedures showed great reduction in their translocation/internalization activities compared with the native toxin. However, all PTds at higher concentrations could translocate into the target cells with differences in fluorescence intensity under the same conditions, indicating different detoxification methods could result in PTds with different activities. The differences in translocation capacity on CHO cells between PTx and PTds are consistent with previous findings on their carbohydrate binding activities which added further scientific evidence to support the developed in vitro assay system as a potential alternative to HIST as safety test for ACV quality control.
Disclosure of Potential Conflicts of Interest
No potential conflicts of interest were disclosed.
References
- World Health Organization (WHO). Pertussis vaccines. WHO position paper. Wkly Epidemiol Rec 2005; 80:29 - 40; http://www.who.int/wer/2005/wer8004.pdf PMID: 15715139
- Tamura M, Nogimori K, Murai S, Yajima M, Ito K, Katada T, et al. Subunit structure of islet-activating protein, pertussis toxin, in conformity with the A-B model. Biochemistry 1982; 21:5516 - 22; http://dx.doi.org/10.1021/bi00265a021; PMID: 6293544
- Kaslow HR, Burns DL. Pertussis toxin and target eukaryotic cells: binding, entry, and activation. FASEB J 1992; 6:2684 - 90; PMID: 1612292
- Locht C, Coutte L, Mielcarek N. The ins and outs of pertussis toxin. FEBS J 2011; 278:4668 - 82; http://dx.doi.org/10.1111/j.1742-4658.2011.08237.x; PMID: 21740523
- Sekura RD, Fish F, Manclark CR, Meade B, Zhang YL. Pertussis toxin. Affinity purification of a new ADP-ribosyltransferase. J Biol Chem 1983; 258:14647 - 51; PMID: 6315733
- Tamura M, Nogimori K, Yajima M, Ase K, Ui M. A role of the B-oligomer moiety of islet-activating protein, pertussis toxin, in development of the biological effects on intact cells. J Biol Chem 1983; 258:6756 - 61; PMID: 6343381
- Wong WS, Rosoff PM. Pharmacology of pertussis toxin B-oligomer. Can J Physiol Pharmacol 1996; 74:559 - 64; http://dx.doi.org/10.1139/y96-045; PMID: 8884020
- Nencioni L, Pizza MG, Volpini G, De Magistris MT, Giovannoni F, Rappuoli R. Properties of the B oligomer of pertussis toxin. Infect Immun 1991; 59:4732 - 4; PMID: 1937835
- Ui M. In: Moss J, Vaughan M, editors. ADP-ribosylating toxins and G protein. Washington (DC): American Society for Microbiology; 1990; 45-77.
- Katada T, Ui M. Islet-activating protein. A modifier of receptor-mediated regulation of rat islet adenylate cyclase. J Biol Chem 1981; 256:8310 - 7; PMID: 7021547
- Loosmore SM, Zealey GR, Boux HA, Cockle SA, Radika K, Fahim RE, et al. Engineering of genetically detoxified pertussis toxin analogs for development of a recombinant whooping cough vaccine. Infect Immun 1990; 58:3653 - 62; PMID: 2228237
- Nencioni L, Pizza M, Bugnoli M, De Magistris T, Di Tommaso A, Giovannoni F, et al. Characterization of genetically inactivated pertussis toxin mutants: candidates for a new vaccine against whooping cough. Infect Immun 1990; 58:1308 - 15; PMID: 2323818
- Pizza M, Covacci A, Bartoloni A, Perugini M, Nencioni L, De Magistris MT, et al. Mutants of pertussis toxin suitable for vaccine development. Science 1989; 246:497 - 500; http://dx.doi.org/10.1126/science.2683073; PMID: 2683073
- Edwards KM, Meade BD, Decker MD, Reed GF, Rennels MB, Steinhoff MC, et al. Comparison of 13 acellular pertussis vaccines: overview and serologic response. Pediatrics 1995; 96:548 - 57; PMID: 7659475
- Sekura RD, Zhang YL, Roberson R, Acton B, Trollfors B, Tolson N, et al. Clinical, metabolic, and antibody responses of adult volunteers to an investigational vaccine composed of pertussis toxin inactivated by hydrogen peroxide. J Pediatr 1988; 113:806 - 13; http://dx.doi.org/10.1016/S0022-3476(88)80005-2; PMID: 3263485
- Gupta RK, Sharma SB, Ahuja S, Saxena SN. Glutaraldehyde inactivated pertussis vaccine: a less histamine sensitizing vaccine. J Biol Stand 1987; 15:159 - 64; http://dx.doi.org/10.1016/0092-1157(87)90038-2; PMID: 3110164
- Ibsen PH. The effect of formaldehyde, hydrogen peroxide and genetic detoxification of pertussis toxin on epitope recognition by murine monoclonal antibodies. Vaccine 1996; 14:359 - 68; http://dx.doi.org/10.1016/0264-410X(95)00230-X; PMID: 8735545
- Nogimori K, Ito K, Tamura M, Satoh S, Ishii S, Ui M. Chemical modification of islet-activating protein, pertussis toxin. Essential role of free amino groups in its lymphocytosis-promoting activity. Biochim Biophys Acta 1984; 801:220 - 31; http://dx.doi.org/10.1016/0304-4165(84)90071-0; PMID: 6541059
- Fowler S, Byron O, Jumel K, Xing D, Corbel MJ, Bolgiano B. Novel configurations of high molecular weight species of the pertussis toxin vaccine component. Vaccine 2003; 21:2678 - 88; http://dx.doi.org/10.1016/S0264-410X(03)00105-1; PMID: 12744905
- Petre J, Pizza M, Nencioni L, Podda A, De Magistris MT, Rappuoli R. The reaction of bacterial toxins with formaldehyde and its use for antigen stabilization. Dev Biol Stand 1996; 87:125 - 34; PMID: 8854009
- Fowler S, Xing DK, Bolgiano B, Yuen CT, Corbel MJ. Modifications of the catalytic and binding subunits of pertussis toxin by formaldehyde: effects on toxicity and immunogenicity. Vaccine 2003; 21:2329 - 37; http://dx.doi.org/10.1016/S0264-410X(03)00104-X; PMID: 12744863
- Habeeb AJ, Hiramoto R. Reaction of proteins with glutaraldehyde. Arch Biochem Biophys 1968; 126:16 - 26; http://dx.doi.org/10.1016/0003-9861(68)90554-7; PMID: 4174905
- Metz B, Kersten GF, Hoogerhout P, Brugghe HF, Timmermans HA, de Jong A, et al. Identification of formaldehyde-induced modifications in proteins: reactions with model peptides. J Biol Chem 2004; 279:6235 - 43; http://dx.doi.org/10.1074/jbc.M310752200; PMID: 14638685
- Adsorbed diphtheria-purified pertussis-tetanus combined vaccine. In: Minimum requirements for biological products. Ministry of Health, Japan, Labour and Welfare 2004; 41-9.
- Guidelines for the production and control of the acellular pertussis component of monovalent or combined vaccines. In: WHO Technical Report Series 1998; 878:57-72.
- Title 21. Pertussis vaccine 620.1. U.S. Code of federal regulations. Washington DC: U.S. Government Printing Office 1983; 58-61.
- Pertussis vaccine (acellular, component, adsorbed). Eur Pharmacopoeia 2000; Suppl 1050 - 2
- Graf R, Codina J, Birnbaumer L. Peptide inhibitors of ADP-ribosylation by pertussis toxin are substrates with affinities comparable to those of the trimeric GTP-binding proteins. Mol Pharmacol 1992; 42:760 - 4; PMID: 1435750
- Scheuring J, Schramm VL. Pertussis toxin: transition state analysis for ADP-ribosylation of G-protein peptide alphai3C20. Biochemistry 1997; 36:8215 - 23; http://dx.doi.org/10.1021/bi970379a; PMID: 9204866
- Finck-Barbançon V, Barbieri JT. ADP-ribosylation of alpha i3C20 by the S1 subunit and deletion peptides of S1 of pertussis toxin. Biochemistry 1995; 34:1070 - 5; http://dx.doi.org/10.1021/bi00003a043; PMID: 7827022
- Cyr T, Menzies AJ, Calver J, Whitehouse LW. A quantitative analysis for the ADP-ribosylation activity of pertussis toxin: an enzymatic-HPLC coupled assay applicable to formulated whole cell and acellular pertussis vaccine products. Biologicals 2001; 29:81 - 95; http://dx.doi.org/10.1006/biol.2001.0280; PMID: 11580213
- Yuen CT, Canthaboo C, Menzies JA, Cyr T, Whitehouse LW, Jones C, et al. Detection of residual pertussis toxin in vaccines using a modified ribosylation assay. Vaccine 2002; 21:44 - 52; http://dx.doi.org/10.1016/S0264-410X(02)00446-2; PMID: 12443661
- Gomez SR, Yuen C-T, Asokanathan C, Douglas-Bardsley A, Corbel MJ, Coote JG, et al. ADP-ribosylation activity in pertussis vaccines and its relationship to the in vivo histamine-sensitisation test. Vaccine 2007; 25:3311 - 8; http://dx.doi.org/10.1016/j.vaccine.2007.01.009; PMID: 17287049
- Gomez SR, Xing DKL, Corbel MJ, Coote J, Parton R, Yuen C-T. Development of a carbohydrate binding assay for the B-oligomer of pertussis toxin and toxoid. Anal Biochem 2006; 356:244 - 53; http://dx.doi.org/10.1016/j.ab.2006.05.012; PMID: 16782039
- Yuen CT, Horiuchi Y, Asokanathan C, Cook S, Douglas-Bardsley A, Ochiai M, et al. An in vitro assay system as a potential replacement for the histamine sensitisation test for acellular pertussis based combination vaccines. Vaccine 2010; 28:3714 - 21; http://dx.doi.org/10.1016/j.vaccine.2010.03.009; PMID: 20307594
- Nencioni L, Volpini G, Peppoloni S, Bugnoli M, De Magistris T, Marsili I, et al. Properties of pertussis toxin mutant PT-9K/129G after formaldehyde treatment. Infect Immun 1991; 59:625 - 30; PMID: 1702767
- Xing D, Yuen CT, Asokanathan C, Rigsby P, Horiuchi Y. Evaluation of an in vitro assay system as a potential alternative to current histamine sensitization test for acellular pertussis vaccines. Biologicals 2012; 40:456 - 65; http://dx.doi.org/10.1016/j.biologicals.2012.07.013; PMID: 22890129