Abstract
The potential use of Bacillus anthracis as a bioterrorism weapon threatens the security of populations globally, requiring the immediate availability of safe, efficient and easily delivered anthrax vaccine for mass vaccination. Extensive research efforts have been directed toward the development of recombinant subunit vaccines based on protective antigen (PA), the principal virulence factor of B. anthracis. Among the emerging technologies for the production of these vaccine antigens is our launch vector-based plant transient expression system. Using this system, we have successfully engineered, expressed, purified and characterized full-length PA (pp-PA83) in Nicotiana benthamiana plants using agroinfiltration. This plant-produced antigen elicited high toxin neutralizing antibody titers in mice and rabbits after two vaccine administrations with Alhydrogel. In addition, immunization with this vaccine candidate protected 100% of rabbits from a lethal aerosolized B. anthracis challenge. The vaccine effects were dose-dependent and required the presence of Alhydrogel adjuvant. In addition, the vaccine antigen formulated with Alhydrogel was stable and retained immunogenicity after two-week storage at 4°C, the conditions intended for clinical use. These results support the testing of this vaccine candidate in human volunteers and the utility of our plant expression system for the production of a recombinant anthrax vaccine.
Introduction
Bacillus anthracis, a Gram-positive, facultative anaerobic, spore-forming bacterium, is the etiologic agent of anthrax.Citation1,Citation2 Anthrax is a zoonotic disease, but it can occur in humans after exposure to infected animals or animal tissue.Citation3,Citation4 Due to the ability of the pathogen to form endospores that can be easily concealed, transported and released, B. anthracis is considered to have a high potential for use as a bioterrorism weapon,Citation5,Citation6 which was supported by the incidences of spore-containing letter attacks that occurred in the US in 2001.Citation7 Of three forms of the disease caused by B. anthracis – cutaneous, gastrointestinal and inhalation – anthrax caused by inhalation of aerosolized spores is most severe with the highest mortality rates of about 86–89%.Citation8
During anthrax infection, B. anthracis secretes protective antigen (PA), lethal factor (LF, a metalloprotease), and edema factor (EF, a calmodulin-dependent adenylate cyclase). In binary combinations, PA forms with LF and EF two exotoxins known as lethal toxin (LeTx = PA + LF) and edema toxin (EdTx = PA + EF). After binding to the cell surface, PA undergoes proteolytic activation and oligomerization, binds LF or EF, and facilitates the exotoxin entry into the cytoplasm, leading to the cell death.Citation9
Vaccination is considered to be the most effective measure of prophylaxis against anthrax infection. As shown in animal models, protective immunity against anthrax correlates with production of anti-PA antibodies neutralizing the activities of anthrax exotoxins.Citation10–Citation14 Therefore, PA has become the target for anthrax vaccine development. The only approved anthrax vaccine in the US is BioThrax® (Anthrax Vaccine Adsorbed) indicated for individuals at high risk of exposure.Citation15 The BioThrax® vaccine contains the 83 kDa PA protein prepared from cell-free filtrates of microaerophilic cultures of an avirulent, non-encapsulated strain of B. anthracis and formulated with aluminum hydroxide adjuvant. In addition to PA, the vaccine may also contain other anthrax proteins including LF and EF, which can potentially cause serious adverse events, in particular severe allergic reactions including anaphylaxis.Citation15 To achieve protective immunity, BioThrax® should be administered in five doses over a period of 18 mo and at 1-y intervals thereafter.Citation15 Due to the limitations of BioThrax®, such as undefined composition, lot-to-lot variability, multiple-dose administrations and safety concerns, efforts are being made to develop a safer and effective anthrax vaccine which can be administered in fewer doses.
Recombinant subunit vaccines, produced using recombinant DNA technology in heterologous expression systems, contain only target antigen and represent a safer and more reliable vaccine alternative to conventional vaccines. Recently, plants have emerged as a promising production system for manufacturing subunit vaccines, due to safety (e.g., lack of human pathogens), scalability, cost-effectiveness, and possession of eukaryotic post-translational protein modification machinery broadly similar to that of mammals.Citation16–Citation18 We have developed a transient plant-based expression system for the production of vaccine antigens, in which whole plants are infiltrated with Agrobacterium tumefaciens harboring a hybrid launch vector that contains elements of plant RNA viral vectors controlling target expression and an Agrobacterium binary plasmid to facilitate delivery to the plant cell nucleus.Citation19 This launch vector technology enables uniformly high levels of target protein expression in Nicotiana benthamiana leaves with rapid scale-up, and has been used to produce vaccine antigens from a number of pathogens that have been successfully evaluated in animal models.Citation20–Citation24
In this study, using our launch vector-based plant expression system, we have produced a vaccine candidate containing the full-length, 83 kDa PA (pp-PA83) protein of B. anthracis. The protein was purified from plants, characterized and evaluated for immunogenicity and protective efficacy in mice and rabbits, respectively.
Results
Production and characterization of plant-based recombinant pp-PA83
The engineered protein was produced in N. benthamiana and purified using three chromatography steps and two ultrafiltration and diafiltration (UF/DF) steps as described in Materials and Methods ().
Analysis by sodium dodecyl sulfate PAGE (SDS-PAGE) followed by Coomassie staining demonstrated > 90% purity of the pp-PA83 antigen (). pp-PA83 identity was confirmed by western blot analysis using anti-poly-histidine (His) tag and anti-PA monoclonal antibodies (mAbs) (). N-terminal sequencing for purified pp-PA83 was also performed and the results agree with the expected N-terminal sequence, confirming correct processing of the signal peptide with the protein retaining the correct N-terminus (). To further confirm the target identity, tri-enzyme digestion followed by liquid chromatography coupled with tandem mass spectrometry (LC/MS/MS) peptide mapping was performed. Data obtained by the chymotrypsin, elastase and trypsin digestion provided 100% coverage of the pp-PA83 sequence, further confirming that the purified target molecule is PA83 (data not shown).
Figure 2. Electrophoretic mobility and immunoblot analysis of purified pp-PA83. (A) Coomassie-stained SDS-PAGE. pp-PA83 was loaded at 1 µg/lane. (B and C) Immunoblot. pp-PA83 was loaded at 100 ng/lane. pp-PA83 was separated by SDS-PAGE, transferred to polyvinylidene fluoride membranes and blotted with (B) a commercially available anti-4 × His mAb or (C) a pp-mAbPA.
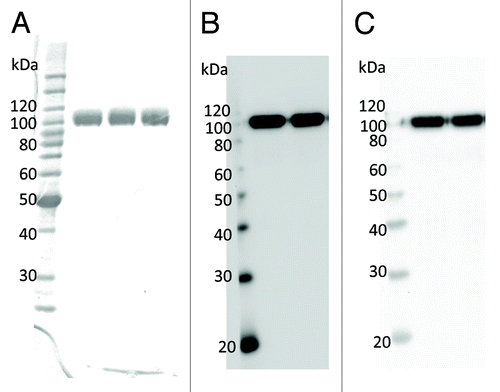
Figure 3. N-terminal sequencing of purified pp-PA83. Identification of the first five amino acids confirms that the signal peptide was processed correctly.
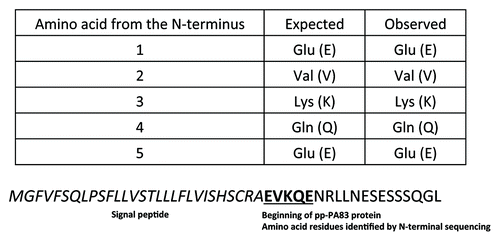
Fractionation of purified pp-PA83 over a size exclusion chromatography (SEC) column followed by detection with multi-angle laser light spectroscopy (MALLS) demonstrated that in solution purified pp-PA83 is predominantly present as a monomer (). As further determined by MALLS, both the leading peaks of pp-PA83 at ~12 mL and ~13.6 mL had similar calculated molar weights, 84.9 ± 1.3 kDa ().
Figure 4. Representative SEC-MALLS profile for pp-PA83. pp-PA83 elutes from SEC with two main peaks at ~12 mL and ~13.6 mL, and a light scattering calculated average molecular weight of 84.9 ± 1.3 kDa.
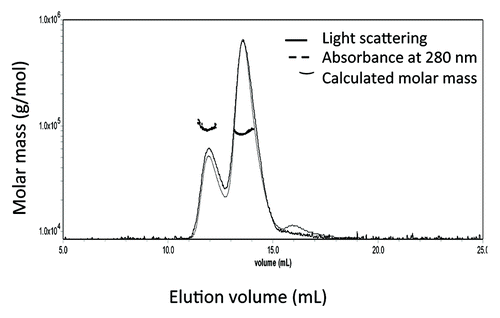
Evaluation of the pp-PA83 amino acid sequence revealed nine potential N-linked glycosylation sites. Glycosylation profiling by LC/MS/MS determined that six out of nine sites have some level of glycosylation (Asn-246, 328, 388, 476, 570 and 664) (data not shown). The results of glycosylation profiling are further supported by western blot analysis of pp-PA83 ( and B) with and without treatment with Peptide: N-Glycosidase F (PNGase F). PNGase F treatment resulted in a single reduced-size band as a result of glycan removal (), which is in agreement with the presence of a single N-terminus in the purified preparation (). The results of microfluidic electrophoresis further confirmed the results of western blot analysis demonstrating that there are multiple glycoforms, and also showed that the presence of multiple glycoforms is consistent between different preparations and production scales of pp-PA83 (data not shown).
Figure 5. Glycoform analysis of pp-PA83 by SDS-PAGE and western blotting. Protein bands were visualized by Coomassie staining (A) and western blotting using pp-mAbPA (B). Separated bands are shown with arrowheads in (B). Western blot analysis of pp-PA83 with (+) and without (-) pre-treatment with PNGase F (C).
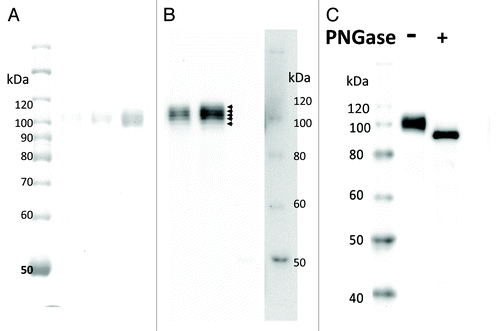
Storage stability of the formulated pp-PA83 antigen
Stability of pp-PA83 in saline at a clinically relevant concentration adsorbed to the 0.3% Alhydrogel® was also monitored. In order to determine if the target molecule stays bound to the adjuvant for more than 2 weeks (conditions intended for clinical use), we used protein absorbance at 280 nm as a measure of protein concentration in the supernatant of Alhydrogel-bound samples. shows the data obtained for a period of 7-week storage at 2–8°C for the supernatant of the control sample (pp-PA83 without Alhydrogel) and the supernatant of the formulated vaccine candidate after the removal of the adjuvant by centrifugation. The absence of absorbance in the supernatant of the formulated pp-PA83 suggested that pp-PA83 stays bound to the adjuvant for at least 7 weeks when stored at 2–8°C. Furthermore, thermal stability analysis demonstrated no changes in melting temperature (Tm) values for either the control sample or the formulated vaccine antigen, indicating that in both cases pp-PA83 retained its global stability ().
Immunogenicity of pp-PA83 in mice
The initial proof-of-concept immunogenicity study evaluated a dose range of 45, 15 and 3 µg of pp-PA83 plus Alhydrogel in mice. Assessment of sera from individual mice indicated that two administrations of 45 or 15 µg of pp-PA83 plus Alhydrogel elicited high titers of anti-PA IgG observed on study day 42 (3 weeks post second vaccination) that were maintained through study day 70 (). Furthermore, by study day 70, pp-PA83 plus Alhydrogel elicited high toxin-neutralizing antibody (TNA) titers in 100% and 50% of vaccinated mice after administering two doses of 45 and 15 µg of antigen, respectively (). In contrast, two administrations of 3 µg of pp-PA83 plus Alhydrogel elicited low anti-PA IgG titers on both study days 42 and 70, and failed to elicit TNA titers in mice by study day 70 ( and B).
Table 1A. Anti-PA IgG titers in mice immunized with pp-PA83 plus Alhydrogel
Table 1B. LeTx neutralizing antibody titers in mice immunized with pp-PA83 plus Alhydrogel
In a follow up study, the immunogenicity of the formulated pp-PA83 vaccine stored on 0.3% Alhydrogel at 4°C for 2 weeks was evaluated and compared with that of freshly prepared vaccine with or without Alhydrogel. Assessment of anti-PA IgG and TNA titers in sera of individual mice that received two 7.5 µg doses of adjuvanted pp-PA83 indicated that the biological activity of the vaccine was retained after 2-week storage at 4°C on Alhydrogel (). Moreover, 2-week storage at 4°C appeared to enhance the TNA response elicited by the formulated vaccine compared with responses generated by the freshly prepared vaccine (). No detectable immune responses were generated by the pp-PA83 vaccine in the absence of Alhydrogel ().
Table 2. Immunogenicity of formulated pp-PA83 plus Alhydrogel in mice
Taken together, the results of these studies indicate that plant-produced recombinant pp-PA83 administered with Alhydrogel adjuvant is immunogenic in mice and capable of eliciting anthrax LeTx neutralizing antibodies.
Immunogenicity of pp-PA83 in rabbits
The immunogenicity of pp-PA83 was further evaluated in rabbits. In this study, New Zealand White rabbits received three administrations of 135, 67.5, 22.5 or 7.5 µg of pp-PA83 plus Alhydrogel on study days 0, 21 and 42. Assessment of serum anti-PA IgG titers on study day 35 demonstrated that two administrations of the pp-PA83 vaccine plus Alhydrogel at 125 and 67.5 µg doses elicited strong anti-PA IgG as well as TNA responses with weaker responses detected in groups that received 22.5 or 7.5 µg ( and ). With the addition of a third dose of pp-PA83 plus Alhydrogel, strong serum anti-PA IgG and TNA responses were elicited in 100% of vaccinated animals by study day 56 at all dose levels tested in this study ( and B). Thus, pp-PA83 plus Alhydrogel is immunogenic in rabbits after two doses at levels equal or above 67.5 µg and after three doses down to a 7.5 µg dose.
Table 3A. Anti-PA IgG titers in rabbits immunized with pp-PA83 plus Alhydrogel
Table 3B. LeTx neutralizing antibody titers in rabbits immunized with pp-PA83 plus Alhydrogel
Protection of pp-PA83-vaccinated rabbits against inhalation challenge with B. anthracis spores
The objective of this study was to determine the protective efficacy of the pp-PA83 vaccine against B. anthracis (Ames strain) in New Zealand White rabbits. Rabbits were immunized with a dose range of the pp-PA83 vaccine: 135, 67.5, 22.5 or 7.5 µg plus Alhydrogel. Following two vaccine administrations, animals that received either 135 or 67.5 µg of pp-PA83 developed strong anti-PA IgG as well as TNA responses, with significant TNA titers detected in 88% of rabbits. The levels of antigen-specific immune responses were shown to be proportional to the pp-PA83 antigen dose level ( and ). Following a third vaccination on study day 42, the rabbits were challenged with a mean of 368 lethal dose 50 (LD50) aerosolized B. anthracis Ames strain spores. Unvaccinated animals succumbed to infection within 4 d post challenge, while 100% of the vaccinated animals at all doses tested survived the infection (). Challenged vaccinated rabbits showed no clinical signs of anthrax post challenge and bacteremia was only identified in the unvaccinated control group (data not shown). In conclusion, the pp-PA83 vaccine demonstrated 100% protective efficacy against inhalation anthrax.
Table 4A. Anti-PA IgG titers in rabbits immunized with pp-PA83 plus Alhydrogel prior to challenge
Table 4B. LeTx neutralizing antibody titers in rabbits immunized with pp-PA83 plus Alhydrogel prior to challenge
Figure 7. Percent survival of pp-PA83-immunized rabbits after inhalation challenge with B. anthracis. New Zealand White rabbits were vaccinated with three doses of pp-PA83 plus Alhydrogel on study days 0, 21 and 42, and then challenged intranasally with 368 LD50 of aerosolized B. anthracis Ames strain on study day 53.
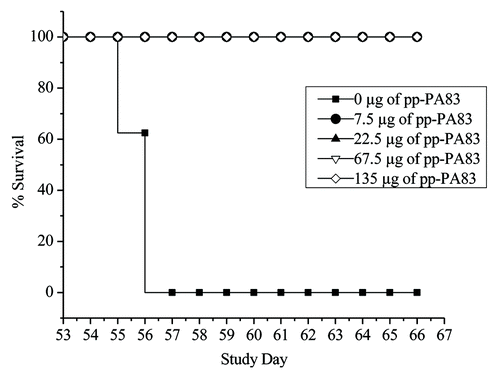
Discussion
The potential bioterror threat that anthrax poses along with the limitations of the licensed conventional anthrax vaccine have stimulated significant efforts toward the development of a safe and effective subunit anthrax vaccine based on recombinant PA (rPA), the principal virulence factor of B. anthracis. Several experimental rPA vaccine candidates have been produced in bacterial expression systems. These vaccines have been demonstrated to elicit serum anti-PA and LeTx neutralizing antibodies and to confer protection against lethal aerosolized anthrax challenge in several animal models, including rabbits and non-human primates.Citation13,Citation25–Citation27 In addition, several of these vaccine candidates have been shown to be safe and immunogenic in humans, despite differences in the amount of Alhydrogel adjuvant used and the number of doses administered.Citation28–Citation31
Plant expression systems have recently demonstrated their potential for producing safe and cost-effective vaccine antigens, mAbs and therapeutic proteins,Citation16–Citation18 some of which are undergoing clinical development and a few of them are on the market.Citation32 Several groups have developed plant-based expression systems for the production of rPA. Using a transplastomic plant approach, anthrax rPA was successfully produced in tobacco chloroplasts.Citation33 Subcutaneous immunization of mice with partially purified, adjuvanted, chloroplast-derived rPA was demonstrated to elicit high anti-PA IgG titers (up to 1:320,000) in mouse serum and confer 100% survival of mice upon challenge with lethal doses of LeTx, the same protection level as provided by B. anthracis-derived PA.Citation33 In a previous study conducted at Fraunhofer USA Center for Molecular Biotechnology, a launch vector-based transient plant expression approach was used to produce a dual subunit anthrax vaccine comprising domain 4 of PA and domain 1 of LF that were separately fused to the engineered lichenase protein (LicKM)Citation19 used as a carrier molecule. Two administrations of this dual vaccine adjuvanted with complete or incomplete Freund’s adjuvant were shown to generate high titers of anti-PA and anti-LF IgG1 as well as LeTx neutralizing antibodies in mice.Citation20
In the current study, we have used the same launch vector-based transient plant expression system to engineer and produce in N. benthamiana a single-component anthrax vaccine candidate based on non-fused, full-length rPA (pp-PA83), and demonstrated its immunogenicity in mice and rabbits. In addition, the protective efficacy of the pp-PA83 vaccine candidate was evaluated in a rabbit challenge model. The rabbit model has been used to understand the pathogenesis of anthrax and is considered to be a good model for inhalation anthrax.Citation34 Inhalation challenge of B. anthracis Ames strain in rabbits is highly pathogenic and induces a rapid and fatal disease, with death occurring within 2 to 4 d after exposure. Pathological changes and patterns are similar to those found in rhesus monkeys and humans that succumb to inhalation anthrax. The rabbit model is believed to be predictive of human disease and has been used in numerous vaccine efficacy studies.Citation12,Citation26 Using this rabbit challenge model, the pp-PA83 vaccine immunogenicity and protective efficacy were shown to be dose-dependent and required the presence of Alhydrogel adjuvant to confer 100% protection at doses as low as 7.5 µg. Furthermore, the vaccine antigen formulated and adsorbed on Alhydrogel was stable and retained immunogenicity after 2-week storage at 4°C, the conditions intended for clinical use. The results of this study support clinical development of this vaccine candidate and the utility of our plant expression system for the production of a recombinant anthrax vaccine.
Materials and Methods
Cloning, expression in plants and purification of pp-PA83
To engineer pp-PA83, the nucleotide sequence encompassing amino acids 30–764 of B. anthracis PA (GenBank accession number P13423) was optimized for expression in plants and synthesized by GENEART AG (Regensburg, Germany). Optimized pp-PA83 was engineered to contain the N-terminal tobacco PR-1a signal peptide (removed during protein secretion) and C-terminal 6 × His affinity purification tagCitation35 and the endoplasmic reticulumretention signal (KDEL).Citation36 The resulting sequence was inserted into the launch vector pGRD4Citation19,Citation23 to obtain the pGRD4-PA83 construct. The construct was transformed into A. tumefaciens strain GV3101 which was then introduced into hydroponically grown N. benthamiana by vacuum infiltration, as described previously.Citation23,Citation37
At 7 days post infiltration, leaf tissues of the infiltrated plants were harvested, homogenized and extracted in a phosphate-based buffer containing 20 mM imidazole and 1mM diethylcarbamic acid. The extract was clarified by centrifugation and microfiltration. The recombinant PA83 antigen was captured by immobilized metal affinity chromatography (IMAC; Ni-Sepharose, GE Healthcare Biosciences, Pittsburgh, PA), and eluted with a phosphate-based buffer containing 300 mM imidazole. In preparation for the polishing step, 0.65 M ammonium sulfate was added to the IMAC eluted sample, and the antigen was further purified using polishing hydrophobic interaction chromatography (HIC) (GE Healthcare Biosciences) in the flow-through (FT) mode. Target in the FT fraction was concentrated and buffer exchanged into the 20 mM NaPi, pH 7.5 by ultrafiltration/diafiltration (UF/DF) (using a membrane with molecular weight cutoff of 5 kDa, EMD Millipore, Billerica, MA), followed by refining ion exchange chromatography (IEX; GE Healthcare Biosciences) in the binding mode. The pp-PA83 antigen was eluted from the IEX column with the buffer containing 120 mM NaCl, and concentrated and buffer exchanged into phosphate-buffered saline (PBS), pH 7.5 by the second UF/DF step. The entire pp-PA83 purification process was performed at the same pH. The purified pp-PA83 preparation was filter-sterilized using a 0.2 µm membrane (Millipore, Billerica, MA), aliquoted and stored at -70°C.
pp-PA83 product characterization
Purity of purified pp-PA83 was determined by separation by 10% SDS-PAGE followed by staining with Coomassie blue (Pierce, Rochford, IL), as well as by reversed phase ultra-performance liquid chromatography (RP-UPLC) using a Waters Acquity system (Waters, Milford, MA) with a C4 column and water/acetonitrile (both containing 0.1% trifluoroacetic acid) mobile phases. The yield of pp-PA83 was estimated by measuring optical density (OD) of the preparations at 280 nm.
Identity of recombinant pp-PA83 was confirmed by reactivity in immunoblots using a mAb specific to the His tag (Qiagen, Valencia, CA) or the plant-produced mAb targeting the functional epitope located in the domain 4 of the PA83 molecule (pp-mAbPA) (Fraunhofer USA CMB, Newark, DE). To confirm the identity of pp-PA83, peptide mapping was also performed by MS Bioworks LLC. using tri-enzyme digestion and analyzing the gel-digest by LC/MS/MS using the Waters NanoAcquity high-performance liquid chromatography (HPLC) system (Waters) interfaced to a LTQ Orbitrap Velos (Thermo Fisher Scientific, Pittsburgh, USA). Correct signal peptide processing and retention of the N-terminus were assessed by N-terminal sequencing conducted at Proteos, Inc. using automated Edman degradation in an Applied Biosciences model 494 sequencer (Life Technologies Corp., Carlsbad, CA).
The solution state and molecular weight of purified pp-PA83 were assessed by SEC using Superdex 200 10/300 mm GL column (GE Healthcare) coupled with MALLS (Wyatt Technologies, Santa Barbara, CA). Post-translational modifications due to glycosylation were assessed using treatment with PNGase F (New England BioLabs, Ipswich, MA) according to the manufacturer’s instructions as well as by LC/MS/MS using the Waters NanoAcquity HPLC system interfaced to a LTQ Orbitrap Velos.
Stability assessments of pp-PA83
Stability of formulated pp-PA83 was monitored at a clinically relevant concentration of 0.1 mg/mL in saline after adsorbing to 0.3% Alhydrogel. pp-PA83 in saline (0.1 mg/mL) without adjuvant was used as a control. Stability was evaluated by measuring the OD of the sample with and without the adjuvant. In brief, pp-PA83 at the clinically relevant concentration of 0.1 mg/mL in saline solution adsorbed on 0.3% Alhydrogel was stored at 2–8°C. Absorbance at 280 nm was measured on the supernatants of the control sample (without the adjuvant) and the Alhydrogel-bound sample after adjuvant removal via centrifugation, for a period of 7 weeks. Thermal stability of the target molecule in the presence or absence of the adjuvant was also assessed to monitor the storage stability at 2–8°C for a period of 7 weeks, as described previously.Citation38
Immunogenicity studies with pp-PA83
Immunogenicity of the recombinant pp-PA83 antigen was evaluated in mice and rabbits. The animal protocols were approved by the Institutional Animals Care and Use Committees (IACUC) at the University of Delaware (Newark, DE) and Covance Discovery and Translational Services (Denver, PA) for the mouse and rabbit studies, respectively.
pp-PA83 immunogenicity studies in mice
In the first study, 7-week-old BALB/c mice (Harlan, Indianapolis, IN), 6 per group, were immunized intramuscularly (IM) on study days 0 and 21 with pp-PA83 at doses of 45, 15 or 3 µg adsorbed to a 1:10 ratio ([w:w]) of Alhydrogel (Accurate Chemicals, Westbury, NY). Serum samples were collected on days -1 (pre-bleed), 20 (prior to second vaccination), 42 (post second vaccination) and 70. In the second study, mice received 7.5 µg of the pp-PA83 vaccine on study days 0 and 21 formulated with 0.3% Alhydrogel and stored at 4°C for 2 weeks. Serum samples were collected on days -1 (pre-bleed), 20 (prior to second vaccination), 42 (post second vaccination) and 70.
pp-PA83 immunogenicity studies in rabbits
New Zealand White rabbits (Covance Research Products, Denver, PA), 5 per group, were immunized IM with 135, 67.5, 22.5 or 7.5 µg of pp-PA83 plus 0.1% Alhydrogel® (0.5 mg/mL aluminum) on study days 0, 21 and 42. The vaccine was prepared by thawing a frozen stock and diluting it in saline prior to injection. In the control group, animals received saline plus Alhydrogel only on the same study days. Serum samples were collected on days -1 (pre-bleed), 21 (prior to second vaccination), 35 (post second vaccination) and 56 (post 3rd vaccination).
Assessment of serum anti-PA IgG and TNA antibody titers
Serum anti-PA IgG was detected by enzyme-linked immunosorbent assay (ELISA) as described previously,Citation20 and data was expressed as the geometric mean titer (GMT) ± standard error (SE) per group. Briefly, serum samples were titrated on plates coated with commercially available PA (List Biological Laboratories, Campbell, CA). End point titers were defined as reciprocal serum dilutions that give a mean OD value three times greater than the pre-immune control samples. TNA titers in sera of immunized mice were measured using a LeTx neutralization assay as described previouslyCitation39 and expressed as the GMT of the Effective Dilution 50 (ED50) values per group. Briefly, serum samples were titrated on a plated murine macrophage cell line (RAW 264.7, ATCC, Manassas, VA) in the presence or absence of LeTx. After incubation, cell viability was accessed by the addition of WST-1 (Roche Applied Sciences, Indianapolis, IN), a proliferation reagent, followed by a spectrophotometric measurement at 450 nm. In addition, the percent responders in a given group was calculated based on the ability of serum antibodies to equal or exceed the TNA activity of an anti-PA mAb used as an assay control.Citation40
B. anthracis inhalation challenge study in rabbits
The inhalation challenge study was conducted at Southern Research Institute (Birmingham, AL). New Zealand White rabbits, 8 per group (4 male and 4 female), were immunized IM with 135, 67.5, 22.5 or 7.5 µg of pp-PA83 plus 0.1% Alhydrogel (0.5 mg/mL aluminum) on study days 0, 21 and 42. A frozen stock vial of pp-PA83 stored in PBS was thawed, diluted in saline to the desired concentration and mixed with 0.1% Alhydrogel cage-side. In the control group, animals received saline plus Alhydrogel only on the same study days. Serum samples were collected on days -1 (pre-bleed), 20 (prior to second vaccination) and 41 (post second vaccination) and analyzed for anti-PA IgG and TNA antibody titers.
On study day 53, the immunized rabbits were challenged by nose-only inhalation using head-out plethysmography and monitored for up to 14 d post challenge. The rabbits received a mean of 368 LD50 (1 LD50 = 105,000 colony forming units), ranging between 100 to 555 LD50, of B. anthracis Ames strain. Clinical signs as well as body temperatures and weights were monitored daily. Blood was collected on study days 0, 20 and 41 (prior to vaccination) and on study day 66, and analyzed for the presence or absence of B. anthracis. Percent survival data were displayed using the Kaplan-Meier plot.
Acknowledgments
The authors would like to thank MS Bioworks LLC. and Proteos, Inc. for performing peptide mapping and N-terminal sequencing, respectively. Covance Research Products Inc. and Southern Research Institute conducted the rabbit immunogenicity and challenge studies, respectively. The authors would like to thank Robert Stevens and Rebecca Snow for technical support and Dr. Natasha Kushnir for editorial assistance. This project was supported by grants from the Naval Medical Research Center and the Defense Threat Reduction Agency (contract number HDTRA1-09-C-0049).
Submitted
11/05/12
Accepted
11/21/12
Disclosure of Potential Conflicts of Interest
No potential conflicts of interest were disclosed.
References
- Koch R.. The aetiology of anthrax based on the ontogeny of the anthrax bacillus. Med Classics 1937; 2:787 - 821
- Brachman P, Kauffman A. Anthrax. 1998. In Evans AS and Brachman PS (Eds.). Bacterial infections of humans. New York: Plenum Medical Book Company.
- Davies JCA. A major epidemic of anthrax in Zimbabwe. Cent Afr J Med 1982; 28:291 - 8; PMID: 7168859
- Van Ness GB. Ecology of anthrax. Science 1971; 172:1303 - 7; http://dx.doi.org/10.1126/science.172.3990.1303; PMID: 4996306
- Inglesby TV, Henderson DA, Bartlett JG, Ascher MS, Eitzen E, Friedlander AM, et al, Working Group on Civilian Biodefense. Anthrax as a biological weapon: medical and public health management. [Consensus statement from the Working Group on Civilian Biodefense] JAMA 1999; 281:1735 - 45; http://dx.doi.org/10.1001/jama.281.18.1735; PMID: 10328075
- Department of Homeland Security. 2006. Bioterrorism risk assessment. Biological Threat Characterization Center of the National Biodefense Analysis and Countermeasures Center. Fort Detrick, MD.
- Jernigan JA, Stephens DS, Ashford DA, Omenaca C, Topiel MS, Galbraith M, et al, Anthrax Bioterrorism Investigation Team. Bioterrorism-related inhalational anthrax: the first 10 cases reported in the United States. Emerg Infect Dis 2001; 7:933 - 44; http://dx.doi.org/10.3201/eid0706.010604; PMID: 11747719
- Brachman PS, Plotkin SA, Bumford FH, Atchison MM. An epidemic of inhalation anthrax: the first in the twentieth century. II. Epidemiology. Am J Hyg 1960; 72:6 - 23; PMID: 13803672
- Young JA, Collier RJ. Anthrax toxin: receptor binding, internalization, pore formation, and translocation. Annu Rev Biochem 2007; 76:243 - 65; http://dx.doi.org/10.1146/annurev.biochem.75.103004.142728; PMID: 17335404
- Little SF, Ivins BE, Fellows PF, Friedlander AM. Passive protection by polyclonal antibodies against Bacillus anthracis infection in guinea pigs. Infect Immun 1997; 65:5171 - 5; PMID: 9393812
- Fowler K, McBride BW, Turnbull PC, Baillie LW. Immune correlates of protection against anthrax. J Appl Microbiol 1999; 87:305; http://dx.doi.org/10.1046/j.1365-2672.1999.00898.x; PMID: 10475976
- Pitt ML, Little SF, Ivins BE, Fellows P, Barth J, Hewetson J, et al. In vitro correlate of immunity in a rabbit model of inhalational anthrax. Vaccine 2001; 19:4768 - 73; http://dx.doi.org/10.1016/S0264-410X(01)00234-1; PMID: 11535328
- Little SF, Ivins BE, Fellows PF, Pitt ML, Norris SL, Andrews GP. Defining a serological correlate of protection in rabbits for a recombinant anthrax vaccine. Vaccine 2004; 22:422 - 30; http://dx.doi.org/10.1016/j.vaccine.2003.07.004; PMID: 14670324
- Weiss S, Kobiler D, Levy H, Marcus H, Pass A, Rothschild N, et al. Immunological correlates for protection against intranasal challenge of Bacillus anthracis spores conferred by a protective antigen-based vaccine in rabbits. Infect Immun 2006; 74:394 - 8; http://dx.doi.org/10.1128/IAI.74.1.394-398.2006; PMID: 16368995
- BioThrax® (Anthrax Vaccine Adsorbed). Prescribing Information. Emergent BioSolutions. May 2012.
- Chichester JA, Yusibov V. Plants as alternative systems for production of vaccines. Hum Vaccin 2007; 3:146 - 8; http://dx.doi.org/10.4161/hv.3.4.4148; PMID: 17643065
- Mett V, Farrance CE, Green BJ, Yusibov V. Plants as biofactories. Biologicals 2008; 36:354 - 8; http://dx.doi.org/10.1016/j.biologicals.2008.09.001; PMID: 18938088
- Rybicki EP. Plant-made vaccines for humans and animals. Plant Biotechnol J 2010; 8:620 - 37; http://dx.doi.org/10.1111/j.1467-7652.2010.00507.x; PMID: 20233333
- Musiychuk K, Stephenson N, Bi H, Farrance CE, Orozovic G, Brodelius M, et al. A launch vector for the production of vaccine antigens in plants. Influenza Other Respi Viruses 2007; 1:19 - 25; http://dx.doi.org/10.1111/j.1750-2659.2006.00005.x; PMID: 19453476
- Chichester JA, Musiychuk K, de la Rosa P, Horsey A, Stevenson N, Ugulava N, et al. Immunogenicity of a subunit vaccine against Bacillus anthracis. Vaccine 2007; 25:3111 - 4; http://dx.doi.org/10.1016/j.vaccine.2007.01.068; PMID: 17280756
- Mett V, Lyons J, Musiychuk K, Chichester JA, Brasil T, Couch R, et al. A plant-produced plague vaccine candidate confers protection to monkeys. Vaccine 2007; 25:3014 - 7; http://dx.doi.org/10.1016/j.vaccine.2007.01.017; PMID: 17287055
- Shoji Y, Chichester JA, Bi H, Musiychuk K, de la Rosa P, Goldschmidt L, et al. Plant-expressed HA as a seasonal influenza vaccine candidate. Vaccine 2008; 26:2930 - 4; http://dx.doi.org/10.1016/j.vaccine.2008.03.045; PMID: 18440103
- Shoji Y, Bi H, Musiychuk K, Rhee A, Horsey A, Roy G, et al. Plant-derived hemagglutinin protects ferrets against challenge infection with the A/Indonesia/05/05 strain of avian influenza. Vaccine 2009; 27:1087 - 92; http://dx.doi.org/10.1016/j.vaccine.2008.11.108; PMID: 19100806
- Shoji Y, Chichester JA, Jones M, Manceva SD, Damon E, Mett V, et al. Plant-based rapid production of recombinant subunit hemagglutinin vaccines targeting H1N1 and H5N1 influenza. Hum Vaccin 2011; 7:Suppl 41 - 50; http://dx.doi.org/10.4161/hv.7.0.14561; PMID: 21266846
- Ivins BE, Pitt ML, Fellows PF, Farchaus JW, Benner GE, Waag DM, et al. Comparative efficacy of experimental anthrax vaccine candidates against inhalation anthrax in rhesus macaques. Vaccine 1998; 16:1141 - 8; http://dx.doi.org/10.1016/S0264-410X(98)80112-6; PMID: 9682372
- Fellows PF, Linscott MK, Ivins BE, Pitt ML, Rossi CA, Gibbs PH, et al. Efficacy of a human anthrax vaccine in guinea pigs, rabbits, and rhesus macaques against challenge by Bacillus anthracis isolates of diverse geographical origin. Vaccine 2001; 19:3241 - 7; http://dx.doi.org/10.1016/S0264-410X(01)00021-4; PMID: 11312020
- Williamson ED, Hodgson I, Walker NJ, Topping AW, Duchars MG, Mott JM, et al. Immunogenicity of recombinant protective antigen and efficacy against aerosol challenge with anthrax. Infect Immun 2005; 73:5978 - 87; http://dx.doi.org/10.1128/IAI.73.9.5978-5987.2005; PMID: 16113318
- Gorse GJ, Keitel W, Keyserling H, Taylor DN, Lock M, Alves K, et al. Immunogenicity and tolerance of ascending doses of a recombinant protective antigen (rPA102) anthrax vaccine: a randomized, double-blinded, controlled, multicenter trial. Vaccine 2006; 24:5950 - 9; http://dx.doi.org/10.1016/j.vaccine.2006.05.044; PMID: 16797805
- Campbell JD, Clement KH, Wasserman SS, Donegan S, Chrisley L, Kotloff KL. Safety, reactogenicity and immunogenicity of a recombinant protective antigen anthrax vaccine given to healthy adults. Hum Vaccin 2007; 3:205 - 11; http://dx.doi.org/10.4161/hv.3.5.4459; PMID: 17881903
- Bellanti JA, Lin FY, Chu C, Shiloach J, Leppla SH, Benavides GA, et al. Phase 1 study of a recombinant mutant protective antigen of Bacillus anthracis. Clin Vaccine Immunol 2012; 19:140 - 5; http://dx.doi.org/10.1128/CVI.05556-11; PMID: 22190398
- PharmAthene. RECOMBINANT PROTECTIVE ANTIGEN (rPA) ANTHRAX VACCINES SPARVAX(TM) – NOVEL SECOND GENERATION VACCINE TECHNOLOGY. http://www.pharmathene.com/FactSheet_SparVax.pdf (accessed 10 October 2012).
- Yusibov V, Streatfield SJ, Kushnir N. Clinical development of plant-produced recombinant pharmaceuticals: vaccines, antibodies and beyond. Hum Vaccin 2011; 7:313 - 21; http://dx.doi.org/10.4161/hv.7.3.14207; PMID: 21346417
- Koya V, Moayeri M, Leppla SH, Daniell H. Plant-based vaccine: mice immunized with chloroplast-derived anthrax protective antigen survive anthrax lethal toxin challenge. Infect Immun 2005; 73:8266 - 74; http://dx.doi.org/10.1128/IAI.73.12.8266-8274.2005; PMID: 16299323
- Zaucha GM, Pitt LM, Estep J, Ivins BE, Friedlander AM. The pathology of experimental anthrax in rabbits exposed by inhalation and subcutaneous inoculation. Arch Pathol Lab Med 1998; 122:982 - 92; PMID: 9822127
- Porath J. Immobilized metal ion affinity chromatography. Protein Expr Purif 1992; 3:263 - 81; http://dx.doi.org/10.1016/1046-5928(92)90001-D; PMID: 1422221
- Herman EM, Tague BW, Hoffman LM, Kjemtrupp SE, Chrispeels MJ. Retention of phytohemagglutinin with carboxyterminal tetrapeptide KDEL in the nuclear envelope and the endoplasmic reticulum. Planta 1990; 182:302 - 12; http://dx.doi.org/10.1007/BF00197126
- Green BJ, Fujiki M, Mett V, Kaczmarczyk J, Shamloul M, Musiychuk K, et al. Transient protein expression in three Pisum sativum (green pea) varieties. Biotechnol J 2009; 4:230 - 7; http://dx.doi.org/10.1002/biot.200800256; PMID: 19156736
- Ausar SF, Chan J, Hoque W, James O, Jayasundara K, Harper K. Application of extrinsic fluorescence spectroscopy for the high throughput formulation screening of aluminum-adjuvanted vaccines. J Pharm Sci 2011; 100:431 - 40; http://dx.doi.org/10.1002/jps.22282; PMID: 20740682
- Hull AK, Criscuolo CJ, Mett V, Groen H, Steeman W, Westra H, et al. Human-derived, plant-produced monoclonal antibody for the treatment of anthrax. Vaccine 2005; 23:2082 - 6; http://dx.doi.org/10.1016/j.vaccine.2005.01.013; PMID: 15755575
- Mett V, Chichester JA, Stewart ML, Musiychuk K, Bi H, Reifsnyder CJ, et al. A non-glycosylated, plant-produced human monoclonal antibody against anthrax protective antigen protects mice and non-human primates from B. anthracis spore challenge. Hum Vaccin 2011; 7:Suppl 183 - 90; http://dx.doi.org/10.4161/hv.7.0.14586; PMID: 21270531