Abstract
Colonization of the nasopharynx by non-pathogenic Neisseria species, including N. lactamica, has been suggested to lead to the acquisition of natural immunity against Neisseria meningitidis in young children. The aim of this study was to identify a model complex of antigens and adjuvant for immunological preparation against N. meningitidis B, based on cross reactivity with N. lactamica outer membrane vesicles (OMV) antigens and the (DDA-BF) adjuvant. Complexes of 25 µg of OMV in 0.1 mM of DDA-BF were colloidally stable, exhibiting a mean diameter and charge optimal for antigen presentation. Immunogenicity tests for these complexes were performed in mice. A single dose of OMV/DDA-BF was sufficient to induce a (DTH) response, while the same result was achieved only after two doses of OMV/alum. In addition, to achieve total IgG levels that are similar to a single immunization with OMV/DDA-BF, it was necessary to give the mice a second dose of OMV/alum. Moreover, the antibodies induced from a single immunization with OMV/DDA-BF had an intermediate avidity, but antibodies with a similar avidity were only induced by OMV/alum after two immunizations. The use of this novel cationic adjuvant for the first time with a N. lactamica OMV preparation revealed good potential for future vaccine design.
Introduction
The encapsulated gram-negative bacteria Neisseria meningitidis represents a serious health problem in many parts of the world, and it has long been a challenge for vaccine producers.Citation1 Immunological and epidemiological evidence suggests that the development of a natural immunity to meningococcal disease results from the colonization of the nasopharynx by commensal Neisseria sp, particularly N. lactamica.Citation2 Moreover, the outer membrane proteins (OMPs) from group B meningococci have been shown to be clearly immunogenic in humans.Citation3 Several OMP-based vaccines for the group B meningococcal disease have shown 50 to 80% efficacy in older children.Citation4 However, the efficacy for these vaccines in young children is much lower, despite the induction of higher levels of antibodies.Citation4,Citation5 Natural immunity against N. meningitidis is acquired during childhood and adolescence through the successive colonization by a commensal Neisseria carrier, N. meningitidis, as well as other bacteria genera that share cross-reactive antigens.Citation6-Citation8 In particular, the importance of N. lactamica in this natural immunity has been investigated.Citation6-Citation8 Particulate vaccines to Neisseria are currently receiving a high level of interest.Citation9 Also, examining the quality of the antibodies that are produced is important to correlate with any protection against Neisseria.Citation10 For example, methods that quantify only total IgG antibody levels have later shown poor correlation with functional antibody activity as measured by serum bactericidal activity (SBA).Citation11 However, high avidity antibodies have been shown to have higher activity in bacteriolysis and passive protection in animal models as serogroup C meningococci.Citation12 Methods to detect high-avidity antibodies have also been developed for meningococcal polysaccharides.Citation13 The avidity of antibodies and the proportions of high-avidity antibodies have shown higher correlation with SBA levels from sera obtained after vaccination with conjugated and unconjugated serogroup C or A polysaccharide vaccines.Citation14
Outer membrane vesicles (OMVs) are vesicles similar to the blebs that are naturally derived from Neisseria during their growth.Citation15 These vesicles have a similar composition as OMPs. However, they are more immunogenic than OMPs.Citation16
Bilayer fragments of the cationic lipid dioctadecyldimethylammonium bromide (DDA-BF) can be obtained by dispersion of the white powder in an aqueous solution at low ionic strength after sonication at a temperature below 60°C. This low ionic strength allows the compound to remain stable due to the electrostatic repulsion of the molecules.Citation17-Citation19 DDA-BF can be used as an adjuvant, and its main advantage is that DDA-BF requires a lipid concentration lower than the concentrations traditionally used in liposomal formulationsCitation20-Citation22 Therefore, DDA-BF typically causes less toxicity. As a cationic molecule, DDA-BF can adsorb molecules with negative charges,Citation23 such as N. lactamica OMVs.This adsorption leads to the production of particles that are effectively taken up by both macrophages and dendritic cells. Particulate antigens, such as OMV-DDA-BF, are natural dendritic cells targets because particles deliver to antigen-presenting cells (APCs) more efficiently than soluble antigens.Citation24,Citation25 Cationic nanoparticles carrying antigen significantly increased both the production of antibodies and cytotoxic T cells with low doses of antigenCitation26 and induced maturation of dendritic cells.Citation27 Aluminum compounds, which were identified as having immunostimulatory properties more than 70 y ago, remain the only adjuvant that is licensed worldwide.Citation28 However, this adjuvant exhibits low colloid stability.Citation23
In the present work, cationic nanoparticles formed by DDA-BF and N. lactamica OMV (OMV-DDA-BF) were tested as an immunogen against N. meningitidis for the first time. The humoral and cellular immune responses induced by stable cationic adjuvant/antigen complexes were evaluated in mice by the determination of the antigen-specific-IgG antibody levels in serum by ELISA and by measuring delayed type hypersensitivity (DTH) reactions from footpad swelling tests. The specificity and avidity of the antigen-specific-IgG antibodies were also evaluated alum which is the standard adjuvant worldwide, was used as a control for the DDA-BF.
Results
Sizing and zeta-potential analysis of OMV/DDA-BF complexes
The sizing and zeta-potentials for the OMV/DDA-BF complexes were determined after a 1 h interaction at 25°C, in 1 mM NaCl. The antigen, OMV of N. lactamica, was slightly negatively charged in water (pH of 6.3), exhibiting a charge of -28 ± 1 mV. DDA-BF was positively charged (33 ± 2 mV), and had a size of 63 ± 1 nm. shows some physical properties of DDA-BF in the presence or absence of N. lactamica OMV. The DDA concentration was fixed at 0.1 mM because the colloidal stability of DDA complexes improves significantly as concentrations above 0.01 mM. At the fixed concentration of DDA-BF, the resulting charge of the complexes depended on the concentrations of the OMV proteins. The formation of complexes of OMV with DDA-BF was evaluated by changes in the mean diameter and zeta-potential of the particles. Upon addition of OMV to the DDA-BF solution, positively charged complexes were formed at low protein concentrations (). However, at the highest protein concentrations, the final charge was negative. Polydispersity was only moderately affected by addition of OMV to DDA-BF ( and ).
Table 2. Immunization of animals with outer membrane vesicles (OMVs) of Neisseria lactamica with different adjuvants
Table 1. Physical properties of DDA, OMV and DDA/OMV interactiona
Figure 1. The size distribution and zeta-potential (ζ) for different dispersions in 1 mM NaCl. One mM DDA-BF dispersion (A) and 0.1 mM DDA-BF dispersions carrying OMV of N. lactamica at concentrations of 5 (C), 25 (D) and 50 μg/mL (E). OMV alone at a concentration of 100 μg/mL is shown in (B). The interaction time for DDA-BF and protein was 1 h. In each subfigure, mean zeta-potential and diameter ± SE are quoted. The figures above the main peak correspond to the mean diameter of this specific peak.
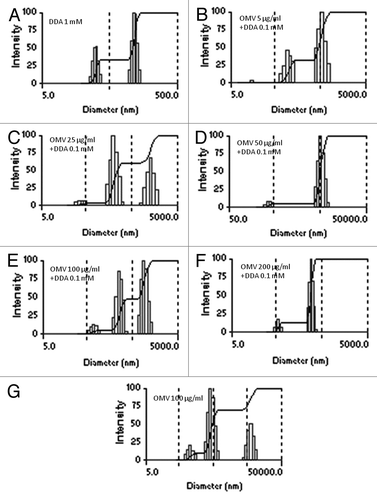
Because DDA-BF is cationic, it has the ability to adsorb negatively charged particles, such as OMV (ζ = -28 ± 1 mV). The interaction of OMV with 0.1 mM DDA was evaluated at the following protein concentrations: 5, 25, 50, 100 and 200 µg/mL. The formation of complexes of OMV with DDA-BF was demonstrated by changes in the mean diameter and zeta-potential following the complex formation. The polidispersion indexes of the complexes were similar to that of DDA-BF alone, except when 50 µg/mL of OMV was added to DDA-BF. This is probably because at this protein concentration, the OMV/DDA-BF complexes loss colloid stability, causing the formation of large complexes (3833 ± 218 nm) ( and ). The colloid stability is associated with small particles that remain stable for long periods. If after 1 h of protein-DDA interaction the complex consists of positively charged nanoparticles, this formulation is considered colloidally stable.Citation23 The final charge of the complex depends on both the protein nature and the concentrations of the protein and DDA-BF. In this study, up to 25 µg/mL of OMV and 0.1 mM of DDA-BF exhibited a positive charge. From 25 µg/mL of OMV, the negative charges of the proteins were predominant, and the final charges of the complexes were negative ().
For the animal immunization experiments, the OMV concentration was chosen based on the dynamic light scattering studies above. The 25 µg/mL concentration was chosen because it was the highest protein concentration that exhibited both a positive charge (8 ± 1 mV) and small complexes (288 ± 7 nm).
Humoral immunity induced by the antigen preparation
For the immunogenicity assays, the animals were immunized according to the scheme shown in . Administration of a single dose of the antigen was compared with two doses, and the results of the DDA-BF used as an adjuvant were compared with alum. In the G1 to G5 groups of mice, only one immunization dose was given to animals. The animals of groups G6 to G8 received two doses that were 15 d apart. The production of antigen-specific-IgG antibodies against OMVs from N. lactamica was tested by ELISA at 15, 30 and 45 d after the first immunization. The results are shown in . To facilitate comparison among the groups, the absorbance values are shown in , which represents the result of ELISA absorbance averages performed with serum collected from animals 45 d after the first immunization, at a dilution of 1/100. The composition of OMV-DDA-BF was statistically superior (p < 0.01) to the OMV-alum or OMV alone when examining the production of total IgG. The OMV-alum complex was not better than OMVs alone. Moreover, administration of two doses of antigen led to the production of total IgG levels significantly higher than those observed with only one immunization. shows that the levels of total IgG increased over time in all groups.
Figure 2. IgG levels in the sera of mice immunized with OMVs from N.lactamica. The serum of 6 animals per group was collected, pooled and analyzed by ELISA: (A) 15 d after immunization; (B) 30 d after immunization; (C) 45 d after immunization; (D) Kinetics of IgG production over time. The composition of OMV-DDA-BF was statistically superior (p < 0.01) to the OMV-alum or OMV alone for the production of total IgG, both with just one dose or two doses of antigens (results represent mean ± SD of the pooled serum of six animals per group).
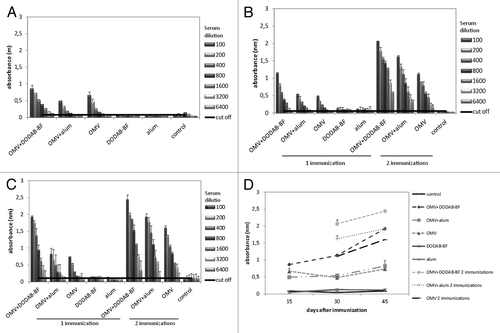
Table 3. Average absorbance values (nm) in ELISA to evaluate specific antibody production against OMVs of Neisseria lactamica in the serum of immunized animals diluted 100 times, 45 d after immunization
As previously shown, the avidity index (AI) of antibodies against meningococcal antigen shows a positive correlation with the serum bactericidal activity. Therefore, AI is a good parameter for evaluating the immune response against meningococci.Citation29 The evaluation of the AI of the serum of the animals was performed 45 d after the first immunization (). Even after only one immunization, the animals immunized with OMV-DDA-BF produced antibodies with intermediate avidity. For OMV-alum, antibodies with intermediate avidity were only found after two rounds of immunization. However, even in animals immunized 2 times with OMV alone, only low avidity antibodies were ever generated ().
Table 4. Avidity index of IgG antibody in the serum of immunized animals, 45 d after the first immunization
The production of IgM against OMV from N. lactamica was also evaluated. However, there was no detectable production of this class of antibodies (data not shown).
Isotypes
We evaluated the production of IgG1, IgG2a and IgG2b in immunized mice, 45 d after the first dose of the antigen. The absorbance for each class of antibodies was subtracted from the mean absorbance of the non-immunized group (). In animals immunized with a single dose, there was a small production of IgG1 and IgG2b only in animals immunized with OMV-DDA-BF. In animals immunized with two doses, the production of these IgG subclasses increased significantly, especially in the groups immunized with OMV-DDA-BF. The production of IgG1 and IgG2a in the OMV-DDA-BF animals was similar to the production of these immunoglobulins in animals immunized with OMV-alum. The main difference observed was in the production of IgG2b, which was markedly higher in animals immunized with OMV-DDA-BF.
Antibody cross-reactivity
Because vaccines based on N. lactamica OMVs aim to protect individuals against meningococcal infection, the cross-reactivity of the antibodies against N. meningitidis was tested by an ELISA and immunoblot. For ELISA, the plates were adsorbed with OMVs from N. meningitidis (). In animals immunized with a single dose of antigens, only those receiving OMV-DDA-BF produced IgG that were cross-reactive with N. meningitidis antigens. All of the animals that were immunized with two doses produced cross-reactive antibodies. However, this production was more pronounced in animals vaccinated with OMV-DDA-BF. The cross reactivity of antibodies was also evaluated by immunoblot. In , it is possible to comparatively analyze the proteins of N. lactamica and N. meningitidis that are recognized by the serum of the animals immunized with N. lactamica OMV. The animals from G1 to G9 were immunized according to the scheme represented in , and the serum from these animals was tested for the recognition of proteins from N. lactamica or N. meningitidis whole cells. Serum from immunized animals specifically recognized a 25 kDa protein of N.lactamica. Interestingly, this antigen was also recognized in the N. meningitidis lysates, and this protein was identified as the “Opa protein..”Citation30 These Opa proteins are responsible for the induction of protective antibodies with bactericidal activity. In this study, an 11 kDa antigen was also recognized in the N. lactamica lysates (NspA).Citation31
Figure 4. Levels of IgG cross reactive with antigens derived from N. meningitidis The serum of 6 animals per group was collected 45 d after the first immunization, pooled and analyzed by ELISA.
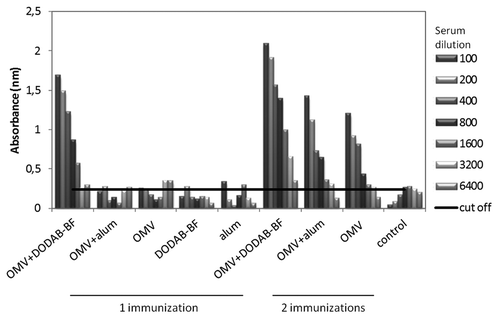
Figure 5. Immunoblot analysis for cross reactivity of the antibodies produced after immunization. Twenty µg of protein of N. lactamica (A) or N. meningitides (B) whole cell lysates were submitted to electrophoresis in a polyacrylamide gel (13% SDS-PAGE). After electrophoresis, the protein fractions were transferred to nitrocellulose membranes. The membranes containing the whole cell lysates were incubated for 1 h in the presence of serum from outbred mice immunized with OMV-DDA-BF single dose (G1), OMV-alum single dose (G2), OMV single dose (G3), DDA-BF (G4), alum (G5), OMV-DDA-BF 2 doses (G6), OMV-alum 2 doses (G7), OMV 2 doses (G8) or non-immunized controls (G9), (diluted 1/500). The immunoreactions were visualized with goat anti-mouse IgG conjugated to peroxidase diluted 1/60,000. The membranes were washed with saline phosphate buffer and luminol.
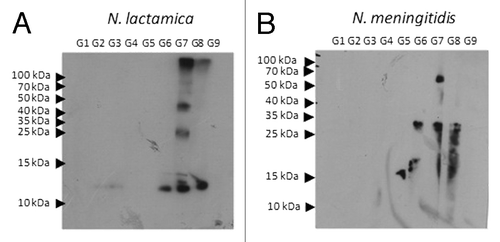
Delayed type hypersensitivity
To evaluate the DTH response, which is an important manifestation of cellular immunity induced by an effective immunization,Citation32 The animals were challenged with 10 µg of OMVs 5 d after the final immunization. For animals in groups G1 to G5, this was after one immunization, and the challenge was 5 d after the second immunization in animals in groups G6 to G8. The G9 group was used as a control for both cases. In animals that received a single immunization, DDA-BF was better than alum adjuvant because the percentage of swelling in the footpads of the animals vaccinated with OMV-DDA-BF was significantly higher (p < 0.01) than that from all other animals vaccinated with a single dose or not vaccinated at all. In animals immunized with OMV-alum, a second dose of immunization was required to achieve results similar to a single dose of OMV-DDA-BF ().
Figure 6. Delayed type hypersensitivity (DTH) reaction. The percentage of swelling of the footpad of mice challenged with 10 µg/mL of outer membrane vesicles of N. meningitidis after 24 h of challenge. The percentage of swelling of the footpads of the animals vaccinated with a single dose of OMV-DDA-BF was significantly higher (p < 0.01) than the swelling of the footpads from all other animals, either vaccinated or not.
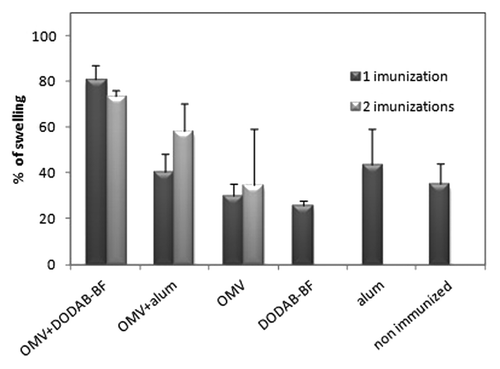
Discussion
To our knowledge, this is the first study showing an immune response induced by DDA-BF and Neisseria genera. These gram-negative bacteria possess an (OM) and (IM). The OM is composed of a lipid bilayer, which in its outer surface contains surface proteins and (LPS).Citation33 In the inner portion of the outer membrane there are phospholipids and lipoproteins that connect the outer membrane to the peptidoglycan. Between the inner and outer membrane, there is a small space known as the periplasmic space.Citation33 (OMVs) are spherical lipid vesicles that have an average diameter between 50 and 250 nm, and they are usually released by the membrane of growing gram-negative cells.Citation34-Citation36 The composition of OMVs is generally similar to the OM, containing phospholipids and proteins from the OM, as well as including periplasmic components.Citation35 These vesicles represent a secretory pathway for bacteria and have important roles in cell growth, the survival of the bacteria, virulence, signaling, the stress response, internalization of nutrients, biofilm formation and horizontal gene transfer. Several pathogenic bacteria can secrete OMVs, including N. meningitidis, N. lactamica, P. aeruginosa, Brucella melitensis and Helicobacter pylori.Citation37
Because OMVs represent the components of the outer membrane of Gram-negative bacteria, these vesicles contain a variety of antigens that can be immunogenic and have been shown to be safe when used as vaccines.Citation38 Furthermore, the antigenic properties of OMVs are stable for several months at 4°C after lyophilization.Citation39 The use of OMVs as a vaccine began with a protein-based vaccine using the OMVs of N meningitidis serogroup B, and this vaccine was successfully used in Cuba, Norway, Brazil and New Zealand.Citation39 In addition, OMVs also have potential to be used as an adjuvant.Citation39 In Norway, Cuba and New Zealand, licensed OMVs vaccines are safe and protective, but they need up to three doses for the vaccinated individuals to reach the appropriate level of protective antibodies.Citation40
Current preparations of OMVs are usually derived from OMVs extracted with detergent, which contain much less LPS than the native OMVs and are therefore safe for intramuscular use in humans.Citation36,Citation38 The protection that is promoted by the OMV-based vaccines for N. meningitidis is due to the bactericidal antibody response.Citation40,Citation41 However, this response appears to be dependent on the cell surface protein PorA,Citation42 which has the disadvantage that PorA is variable in the strains of meningococcus, leading to the need for periodic changes in the vaccine formulation.Citation43
OMVs from N. lactamica have emerged as an alternative to vaccination against meningococcal disease.Citation42 Considering that this species has no capsule nor PorA, OMVs vaccines based on N. lactamica are not serogroup or subtype specific, but they have been shown to protect mice against a lethal challenge in a model of meningitis, even in the absence of a bactericidal antibody response Moreover, this vaccine proved safe in a phase I study with adult volunteers.Citation43,Citation44
In the present study, the immunogenicity of the OMVs of N.lactamica was tested in association with fragments of the lipid bilayer of (DDA-BF) used as adjuvant. In addition, DDA-BF was compared with alum, which had been implicated in the increased immunogenicity of OMV vaccines to N meningitidis B.Citation16 One of the main advantages of using cationic lipid adjuvants is the ability to adsorb negatively charged antigens,Citation23 such as the OMVs, which are negatively charged (ζ = -28 ± 1 mV, ). Furthermore, it is possible that the formation of complexes of antigen with the DDA-BF occurs through the hydrophobic effect because the OMVs contain phospholipids and lipoproteins.Citation35
The interaction of the OMVs with 0.1 mM DDA was evaluated using a range of OMV protein concentrations: 5, 25, 50, 100 and 200 µg/mL. The formation of complexes with DDA-BF was demonstrated by the change in charge and particle size after the addition of OMVs. The polydispersity of the complexes remained similar to that observed with DDA-BF alone, except with the 50 µg/mL concentration of protein. At this dose of protein, the OMV-DDA-BF complex may lose colloid stability, causing the formation of large complexes (). The stability of the antigen/adjuvant complex is associated with a small particle size. Therefore, if after one hour the interaction of the DDA-BF-protein complex is stable, it tends to remain stable for long periods.Citation23 The final charge of the complex depends on both the nature of the adsorbed protein and the concentrations of the protein and DDA-BF.Citation23 The protein concentration of 25 µg/mL was chosen because this was the highest protein concentration supporting the formation of cationic nanocomplexes (8 ± 1 mV) and did not form negatively charged complexes. Large negatively charged complexes could not be internalized by cells that are not professional phagocytes and should be avoided.
Stable colloid complexes between protein and DDA-BF can act similarly to inert nanometer scale beads, which have the ability deliver antigen to antigen presenting cells (APCs). This method of antigen delivery generates strong humoral and cellular responses.Citation45 It is expected that particles smaller than 500 nm are an optimal size for uptake by dendritic cells, which can then elicit an adequate immune response.Citation46,Citation47
It appeared that protein-protein aggregation occurred when OMVs were dispersed in 1 mM NaCl (average diameter 477 ± 41 nm). This aggregation did not occur in the presence of DDA-BF when using small OMV protein concentrations (288 ± 7 nm for 25 µg/mL of protein in 0.1 mM DDA). This result is similar to that observed by Lincopan et al. (2007).Citation48
Animals were immunized with 200 µL of antigen injected into 2 sites (100 µL/site) subcutaneously, which totaled 5 µg of protein/animal. The animals were immunized according to the scheme showed in . One dose of immunization was compared with 2 doses, and DDA-BF was compared with alum as adjuvants. Animals from groups G1 to G5 received a single immunization, while animals from G6 to G8 received 2 doses at a 15-d interval. The production of IgG that were specific against N. lactamica OMV was tested by ELISA at 15, 30 and 45 d after the first immunization. The immunization with OMV-DDA-BF was better than OMV-alum or OMV alone. However, OMV-alum was not better than OMV alone. When comparing the responses of the animals to the 2 different doses, the animals that received 2 doses of immunization exhibited an increase in IgG production.
Because antibody AI for meningococci has been shown to have a linear correlation with SBA, it is a good parameter to evaluate the immune response against meningococcal bacteria.Citation29 AI was evaluated in serum of animals 45 d after the first immunization. Even after a single immunization, animals immunized with OMV-DDA-BF produced antibodies with intermediate avidity. To achieve a similar AI response, 2 doses of OMV-alum immunizations were necessary. The animals immunized with OMV alone produced only low affinity antibodies. IgM production was also examined in these animals, but this immunoglobulin class was not detected (data not shown).
Antibodies that were cross reactive to N.meningitidis were produced more efficiently by the animals that were immunized with OMV-DDA-BF. In addition, immunoblot analysis identified cross reactivity with a protein of approximate 25 kDa in N. meningitidis lysate, which is consistent with the Opa protein.Citation30
Immunization with OMV-DDA-BF was also better able to generate cellular immunity than OMV-alum or OMV alone, because the DTH response to antigen challenge, a good parameter of cellular immunity, was more pronounced in these animals. The immunization with OMV-DDA-BF also led to the production of the highest levels of the IgG2b antibody class. This IgG subclass is an opsonizing immunoglobulin, and it is also related to cellular immunity. This improvement in cellular immunity that is apparently induced by DDA is in accordance with previous work.Citation49
Material and Methods
Bacteria and animals
N. meningitidis was isolated from a patient in Brazil. This isolate is representative of the epidemic strain prevalent in Brazil since 1987.Citation50 Strains of N. lactamica were isolated from the oropharynx of healthy carriers in our community,Citation51 and Neisseria meningitidis was isolated from the spinal fluid or blood at the Bacteriology Section of the Adolfo Lutz Institute. N. lactamica and N. meningitidis strains were cultured on blood agar containing 1% (v/v) horse blood. Strains were maintained at –70°C in a skim milk solution or were lyophilized and stored at 4°C. Strains were grown overnight in candle jars on Tryptic Soy Broth (TSB, Difco) plates supplemented with 1% horse serum (Sigma, St. Louis, MO, USA) in a 5% CO2 atmosphere at 37°C. Female outbred Swiss Webster mice (6-week-old) were obtained from the breeding facilities of the Adolfo Lutz Institute, São Paulo /SP, Brazil. All procedures with the animals were in accordance with the guidelines of the Brazilian Code for the Use of Laboratory Animals.
Isolation of native outer membrane vesicles of N. lactamica and N. meningitidis
Outer membrane vesicles (OMVs) of N. lactamica were used as antigen, and DODAB-BF (Sigma–Aldrich (St Louis, MO, USA). and alum (Merial do Brasil (Merial Ltda.) were compared as adjuvants. OMVs from N. lactamica and N. meningitidis were obtained as previously described.Citation50 Briefly, bacteria from five plates were recovered by centrifugation at 10,000 x g for 10 min at 4°C and resuspended in 0.1 M acetate buffer, pH 5.8 with 0.2 M LiCl (5 mL of buffer was used per gram of cells). The suspension was incubated for 2 h at 45°C in a shaking water bath with 6-mm glass beads at 300 rpm for 2 h at 45°C. The supernatant was collected after centrifugation for 2 h at 4°C and sonicated. The pellet was washed three times and suspended in distilled water. The OMVs were purified using a column of polymixin B (Pierce) as described by the manufacture. The preparation was diluted to a final concentration of 1 mg per mL and stored at –70°C in aliquots. The protein concentration of the OMV preparations was determined by the method described by Bradford.Citation51 The proteins of N. lactamica were adsorbed to the adjuvants, in 1 mM NaCl, as previously described.Citation23
Electrophoretic analysis of proteins
A 13% Sodium dodecyl sulfate (SDS)–polyacrylamide gel was prepared under denaturing conditions.Citation52 Gels were run in 0.25 M tris, 1.92 M glycine, 1% SDS buffer at 100 V/cm2 at room temperature for 1.5 h. Protein bands were detected by staining with Coomassie brilliant blue (Pharmacia LKB, Biotechnology, Piscataway, NJ, USA).
Preparation of lipid dispersions
Colloidally stable DDA bilayer fragments (DDA-BF) with a 63 ± 1 nm mean diameter and 33 ± 2 mV mean zeta potential were prepared by sonication with a titanium macrotip in 1 mM NaCl prepared with Milli-Q water at a final concentration of 2 mM DDA. The preparation was subsequently centrifuged (1 h at 14.000 rpm and 4°C) to remove any titanium particles.Citation53
Preparation and analysis of OMV/DDA-BF complexes
The complexes were always prepared in 1 mM NaCl. DDA-BF was analyzed at a concentration of 1 mM. To study the DDA-BF/OMV interaction, the DDA concentration was fixed in 0.1 mM, and the OMV was added over a range of protein concentrations (5–200 µg/mL). Protein alone was analyzed at a final concentration of 100 µg/mL. Particle size (mean diameter Dz), size distribution, polydispersity, and zeta potential (ζ) of the DDA-BF/OMV complexes were determined using the ZetaPlus-Zeta Potential Analyzer (Brookhaven Instruments Corporation, Holtsville, NY) equipped with a 677 nm laser and dynamic light-scattering (PCS) at 90° for measuring sizes.Citation54
Experimental design and determination of delayed type hypersensitivity
Nine different groups of mice were immunized as described in . Immunization with OMV/DODAB-BF was compared with OMV/alum and OMV alone. In addition, a single immunization was compared with 2 doses. DDA-BF and alum alone were used as controls. Immunization was performed subcutaneously in the abdomen of the animals at 2 separate sites, with 0.1 mL/site. This totaled 0.2 mL and 5 µg of protein per animal. The animals were evaluated for cellular immunity 5 d after the final immunization. The swelling in the footpads of the animals was evaluated as previously described, to observe the reaction of delayed type hypersensitivity (DTH) promoted by antigen.Citation23 On the fifth day after immunization, each animal received a challenge dose of OMV containing 10 µg of protein in their left hind footpad. The swelling was measured 24 h later using a caliper, and the percentage of swelling was calculated in relation to the contralateral footpad. Five animals per group received the challenge. The results were expressed as the mean ± standard deviation.
ELISA
The enzyme linked immunosorbent assay (ELISA) is briefly described here. The polystyrene ELISA plates (MaxiSorb ® - Nunc) were adsorbed with 0.1 mg of antigen/well, using either OMV of N. lactamica or N. meningitidis (to determine cross reactivity), for 18 h in carbonate bicarbonate buffer at 4°C. The next day, the plates were washed 4 times with PBS-Tween (0.05%), and each well was blocked with 200 µL of PBS-Tween-nonfat milk (5%). The plates were again washed 4 times and then incubated with 100 µL of serum diluted in PBS-Tween-nonfat milk (2.5%) at the following range of dilutions: 1/100, 1/200, 1/400, 1/800, 1/1,600, 1/3,200 and 1/6,400. The plates were washed 4 times again and incubated with 100 µL of conjugated antibody (anti-mouse IgG - peroxidase-conjugated Heavy chain - Sigma ® diluted 1/60,000 and anti-IgG1, 2a, 2b and IgM Kirkegaard and Perry, Gaithersburg, MD at a dilution of 1/1000) in PBS-Tween non-fat milk 2.5%. The plates were washed again 4 times and incubated for 15 min with 100 µL of citrate-phosphate buffer containing 1 mg/mL of OPD (o-Phenylenediamine dihydrochloride - Sigma ®) and 1 µL/mL of H2O2 (Sigma ®). The reaction was stopped with 50 µL of 4N H2SO4. The colorimetric reaction was measured in an ELISA reader at 492 nm. All incubations were performed at 37°C. Controls were made with non-immunized animals and animals immunized with the antigen or adjuvants alone. Serum from 5 animals per group were collected and pooled at 15, 30 and 45 d after the first immunization. The samples were analyzed in duplicate. The results were expressed as the mean ± standard deviation. Antibody endpoint titers were defined as the highest dilution of samples giving an optical density (OD) 2-fold greater than that of the negative controls.
Avidity index
To assess the avidity index (AI) of the IgG antibodies, ELISA plates were sensitized with 100 µL of OMVs from N. lactamica for 18 h at 4°C. Then, the plates were washed with PBS-Tween 0.05%, and the active sites on the plates were blocked with 200 µL of 5% non-fat milk in PBS-Tween for 2 h at 37°C. The plates were washed again 4 times with PBS-Tween. Next, 4 dilutions of the samples were prepared (2 fold dilution starting from 1/100), and 100 µL of diluted serum was incubated in the plates at 37°C for 2 h. The plates were washed again, and half of the samples were incubated with potassium thiocyanate (KSCN) (a chaotropic agent in the reaction) for 30 min. The other half of the samples served as controls. After this step, the plates were washed four times and incubated with anti-IgG conjugated to peroxidase at a dilution 1/60.000 (Sigma ®) for 1 h at 37°C. Then, the plates were washed and incubated with 100 µL of chromogen solution [phosphate-citrate buffer containing 1 µg/mL of OPD (o-Phenylenediamine dihydrochloride - Sigma ®) and 1 µL of H2O2 (Sigma ®)] for 15 min. The reaction was stopped with 50 µL of 1N H2SO4 and evaluated in an ELISA reader at 492 nm. The avidity index was expressed as the ratio of the OD in the presence of KSCN to the OD of the control well. Controls were made with non-immunized animals. The results were interpreted as follows: Avidity index (AI) above 0.5 = high avidity; AI between 0.3 and 0.49 = intermediate avidity; AI below 0.29 = low avidity.
Immunoblotting
The technique was performed as described by TowbinCitation55 with some modifications. This technique was used to demonstrate the reactivity of the serum of animals immunized with OMVs from N. lactamica to antigens from N. lactamica and N meningitidis (to demonstrate cross-reactivity). For this purpose, gels were run with whole cells lysates of N. lactamica or N. meningitidis (20 µg of protein/well). The proteins were transferred to a nitrocellulose membrane, which was cut and each strip was incubated with serum samples from immunized animals. Serum of animals of all 9 groups was incubated with the membranes at a dilution of 1/500 for 1 h (see immunization groups in the ). Secondary antibodies used were anti-mouse IgG (anti-mouse IgG - peroxidase-conjugated Heavy chain - Sigma ®) at a concentration of 1/60.000, for 1 h. The reaction was revealed using luminol (ECL ™ Western Blotting Detection Reagents - GE Healthcare ®), and the membrane was exposed onto photographic film (Amersham Hyperfilm ECL™ - GE Healthcare®).
Statistical analysis
The significance of the results was evaluated by ANOVA. P values were considered significant when p ≤ 0.05.
Conclusion
In conclusion, OMVs of N. lactamica were effective in generating cross-reactive IgG antibodies to N. meningitidis B. Interestingly, DDA-BF was superior to alum as an adjuvant for subcutaneous immunization with OMVs, both with measures of humoral and cellular immunity. Future work based on this study intends to examine any protective effects offered by the combination of OMV-DDA-BF and bactericidal activity.
Abbreviations: | ||
N.lactamica | = | Neisseria lactamica |
N.meningitidis | = | Neisseria meningitidis |
OMV | = | outer membrane vesicles |
DDA-BF | = | dioctadecyldimethylammonium bromide bilayer fragments |
DTH | = | delayed type hypersensitivity |
alum | = | aluminum hydroxide |
OMPs | = | outer membrane proteins |
OM | = | outer membrane |
IM | = | inner membrane |
LPS | = | lipopolysaccharides |
Acknowledgments
Financial support from FAPESP and CNPq is gratefully acknowledged. E.B.G. was the recipient of a posdoctoral FAPESP fellowship. A.S.R. was the recipient of a technical fellowship from FAPESP and E.N.G a fellowship from CNPq 303973/2011–0. N.L. is a research fellow at CNPq. We thank Prof. Iolanda Cuccovia in the Department of Biochemstry at the Univeristy of São Paulo, for his help in zeta potential and particle size analysis measurements.
Submitted
10/06/12
Accepted
10/23/12
Disclosure of Potential Conflicts of Interest
No potential conflicts of interest were disclosed.
References
- WHO. Meningococcal vaccines: WHO position paper, November 2011. Wkly Epidemiol Rec 2011; 86:521 - 39; PMID: 22128384
- Evans CM, Pratt CB, Matheson M, Vaughan TE, Findlow J, Borrow R, et al. Nasopharyngeal colonization by Neisseria lactamica and induction of protective immunity against Neisseria meningitidis.. Clin Infect Dis 2011; 52:70 - 7; http://dx.doi.org/10.1093/cid/ciq065; PMID: 21148522
- Urwin R, Russell JE, Thompson EA, Holmes EC, Feavers IM, Maiden MC. Distribution of surface protein variants among hyperinvasive meningococci: implications for vaccine design. Infect Immun 2004; 72:5955 - 62; http://dx.doi.org/10.1128/IAI.72.10.5955-5962.2004; PMID: 15385499
- de Moraes JC, Perkins BA, Camargo MC, Hidalgo NT, Barbosa HA, Sacchi CT, et al. Protective efficacy of a serogroup B meningococcal vaccine in Sao Paulo, Brazil. Lancet 1992; 340:1074 - 8; http://dx.doi.org/10.1016/0140-6736(92)93086-3; PMID: 1357461
- Keiser PB, Gibbs BT, Coster TS, Moran EE, Stoddard MB, Labrie JE 3rd, et al. A phase 1 study of a group B meningococcal native outer membrane vesicle vaccine made from a strain with deleted lpxL2 and synX and stable expression of opcA. Vaccine 2010; 28:6970 - 6; http://dx.doi.org/10.1016/j.vaccine.2010.08.048; PMID: 20732470
- Troncoso G, Sánchez S, Criado MT, Ferreirós CM. Analysis of Neisseria lactamica antigens putatively implicated in acquisition of natural immunity to Neisseria meningitidis.. FEMS Immunol Med Microbiol 2002; 34:9 - 15; http://dx.doi.org/10.1111/j.1574-695X.2002.tb00597.x; PMID: 12208601
- Finney M, Vaughan T, Taylor S, Hudson MJ, Pratt C, Wheeler JX, et al. Characterization of the key antigenic components and pre-clinical immune responses to a meningococcal disease vaccine based on Neisseria lactamica outer membrane vesicles. Hum Vaccin 2008; 4:23 - 30; http://dx.doi.org/10.4161/hv.4.1.4806; PMID: 17921703
- Tunes CF, Ferraz AS, Scola MCG, De Gaspari EN. Intranasal Delivery of Whole Cells of Neisseria Species: Study of Cross - Reactive Antigens in Rabbits. The Open Vaccine Journal 2008; 1:13 - 21; http://dx.doi.org/10.2174/1875035400801010013
- Santolaya ME, O’Ryan ML, Valenzuela MT, Prado V, Vergara R, Muñoz A, et al, V72P10 Meningococcal B Adolescent Vaccine Study group. Immunogenicity and tolerability of a multicomponent meningococcal serogroup B (4CMenB) vaccine in healthy adolescents in Chile: a phase 2b/3 randomised, observer-blind, placebo-controlled study. [Epub ahead of print] Lancet 2012; 379:617 - 24; http://dx.doi.org/10.1016/S0140-6736(11)61713-3; PMID: 22260988
- McVernon J, Nolan T, Richmond P, Reynolds G, Nissen M, Lambert SB, et al. A randomized trial to assess safety and immunogenicity of alternative formulations of a quadrivalent meningococcal (A, C, Y, and W-135) tetanus protein conjugate vaccine in toddlers. Pediatr Infect Dis J 2012; 31:e15 - 23; http://dx.doi.org/10.1097/INF.0b013e31823e1e34; PMID: 22094636
- Wedege E, Bolstad K, Aase A, Herstad TK, McCallum L, Rosenqvist E, et al. Functional and specific antibody responses in adult volunteers in new zealand who were given one of two different meningococcal serogroup B outer membrane vesicle vaccines. Clin Vaccine Immunol 2007; 14:830 - 8; http://dx.doi.org/10.1128/CVI.00039-07; PMID: 17494638
- Welsch JA, Granoff D. Naturally acquired passive protective activity against Neisseria meningitidis Group C in the absence of serum bactericidal activity. Infect Immun 2004; 72:5903 - 9; http://dx.doi.org/10.1128/IAI.72.10.5903-5909.2004; PMID: 15385492
- Vermont CL, van Dijken HH, van Limpt CJ, de Groot R, van Alphen L, van Den Dobbelsteen GP. Antibody avidity and immunoglobulin G isotype distribution following immunization with a monovalent meningococcal B outer membrane vesicle vaccine. Infect Immun 2002; 70:584 - 90; http://dx.doi.org/10.1128/IAI.70.2.584-590.2002; PMID: 11796586
- Harris SL, Finn A, Granoff DM. Disparity in functional activity between serum anticapsular antibodies induced in adults by immunization with an investigational group A and C Neisseria meningitidis-diphtheria toxoid conjugate vaccine and by a polysaccharide vaccine. Infect Immun 2003; 71:3402 - 8; http://dx.doi.org/10.1128/IAI.71.6.3402-3408.2003; PMID: 12761124
- Devoe IW, Gilchrist JE. Release of endotoxin in the form of cell wall blebs during in vitro growth of Neisseria meningitidis. J Exp Med 1973; 138:1156 - 67; http://dx.doi.org/10.1084/jem.138.5.1156; PMID: 4200775
- Wang LY, Frasch CE. Development of a Neisseria meningitidis group B serotype 2b protein vaccine and evaluation in a mouse model. Infect Immun 1984; 46:408 - 14; PMID: 6437983
- Carmona-Ribeiro AM. Bilayer vesicles and liposomes as interface agents. Chem Soc Ver 2001; 30:241 - 7
- Carmona-Ribeiro AM, Castuma CE, Sesso A, Schreier S. Bilayer structure and stability in dihexadecyl phosphate dispersions. J Phys Chem 1991; 95:5361 - 6; http://dx.doi.org/10.1021/j100166a080
- Kano K, Romero A, Djermouni B, Ache H, Fendler JH. Characterization of surfactant vesicle as a membrane mimetic agent. 2. temperature-dependant changes of turbidity, viscosity, fluorescence polarization of 2 methylantracene, and positron annihilation in sonicated dioctadecyldimethylammoniun chloride. J Am Chem Soc 1979; 101:4030 - 7; http://dx.doi.org/10.1021/ja00509a002
- Davidsen J, Rosenkrands I, Christensen D, Vangala A, Kirby D, Perrie Y, et al. Characterization of cationic liposomes based on dimethyldioctadecylammonium and synthetic cord factor from M. tuberculosis (trehalose 6,6′-dibehenate)-a novel adjuvant inducing both strong CMI and antibody responses. Biochim Biophys Acta 2005; 1718:22 - 31; http://dx.doi.org/10.1016/j.bbamem.2005.10.011; PMID: 16321607
- Vangala A, Kirby D, Rosenkrands I, Agger EM, Andersen P, Perrie Y. A comparative study of cationic liposome and niosome-based adjuvant systems for protein subunit vaccines: characterisation, environmental scanning electron microscopy and immunisation studies in mice. J Pharm Pharmacol 2006; 58:787 - 99; http://dx.doi.org/10.1211/jpp.58.6.0009; PMID: 16734980
- Korsholm KS, Agger EM, Foged C, Christensen D, Dietrich J, Andersen CS, et al. The adjuvant mechanism of cationic dimethyldioctadecylammonium liposomes. Immunology 2007; 121:216 - 26; http://dx.doi.org/10.1111/j.1365-2567.2007.02560.x; PMID: 17302734
- Lincopan N, Espíndola NM, Vaz AJ, da Costa MH, Faquim-Mauro E, Carmona-Ribeiro AM. Novel immunoadjuvants based on cationic lipid: Preparation, characterization and activity in vivo. Vaccine 2009; 27:5760 - 71; http://dx.doi.org/10.1016/j.vaccine.2009.07.066; PMID: 19664738
- Kovacsovics-Bankowski M, Clark K, Benacerraf B, Rock KL. Efficient major histocompatibility complex class I presentation of exogenous antigen upon phagocytosis by macrophages. Proc Natl Acad Sci U S A 1993; 90:4942 - 6; http://dx.doi.org/10.1073/pnas.90.11.4942; PMID: 8506338
- Vidard L, Kovacsovics-Bankowski M, Kraeft SK, Chen LB, Benacerraf B, Rock KL. Analysis of MHC class II presentation of particulate antigens of B lymphocytes. J Immunol 1996; 156:2809 - 18; PMID: 8609400
- Singh M, Briones M, Ott G, O’Hagan D. Cationic microparticles: A potent delivery system for DNA vaccines. Proc Natl Acad Sci U S A 2000; 97:811 - 6; http://dx.doi.org/10.1073/pnas.97.2.811; PMID: 10639162
- Thiele L, Rothen-Rutishauser B, Jilek S, Wunderli-Allenspach H, Merkle HP, Walter E. Evaluation of particle uptake in human blood monocyte-derived cells in vitro. Does phagocytosis activity of dendritic cells measure up with macrophages?. J Control Release 2001; 76:59 - 71; http://dx.doi.org/10.1016/S0168-3659(01)00412-6; PMID: 11532313
- Pérez O, Batista-Duharte A, González E, Zayas C, Balboa J, Cuello M, et al. Human prophylactic vaccine adjuvants and their determinant role in new vaccine formulations. Braz J Med Biol Res 2012; 45:681 - 92; http://dx.doi.org/10.1590/S0100-879X2012007500067; PMID: 22527130
- Granoff DM, Maslanka SE, Carlone GM, Plikaytis BD, Santos GF, Mokatrin A, et al. A modified enzyme-linked immunosorbent assay for measurement of antibody responses to meningococcal C polysaccharide that correlate with bactericidal responses. Clin Diagn Lab Immunol 1998; 5:479 - 85; PMID: 9665952
- De Gaspari E, Zollinger W. Expression of class 5 antigens by meningococcal strains obtained from patients in Brazil and evaluation of two new monoclonal antibodies. Braz J Infect Dis 2001; 5:143 - 53; http://dx.doi.org/10.1590/S1413-86702001000300007; PMID: 11506778
- Martin D, Cadieux N, Hamel J, Brodeur BR. Highly conserved Neisseria meningitidis surface protein confers protection against experimental infection. J Exp Med 1997; 185:1173 - 83; http://dx.doi.org/10.1084/jem.185.7.1173; PMID: 9104804
- Hilgers LA, Snippe H. DDA as an immunological adjuvant. Res Immunol 1992; 143:494 - 503, discussion 574-6; http://dx.doi.org/10.1016/0923-2494(92)80060-X; PMID: 1439129
- Beveridge TJ. Structures of gram-negative cell walls and their derived membrane vesicles. J Bacteriol 1999; 181:4725 - 33; PMID: 10438737
- Mayrand D, Grenier D. Biological activities of outer membrane vesicles. Can J Microbiol 1989; 35:607 - 13; http://dx.doi.org/10.1139/m89-097; PMID: 2670152
- Mashburn-Warren L, McLean RJ, Whiteley M. Gram-negative outer membrane vesicles: beyond the cell surface. Geobiology 2008; 6:214 - 9; http://dx.doi.org/10.1111/j.1472-4669.2008.00157.x; PMID: 18459967
- Collins BS. Gram-negative outer membrane vesicles in vaccine development. Discov Med 2011; 12:7 - 15; PMID: 21794204
- Lee EY, Choi DS, Kim KP, Gho YS. Proteomics in gram-negative bacterial outer membrane vesicles. Mass Spectrom Rev 2008; 27:535 - 55; http://dx.doi.org/10.1002/mas.20175; PMID: 18421767
- Rosenqvist E, Høiby EA, Bjune G, Aase A, Halstensen A, Lehmann AK, et al. Effect of aluminium hydroxide and meningococcal serogroup C capsular polysaccharide on the immunogenicity and reactogenicity of a group B Neisseria meningitidis outer membrane vesicle vaccine. Dev Biol Stand 1998; 92:323 - 33; PMID: 9554288
- Danzig L. Meningococcal vaccines. Pediatr Infect Dis J 2004; 23:Suppl S285 - 92; PMID: 15597071
- Holst J, Feiring B, Fuglesang JE, Høiby EA, Nøkleby H, Aaberge IS, et al. Serum bactericidal activity correlates with the vaccine efficacy of outer membrane vesicle vaccines against Neisseria meningitidis serogroup B disease. Vaccine 2003; 21:734 - 7; http://dx.doi.org/10.1016/S0264-410X(02)00591-1; PMID: 12531351
- Tappero JW, Lagos R, Ballesteros AM, Plikaytis B, Williams D, Dykes J, et al. Immunogenicity of 2 serogroup B outer-membrane protein meningococcal vaccines: a randomized controlled trial in Chile. JAMA 1999; 281:1520 - 7; http://dx.doi.org/10.1001/jama.281.16.1520; PMID: 10227322
- Gorringe AR, Taylor S, Brookes C, Matheson M, Finney M, Kerr M, et al. Phase I safety and immunogenicity study of a candidate meningococcal disease vaccine based on Neisseria lactamica outer membrane vesicles. Clin Vaccine Immunol 2009; 16:1113 - 20; http://dx.doi.org/10.1128/CVI.00118-09; PMID: 19553555
- Oliver KJ, Reddin KM, Bracegirdle P, Hudson MJ, Borrow R, Feavers IM, et al. Neisseria lactamica protects against experimental meningococcal infection. Infect Immun 2002; 70:3621 - 6; http://dx.doi.org/10.1128/IAI.70.7.3621-3626.2002; PMID: 12065503
- Gorringe AR. Can Neisseria lactamica antigens provide an effective vaccine to prevent meningococcal disease?. Expert Rev Vaccines 2005; 4:373 - 9; http://dx.doi.org/10.1586/14760584.4.3.373; PMID: 16026250
- Caputo A, Sparnacci K, Ensoli B, Tondelli L. Functional polymeric nano/microparticles for surface adsorption and delivery of protein and DNA vaccines. Curr Drug Deliv 2008; 5:230 - 42; http://dx.doi.org/10.2174/156720108785914961; PMID: 18855591
- Fifis T, Gamvrellis A, Crimeen-Irwin B, Pietersz GA, Li J, Mottram PL, et al. Size-dependent immunogenicity: therapeutic and protective properties of nano-vaccines against tumors. J Immunol 2004; 173:3148 - 54; PMID: 15322175
- Xiang SD, Scholzen A, Minigo G, David C, Apostolopoulos V, Mottram PL, et al. Pathogen recognition and development of particulate vaccines: does size matter?. Methods 2006; 40:1 - 9; http://dx.doi.org/10.1016/j.ymeth.2006.05.016; PMID: 16997708
- Lincopan N, Espíndola NM, Vaz AJ, Carmona-Ribeiro AM. Cationic supported lipid bilayers for antigen presentation. Int J Pharm 2007; 340:216 - 22; http://dx.doi.org/10.1016/j.ijpharm.2007.03.014; PMID: 17452086
- Hilgers LA, Snippe H. DDA as an immunological adjuvant. Res Immunol 1992; 143:494 - 503, discussion 574-6; http://dx.doi.org/10.1016/0923-2494(92)80060-X; PMID: 1439129
- Ito AY, Néri S, Machado MS, Tunes CF, De Gaspari EN. Homologous prime-boost strategy in neonate mice using Neisseria lactamica.. Vaccine 2009; 27:3422 - 8; http://dx.doi.org/10.1016/j.vaccine.2009.01.114; PMID: 19460600
- Bradford MM. A rapid and sensitive method for the quantitation of microgram quantities of protein utilizing the principle of protein-dye binding. Anal Biochem 1976; 72:248 - 54; http://dx.doi.org/10.1016/0003-2697(76)90527-3; PMID: 942051
- Laemmli UK. Cleavage of structural proteins during the assembly of the head of bacteriophage T4. Nature 1970; 227:680 - 5; http://dx.doi.org/10.1038/227680a0; PMID: 5432063
- Schales O, Schales SS. A simple and accurate method for the determination of chloride in biological fluids. J Biol Chem 1941; 140:879 - 84
- Grabowski E, Morrison I. Particle size distribution from analysis of quasi-elastic light scattering data. In: Dahneke, B. Measurements of Suspended Particles by Quasi-Elastic Light Scattering, New York: Wiley-Interscience; 1983, p. 199-236.
- Towbin H, Staehelin T, Gordon J. Electrophoretic transfer of proteins from polyacrylamide gels to nitrocellulose sheets: procedure and some applications. Proc Natl Acad Sci U S A 1979; 76:4350 - 4; http://dx.doi.org/10.1073/pnas.76.9.4350; PMID: 388439