Abstract
Previous studies using rodent respiratory infection models of nontypeable Haemophilus influenzae (NTHi) infection have established the 26-kDa outer membrane protein of the bacterium, OMP26, as a potential vaccine antigen for NTHi. This study undertook a comprehensive immunological identification of OMP26 T- and B-cell epitopes. A series of OMP26 peptides were constructed and regions of the OMP26 antigen involved in recognition by lymphocyte receptors and induction of acquired immune responses were identified. The dominant T-cell epitopes for OMP26 were located toward the C-terminus between amino acid residues 95 and 197 (T3+T4 region) as mapped using antigen-specific lymphocyte proliferation assays. The newly identified T-cell epitopes exhibited strong capacity for efficient T-cell activation, suggesting that, compared with other OMP26 regions; epitopes within the T3+T4 region have the highest affinity for binding to major histocompatibility complex molecules. In contrast, the predominant B-cell epitopes of OMP26 were located more centrally within the molecule between amino acid residues 45 and 145 (T2+T3 region) as determined using enzyme-linked immunosorbent assay and surface plasmon resonance assays. The T2+T3 region was immunodominant in several species including chinchilla, mice and rats when assessed using both mucosal and parenteral immunization regimes. In addition, the antibodies directed against the T2+T3 region bound to intact NTHi cell surface, according to flow cytometry. Collectively, these results specifically locate the amino acid sequences containing the OMP26 T- and B-cell epitopes, which, as newly mapped antigenic epitopes for lymphocyte recognition, will be useful to improve existing NTHi vaccine strategies. Comprehensive definition of the minimum epitope length required for optimal B- and T-cell responses requires further study.
Introduction
Nontypeable Haemophilus influenzae (NTHi) is a significant human pathogen causing a wide range of respiratory infections. Several outer membrane proteins (OMPs) of NTHi and its oligosaccharides have been investigated as possible vaccine antigens against NTHi.Citation1-Citation15 One OMP that has shown promise as a potential vaccine candidate is OMP26. The amino acid sequence of this 26kDa OMPCitation16 is conserved among NTHi isolates from various disease states.Citation17
Our laboratory has previously shown that immunization with OMP26 can stimulate enhanced pulmonary clearance of NTHi in a rat model in which animals were initially immunized via intra-Peyer’s patches followed by intra-tracheal boost (IPP/IT).Citation16,Citation17 Mucosal immunization with OMP26 protected animals against subsequent pulmonary challenge with both homologous and heterologous strains of NTHi and induced high levels of OMP26-specific IgA and IgG antibodies.Citation16 Furthermore, parenteral immunization of chinchillas with OMP26 demonstrated good immunogenicity and enhanced the clearance of NTHi from the nasopharynx.Citation18 Thus, OMP26 is appealing as an immunogen against NTHi and has demonstrated potential as a candidate vaccine antigen for this pathogen.
A high degree of antigenic heterogenicity between NTHi strainsCitation19-Citation22 has led to vaccine approaches based on peptide formulations of immunodominant epitopes of the native protein.Citation7 In one study, T-cell epitopes were included in a peptide-based approach to maximize induction of antibodies with higher affinity for the incorporated B-cell epitopes.Citation23 This approach offers an additional advantage of accommodating multiple epitopes to cover a broader range of antigenically-distinct NTHi strains.
OMP26 is highly conserved among a large number of clinical NTHi isolates collected from a range of anatomical sites.Citation17 Typically, vaccine formulations do not favor the use of a single protein, however, a highly conserved protein such as OMP26 may provide the necessary broad-based protection against geographically-diverse and antigenically-distinct isolates of NTHi.
This study assessed epitope specificity of the immune responses to OMP26 by mapping the location of T- and B-cell epitopes within the protein to further characterize the immune response to OMP26. These results reveal unique T- and B-cell-targeting regions within OMP26 that will aid in the development of improved peptide-based vaccine strategies for NTHi.
Results
Lymphoproliferative responses to OMP26 peptides
Lymphoproliferative response studies were conducted using splenocytes derived from rats and mice. Unfortunately, background cross reactivity against E. coli proteins within the mouse samples masked any specific responses and thus only rat data are presented. To localize the immunologically important regions within OMP26 in this response, a series of overlapping OMP26 peptides spanning the entire sequence of full-length OMP26 was used as the in vitro proliferation stimulus. Proliferation in response to Concanavalin A ranged from 85,000 to 110,000 counts per minute (CPM).
At a concentration of 1 µg/ml the OMP26 peptides stimulated little or no response from OMP26-primed lymphocytes with the exception of T3+T4 peptide and the whole OMP26 molecule itself where significant stimulation was observed (p < 0.001) (data not shown). In contrast, compared with naïve lymphocytes, a peptide concentration of 10 µg/ml stimulated significant increases in lymphocyte proliferation () in response to the T4 peptide (p < 0.02; amino acid residues 140–197), T2+T3 peptide (p < 0.05; amino acid residues 45–145) and T3+T4 peptide (p < 0.001; amino acid residues 95–197). Lymphocyte proliferation stimulated by the individual peptides, T1 (amino acid residues 1–55), T2 (amino acid residues 45–100) or T3 (amino acid residues 95–145) and the T1a+T2 peptide (amino acid residues 24–100), did not differ significantly between the immunized vs. non-immunized group. These data suggested that in terms of lymphocyte proliferation, peptides that represented the C-terminus of OMP26 were more stimulatory than peptides that represented the N-terminus of the protein.
Figure 1. Antigen-specific proliferative responses to T1, T2, T3, T4, T1a+T2, T2+T3 and T3+T4 OMP26 peptides from OMP26-primed and naïve DA rats. Splenic lymphocytes from immunized and non-immunized rats were cultured with individual OMP26 peptides or Concanavalin A (positive control) at concentrations of 10 μg/ml and 1 μg/ml for 3 d. Proliferative responses to peptides at 10 μg/ml are shown and values presented are expressed as mean counts per minute (CPM) ± standard deviation. Background lymphoproliferative responses to the negative E. coli protein control have been subtracted. Significance shown as ***p < 0.001, ** p < 0.02, * p < 0.05 compared with naïve lymphocytes stimulated with the same peptide on logCitation10 transformed data.
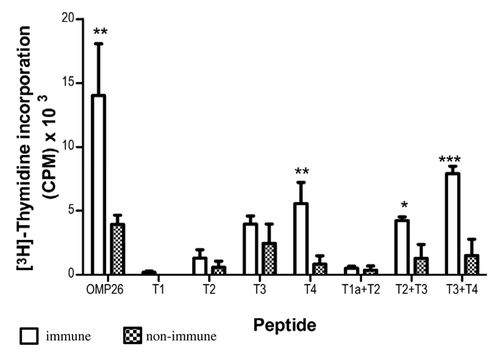
Lymphoproliferative responses to OMP26 protein
The capacity of OMP26 peptides to prime T cells and induce lymphoproliferative responses against OMP26 protein was examined using rat lymphocytes obtained after immunization with OMP26 peptides. Lymphocytes from immune and non-immune rats were stimulated with whole OMP26 protein (10 µg/ml). Only lymphocytes from animals immunized with the T3+T4 peptide demonstrated a significant proliferative response to OMP26 protein stimulation (), further implicating the C-terminus (AA 95–197) of OMP26 as a region of immunologic importance.
Figure 2. Lymphoproliferative responses induced by OMP26 protein by rat lymphocytes obtained after immunization with OMP26 peptides. Lymphocytes from immune and non-immune rats were stimulated with whole OMP26 protein (10 μg/ml). Background lymphoproliferative responses to the negative E. coli control have been subtracted. Only lymphocytes from animals immunized with the T3+T4 peptide demonstrated a significant response to OMP26 protein stimulation compared with non-immunized rats (**p < 0.02). The OMP26 control showed a significant response (***p < 0.001). Values presented are expressed as mean counts per minute (CPM) ± standard deviation. NI = non immune.
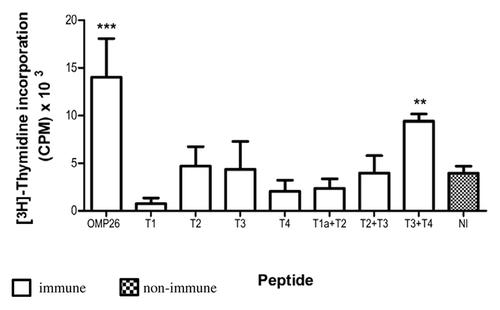
Immunogenicity of OMP26 peptides
Immunogenicity of each of the individual peptides was confirmed by immunization of the rats (IPP/IT) with the individual peptides and subsequent analysis of the anti-OMP26 sera using both enzyme-linked immunosorbent assay (ELISA) and surface plasmon resonance (SPR). All antipeptide sera were reactive with their individual immunizing peptides, in addition to being reactive to the whole OMP26 protein, with the exception of the anti-T1 sera (). These sera were then used to determine whether the peptides were surface exposed on NTHi whole cells by flow cytometry.
Table 1. Antibody reactivity of rat anti-OMP26 peptide against OMP26 protein and peptides
Determination of surface-exposed regions of OMP26
Antibodies to the 50-mer peptides (T1, T2, T3, T4) all showed distinct and reproducible shifts of the curve to the right () which indicated binding of antibody to native structure on the bacterium. In addition, antisera to the 100-mer peptide T1a+T2 also showed a distinct shift to the right. In comparison, antisera to the 100 mer T2+T3 peptide demonstrated a lesser but consistent shift to the right while antisera against the T3+T4 peptide gave results similar to the non-immune serum.
Figure 3. Binding of anti-OMP26 peptide sera to intact NTHi-289 cells. The x axes represent the levels of fluorescence and the y axes represent the number of cells counted. Intact bacteria were incubated with a 1:50 dilution of antisera, stained with Alexa Fluor® 488 goat anti-rat IgG and analyzed for intensity of green fluorescence by Flow Cytometry. The gray areas represent fluorescence in the absence of antibody. Blue lines represent the negative control of bacterial cells incubated with non-immune sera. The results with each immune sera are indicated in red. The total population analyzed was 8 × 104 bacteria.
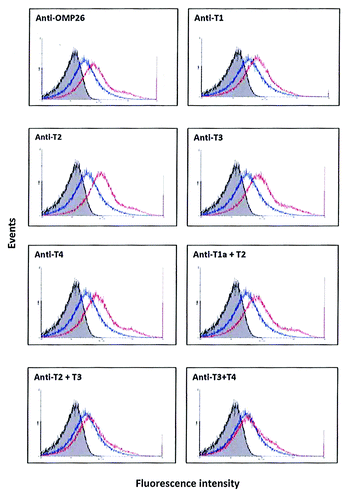
Antibody responses assessed by ELISA and SPR analysis
Reactivity of mouse anti-OMP26 antibodies to OMP26 peptides
B-cell epitope regions of OMP26 were examined in mice using pooled anti-OMP26 sera. The antibody responses against the OMP26 protein and the OMP26 peptides in the mouse were measured using ELISA and SPR. For the ELISA results, animals were immunized either; IPP/IT ( and C) or intra-peritoneal (IP) alone ( and D). SPR assays to determine the antigen/antibody binding in real time are also shown for the IPP/IT and IP immunized groups ( and D, respectively).
Figure 4. Antibody reactivities to the OMP26 peptides of sera from mice immunized with OMP26 protein using the IPP/IT (Panel A, C) and IP (Panel B, D) immunization regimens and assayed by ELISA (Panel A, B) and SPR (Panel C, D). Immune and non-immune sera collected from 3–5 mice were pooled and assayed in duplicate for antibody binding against the whole OMP26 protein and peptides. Antibody reactivities are expressed as IgG concentration in μg/ml in ELISA and Resonance Units (RU) in SPR. No specific antibodies to OMP26 peptides were detected in non-immune sera at the lowest sample dilution (1:25) by ELISA.
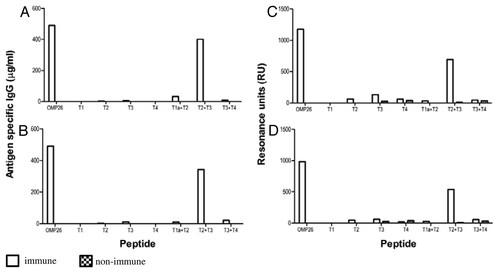
In mice, the predominant reactivity measured by ELISA was seen against the T2+T3 peptide, regardless of immunization regime ( and B). Minor reactivity was also observed against the T1a+T2 peptide in the IPP/IT immunized group (), while in the IP immunized group, low level reactivity was also observed in the T3 and T3+T4 peptides ().
Similarly, SPR analyses showed predominant OMP26 reactivity against the T2+T3 peptide regardless of immunization regime ( and D). In both the IPP/IT and IP immunization groups, low levels of reactivity were also observed against the T2, T3, T4, T1a+T2 and T3+T4 peptides, while no reactivity was observed for the T1 peptide. These data indicated that immunization of mice with OMP26 protein yielded the production of antibodies with affinity primarily to the larger T2+T3 peptide, which represents the mid- region of OMP26 (AA 45–145).
Reactivity of rat anti-OMP26 antibodies to OMP26 peptides
The antibody responses against the OMP26 protein and the OMP26 peptides in the rat were measured using ELISA and SPR and the IPP/IT and IP immunization regimens ().
Figure 5. Antibody reactivities to the OMP26 peptides of sera from rats immunized with OMP26 protein and non-immune DA rats, using the IPP/IT (Panel A, C) and IP (Panel B, D) immunization regimens and assayed by ELISA (6A, B) and SPR (Panel C, D). Immune and non-immune sera collected from 3–5 rats were pooled and assayed in duplicate for antibody binding against the whole OMP26 protein and peptides. Antibody reactivities are expressed as IgG concentration in μg/ml in ELISA and Resonance Units (RU) in SPR. No specific antibodies to OMP26 peptides were detected in non-immune sera at the lowest sample dilution (1:25) by ELISA.
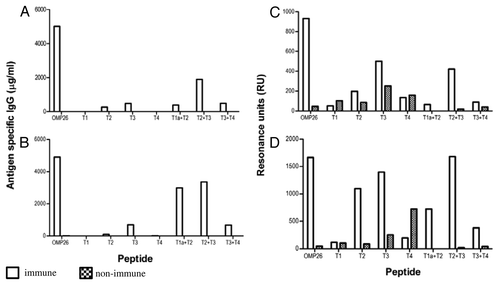
In the rats immunized IPP/IT, a predominant response was observed against the T2+T3 peptide using ELISA, and minor reactivities were observed against the T2, T3, T1a+T2 and the T3+T4 peptides (). For animals immunized IP, the ELISA results again showed a substantial response against the T2+T3 peptide (). In addition, similar response was observed against the T1a+T2 peptide with substantially less reactivity stimulated in response to T3 and T3+T4 peptides.
In general, reactivity of rat serum assessed using SPR was greater and thus resulted in greater overall reactivity to the OMP26 peptides than observed with the ELISA.
Serum from rats immunized IPP/IT demonstrated substantial reactivity against T2+T3 peptide and lesser absolute responses were observed against the T2 and T3 peptides in comparison to the non-immune sera (). In animals immunized IP, the SPR reactivity provided even greater responses by the rat serum antibodies with the predominant response observed to the T2+T3 peptide. Substantial responses were also observed against the T2, T3, T1a+T2 and T3+T4 peptides (). Therefore, as observed with mice, the response induced in rats by immunization with OMP26 yielded antibodies with greater affinity to the mid-region of the protein.
Reactivity of chinchilla anti-OMP26 antibodies to OMP26 peptides
The antibody responses against the OMP26 protein and the OMP26 peptides in the chinchilla after subcutaneous (SC) immunization were also measured using SPR and the results are shown in . Chinchilla anti-OMP26 antibodies exhibited the greatest overall reactivity to the T2+T3 peptide. Peptides T2, T3 and T4 showed lesser reactivity, while the T3+T4 peptide exhibited the lowest level of reactivity among the panel of peptides. Little antibody reactivity was observed to peptides T1 and T1a+T2.
Relative reactivity score
In order to summarize the data across species and immunization regimes, a relative reactivity score was calculated against the OMP26 response. The OMP26 response was assigned a relative reactivity score of 5 and the responses of the other OMP26 peptides were ranked against the OMP26 reactivity such that a response to an individual peptide that was 10–20% of the reactivity to OMP26 protein was scored as 1, 20–40% as 2, 40–60% as 3, 60–80% as 4, 80–100% as 5. No significant reactivity was detected against the T1 or T4 peptides regardless of species or immunization regime, although the chinchilla did demonstrate some reactivity to T2. Some reactivity was observed in response to all other peptides, with the T2+T3 peptide demonstrating the greatest activity (). Therefore, in terms of identification of potential B-cell epitope(s) within OMP26, antibodies against the mid-region of the protein consistently yielded the greatest reactivity, regardless of rodent species or immunization regime.
Figure 7. Summary of relative reactivity score was calculated against the OMP26 response. The OMP26 response was assigned a relative reactivity score of 5 and the responses of the other OMP26 peptides were scored against the OMP26 reactivity such that a response of 10–20% was scored as 1, 20–40% as 2, 40–60% as 3, 60–80% as 4, 80–100% as 5.
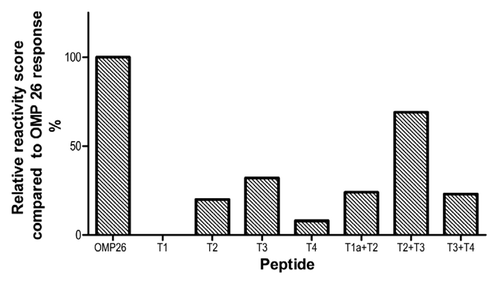
Discussion
NTHi is recognized as a significant human pathogenCitation24 and has become the focus of attention toward the development of an effective vaccine against NTHi infections. Previous investigations have identified the efficacy of OMP26 as a potential vaccine antigen.Citation16-Citation18 The current study examined the immunological recognition of T- and B-cell epitopes within truncated OMP26 to further characterize this vaccine candidate.
A series of overlapping peptides was constructed to localize the immunologically important regions for the OMP26. These peptides utilized the amino acid sequence of OMP26 identified in the strain NTHi-289 and included the 23-amino acid leader peptide. Previous studies have shown that the inclusion of the leader peptide and signal sequence in the recombinant OMP26 increased efficacy in a rodent model of acute respiratory infection.Citation17
In the current study, each OMP26 peptide overlapped the preceding and/or subsequent peptide by 6–11 amino acid residues. The OMP26 peptide constructs consisted of four overlapping peptides (T1-T4) of approximately 50 amino acids in length that spanned the entire OMP26 sequence. In addition, combination peptides of approximately 100 amino acids in length (T1a+T2 to T3+T4) were designed to include the adjacent regions of each of the T1-T4 peptides. This design increased the opportunity for detection of all immunologically relevant regions within OMP26 in our analysis and thus provides the first detailed molecular insight into the basis of immune reactivity to OMP26.
In the rat, lymphocyte proliferative responses following priming with OMP26 protein showed strong antigen specific responses to both T4 and the T3+T4 peptides with the magnitude of the T3+T4 peptide exceeding that of the T4 alone. However, individually, T2 and T3 failed to elicit a significant proliferative response. It is concluded that T-cell recognition is enhanced in the shared amino acid region between T3 and T4 and T-cell epitopes for OMP26 are located between amino acid residues 95 to 197, specifically the overlapping amino acid region between T3 and T4, upstream of amino acid residue 140. In addition, an OMP26-primed proliferative response was observed to the combined peptide T2+T3 but not to the individual T2 or T3 peptides suggesting that T-cell epitopes for OMP26 may also occur in the shared amino acid region between T2 and T3 (amino acid residues 94 to 100). When rats were immunized with each of the OMP26 peptides individually, the only lymphocyte proliferative response observed to the OMP26 protein was for the T3+T4 peptide. This supports the identification of a T-cell epitope within the T3+T4 peptide which is most likely located in the overlapping region and may be conformational, although typically linear amino acid motifs are more readily recognized by the major histocompatibility complex (MHC) molecules.Citation25
Processing of the OMP26 protein in this study resulted in a peptide with similar flanking amino acid residues or similar conformation to that of the T3+T4 peptide, and presumably similar affinity for the MHC molecules. Lymphocytes primed with T4 and T2+T3 peptides did not prime any T-cell response, in contrast to the T3+T4 peptide-primed lymphocytes, despite proliferative responses to these peptides occurring in lymphocytes primed with the whole OMP26. Changes in the flanking amino acids and/or conformation of the T4 and the T2+T3 peptides may account for this failure and are of importance for subsequent vaccine designs utilizing epitopes.
Selection of T-cell epitopes may include competitive binding between the processed peptides contained within a single antigenic molecule for MHC molecules.Citation26 Thus, only the peptides with the highest affinity to MHC molecules are ultimately presented to T-cells and the competition would be void when inoculation uses only a single peptide.Citation25 The lack of apparent difference between the extent of proliferation generated by the T3+T4 peptide and intact OMP26 protein suggests that the T3+T4 region has highest affinity binding for MHC molecules in the current model.
Specificity of the epitope regions recognized by B- and T-cells vary and are dependent upon a number of factors including the host’s genetic background and route of immunization. Use of the two immunization regimes in this study has potentially presented the antigen to different sets of antigen presenting cells (APC)s for the primary encounter. Different APC populations have been postulated to process and present antigens differently and thus can account for differences in epitope recognition. Differences in B-cell epitope recognition following different routes of immunization may result from extracellular degradation at the site of immunization,Citation27 although, the T2+T3 region may also be resistant to the extracellular medium encountered by these two immunization routes resulting in the identical B-cell recognition patterns. The route of immunization can also alter both the hierarchical organization of B- and T-cell epitopes,Citation27-Citation29 as well as, the titer and affinity of antibody responses.Citation30 Use of multiple animal species (chinchilla, mice and rats) and immunization routes in the present study showed that the immunodominant B-cell epitopes of OMP26 are located toward the center of the molecule between amino acid residues 45–145 (T2+T3).
In this study, the T2+T3 peptide exhibited the highest reactivity in mouse and rat anti-OMP26 sera regardless of immunization regime. For the development of a peptide based vaccine, the T2+T3 peptide would be a lead candidate for a genetically diverse human population.
Following SC immunization of chinchillas, anti-OMP26 sera also strongly recognized peptides T2, T3 and T4. While antibody recognition levels to these peptide were not as high as those to the T2+T3 peptide. It does suggest additional B-cell epitopes within the T2, T3 and T4 regions.
Immune sera generated from rats immunized IP with OMP26 also demonstrated strong reactivity to the T1a+T2 peptide. Thus, apart from the T2+T3 region, additional B-cell epitopes may be located between amino acid residues 24 and 100.
The T1 peptide (amino acid residues 1–50) was not recognized by any immune sera from all three animal species tested and the T4 peptide (amino acid residues 140–197) was not recognized by the immune serum pools from both mice and rats. This lack of antibody reactivity suggests that the T1 and T4 regions were sequestered or inaccessible despite clear expression on the surface of NTHi cells being detected by flow cytometry. This finding is suggestive of less surface exposure of these regions and is consistent with evidence from previous studies showing a hydrophobic character exists at the N-terminal and C-terminal regions of OMP26.Citation17 The lack of response to T1 and T4 peptides suggest that these peptide regions are not immunogenic; however, conformational diversion by T2+T3 dominanceCitation31 or assay bias cannot be excluded. Assay bias in this study is unlikely as antibody recognition patterns obtained from both ELISA and SPR were mostly similar despite potential differences in the orientation of the bound peptides on the microtiter plates and biosensor chips.
Anti-OMP26 serum pools from all three animal species strongly recognized the T2+T3 region, but reactivities to the T2 or T3 region were also detected by SPR. Reactivity to the individual T2 and T3 regions are interesting and do not approach the level stimulated by the T2+T3 region. While additional epitopes cannot be excluded, it is likely that the anti-OMP26 sera were reactive to the shared amino acid region between the T2 and T3 regions.
Since the majority of B-cell epitopes are discontinuous in nature,Citation32,Citation33 it is also possible that these identified B-cell epitopes are conformational. The sequence within T2+T3 region may have brought amino acids into spatial proximity to form the conformation that resembles the OMP26 protein, and thus allowing antibodies raised against the whole protein to bind most efficiently.
Previous experiments involving immunolabeling of NTHi with antisera to OMP26 showed surface binding of gold-conjugated particles by transmission electron microscopy,Citation16 suggesting at least one surface exposed region on OMP26. Flow cytometry analysis revealed the binding of anti-T1, anti-T2, anti-T3 and anti-T4 to OMP26 on intact NTHi cells, indicating that within the T1 to T4, there were surface exposed regions. Interestingly, except for anti-T1a+T2 sera, antisera raised to T2+T3 and T3+T4 peptides did not bind to OMP26 expressed on NTHi cells. The T2+T3 and T3+T4 peptides were 100 and 102 acids in length respectively, while the T1a+T2 combination peptide was 76 amino acids in length and the other individual peptides were between 50 and 57 amino acids in length.
It is possible that the solubilized longer peptides (T2+T3, T3+T4) could be conformationally different to the native protein. As such, antibodies raised against these peptides could have recognized epitopes that were conformationally different from those of the native OMP26 protein, thus resulting in a failure to bind to NTHi cells. Again, this finding demonstrates the limitations of using long peptides as immunizing antigens. It is important to note that binding of antisera to the shorter individual peptides (T2, T3, T4) to OMP26 on the surface of NTHi cells occurred and further analysis will be needed to determine which particular location within the long peptide regions (T2+T3 and T3+T4) contributes to conformational changes.
Key target regions of the acquired immune responses for the NTHi vaccine candidate, OMP26, have been identified in this study. The regions covering amino acid residues 45–145 (T2+T3 region), and 95–197 (T3+T4 region) were the dominant B- and T-cell epitopes for OMP26, respectively. At least two potential T-cell epitopes for OMP26 were located within the T3+T4 region, one was within the T4 region, and another one was within the shared amino acids upstream of residue 140. The T-cell epitopes within the T3+T4 region potentially had the highest affinity binding for MHC molecules compared with other OMP26 regions, and contained a full-length sequence required for efficient T-cell activation. The identified B-cell epitopes were immunodominant across all animal species tested, and with all immunization regimens used. Additionally, these particular epitopes were expressed on the surface of NTHi cells. The significant outcomes from the present study provide a better understanding of the specificity of the host immune responses, both humoral and cell-mediated, to the NTHi vaccine candidate, OMP26. Significantly, knowledge of the key target regions identified will be of great benefit for the future design of a better whole protein, peptide and/or epitope based vaccine against NTHi infections.
Future studies are still needed to define the minimum epitope length required for B- and T-cell responses identified in this study. The significance of these responses in immune protection against NTHi infection also requires further investigation.
Materials and Methods
Bacterial strains, plasmids and growth conditions
This study used NTHi-289, a biotype I strain isolated from the sputum of a patient with chronic bronchitis.Citation16 Bacteria were grown at 37°C in 5% CO2 on brain heart infusion agar (Oxoid, UK) supplemented with 5% (v/v) defibrinated horse blood (BioMerleux, Australia).
Escherichia coli M15 (pREP4) (Qiagen, USA) was used as a host strain for the recombinant plasmid DNA. Transformed E. coli was propagated on Luria-Bertani (LB) (Life Technologies, USA) or in liquid LB supplemented with 100 μg/ml of ampicillin and 50 μg/ml of kanamycin. Bacteria were grown at 37°C and liquid cultures were aerated with shaking at 220–250 rpm in a BioLine Shaking Incubator (Edwards Instrument Company, Australia) unless stated otherwise. Plasmid pQE30 was purchased from Qiagen and purified using QIAprep Spin Miniprep columns (Qiagen) according to the manufacturer’s instruction.
OMP26 protein and peptides
OMP26 protein
The OMP26 protein used in this study was purified from a OMP26VTAL clone of NTHi-289. The 6× His-tagged recombinant protein contains all but the first 6 amino acids (MKNIAK) of the complete OMP26 protein, and performs in a similar manner to the complete protein in animal immunization studies, as previously reported.Citation17 The leader peptide of the recombinant protein is less efficiently cleaved by E. coli signal peptidases, therefore, use of the MKNIAK-negative clone results in higher yields of purified protein and thus, is an improvement on the original OMP26 (McGrath, unpublished data).
OMP26 peptides
A series of overlapping peptides was designed and constructed to span the entire sequence of OMP26, based on the amino acid sequence of the full length OMP26 from NTHi-289. The locations of the peptide regions are shown in .
T1 peptide
The T1 peptide, representing the first 50 amino acids of full-length OMP26, was synthesized by Auspep Pty., Australia. The synthesized peptide was purified by high performance liquid chromatography on a SuperspherR, 250–4, LiChroCART, 100 RP-18 column (Merck Serono, Australia) using an acetonitrile gradient of 0 to 70% and 0.1% trifluoroacetic acid as the mobile phase. The molecular weight, confirmed by mass spectral analysis, was 5344 Da.
Inclusion of the hydrophobic 23-amino acid signal sequence of OMP26 resulted in difficulty in the expression and purification of the T1 peptide, regardless of the E. coli expression system used. This phenomenon has been previously reported for short hydrophobic proteins.Citation34 Exclusion of this hydrophobic sequence in the peptide design resulted in the successful expression and purification of the T1a+T2 peptide (results not shown). To ensure that the immunological studies were not compromised, we did not attempt to improve solubility of the protein and utilized a commercially synthesized T1 peptide for the immunological studies.
T2, T3, T4, T1a+T2, T2+T3 and T3+T4 peptides
Peptides were constructed by cloning various OMP26 gene segments into a pQE30 expression vector. Gene segments were obtained by PCR amplification of genomic DNA of NTHi-289 using primer pairs as listed in . Recombinant plasmids were transformed into E. coli M15 (pREP4) and expression and purification of recombinant OMP26 peptides was performed in accordance with the manufacturer’s instructions (Qiagen). An overnight culture of E. coli M15 harboring recombinant pQE30 was diluted 1:50 in 400 ml of fresh, pre-warmed LB containing ampicillin and kanamycin and grown at 30°C with shaking until the OD600 reached 0.6–1.0. Expression of recombinant protein was then induced with 1 mM isopropylthio-β-galactoside (Invitrogen ™; Life Technologies) for 2 h at 30°C. Bacteria were harvested (5,000 g, 20 min at 4°C: Hettich Zentrifugen Universal 32 Centrifuge; Hettich, Germany) and the pellet was stored at -80°C until protein purification. For purification, the cell pellet was thawed on ice and resuspended with lysis buffer (50 mM NaH2PO4, 300 mM NaCl, 10 mM imidazole, pH 8.0) to give 25× concentration factor (e.g., 4 ml/100 ml culture). To prevent protein degradation, phenylmethylsulfonyl fluoride (PMSF) (Sigma-Aldrich, USA) was added at a final concentration of 0.1 mM. The cells were then treated with lysozyme (final concentration of 1 mg/ml; Sigma-Aldrich) for 30 min on ice, and subsequently sonicated (Branson Sonifier 250 micro tip; 20–40 W output: Branson Ultrasonics, USA) on ice in 6 × 10 sec bursts, with 20 sec cooling periods between each burst. After centrifugation (10,000 g, 30 min at 4°C), 4 ml of the cleared lysate was transferred to a 10 ml centrifuge tube containing 1 ml of 50% (w/v) nickel-nitriloacetic acid (Ni-NTA) slurry (Qiagen). The lysate-Ni-NTA mixture was rocked on ice for 60 min, followed by centrifugation at 1000 g for 3 min. The supernatant was discarded and the Ni-NTA agarose pellet was washed twice with 4 ml of wash buffer (50 mM NaH2PO4, 300 mM NaCl, 50 mM imidazole, pH 8.0). Recombinant proteins bound to Ni-NTA matrices were eluted 4 times with 0.5 ml of elution buffer (50 mM NaH2PO4, 300 mM NaCl, 250 mM imidazole, pH 8.0) and stored at 4°C prior to analysis. OMP26 peptide purity was determined using sodium dodecyl sulfate polyacrylamide gel electrophoresis (SDS-PAGE) (16% w/v). Removal of imidazole in the elution buffer was performed by dialyzing the pooled eluates (containing purified OMP26 peptides) against 1 L of wash buffer for 3 h, dialysis buffer (50 mM NaH2PO4, 300 mM NaCl, 10% (v/v) glycerol) for 1 h and then phosphate buffered saline (PBS) overnight at 4°C. Following dialysis, PMSF (final concentration of 0.1 mM) was added to the dialyzed protein solutions. The concentration of purified OMP26 peptides was determined by a micro bicinchoninic acid (BCA) kit (Thermo Scientific, USA) as per the manufacturer’s instructions. Amino acid sequences for the OMP26 peptides are shown in .
Table 2. Oligonucleotide primers used in PCR to construct OMP26 peptides
Table 3. Amino acid sequences of the OMP26 peptides
Immunization
Animals
Specific-pathogen free (SPF) male DA rats aged 8–10 weeks or BALB/c mice aged 6–8 weeks were purchased from Animal Resources Centre, Western Australia, Australia and maintained under SPF conditions with food and water accessible ad libitum. Monitoring of rats and mice in experimental infection assays was performed according to protocols approved by the University of Canberra Animal Ethics Committee, Canberra, Australia, until harvest, 21 d post immunization. Adult chinchillas (Chinchilla lanigera) were also included in this study and were examined using otoscopy or tympanometry prior to inclusion to confirm the absence of clinically recognizable signs of middle ear infection. Pre-immune serum samples, collected by cardiac puncture, were tested to determine the presence of pre-existing antibodies to NTHi-OMPCitation18 and only sero-negative animals were used in the study. All chinchilla experiments were conducted at Nationwide Children’s Hospital Research Institute, Columbus, Ohio. Chinchilla studies were approved by Nationwide Children's Hospital Research Institute’s Animal Care and Use Committee.
IPP/IT Immunization
Mucosal immunizations were performed for the mice and rats using an IPP/IT approach, as previously described.Citation9,Citation35,Citation36 Twenty-one days after the first immunization, animals were euthanized using pentobarbital sodium overdose and tissue harvest and blood collection were performed. Blood volumes of 0.5–1 ml (mice) or 8–10 ml (rats) were collected by cardiac puncture into plain 1ml or 10 ml blood collection tubes (Sarstedt, Germany). Sera were pooled by cohort, separated by centrifugation, then frozen and stored at -20°C until analysis.
SC immunization
Six adult chinchillas were immunized SC with 10 μg of OMP26 plus 10 µg of SBAS-4 (GSK, Belgium) in 100 µl along the rear flank three times at 30 d intervals. Ten days after receipt of the third dose, the animals were bled by cardiac puncture, serum separated, pooled and frozen at -80°C until subsequent analysis.
IP immunization
The immunization antigen was prepared by emulsifying a required amount of antigen with Incomplete Freund's Adjuvant (Sigma-Aldrich, USA). Animals were anaesthetized via halothane inhalation and antigen was administered IP (40 μg per dose to rats; mice, received 10 μg per dose). Antigens were injected on days 0 and 14. Sera were collected 21 d post-immunization, at euthanasia, and frozen at -20°C for subsequent analysis.
Antigen-specific lymphocyte proliferation assay
Spleens from euthanized rats and mice were aseptically removed, homogenized and suspended in cold sterile buffer [PBS containing 1mM CaCI2, 1mM MgCI2, 5% (v/v) fetal bovine serum (FBS) (JR Scientific, USA), 100 U of penicillin per ml, 100 μg of streptomycin per ml and 0.25 μg of amphotericin B per ml (Fungizone; Life Technologies)]. A single cell preparation was then obtained by passage of the suspension through a nylon sieve (Becton Dickinson, USA), after which, the suspension was centrifuged at 100 g for 10 min and the supernatant discarded. Lysis of red blood cells was performed by addition of 0.5ml 0.17M NH4Cl, pH 7.65 for 1 min and then diluted with 10 ml of the above buffer. Centrifugation was repeated (100 g, 10 min at room temperature) and cells were washed 3 times in the buffer. The final cell concentration was 106 cells/ml in the same buffer, with viability determined by 0.1% trypan blue exclusion using a hemocytometer. Cells were cultured in RPMI medium containing 0.01 M HEPES, pH 7.4 (Life Technologies); 5 × 10−5 M β-mercaptoethanol (BDH-Prolabo Chemicals, Australia) 2 mM L-glutamine (Life Technologies); 5% (v/v) FBS (JR Scientific) and penicillin-streptomycin-amphotericin B (Fungizone; Life Technologies). Ten-fold dilutions of antigens, peptides or Concanavalin A (1 μg/ml; Sigma-Aldrich) were dispersed into the wells of flat-bottom 96-well culture plates (Nunc; Thermo Scientific, USA). Blank control wells (without antigens) were also included in each run. A total of 2 × 105 cells were added to each well and plates were incubated at 37°C in 5% CO2. On day 3, cells were pulsed with 25 μl/well with (methyl -3H)-thymidine (aqueous solution, specific activity of 20 Ci/mmol; PerkinElmer, Australia), which was diluted with culture media at a ratio of 20 μl/ml. Cells were harvested onto a printed filter mat A 1450–421 at 18–20 h post-pulsing using a Tomtec Harvester 96 (Tomtec, USA). Radioactivity in the cells was measured in CPM via liquid scintillation (Wallac MicroBeta 1450 TriLux liquid scintillation counter; PerkinElmer). An average count for each specimen was calculated using triplicate cultures stimulated with each antigen or peptide. Similarly, blank control samples, lacking antigens were performed in triplicate. The experiments were repeated on four occasions. Cell proliferation was expressed as mean CPM ± standard deviation of antigen-stimulated cultures minus the CPM of antigen-free cultures. An additional antigen of E. coli protein components from a sham purification extraction was also included to control for the potential presence of trace components of E. coli proteins in the purified OMP26 peptide preparations. The concentration of E. coli proteins in these controls represented the maximum amount that may potentially exist in any purified OMP26 peptide preparation. For each antigen the proliferative response for this control was also deducted from the total lymphoproliferative response observed for each peptide to determine the antigen specific response due solely to the recombinant OMP26 peptides. Data were analyzed for statistical significance by a fully factorial analysis of variance (Macintosh Systat; Systat Software, USA) using log10-transformed data.
Antigen-specific ELISA
Immunogenicity of OMP26 peptides to induce antibody responses was examined by testing rat antipeptide sera, generated by immunization using individual OMP26 peptides, against the immunizing peptide and the whole OMP26 protein.Citation16 Antigen-specific ELISA were performed and standardized as previously described by Kyd, Cripps, Novotny, Bakaletz.Citation18 There was minimal background reactivity against an E.coli antigen control for both immune and non-immune animals.
SPR analysis
Serum and OMP26 protein and peptide interactions were assessed by SPR using the Biacore 3000 instrument (GE Healthcare Life Sciences, USA) as previously described.Citation37 The Biacore system measures label-free binding of an analyte from a continuous flow to an immobilized ligand in real time.Citation38 Changes in refractive index due to variation of the mass on the sensor chip, which results from the interaction of the analyte and ligand, is expressed in relative Resonance Units (RU).Citation39 In brief, reagent grade carboxymethylated dextran matrix (CM5) reagent grade sensor chips (GE Healthcare Life Sciences) were activated with 35µl 400mM N-ethyl-N'-(3-diethlyaminopropyl carbodiimide hydrochloride-100mM N-hydroxysuccinimide solution at a flow rate of 5µl/min. OMP26 protein or peptides, suspended in 10 mM sodium acetate, pH 4.5 were injected to immobilize approximately 0.1–0.2ng of OMP26 protein or peptides per mm2 chip surface followed by deactivation of unbound sites with 1.0 M ethanolamine-HCl, pH 8.5. Ten µl of each serum sample diluted 1:5 with HBS-EP buffer (0.01 M HEPES, pH 7.4, 0.15 M NaCl, 3 mM EDTA, 0.005% (v/v) Surfactant P20; GE Healthcare Life Sciences), was exposed to the immobilized protein or peptides at a flow rate of 5 µl/min. Between samples, the sensor surface chip was regenerated with 10 µl injection of 50 mM NaOH. The relative amount of antibody bound to each peptide was determined by comparison of the change of RU between sample injection cycles.
Flow cytometry
Flow cytometry was used to determine whether antibodies induced by immunization with OMP26 or peptides could bind to the surface of NTHi. To remove any potential E. coli antibodies that may have cross-reacted with NTHi, sera were pre-absorbed with E. coli prior to flow cytometry as previously described.Citation40
NTHi-289 was grown as previously described,Citation16 harvested and washed twice with sterile PBS by centrifugation (400 g, 10 min at room temperature). The bacterial cell pellets were subsequently incubated for 1 h at 37°C with 200 μl of pre-adsorbed sera from the immunized rats, which had been diluted 1:50 in PBS. Following incubation, the bacterial cells were washed twice with sterile PBS as before. Cells were then incubated with 200 μl of Alexa Fluor® 488 goat anti-rat IgG (Life Technologies) diluted 1:50 in PBS, pH 7.4, for 30 min at 37°C. After washing twice with sterile PBS, cells were re-suspended in 500 μl of PBS, pH 7.4 and analyzed on a Flow Epic® XL-MCL Flow Cytometer (Beckman Coulter, Australia).
Identification of surface exposed epitopes was determined by incubation of NTHi-289 with seven antisera developed by immunizing rats (see above) with individual OMP26 peptides. As a negative control, PBS replaced the antibody and a bacterial population of 8 × 104 bacteria was analyzed. Intact bacteria were incubated with a 1:50 dilution of antisera, revealed with Alexa Fluor® 488 goat anti-rat IgG and analyzed for fluorescent intensity by flow cytometry.
Abbreviations: | ||
APC | = | antigen presenting cell |
BCA | = | bicinchoninic acid |
CPM | = | counts per minute |
ELISA | = | enzyme-linked immunosorbent assay |
FBS | = | fetal bovine serum |
IPP/IT | = | intra-Peyer’s patches/intra-tracheal |
IP | = | intra-peritoneal |
MHC | = | major histocompatibility complex |
Ni-NTA | = | nickel-nitriloacetic acid |
NTHi | = | nontypeable Haemophilus influenzae |
OMP | = | outer membrane protein |
OMP26 | = | outer membrane protein 26 |
PBS | = | phosphate buffered saline |
PMSF | = | phenylmethylsulfonyl fluoride |
RU | = | Resonance Units |
SC | = | subcutaneous |
SDS-PAGE | = | sodium dodecyl sulfate polyacrylamide gel electrophoresis |
SPF | = | specific-pathogen free |
SPR | = | surface plasmon resonance |
Acknowledgments
The authors thank Penny Chapman for her editorial assistance in the preparation of the manuscript
Submitted
10/10/12
Accepted
10/25/12
Disclosure of Potential Conflicts of Interest
No potential conflicts of interest were disclosed.
References
- Munson RS Jr.. Haemophilus influenzae: surface antigens and aspects of virulence. Can J Vet Res 1990; 54:Suppl S63 - 7; PMID: 2193707
- Murphy TF, Bartos LC. Human bactericidal antibody response to outer membrane protein P2 of nontypeable Haemophilus influenzae. Infect Immun 1988; 56:2673 - 9; PMID: 2843467
- Neary JM, Yi K, Karalus RJ, Murphy TF. Antibodies to loop 6 of the P2 porin protein of nontypeable Haemophilus influenzae are bactericidal against multiple strains. Infect Immun 2001; 69:773 - 8; http://dx.doi.org/10.1128/IAI.69.2.773-778.2001; PMID: 11159967
- Yi K, Murphy TF. Mapping of a strain-specific bactericidal epitope to the surface-exposed loop 5 on the P2 porin protein of non-typeable Haemophilus influenzae. Microb Pathog 1994; 17:277 - 82; http://dx.doi.org/10.1006/mpat.1994.1073; PMID: 7536289
- Bakaletz LO, Leake ER, Billy JM, Kaumaya PTP. Relative immunogenicity and efficacy of two synthetic chimeric peptides of fimbrin as vaccinogens against nasopharyngeal colonization by nontypeable Haemophilus influenzae in the chinchilla. Vaccine 1997; 15:955 - 61; http://dx.doi.org/10.1016/S0264-410X(96)00298-8; PMID: 9261941
- Bakaletz LO, Kennedy B-J, Novotny LA, Duquesne G, Cohen J, Lobet Y. Protection against development of otitis media induced by nontypeable Haemophilus influenzae by both active and passive immunization in a chinchilla model of virus-bacterium superinfection. Infect Immun 1999; 67:2746 - 62; PMID: 10338477
- Bakaletz LO. Peptide and recombinant antigens for protection against bacterial middle ear infection. Vaccine 2001; 19:2323 - 8; http://dx.doi.org/10.1016/S0264-410X(00)00522-3; PMID: 11257356
- Webb DC, Cripps AWA. A P5 peptide that is homologous to peptide 10 of OprF from Pseudomonas aeruginosa enhances clearance of nontypeable Haemophilus influenzae from acutely infected rat lung in the absence of detectable peptide-specific antibody. Infect Immun 2000; 68:377 - 81; http://dx.doi.org/10.1128/IAI.68.1.377-381.2000; PMID: 10603411
- Kyd JM, Dunkley ML, Cripps AW. Enhanced respiratory clearance of nontypeable Haemophilus influenzae following mucosal immunization with P6 in a rat model. Infect Immun 1995; 63:2931 - 40; PMID: 7622215
- Hotomi M, Yamanaka N, Saito T, Shimada J, Suzumoto M, Suetake M, et al. Antibody responses to the outer membrane protein P6 of non-typeable Haemophilus influenzae and pneumococcal capsular polysaccharides in otitis-prone children. Acta Otolaryngol 1999; 119:703 - 7; http://dx.doi.org/10.1080/00016489950180667; PMID: 10587005
- Sabirov A, Kodama S, Hirano T, Suzuki M, Mogi G. Intranasal immunization enhances clearance of nontypeable Haemophilus influenzae and reduces stimulation of tumor necrosis factor alpha production in the murine model of otitis media. Infect Immun 2001; 69:2964 - 71; http://dx.doi.org/10.1128/IAI.69.5.2964-2971.2001; PMID: 11292713
- Barenkamp SJ, Bodor FF. Development of serum bactericidal activity following nontypable Haemophilus influenzae acute otitis media. Pediatr Infect Dis J 1990; 9:333 - 9; http://dx.doi.org/10.1097/00006454-199005000-00006; PMID: 2352818
- Gu X-X, Tsai C-M, Ueyama T, Barenkamp SJ, Robbins JB, Lim DJ. Synthesis, characterization, and immunologic properties of detoxified lipooligosaccharide from nontypeable Haemophilus influenzae conjugated to proteins. Infect Immun 1996; 64:4047 - 53; PMID: 8926067
- DeMaria TF, Apicella MA, Nichols WA, Leake ER. Evaluation of the virulence of nontypeable Haemophilus influenzae lipooligosaccharide htrB and rfaD mutants in the chinchilla model of otitis media. Infect Immun 1997; 65:4431 - 5; PMID: 9353016
- Gu X-X, Rudy SF, Chu C, McCullagh L, Kim HN, Chen J, et al. Phase I study of a lipooligosaccharide-based conjugate vaccine against nontypeable Haemophilus influenzae. Vaccine 2003; 21:2107 - 14; http://dx.doi.org/10.1016/S0264-410X(02)00768-5; PMID: 12706701
- Kyd JM, Cripps AW. Potential of a novel protein, OMP26, from nontypeable Haemophilus influenzae to enhance pulmonary clearance in a rat model. Infect Immun 1998; 66:2272 - 8; PMID: 9573117
- El-Adhami W, Kyd JM, Bastin DA, Cripps AW. Characterization of the gene encoding a 26-kilodalton protein (OMP26) from nontypeable Haemophilus influenzae and immune responses to the recombinant protein. Infect Immun 1999; 67:1935 - 42; PMID: 10085039
- Kyd JM, Cripps AW, Novotny LA, Bakaletz LO. Efficacy of the 26-kilodalton outer membrane protein and two P5 fimbrin-derived immunogens to induce clearance of nontypeable Haemophilus influenzae from the rat middle ear and lungs as well as from the chinchilla middle ear and nasopharynx. Infect Immun 2003; 71:4691 - 9; http://dx.doi.org/10.1128/IAI.71.8.4691-4699.2003; PMID: 12874350
- Murphy TF, Apicella MA. Antigenic heterogeneity of outer membrane proteins of nontypable Haemophilus influenzae is a basis for a serotyping system. Infect Immun 1985; 50:15 - 21; PMID: 3876283
- Weinberg GA, Lehmann D, Tupasi TE, Granoff DM. Diversity of outer membrane protein profiles of nontypable Haemophilus influenzae from children from Papua New Guinea and the Philippines. Rev Infect Dis 1990; 12:Suppl 8 S1017 - 20; http://dx.doi.org/10.1093/clinids/12.Supplement_8.S1017; PMID: 2270398
- van Alphen L, Caugant DA, Duim B, O’Rourke M, Bowler LD. Differences in genetic diversity of nonecapsulated Haemophilus influenzae from various diseases. Microbiology 1997; 143:1423 - 31; http://dx.doi.org/10.1099/00221287-143-4-1423; PMID: 9141705
- Porras O, Caugant DA, Lagergård T, Svanborg-Edén C. Application of multilocus enzyme gel electrophoresis to Haemophilus influenzae. Infect Immun 1986; 53:71 - 8; PMID: 3522433
- Kaumaya PT, Kobs-Conrad S, Seo YH, Lee H, VanBuskirk AM, Feng N, et al. Peptide vaccines incorporating a ‘promiscuous’ T-cell epitope bypass certain haplotype restricted immune responses and provide broad spectrum immunogenicity. J Mol Recognit 1993; 6:81 - 94; http://dx.doi.org/10.1002/jmr.300060206; PMID: 7508238
- Sunakawa K, Takeuchi Y, Iwata S. [Nontypeable Haemophilus influenzae (NTHi) epidemiology]. Kansenshogaku Zasshi 2011; 85:227 - 37; PMID: 21706841
- Williams KM, Bigley EC 3rd, Raybourne RB. Identification of murine B-cell and T-cell epitopes of Escherichia coli outer membrane protein F with synthetic polypeptides. Infect Immun 2000; 68:2535 - 45; http://dx.doi.org/10.1128/IAI.68.5.2535-2545.2000; PMID: 10768941
- Adorini L, Nagy ZA. Peptide competition for antigen presentation. Immunol Today 1990; 11:21 - 4; http://dx.doi.org/10.1016/0167-5699(90)90006-U; PMID: 2405874
- Todryk SM, Kelly CG, Lehner T. Effect of route of immunisation and adjuvant on T and B cell epitope recognition within a streptococcal antigen. Vaccine 1998; 16:174 - 80; http://dx.doi.org/10.1016/S0264-410X(97)00183-7; PMID: 9607027
- Thatte J, Rath S, Bal V. Analysis of immunization route-related variation in the immune response to heat-killed Salmonella typhimurium in mice. Infect Immun 1995; 63:99 - 103; PMID: 7806391
- Gutierro I, Hernández RM, Igartua M, Gascón AR, Pedraz JL. Influence of dose and immunization route on the serum Ig G antibody response to BSA loaded PLGA microspheres. Vaccine 2002; 20:2181 - 90; http://dx.doi.org/10.1016/S0264-410X(02)00146-9; PMID: 12009271
- Partidos CD, Vohra P, Stanley CM, Howard CR, Steward MW. Induction of systemic immune responses to orally administered synthetic peptides. Vaccine Res 1995; 4:113 - 20
- Scheerlinck J-PY, DeLeys R, Saman E, Brys L, Geldhof A, De Baetselier P. Redistribution of a murine humoral immune response following removal of an immunodominant B cell epitope from a recombinant fusion protein. Mol Immunol 1993; 30:733 - 9; http://dx.doi.org/10.1016/0161-5890(93)90144-Z; PMID: 7684820
- Partidos CD, Salani FB, Ripley J, Steward MW. Deconstructing the antigenic profile of a protective epitope from measles virus fusion protein using overlapping peptides. Vaccine 1999; 18:321 - 4; http://dx.doi.org/10.1016/S0264-410X(99)00211-X; PMID: 10506658
- Van Regenmortel MHV. Antigenicity and immunogenicity of synthetic peptides. Biologicals 2001; 29:209 - 13; http://dx.doi.org/10.1006/biol.2001.0308; PMID: 11851317
- Lajmi AR, Wallace TR, Shin JA. Short, hydrophobic, alanine-based proteins based on the basic region/leucine zipper protein motif: overcoming inclusion body formation and protein aggregation during overexpression, purification, and renaturation. Protein Expr Purif 2000; 18:394 - 403; http://dx.doi.org/10.1006/prep.2000.1209; PMID: 10733895
- Murphy TF, Kyd JM, John A, Kirkham C, Cripps AW. Enhancement of pulmonary clearance of Moraxella (Branhamella) catarrhalis following immunization with outer membrane protein CD in a mouse model. J Infect Dis 1998; 178:1667 - 75; http://dx.doi.org/10.1086/314501; PMID: 9815219
- Kyd J, John A, Cripps A, Murphy TF. Investigation of mucosal immunisation in pulmonary clearance of Moraxella (Branhamella) catarrhalis. Vaccine 1999; 18:398 - 406; http://dx.doi.org/10.1016/S0264-410X(99)00262-5; PMID: 10519928
- Novotny LA, Jurcisek JA, Pichichero ME, Bakaletz LO. Epitope mapping of the outer membrane protein P5-homologous fimbrin adhesin of nontypeable Haemophilus influenzae. Infect Immun 2000; 68:2119 - 28; http://dx.doi.org/10.1128/IAI.68.4.2119-2128.2000; PMID: 10722609
- Malmqvist M. Epitope Mapping by Label-Free Biomolecular Interaction Analysis. Methods 1996; 9:525 - 32; http://dx.doi.org/10.1006/meth.1996.0060; PMID: 8812708
- Johne B. Epitope mapping by surface plasmon resonance in the BIAcore. Mol Biotechnol 1998; 9:65 - 71; http://dx.doi.org/10.1007/BF02752698; PMID: 9592769
- Groeneveld K, van Alphen L, Voorter C, Eijk PP, Jansen HM, Zanen HC. Antigenic drift of Haemophilus influenzae in patients with chronic obstructive pulmonary disease. Infect Immun 1989; 57:3038 - 44; PMID: 2789192